Abstract
Forty unicyanobacterial cultures of the genus Scytonema, isolated from terrestrial habitats worldwide (Europe, America, Southeast Asia, Australia and Africa), were investigated. The pigments detected were four phycobiliproteins (allophycocyanin, phycocyanin, phycoerythrocyanin and phycoerythrin), scytonemin, six carotenoids (β-carotene, canthaxanthin, echinenone, isozeaxanthin, myxoxanthophyll and zeaxanthin) and14 different mycosporines, including shinorine, stanierine and asterina-330. Cluster analyses indicated three groups among the isolates which were not related to their biogeographical origins. The first was composed of isolates with allophycocyanin and phycocyanin; β-carotene, canthaxanthin, echinenone and zeaxanthin; and scytonemin but no MAAs. These isolates had disc-shaped or elongated cells with blue-green cytoplasm and slight meristematic zones. The second group comprised isolates containing allophycocyanin, phycocyanin and phycoerythrin; β-carotene, canthaxanthin, echinenone, isozeaxanthin and myxoxanthophyll; and also both MAAs and scytonemin. Here, the cells were elongated with brown-violet cytoplasm and marked meristematic zones. The third group comprised isolates with allophycocyanin, phycocyanin and phycoerythrocyanin; β-carotene, canthaxanthin and echinenone; shinorine-type MAA, but no scytonemin. The cells were elongate with blue-green cytoplasm and marked terminal meristematic zones, and the organisms grew in dimly lit places. Multivariate statistical analyses were performed to identify compounds with especial value for taxonomy. Allophycocyanin, phycocyanin, phycoerythrin and phycoerythrocyanin; scytonemin; isozeaxanthin, myxoxanthophyll and zeaxanthin; and shinorine, asterina-330 and mycosporine 13, showed statistically significant variations among the three groups of Scytonema unicyanobacterial cultures. These results, and the fact that the biomarkers studied were conserved, even during long-term cultivation, suggest that these compounds can be used as chemotaxonomic markers in taxonomic and phylogenetic studies of Scytonema.
Introduction
The taxonomy of cyanobacteria has had to face many problems, particularly at the species level, because of the lack of morphological features, as well as biochemical, ecophysiological, genetic and ultrastructural characters, that permit organisms to be clearly defined (Anagnostidis & Komárek, Citation1985; Komárek & Anagnostidis Citation1989; Komárek Citation2010a). Specific or generic names given to organisms in numerous experimental works have sometimes been used almost arbitrarily, and often no descriptions or illustrations are given to justify the identification of the species worked with. This prevents many cytomorphological, ecophysiological, biochemical and genetic data from being used for taxonomic purposes. It also leads to incongruities between phylogenetic trees, simply because species have not been properly identified. This in turn can delay reassessment of the current classification system (Hoffmann et al., Citation1999; Komárek, Citation2010a, b).
In fact, cytomorphological, ecophysiological, biochemical and genetic data are rarely gathered for large collections of strains, nor are these kinds of data used to check the classification of cyanobacteria (Hoffmann et al., Citation1999). Biochemical characteristics and their stability are important criteria in taxonomic analyses of cyanobacteria (Komárek, Citation2005). Pigment composition is useful for the classification of cyanobacteria. First, there are the biliproteins, which (as well as chlorophyll a) have a well-established function in harvesting light. Secondly, there are carotenoid pigments, whose roles are more diverse: (1) harvesting light energy for photosynthesis; (2) preventing the destruction of activated chlorophyll by oxygen in the light; and (3) dispersing excess heat energy (Demmig-Adams & Adams, Citation2000). Thirdly, a variety of compounds, including mycosporine-like amino acids (MAAs) and scytonemins, occur in cyanobacteria as pigments that screen out harmful ultraviolet radiation (Garcia-Pichel & Castenholz, Citation1991; Squier et al., Citation2004). The presence or absence of phycobiliproteins may be of some taxonomic value according to Suda et al. (Citation2002), who showed that a separation of closely related Planktothrix agardhii strains into phycoerythrin (PE) and non-phycoerythrin groups was supported by DNA–DNA hybridization values. On the other hand, the Microcoleus chthonoplastes-group of strains has been shown to be easily distinguished by carotenoid and MAA content (Karsten & Garcia-Pichel, Citation1996). Likewise, Hodgson et al (Citation2004) revealed statistically significant correlations between a number of cyanobacterial morphotypes (Chondrocystis cf. dermochroea, Gloeocapsa cf. compacta) and their carotenoid and scytonemin content.
The taxonomy of genus Scytonema, characterized by false ramifications and the presence of heterocytes, which are commonplace in aerophytic habitats, particularly in tropical areas, is being studied with a multiphasic approach (Hoffmann et al. Citation1999). It uses a combination of characteristics ranging from classic botanical methods to molecular biology and biochemical methods, to ascertain whether those species recognized upon the basis of their morphology and ecology, actually represent different taxonomic units.
The aim of the present work was to investigate whether phycobiliproteins, scytonemins, carotenoids and MAAs, when used as chemotaxonomic markers, can help not only to explore the relationships between pigment composition and Scytonema morphotypes, but also to review the taxonomy of the Scytonema genus from a biochemical viewpoint. We therefore studied the composition and distribution of phycobiliproteins, scytonemins, carotenoids and MAAs in 40 unicyanobacterial cultures of Scytonema, isolated from various terrestrial habitats worldwide (Europe, America, Southeast Asia, Australia and Africa).
Materials and methods
Organisms, growth conditions and morphology
The 40 branched filamentous heterocystous cyanobacteria used in this study are listed in . The GU1 isolate was purchased from Sammlung von Algenkulturen at the University of Göttingen, Germany. The B1 unicyanobacterial culture was obtained from the Institut Pasteur, Paris, France, and the GE2 isolate was sent by B. Büdel (University of Kaiserslautern, Germany). The remaining cyanobacteria were isolated from material collected in Australia, Florida, Greece, Luxemburg, Nepal, Papua New Guinea, Portugal, Singapore, Spain, Switzerland and Thailand. They were identified to genus according to the cyanobacterial literature (Geitler, Citation1932; Komárek & Anagnostidis, Citation1989) and maintained in BG11 medium (Rippka et al. Citation1979) without nitrogen at constant temperature (20ºC) and with continuous light (20 μmol m−2 s−1). All the cultures were grown for two months under these culture conditions before conducting the analyses. Most isolates grew as biofilms adhering to the bottom of culture flasks.
