Abstract
A new family, genus and species in the new order Entwisleiales of the subclass Nemaliophycidae are described for a subtidal marine red alga (Entwisleia bella F.J. Scott, G.W. Saunders & Kraft) from Tasmania in Australia’s cool-temperate southeast. Thalli are uniaxial, the stout cells of the single central-axial filament bearing varying numbers of whorled periaxial cells and fascicles of determinant cortical filaments at their distal poles, as well as dense aggregates of secondary internodal filaments arising perpendicularly on basipetal rhizoids that issue from periaxial cells. Plants are dioecious, the spermatangia sessile on distal bearing cells of primary and secondary cortical filaments. Carpogonial branches are four- to eight-celled and directed outwardly from the thallus, and produce simple to highly branched laterals from subhypogynous cells. Presumed fertilization results in several gonimoblast initials being cut from the lateral surface of the carpogonium by vertical cross walls, the ensuing gonimoblasts forming a compact carposporophyte subtended by, but not mixed with, the sterile filaments on the carpogonial branch. Terminal carposporangia form across the surface layer of the gonimoblasts and a fusion cell is not produced. The thalli are particularly batrachospermaceous in vegetative and reproductive morphology, a highly anomalous phenomenon considering that the Batrachospermales is an order totally confined to freshwater habitats. Despite the morphological and anatomical similarities to Batrachospermum and closely related genera, the consensus of multigene sequence analyses indicates that the new genus forms a sister clade to the Colaconematales, even though the members of that group differ in virtually every aspect of habit and anatomy from the gametophytic thalli of Entwisleia.
Introduction
With the completion of the last of Womersley’s six volumes on the marine algae of southern Australia (2004), four of which are devoted to the Rhodophyta, the enormous taxonomic richness of that long coastline has been comprehensively demonstrated. Nevertheless, there still remain regions and habitats that are under-explored and capable of yielding new and distinctive taxa. One area that has proved to be particularly rich in recent discoveries is the island state of Tasmania, where new taxa have primarily come from subtidal habitats that have been collected only sporadically by taxonomists. During the course of research for the macroalgal aspect of the Barcode of Life project headed by one of us (GWS), we have been making extensive collections in Tasmania and are unearthing a wealth of both cryptic and morphologically distinct species, the taxonomic status and phylogenetic positions of which are now being revealed by the molecular data that Saunders and colleagues are currently generating.
Among the most unusual of our recent findings was an infrequent component of the sublittoral seaweed flora at a locality southwest of Hobart, the capital city of Tasmania (). It was discovered there by the first author in 2010 and she has charted its seasonality, distribution and community structure over the past 3 years. Particularly intriguing aspects of this organism were its thallus architecture and male and female reproductive structures, as they all immediately suggested strong similarities to the exclusively freshwater genus Batrachospermum. Although subsequent molecular analyses have failed to support our initial hypothesis, as shown below, the anatomy of the alga is confirmed as being startlingly like Batrachospermum and related genera in the family Batrachospermaceae.
Fig. 1. The Australian state of Tasmania: (a), the Derwent Estuary region of southeastern Tasmania and (b), the site of the holotype and paratype collections of Entwisleia bella (arrow).
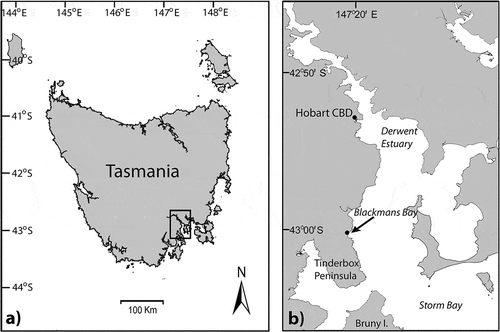
The work reported here was undertaken to investigate the morphology, anatomy and embryology of the newly discovered Tasmanian alga, and to determine its relationships and phylogenetic position. Preliminary analyses indicated that the new alga most likely belonged to the Nemaliophycidae, together with the exclusively freshwater orders Batrachospermales and Thoreales. Saunders & Hommersand (Citation2004) established the Nemaliophycidae for red algae with pit plugs characterized by two outer cap layers, but subsequent molecular investigation reduced the number of orders within it by transferring two, the Corallinales and Rhodogorgonales, to the subclass Corallinophycidae (Le Gall & Saunders, Citation2007). In their analyses, Le Gall & Saunders (Citation2007, ) recovered unequivocal support for the Nemaliophycidae sensu stricto and its constituent orders as monophyletic, but found little support for relationships among the orders except for an alliance between the Acrochaetiales and Palmariales. This portion of the florideophyte tree was one of four regions highlighted by Verbruggen et al. (Citation2010) as poorly supported and in need of additional sequence data and/or inclusion of novel lineages to facilitate phylogenetic resolution. Therefore, we have undertaken a five-gene study of the Nemaliophycidae, aiming to obtain the most robust indications yet of ordinal relationships within the Nemaliophycidae.
Materials and methods
Molecular methods
Samples for molecular investigation (Supplementary information, Table S1) were processed and DNA extracted following Saunders & McDevit (Citation2012). Sequence data were generated for the mitochondrial cytochrome c oxidase 1 gene barcode region (COI-5P) following Saunders & McDevit (Citation2012) and aligned using MacClade 4 (v. 4.06) for OSX (Maddison & Maddison, Citation2003). The primer pair used in each collection is available with the record on GenBank (Table S1). Neighbour-joining analyses (K2P corrected distances), as implemented in PAUP* (v. 4.0b10; Swofford Citation2003) in Geneious Pro (5.6.2; Drummond et al. Citation2009), were used to assess genetic species groups.
For phylogenetic analyses, an extended COI fragment was amplified using the standard forward DNA barcode primer (discussed previously) in combination with one of the reverse primers CoTR1 (5′–CCYARRAARTGCATIGGMA–3′) or CoTR2 (5′–CCYARRAARTGCATNGGCA–3′). The amplification products were sequenced with the respective PCR primers and the resulting data used to identify the best forward and reverse internal primers, facilitating the completion of full bidirectional reads (Saunders & Moore, Citation2013). In addition, data for the small (SSU) and large (LSU) subunit genes of the ribosomal cistron (Harper & Saunders, Citation2001), the nuclear elongation factor 2 (EF2) gene (Le Gall & Saunders, Citation2007) and the plastid RUBISCO large subunit (rbcL) (Vis et al., Citation2007) were generated following established protocols (Saunders & Moore, Citation2013). GenBank accession numbers are recorded in Table S1.
Seven alignments were constructed using MacClade 4 (v. 4.06) for OSX, with percentage completeness determined in gDAM (www.phycoweb.net/software/gDAM). These alignments included the five individual gene regions COI (35 taxa, 1232 aligned sites; 72% complete), SSU (35 taxa, 2782 aligned sites, 1030 excluded; 83% complete; Supplementary information, File 1), LSU (42 taxa, 5617 aligned sites, 2931 excluded; 99% complete; Supplementary information, File 2), EF2 (24 taxa, 1687 aligned sites; 57% complete), and rbcL (41 taxa, 1358 aligned sites; 96% complete). In addition, a ‘total’ combined alignment was made, comprising all taxa and regions (42 taxa, 8720 aligned sites; 84% complete), and a second combined alignment (31 taxa, 7033; 90% complete) that excluded EF2, which was a weakly represented region.