Table 1. Origins of the cyanobacterial isolates investigated and their possible assignment as botanical species on the basis of morphology, morphometry and ecology.
Morphological and morphometric studies were carried out on specimens collected from the cultures in exponential growth phase. Cell sizes were measured using a light microscope (LM: Leica DMR, Leica Microsystems, Wetzlar, Germany) and summarized as the mean ± standard deviation (n = 30).
Phycobiliprotein extraction and characterization
In order to estimate phycobiliprotein content, washed cell pellets were resuspended to a total volume of 6.0 ml with a solution of 0.01 M sodium phosphate at pH 7.0, containing 0.15 M NaCl (buffered saline). Cell suspensions were transferred to Beckman Coulter tubes (Fullerton, CA, USA), quick-frozen in liquid nitrogen, capped with Parafilm® (Wertheim, Germany), and stored at −20°C in the dark before disruption. Thawed cell suspensions were immersed in a stirred ice bath and sonicated with the microtip of a Heat Systems model W185D sonifier (Heat Systems-Ultrasonics, Plainview, New York) operating at power setting 6. Each aliquot of cells was exposed to sonication for 4 min, with alternating 1-min periods of sonication and cooling. Examination by LM (Olympus BX41 microscope) demonstrated that this procedure disrupts cells. Over the cell concentration ranges used in these experiments, the amount of each water-soluble phycobiliprotein released into the buffered saline solution was directly proportional to the number of filaments that were fragmented (Bennett & Bogorad, Citation1973). Sonicates were transferred to Beckman polycarbonate tubes and centrifuged at 4°C and 81 000 × g (at an average radius of centrifugation) for 45 min. This procedure pellets the membrane fragments of cells, which contain chlorophyll a and most carotenoids. No phycobiliproteins could be detected in the membrane fragments when resuspended in buffered saline. Instead, phycobiliproteins and other soluble proteins were contained in the supernatant (crude extract). The upper 5.5 ml of the supernatants were carefully withdrawn and their absorption spectra determined from 730 to 500 nm. Insertion of the optical densities of crude extracts at 560, 570, 620 and 650 mn into the equations derived from the extinction coefficients of the purified phycobiliproteins permitted phycoerythrin, phycoerytrocyanin, phycocyanin and allophycocyanin concentrations in crude extracts to be ascertained in accordance with Bennett & Bogorad (Citation1973).
Scytonemin and chlorophyll a extraction and determination
Cyanobacterial samples were homogenized for pigment extraction with a glass potter in ice-cold 100% acetone p.a. (pro analysi). All the steps were performed under dim light. Following extraction, homogenates were centrifuged at 10 000 × g for 5 min and the supernatants evaporated under a stream of nitrogen and re-dissolved in 50–100 µl 100% acetone. Then 20 µl sample volumes were injected into a high-performance liquid chromatography (HPLC) column through a Rheodyne injection valve (Cotati, CA, USA). Pigments were determined by HPLC using a Waters Associates set-up (Milford, MA, USA), including a 600E gradient module with a system controller and a Model 991 photodiode array detector (Karsten & Garcia-Pichel, Citation1996). Separations were performed in a stainless steel Merck (Darmstadt, Germany) LiChrospher RP 18 cartridge (5 µm packing; 125 mm × 4 mm internal diameter) after passing through a Merck RP 18 guard column (4 mm × 4 mm internal diameter) filled with the same material as in the main cartridge. Scytonemin and chlorophyll a were eluted by a binary gradient system of helium-degassed solvents and were monitored at 436 nm. Absorption spectra were recorded every second between 350 and 680 nm directly on the HPLC-separated peaks. The mobile phases of Solvent A (distilled water) and Solvent B (acetonitrile–methanol–tetrahydrofuran, 75 : 15 : 10, v/v) were regulated at a flow rate of 1.5 ml min−1 by the following gradient programme: 0–15 min for a linear increase from 15% Solvent A to 100% Solvent B, and 15–25 min at 100% Solvent B. Both pigments were identified by their characteristic absorption maxima in a mixture of Solvents A and B with the appropriate retention time. This identification was evaluated by co-chromatography using a commercially available chlorophyll a as a standard (Sigma) and with scytonemin isolated from a pure culture of the well-characterized cyanobacterium Scytonema javanicum (Garcia-Pichel & Castenholz, Citation1991). Pigments were quantified by peak areas using the integrator of the Waters 991 photodiode array detector. For each compound, a molar extinction coefficient [E (mM−1 cm−1)] was determined, which corresponded to the chromatographic conditions used: scytonemin, 27.9, chlorophyll a, 91.7.
Carotenoid extraction and characterization
Cell suspensions (about 20 ml) of Scytonema were collected by filtration using Whatman GF/F glass fibre filters (0.2 µm pore size) and were homogenized with ice-cold 100% acetone p.a. When isolates grew as biofilms adhering to the bottom of culture flasks, 1–2 cm2 pieces of biofilm were used for pigment extraction in ice-cold 100% acetone. All the steps were performed in dim light. Following extraction, homogenates were centrifuged at 10 000 × g for 5 min in a Sigma 2-16K centrifuge with a 12141-H rotor (Sigma, Osterode am Harz, 18 Germany). The supernatants of these pigment extracts were evaporated under a stream of nitrogen and were re-dissolved in 50–100 µl 100% acetone. Next, 20 µl sample volumes were injected into a HPLC column through a Rheodyne Model 5041 injection valve. Pigments were determined by HPLC using a Waters Associates HPLC set-up, including a 600E gradient module with a system controller and a Model 991 photodiode array detector. Separations were performed in a stainless steel Merck LiChrospher RP 18 cartridge (5 µm packing; 125 mm × 4 mm internal diameter) after passing through a Merck RP 18 guard column (4 mm × 4 mm internal diameter) filled with the same material as in the main cartridge.