All phylogenetic alignments were subjected to both maximum likelihood (ML) and Bayesian analyses. For the single-gene alignments, parameters were first estimated (AIC) in Modeltest (v. 3.06; Posada & Crandall, Citation1998) as implemented in PAUP* through Geneious Pro on a MacPro (OS X version 10.6.8). The selected model was used to complete maximum-likelihood analyses in PHYML 3.0 (Guindon & Gascuel, Citation2003) with BIONJ used to designate the starting tree, the best of nearest-neighbour interchange (NNI) or subtree pruning and regrafting (SPR) branch-swapping options, and the tree topology, branch lengths and substitution rates optimized; data partitioning was not implemented. Branch support was estimated using the Shimodaira–Hasegawa-like (SH) approximate likelihood ratio test. MrBayes (v. 3.1.2; Huelsenbeck & Ronquist, Citation2001) was used to complete two independent trials (each with parallel runs) of Bayesian inference under a GTR+I+G model for all alignments. Parallel runs of four Markov chains were completed, with two million generations and sampling each 100 generations. The burn-in for each run was determined by plotting overall likelihood scores against generation, which established the stationary phase of each run for estimating the posterior probability (PP) distribution; the final estimate was based on pooled samples from two independent runs. Initially, all single-gene analyses for protein-coding genes were run with partitioning (by codon position) and without it. The resulting harmonic means were compared (Bayes Factor; Kass & Raftery, Citation1995) to determine whether or not partitioning by codon was a better fit to the data.
The two combined alignments were analysed with a GTR+I+G model, including ML analyses without partitioning in PHYML (as outlined above) and with partitioning (by gene and by codon) in the web-server program RAxML (Stamatakis et al., Citation2008). Robustness was determined with 300 replicates of bootstrap resampling in all ML analyses. Bayesian analyses with partitioning were completed as outlined above for both of the combined alignments, although the stationary phase was only consistently reached in the total alignment with the temperature set to 0.1.
Anatomical methods
Specimens were liquid-preserved in formalin–seawater; whole-mount slides were stained in 1% aqueous aniline blue and photographed with a Zeiss MRc-5 digital camera mounted on a Zeiss Axioskop light microscope (Zeiss, Oberkochen, Germany) and processed using Adobe® PhotoShop CS4 Extended Version 11.0.1 (www.adobe.com/). Type and voucher collections are housed in HO, UNB, AD and MELU (herbarium abbreviations as specified in Holmgren et al., Citation1990).
Material was fixed for transmission electron microscopy (TEM) through chemical fixation steps that included 4% (w/v) glutaraldehyde in phosphate buffer, post-fixation in 1% (w/v) OsO4, rinsing in phosphate buffer, gradual dehydration through an ethanol to acetone series, and embedding in Spurr’s ® low viscosity resin (Polysciences, Warrington, Pennsylvania, U.S.A.; Spurr, Citation1969), in accordance with previously published methods (Scott et al., Citation1982). Thin-sections were cut using a Reichert–Jung E Ultracut microtome, stained with uranyl acetate (30 min) and Reynold’s lead citrate (8 min), and viewed using a Philips CM 100 transmission electron microscope.
Results
Molecular results
Four individual thalli from two independent samplings (26 January 2012, 9 February 2012) at Blackman’s Bay Blowhole were subjected to COI-5P DNA barcoding (Supplementary Table S1). All had identical sequences indicative of a single genetic species group (data not shown).
For phylogenetic analyses, all single-gene alignments were first subjected to ML and Bayesian analyses, the protein-coding genes being analysed with and without partitioning in the latter. Where branches were supported, the trees were generally consistent with regard to the positioning of the new entity (hereafter referred to by its new name, Entwisleia). For the SSU (SH = 0.8; PP = 0.86), LSU (SH = 0.55; PP = 0.81) and the EF2 with (PP = 0.99) and without (SH = 0.90; PP = 0.99) partitioning, Entwisleia was sister to the Colaconematales. For these genes the orders were largely monophyletic, with the exception of the Nemaliales for EF2 (the two included taxa attracting ‘Thorea’ sp.), but the relationships among them were poorly resolved. For both COI and rbcL, which saturate more quickly than the other markers, the genes resolved fewer of the orders as monophyletic, but there were no strongly supported inconsistencies relative to the single-gene analyses. For all three protein-coding genes, the Bayes factor indicated a substantial improvement when the genes were partitioned (1125, 1145, and 801, for EF2, rbcL and COI, respectively), but the interordinal nodes were typically no better resolved.