Pigments were eluted by a binary gradient system of helium-degassed solvents and were monitored at 436 nm. Absorption spectra were recorded every second between 400 and 600 nm directly on the HPLC-separated peaks. The mobile phases of Solvent A (distilled water) and Solvent B (acetonitrile–methanol–tetrahydrofuran, 75 : 15 : 10, v/v) were regulated at a flow rate of 1.5 ml min−1 by the following programme: 0–15 min for a linear increase from 15% Solvent A to 100% Solvent B, and 15–25 min at 100% Solvent B.
Individual pigments were tentatively identified by their characteristic absorption maxima in a mixture of Solvents A and B in the appropriate retention time (Table S1). This identification was evaluated by co-chromatography with commercially available standards, namely canthaxanthin, β-carotene and chlorophyll a from Sigma (Berlin, Germany) or with the pigments isolated from the cultures of characterized cyanobacteria Synechocystis sp. 6803 (echinenone; Schubert et al., Citation1993) and Spirulina platensis NIVA-CYA 136 / 2 (zeaxanthin, myxoxanthophyll; Aakermann et al., Citation1992). Isozeaxanthin was identified by comparing the absorption spectrum with that of the identical zeaxanthin and by performing co-chromatography with a pigment extract made from the lichen Peltigera sp. (Leisner et al., Citation1993). Pigments were quantified by peak areas using the integrator of the waters 991 photodiode array detector. A molar extinction coefficient was determined for each compound, which corresponded to the chromatographic conditions used (Table S1).
MAA extraction and characterization
Cell suspensions (about 20 ml) of Scytonema were collected by filtration using Whatman GF/F glass fibre filters (pore size, 0.2 µm) and were directly used for qualitative and quantitative MAA determinations. As elsewhere, when isolates grew as biofilms, 1–2 cm2 pieces of biofilm were used for MAA determinations. Samples were extracted for 1.5–2 h in the dark in screw-capped centrifuge vials filled with 1 ml 25% aqueous methanol (v/v), and were incubated in a water bath at 45ºC. After centrifugation at 5000 × g for 5 min, 800 µl of the supernatants were evaporated to dryness under vacuum (Speed Vac Concentrator SVC 100H, Thermo Quest Scientific Equipment Group, Egelsbach, Germany). Dried samples were re-dissolved in 800 µl of 100% methanol and vortexed for 30 s. After passing through a 0.2 µm membrane filter, samples were analysed with the Waters HPLC system in accordance with the method of Nakamura et al. (Citation1982), which was modified as follows: MAAs were separated in a stainless steel Knauer (Berlin, Germany) Spherisorb RP-8 column (5 µm, 250 mm × 4 mm internal diameter) protected with an RP-8 guard cartridge (20 mm × 4 mm internal diameter). Mobile phases were 25% aqueous methanol (v/v) plus 0.1% acetic acid (v/v) in water (Solvent 1), or 5% aqueous methanol (v/v) plus 0.1% acetic acid (v/v) in water (Solvent 2). Solvent 1 was run isocratically at a flow rate of 0.3 ml min−1, while a flow rate of 0.5 ml min−1 was used for Solvent 2. MAAs were detected at 335 nm and the absorption spectra were recorded every second between 290 and 400 nm directly on the HPLC-separated peaks (Table S2). Identification was performed by spectra, retention time and, in some cases, by co-chromatography, with standards extracted from marine organisms Lissoclinum patella (ascidian), Palythoa tuberculosa (zoanthid), coral trout lenses, and the red macroalga Porphyra sp., which were kindly provided by Dr D. Karentz.
The extraction procedure for the MAAs did not dissolve or degrade any intracellular chlorophyll a. Therefore after extraction and centrifugation the remaining pellets were homogenized with ice-cold 100% acetone p.a. under dim light to extract and quantify the chlorophyll a content as a reference parameter. Following homogenization, samples were centrifuged at 5000 × g for 5 min. Supernatants were transferred to graduated tubes and made up to 3 ml with 100% acetone, and chlorophyll a was quantified spectrophotometrically according to Jeffrey & Humphrey (Citation1975).
Statistical analysis
Hierarchical cluster analyses were performed to identify the associations among pigments and among isolations. For these analyses, we applied the L2 or Euclidean distance measure and single linkage. Data were standardized to improve normality. Cluster analyses were carried out with STATA version 11.2 software (StataCorp LP., TX, USA).
In order to summarize the variation in pigment composition and the taxonomy characters among isolates, a principal component analysis (PCA), as implemented in STATA version 11.2, was applied to pigment concentrations and the morphometric and morphological characters. For the phycobiliproteins, scytonemin, carotenoids and MAAs, differences among the three groups of Scytonema cultures distinguished in the cluster analysis were evaluated by non-parametric Kruskal–Wallis tests (P < 0.05). Statistical analyses were performed using SPSS software (Chicago, IL, USA).
For the aerophytic, atmophytic, cavernicolous, corticolous, edaphic, epilithic, epizoic habitat data, differences between the habitat groups of the Scytonema cultures were evaluated by non-parametric Kruskal–Wallis tests (P < 0.05). Statistical analyses were performed using SPSS.
Results
Morphological observations
The minimum and maximum cell widths observed in the Scytonema-like isolates were 4.15 μm and 19.46 μm, respectively, whereas the minimum filament and maximum widths noted were 5.95 μm and 26.94 μm, corresponding to the measures of the NG9 and SW2 cyanobacterial isolates, respectively (). The cells had different forms, varying from disc-shaped (length/diameter < 1) to elongate (length/diameter > 1) (). Within those with elongate cells, two distinct groups were recognized. Group I consisted of 15 isolates with a brown-violet cytoplasm (reflecting the presence of phycoerythrin), while Group II contained strains with a blue-green cytoplasm (reflecting a lack of phycoerythrin). The Group II isolates contained two distinct subgroups. Subgroup A consisted of seven isolates with marked terminal meristematic zones, whereas subgroup B contained seven isolates with slight terminal meristematic zones.
Phycobiliproteins
The following phycobiliproteins were present: allophycocyanin, phycocyanin, phycoerythrin and phycoerythrocyanin (). Allophycocyanin and phycocyanin were recorded in all the isolates included in this study. Phycoerythrin was detected in GU1, TH1, TH2, TH5, A1, SI2, NG1, NG2, NG3, NG4, NG6, NG10, NG11, N2 and GE2. Finally, phycoerythrocyanin was detected in isolates F1, F3, B1, GR1, GR2, GE1 and SP1.
Fig. 1. Percentages of total phycobiliproteins in different Scytonema cultures. Scytonema isolate codes are given in .
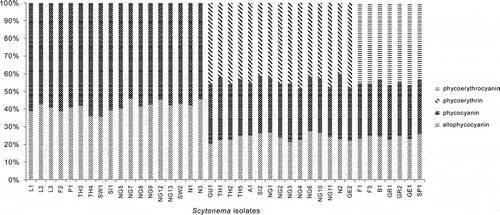
Up to three phycobiliproteins were detected among the Scytonema isolates. Allophycocyanin and phycocyanin were always seen together, and sometimes with either phycoerythrin or phycoerythrocyanin. The two latter compounds were never found together in the same sample. Phycobiliprotein content, expressed as mg of each of the phycobiliproteins per mg chlorophyll a (mg PB × mg−1 chl a; Table S3), varied among isolates and ranged from 1.98 to 9.55. The phycoerythrocyanin values slightly changed if compared with the rest of the phycobiliproteins.
Cluster analyses based on all the pigments analysed () revealed that Scytonema isolates formed three groups () and the distributions of the phycobiliproteins agreed with the overall clustering. Thus, the first group comprised those isolates containing only allophycocyanin and phycocyanin, while the second included those isolates with allophycocyanin, phycocyanin and phycoerythrin. The third group comprised isolates storing allophycocyanin, phycocyanin and phycoerythrocyanin. The phycobiliprotein content – of allophycocyanin, phycocyanin, phycoerythrin and phycoerythrocyanin – showed statistically significant variations among the three groups (Kruskal–Wallis, P < 0.01; ).
Fig. 2. Cluster analysis of Scytonema isolates according to their pigment characteristics. Scytonema isolate codes are given in .
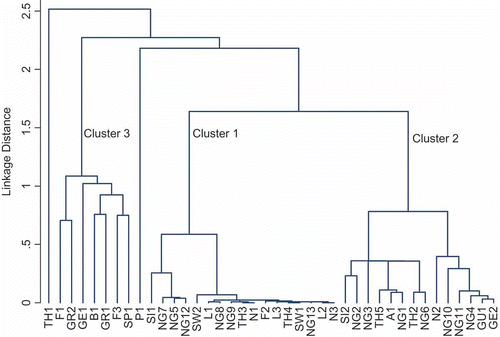
Table 2. Characteristics of the three groups of Scytonema distinguished by cluster analysis of biochemical data ().
Table 3. Comparison of the phycobiliproteins, scytonemin, carotenoids and mycosporine-like amino acid data (mean ± S.D.), evaluated by non-parametric Kruskal–Wallis tests (P < 0.05) among the three groups of Scytonema cultures distinguished by cluster analysis ().
Scytonemin
Scytonemin was detected in 33 of the 40 Scytonema cultures studied (), irrespective of whether MAAs were present or not. The specific scytonemin contents, expressed as absorbance at 370 nm per mg chl a, ranged between 0.1 and 6.69. The groups established according to the cluster analysis () differed in their scytonemin content. Group 1 comprised isolates containing scytonemin but lacking MAAs; Group 2 included those isolates with scytonemin and MAAs, except shinorine; and Group 3 contained isolates lacking scytonemin, where only shinorine was detected. Scytonemin concentrations showed statistically significant variations among the three (Kruskal–Wallis, P < 0.001; ).
Table 4. Mycosporine-like amino acid compounds and scytonemin in different isolates of Scytonema. The numbers represent the order of leaving the HPLC column. The specific MAA contents are expressed as absorbance (at 335 nm) mg−1 chl a. The specific scytonemin contents are expressed as absorbance (at 370 nm) mg−1 chl a. – = not detected. For Scytonema isolate codes, see .
Carotenoids
The following carotenoids were present: β-carotene, canthaxanthin, echinenone, isozeaxanthin, myxoxanthophyll and zeaxanthin (). β-carotene, canthaxanthin and echinenone were observed in all the isolates. Isozeaxanthin and myxoxanthophyll were found in GU1, TH1, TH2, TH5, A1, SI2, NG1, NG2, NG3, NG4, NG6, NG10, NG11, N2 and GE2. Zeaxanthin was present in L1, L2, L3, F2, P1, TH3, TH4, SW1, SI1, NG5, NG7, NG8, NG9, NG12, NG13, N1, SW2 and N3.
Fig. 3. Percentages of total carotenoids in different Scytonema cultures. Scytonema isolate codes are given in .
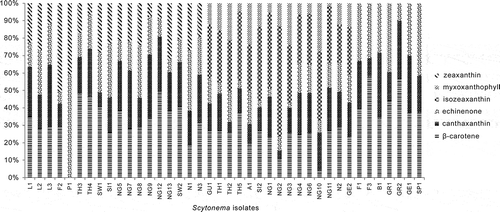
Each Scytonema isolate contained up to five carotenoids. When four carotenoids were present, the fourth was always zeaxanthin (added to the β-carotene, canthaxanthin and echinenone present in all isolates) but when there were five carotenoids, the extra two were isozeaxanthin and myxoxanthophyll. The carotenoids : chl a ratio (by molecular weight; Table S4) varied considerably, from 0.02 to 182.4. Some isolates always showed high concentrations of a specific pigment. For instance, isolates GU1, TH1, TH2, TH5, A1, SI2, NG1, NG2, NG3, NG4, NG6, NG10, NG11, N2 and GE2 had relatively high isozeaxanthin content.
Group 1 contained isolates with four carotenoids (β-carotene, canthaxanthin, echinenone and zeaxanthin). Group 2 comprised the five-carotenoid isolates (i.e. with β-carotene, canthaxanthin, echinenone, isozeaxanthin and myxoxanthophyll), while Group 3 comprised the remaining isolates, containing only β-carotene, canthaxanthin and echinenone. The carotenoids : chl a ratio (for isozeaxanthin, myxoxanthophyll and zeaxanthin only) showed statistically significant variations among the three groups (Kruskal–Wallis, P < 0.001; ).
Mycosporine-like amino acid compounds
Of the 40 Scytonema unicyanobacterial cultures studied, 22 had MAAs. The detected amounts were 0.1–4.52 nm × mg−1 of chl a. HPLC analysis detected 14 different MAAs, of which three were shinorine, stanierine and asterina-330 (). The remaining 11 MAAs were new. The most frequent water-soluble substance in our isolates was asterina-330 (= ‘mycosporine 8’ in ), which was observed in 15 different cultures. It was followed by shinorine, present in seven cultures. Next came the MAAs that we number mycosporine-13 and -1, found in four Scytonema cultures, and finally, there were ten mycosporines (3, 4, 5, 6 [stanierine], 7, 9, 10, 11, 12 and 14), all of which were present in just one culture. Eighteen isolates (L1, L2, L3, F2, P1, TH3, TH4, SW1, SI1, NG5, NG7, NG8, NG9, NG12, NG13, N1, SW2 and N3) lacked MAAs. Where MAAs were detected, an isolate usually had either one (F1, F3, B1, GR1, GR2, GE1, GU1, A1, NG6, SP1 and GE2) or two types (TH2, TH5, SI2, NG2, NG3, NG4, NG10, NG11). Less frequently, greater numbers were found: there were three different MAAs in isolate TH1, four in N2 and six in NG1.
Again, the characterization of the isolates according to their MAAs agreed with the overall classification by cluster analysis, on the basis of all the pigment data (). Group 1 contained isolates without MAAs, Group 2 comprised isolates containing any of the MAAs except shinorine, while Group 3 comprised isolates in which only shinorine was detected. The shinorine, asterina-330 and mycosporine 13 concentrations showed statistically significant variations among the three groups (Kruskal–Wallis, P < 0.05; ).
Habitat correlations and PCA
The shinorine, phycoerythrocyanin and scytonemin concentrations showed statistically significant variations among the habitat groups of the Scytonema cultures (Kruskal–Wallis; P < 0.05) ().
Table 5. Comparison of the phycobiliproteins, scytonemin, carotenoids and mycosporine-like amino acid data among the different habitat groups of Scytonema, evaluated by non-parametric Kruskal–Wallis tests (P < 0.05). The habitats represented among the 40 isolates were aerophytic (2), epilithic (7), cavernicolous (2), atmophytic (18), edaphic (4), and corticolous (7).
The first three axes of the PCA captured 52.74% of total variance (PC1, PC2 and PC3 accounted for 23.97%, 15.43% and 13.34%, respectively). These axes were linear functions of the pigment contents and morphometry of the Scytonema cultures. Despite there being a considerable overlap of the sample scores from the three groups distinguished by cluster analysis, the pigment assemblages from the isolates of Groups 1 and 2 tended to be positioned higher on the first PCA axis than those from the isolates of Group 3 (), and this axis 1 appeared to capture a gradient of carotenoids and scytonemin. The second axis was mainly associated with phycobiliproteins, morphometry and MAAs. The third axis (not shown) separated the isolates from Groups 1 and 3 from those of Group 2.
Discussion
This study differed from previous studies in that it examined the contributions of the phycobiliproteins, scytonemins, carotenoids, and MAAs, morphology and morphometry in grouping Scytonema strains into putative taxa, identifying those biochemical variables that have taxonomic relevance.
The biochemical analysis of Scytonema identified two more-or-less independent trends. The first trend was mainly related to carotenoid composition, arranging isolates in the order Group 1 (β-carotene, canthaxanthin, echinenone and zeaxanthin), Group 2 (β-carotene, canthaxanthin, echinenone, isozeaxanthin and myxoxanthophyll) and Group 3 (β-carotene, canthaxanthin and echinenone). The second trend was mainly determined by phycobiliproteins, with compositions varying from Group 1 (allophycocyanin and phycocyanin), through Group 2 (allophycocyanin, phycocyanin and phycoerythrin), to Group 3 (allophycocyanin, phycocyanin and phycoerythrocyanin). Pigment characteristics agree in separating Scytonema into three clusters, which will probably be worth taxonomic recognition in the future. These groupings do not conflict with groupings based on traditional morphological and morphometric characters, such as the kind of meristematic zone and cell shape.
According to the tree diagram obtained from the cluster analyses of all pigments (), three patterns of incidence occur in the Scytonema isolates studied here, and the various individual phycobiliproteins, scytonemin, carotenoids and mycosporine-like amino acid compounds can be used as chemotaxonomic markers for these three groups (). The first group comprises isolates with allophycocyanin and phycocyanin, β-carotene, canthaxanthin, echinenone and zeaxanthin; they lack MAAs, but contain scytonemin. In these isolates, the filament cells are either disc-shaped or elongate, with blue-green cytoplasm and slight meristematic zones. The second group comprises isolates with allophycocyanin, phycocyanin and phycoerythrin, and β-carotene, canthaxanthin, echinenone, isozeaxanthin and myxoxanthophyll; both MAAs and scytonemin are also present. Here the filament cells are elongate with brown-violet cytoplasm and marked meristematic zones. The third group included isolates with allophycocyanin, phycocyanin and phycoerythrocyanin, and β-carotene, canthaxanthin and echinenone; they also contain shinorine-type MAA, but lack scytonemin. The filament cells are elongate, with blue-green cytoplasm and marked terminal meristematic zones.
The pigment data for the Scytonema cultures suggest that, in terrestrial habitats, cyanobacteria receive so much photosynthetically active radiation (PAR) and ultraviolet radiation (UV) that major cellular efforts are invested in synthesizing a range of PAR and UV screening compounds. Thus, a higher relative abundance of scytonemins, carotenoids and MAAs was found than in the cyanobacteria we have studied from other habitats. Scytonemins are responsible for UV protection and stress responses (Dillon et al., Citation2002), thus preventing UV-dependent bleaching of chlorophyll and DNA damage (Garcia-Pichel & Castenholz, Citation1991). Carotenoids have also been implicated in bright-light photoprotection of cyanobacteria (Castenholz & Garcia-Pichel, Citation2000) and elevated carotenoid content has been reported for UV-resistant cyanobacteria (Lakatos et al., Citation2001). Furthermore, UV-screening MAAs have been detected in terrestrial environments (asterina-330, shinorine). Cyanobacteria with UV screening capabilities appear to have an advantage over those phototrophs without them.
Of the four phycobiliproteins detected, allophycocyanin and phycocyanin appeared in all the Scytonema isolates, which agrees with the results of Glazer & Bryant (Citation1975), whereas phycoerythrocyanin and phycoerythrin were less frequent. Phycoerythrin was observed in only 15 of the 40 isolates studied, despite Bogorad (Citation1975) stating that this protein is found in most cyanobacteria. Phycoerythrocyanin was found in only seven isolates, consistent with Bryant et al.’s (Citation1976) view that the distribution of this phycobiliprotein among cyanobacteria is limited.
Scytonemin was detected in 33 of the 40 Scytonema cultures, irrespective of whether or not mycosporines were present, which was in agreement with Garcia-Pichel & Castenholz (Citation1993). When both types of pigment were recorded, a large number of different types of mycosporines was frequently noted, supporting the idea that these substances have a major protective role in Scytonema in isolates exposed to high light intensities. No isolates were found to lack both mycosporines and scytonemin, as found also by Garcia-Pichel & Castenholz (Citation1993). The frequent presence of scytonemin in our Scytonema isolates implies that although scytonemin is synthesized during periods of high UV, it is likely to persist as a permanent extracellular pigment by providing an enduring UV screening capability; this capability is consistent with some field studies reporting that scytonemin content does not correlate directly with rapid changes in UV irradiance (Pentecost, Citation1993). In contrast, studies of live mats have shown not only seasonal changes in scytonemin concentrations in response to solar irradiance (Karsten et al., Citation1998), but also interspecific differences in scytonemin production in response to UV (Pentecost, Citation1993). Despite this, the global effect of UV in the pigment data for the Scytonema cultures appeared to be positive for some species as it selected for UV-resistant cyanobacteria (Karsten et al., Citation1998; Sheridan, Citation2001).
Of the six carotenoids observed, β-carotene was detected in all the isolates and in large amounts, as in the cultured isolates studied by Goodwin (Citation1980). Canthaxanthin was also present in all the Scytonema isolates, despite Hirschberg & Chamovitz (Citation1994) believing it to be a less frequent carotenoid. Echinenone occurred in all the isolates, as reported by Hirschberg & Chamovitz (Citation1994), who classified it as exclusively cyanobacterial. Isozeaxanthin was observed in 15 Scytonema cultures, whereas Goodwin (Citation1980) and Hirschberg & Chamovitz (Citation1994) did not encounter it. Myxoxanthophyll also appeared in 15 Scytonema cultures; Goodwin (Citation1980) considered it characteristic of free life forms. The amounts of myxoxanthophyll are strongly light-dependent (Palińska et al., Citation2011) and could possibly be below the limit of detection in some samples, even though the strain could have the potential for its synthesis. Zeaxanthin was recorded in only 18 out of the 40 Scytonema cultures, which does not agree with Hirschberg & Chamovitz (Citation1994) or Goodwin (Citation1980), who classified it as one of the most common carotenoids.
In their review of carotenoids in cyanobacteria, Hirschberg & Chamovitz (Citation1994) listed 15 cyanobacterial carotenoids as common. Thus, the limited number of carotenoids found in our study (only six) may itself be a character indicating a fairly close relationship between the strains examined. Analyses of isolates from many genera have demonstrated that the qualitative carotenoid composition is a suitable biochemical criterion for classifying cyanobacteria and species from established genera frequently show the same composition (Palińska et al., Citation2011). However our studies of Scytonema-like isolates provide exceptions, since the strains exhibited different carotenoid compositions, falling into three main carotenoid groups (though β-carotene, canthaxanthin and echinenone were always present). Here carotenoid composition could possibly provide criteria for subdividing the genus.
Of the 40 Scytonema cultures studied, 22 had MAAs, the most frequent being asterina-330, which was found in 15 strains. Shinorine was the next most frequent, occurring in seven cultures, and has already been reported in Scytonema by Gröniger et al. (Citation2000). In contrast, the predominant MAA in Microcoleus is shinorine (Karsten & Garcia-Pichel, Citation1996).
No relationship was found in Scytonema between pigment composition and the biogeographical origins of strains. The ability of a single species to exist over a broad geographical range and a wide range of environmental conditions has been demonstrated in some seaweeds, e.g. Ectocarpus siliculosus (Bolton, Citation1983; Thomas & Kirst, Citation1991), where genotypic variation is thought to underlie local adaptation to different habitats. The striking differences in pigment composition between Scytonema populations, and hence in their physiological ecology, clearly suggests genetically determined adaptation. The same cannot be said of their habitat for which a statistically significant relationship was found, showing that the isolates containing phycoerythrocyanin, shinorine and scytonemin originate from dimly lit environments, such as caves and similar places. The presence of phycoerythrocyanin in isolates growing in dimly lit surroundings helps photosynthesis despite the low radiation impact of their surroundings. This is because it is part of an energy transfer chain, together with other phycobiliproteins, which can transfer energy to eventually reach chlorophyll a at an efficiency rate of nearly 100% (Porter et al., Citation1978; Searle et al., Citation1978).
Chemotaxonomy aims to find correlations between chemical evidence and systematic classifications based on other characters, such as morphological and phylogenetic ones. Although morphological characters have a chemical foundation, chemical characters often have no morphological expression (Liaaen-Jensen, Citation1979), but in Scytonema there were numerous statistically significant relationships between pigment composition and morphological features. Therefore, morphology and chemical data combine in this genus to provide strong, corroborated hypotheses of relationships, each species grouping possessing greater abundances of particular pigments. Allophycocyanin, phycocyanin, phycoerythrin, phycoerythrocyanin, isozeaxanthin, myxoxanthophyll, zeaxanthin; shinorine, asterina-330, mycosporine 13 and scytonemin all showed statistically significant variations among the three groups of Scytonema cultures and have potential as chemotaxonomic tools to improve the current classification system, which is based on morphology alone.
Judging by the pigments we detected, the use of carotenoids and screening pigments to survive high PAR and UV intensities is well developed in these cyanobacteria and may confer a competitive advantage through the preferential inhibition of eukaryotic algae (cf. the studies of periphyton by Vinebrooke & Leavitt, Citation1996). In particular, our analyses identified species that synthesize scytonemin and confirm that UV performs a structuring function in terrestrial environments (Wetzel, Citation2001). Consequently, cyanobacteria were the dominant phototrophs in these environments. Our study demonstrated that cyanobacteria can optimally exploit the light environment of different habitats through adaptive pigment strategies. It is possible that cyanobacteria have possessed this biochemical advantage from an early stage in their evolution, when neither oxygen nor a protecting ozone layer was present, and that they were not restricted to dim environments in order to exploit short wavelength radiation absorption (Häder, Citation1997). This suggests that, for terrestrial cyanobacteria, any increase in the UV receipt (as a result of the ozone hole) will represent a minor challenge, as their capacity to produce screening compounds will continue to protect them and offer a biochemical advantage over other phototrophs, in agreement with Hodgson et al. (Citation2004).
Finally, we should acknowledge that our studies were conducted exclusively on strains obtained from culture collections. It is known that cultured cyanobacteria often show only slight similarity to natural populations, as some phenotypic traits are apparently not expressed in the culture environment. This problem has led to the misidentification of cultures (Palińska et al., Citation1996; Wilmotte & Herdman, Citation2001) and can lower the value of phylogenetic reconstructions and other analyses of evolutionary interrelationships. Furthermore, the physiological state and culture conditions of organisms can influence the intracellular concentrations of many organic compounds. However, it is important to note that the biomarkers we studied were conserved even after long periods in culture (in some cases for more than 15 years).
Supplementary information
The following supplementary material is accessible for this article, accessible via the Supplementary Content tab on the article’s online page at http://dx.doi.org/10.1080/09670262.2013.836682
Table S1. Retention time, the molar extinction coefficients (E) determined at 436 nm, and the absorbance maxima for the separated carotenoids and chlorophyll a.
Table S2. Retention time and the absorbance maxima for separated MAAs.
Table S3. Phycobiliprotein compounds in different isolates of Scytonema. The specific contents are expressed as milligrams each of the phycobiliproteins per milligrams chlorophyll a (mg PB × mg–1 chl. a). Scytonema isolates codes are given in Table 1.
Table S4. Carotenoid Carotenoid : chlorophyll a ratios by molecular weight in different Scytonema cultures. Scytonema isolates codes are given in Table 1.
Acknowledgements
We are grateful to Séneca Foundation for support and H. Warburton for her assistance in the English version of the text. We also wish to thank two anonymous reviewers for their comments.
References
- Aakermann, T., Skulberg, O.M. & Liaaen-Jensen, S. (1992). A comparison of the carotenoids of strains of Oscillatoria and Spirulina (Cyanobacteria). Biochemical Systematics and Ecology, 20: 761–769.
- Anagnostidis, K. & Komárek, J. (1985). Modern approach to the classification system of cyanophytes. 1– Introduction. Algological Studies, 38/39: 291–302.
- Bennett, A. & Bogorad, L. (1973). Complementary chromatic adaptation in a filamentous blue-green alga. Journal of Cell Biology, 58: 419–435.
- Bogorad, L. (1975). Phycobiliproteins and complementary chromatic adaptation. Annual Review of Plant Physiology, 26: 369–401.
- Bolton, J.J. (1983). Ecoclinal variation in Ectocarpus siliculosus (Phaeophyceae) with respect to temperature growth optima and survival limits. Marine Biology, 73: 131–138.
- Bryant, D.A., Glazer, A.N. & Eiserling, F.A. (1976). Characterization and structural properties of the major biliproteins of Anabaena sp. Archives of Microbiology, 110: 61–75.
- Castenholz, R.W. & Garcia-Pichel, F. (2000). Cyanobacterial responses to UV radiation. In: The ecology of Cyanobacteria: their diversity in time and space (Whitton, B.A. & Potts, M. editors), 591–611. Kluwer Academic Publishers, Dordrecht.
- Demmig-Adams, B. & Adams W.W. (2000). Photosynthesis – harvesting sunlight safely. Nature, 403: 371.
- Dillon, J.G., Tatsumi, C.M., Tandingan, P.G. & Castenholz, R.W. (2002). Effect of environmental factors on the synthesis of scytonemin, a UV-screening pigment, in a cyanobacterium (Chroococcidiopsis sp.). Archives of Microbiology, 177: 322–331.
- Garcia-Pichel, F. & Castenholz, R. (1991). Characterization and biological implications of scytonemin, a cyanobacterial sheath pigment. Journal of Phycology, 27: 395–409.
- Garcia-Pichel, F. & Castenholz, R. (1993). Occurrence of UV-absorbing, mycosporine-like compounds among cyanobacterial isolates and an estimate of their screening capacity. Applied and Environmental Microbiology, 59: 163–169.
- Geitler, L. (1932). Cyanophyceae. In: Kryptogamen-Flora von Deutschland, Österreichs und der Schweiz, vol. 14 (Rabenhorst, L., editor), 1–1119. Akademische Verlagsgesellschaft, Leipzig.
- Glazer, A.N. & Bryant, D.A. (1975). Allo-phycocyanin B (λmax 671, 618 nm): a new cyanobacterial phycobiliprotein. Archives of Microbiology, 104: 15–22.
- Goodwin, T.M. (1980). Carotenoids in higher plants. In The biochemistry of the carotenoids. 2nd ed., Vol. 1, Plants (Goodwin, T.W., editor), 143–203. Chapman & Hall, London.
- Gröniger, A., Sinha, R.P., Klisch, M. & Häder, D.-P. (2000). Photoprotective compounds in cyanobacteria, phytoplankton and macroalgae-a database. Journal of Photochemistry and Photobiology B: Biology, 58: 115–122.
- Häder, D.-P. (1997). Effects of solar UV-B radiation on aquatic ecosystems. In Plants and UV-B. Responses to environmental change (Lumsden, P.J., editor), 171–194. Cambridge University Press, Cambridge.
- Hirschberg, J. & Chamovitz, D. (1994). Carotenoids in Cyanobacteria. In The molecular biology of Cyanobacteria (Bryant, D.A., editor), 559–579. Kluwer Academic Publishers, Dordrecht.
- Hodgson, D.A., Vyverman, W., Verleyen, E., Sabbe, K., Leavitt, P.R., Taton, A., Squier, A.H. & Keely, B.H. (2004). Environmental factors influencing the pigment composition of in situ benthic microbial communities in east Antarctic lakes. Aquatic Microbial Ecology, 37: 247–263.
- Hoffmann, L., Asencio, A.D. & Schmit, C. (1999). Towards a taxonomic revision of the genus Scytonema: morphological, biochemical and molecular approaches. Algological Studies, 94: 173–174.
- Jeffrey, S.W. & Humphrey, G.F. (1975). New spectrophotometric equations for determining chlorophylls a, b, c1 and c2 in higher plants, algae and natural phytoplankton. Biochemie und Physiologie der Pflanzen, 167: 191–194.
- Karsten, U. & Garcia-Pichel, F. (1996). Carotenoids and mycosporine-like amino acid compounds in members of the genus Microcoleus (Cyanobacteria): a chemosystematic study. Systematic and Applied Microbiology, 19: 285–294.
- Karsten, U., Maier, J. & Garcia-Pichel, F. (1998). Seasonality in UV absorbing compounds of cyanobacterial mat communities from an intertidal mangrove flat. Aquatic Microbial Ecology, 16: 37–44.
- Komárek, J. (2005). The modern classification of cyanoprokaryotes (Cyanobacteria). Oceanological Hydrobiological Studies 34(3): 5–17.
- Komárek, J. (2010a). Modern taxonomic revision of planktic nostocacean cyanobacteria: a short review of genera. Hydrobiologia, 639: 231–243.
- Komárek, J. (2010b). Recent changes (2008) in cyanobacteria taxonomy based on a combination of molecular background with phenotype and ecological consequences (genus and species concept). Hydrobiologia, 639: 245–259.
- Komárek, J. & Anagnostidis, K. (1989). Modern approach to the classification system of cyanophytes 4 – Nostocales. Algological Studies, 56: 247–345.
- Lakatos, M., Bilger, W. & Büdel, B. (2001). Carotenoid composition of terrestrial Cyanobacteria: response to natural light conditions in open rock habitats in Venezuela. European Journal of Phycology, 36: 367–375.
- Leisner, J.M.R., Bilger, W., Czygan, F.C. & Lange, O.L. (1993). Lipophilous carotenoids of cyanobacterial lichens from different habitats, including an extreme desert site. Cryptogamic Botany, 4: 74–82.
- Liaaen-Jensen, S. (1979). Carotenoids – a chemosystematic approach. Pure and Applied Chemistry, 51: 661–675.
- Nakamura, H., Kobayashi, J. & Hirata, Y. (1982). Separation of mycosporine-like amino acids in marine organisms using reversed-phase high-performance liquid chromatography. Journal of Chromatography, 250: 113–118.
- Palińska. K.A., Liesack, W., Rhiel, E. & Krumbein, W.E. (1996). Phenotype variability of identical genotypes: the need for a combined cyanobacterial taxonomy demonstrated on Merismopedia-like isolates. Archives of Microbiology, 166: 224–233.
- Palińska, K.A., Deventer, B., Hariri, K. & Łotocka, M. (2011). A taxonomic study on Phormidium-group (cyanobacteria) based on morphology, pigments, RAPD molecular markers and RFLP analysis of the 16S rRNA gene fragment. Fottea, 11: 41–55.
- Pentecost, A. (1993). Field relationships between scytonemin density, growth and irradiance in cyanobacteria occurring in low illumination regimes. Microbial Ecology, 26: 101–110.
- Porter, G., Tredwell, C.J., Searleg, F. & Barber, J. (1978). Picosecond time-resolved energy transfer in Porphyridium cruentum. Part I. In the intact alga. Biochimica et Biophysica Acta, 501: 232–245.
- Rippka, R., Deruelles, J., Waterbury, J.B., Herdman, M. & Stanier, R.Y. (1979). Generic assignments, strain histories and properties of pure cultures of cyanobacteria. Journal of General Microbiology, 111: 1–61.
- Schubert, H., Fulda, S. & Hagermann, M. (1993). Effects of adaptation to different salt concentrations on photosynthesis and pigmentation of the cyanobacterium Synechocystis sp PCC 6803. Journal of Plant Physiology, 142: 291–295.
- Searle, G.F.W., Barber, J., Porter, G. & Tredwellc, J. (1978). Picosecond time-resolved energy transfer in Porphyridium cruentum. Part II. In the isolated light harvesting complex (phycobilisomes). Biochimica et Biophysica Acta, 501: 246–256.
- Sheridan, R.P. (2001). Role of ultraviolet radiation in maintaining the three-dimensional structure of a cyanobacterial mat community and facilitating nitrogen fixation. Journal of Phycology, 37: 731–737.
- Squier, A.H., Hodgson, D.A. & Keely, B.J. (2004). A critical assessment of the analysis and distributions of scytonemin and related UV-screening pigments in sediments. Organic Geochemistry, 35: 1221–1228.
- Suda, S., Watanabe, M.M., Otsuka, S., Mahakahant, A., Yongmanitchai, W., Nopartnaraporn, N., Liu, Y.D. & Day, J.G. (2002). Taxonomic revision of water-bloom-forming species of oscillatorioid cyanobacteria. International Journal of Systematic and Evolutionary Microbiology, 52: 1577–1595.
- Thomas, D.N. & Kirst, G.O. (1991). Salt tolerance of Ectocarpus siliculosus (Dillw.) Lyngb: Comparison of gametophytes, sporophytes and isolates of different geographic origin. Botanica Acta, 104: 26–36.
- Vinebrooke, R.D. & Leavitt, P.R. (1996). Effects of ultraviolet radiation on periphyton in an alpine lake. Limnology and Oceanography, 41: 1035–1040.
- Wetzel, R.G. (2001). Limnology: lakes and river ecosystems. Academic Press, London.
- Wilmotte, A. & Herdman, M. (2001). Phylogenetic relationships among the cyanobacteria based on 16S rRNA sequences. In Bergey’s manual of systematic bacteriology, 2nd ed. (Garrity, G.M., editor), 487–493. Springer, New York.