The available data were then concatenated into a ‘total’ combined alignment that was c. 84% complete (see Methods). The alignment was subjected to Bayesian analysis (with partitioning) and ML analysis (with and without partitioning), with the results summarized on the Bayesian tree (). As is previously reported in the literature (e.g. Harper & Saunders, Citation2002), all orders and an alliance between the Acrochaetiales and Palmariales were solidly supported (). The Bayesian result additionally gave the impression of strongly supported interordinal relationships among the Nemaliophycidae (). Key nodes included the relatively deep divergence of the Rhodachlyales (node a), then the Batrachospermales (, node b); an alliance between the Balbianiales and Balliales (node d), the Colaconematales resolving as sister to the Acrochaetiales and Palmariales (AP) complex rather than to the Nemaliales (node f); and the sister relationship between Entwisleia and the Colaconematales (node g). As Bayesian posterior probabilities can overestimate support, we also implemented ML analyses on the ‘total’ alignment with and without partitioning (). As anticipated, bootstrap support was lower across the tree for both of the ML analyses relative to PP support in the Bayesian analyses, and partitioning outperformed the non-partitioned analysis (log likelihood −79867.44232 versus −87158.56126, respectively). Despite lower support, the partitioned ML analysis recovered all of the key branches observed in the Bayesian result, including an alliance between Entwisleia and the Colaconematales (, node g; 89% bootstrap support). Removal of the EF2 data, which were only 57% complete, did not change the position of Entwisleia as sister to the Colaconematales, although support was less for all analyses ().
Fig. 2. Bayesian result for the ‘total’ combined alignment. ‘*’ indicates solid support for ordinal lineages and for an alliance of the Acrochaetiales and Palmariales complex in all six analyses completed for the two combined alignments. The table indicates the support at seven key nodes, labelled a–g, for the six analyses (Bayes and ML, without and with partitioning for both the ‘total’ and EF2-removed combined analyses). ‘NS’ indicates that a particular node was not resolved for that analysis. Support values for intraordinal relationships are not shown.
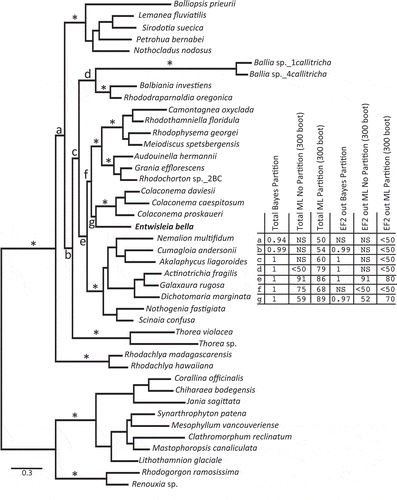
Taxonomic results, morphology and anatomy
The molecular findings strongly indicate the uniqueness of the newly discovered alga at the ordinal, family, genus and species levels, and this is amply supported by the suite of anatomical features that this organism displays (see below). The following new taxa are thus proposed:
Order Entwisleiales G.W. Saunders & Kraft, ord. nov.
Description
Gametophytes uniaxial, thalli differentiated into central axes and determinate cortical fascicles and adventitious filaments. Secondary pit connections lacking; pit plugs with two cap layers; pyrenoids absent. Carpogonial branches multicellular, zygote nuclei forming in, and embryos arising from, the carpogonium; spermatangia developing directly on cortical filaments without intervening mother cells. Tetrasporophytes unknown.
Family Entwisleiaceae G.W. Saunders & Kraft, fam. nov.
Description
Central-axial cells ringed distally by numbers of whorled periaxial cells bearing lax cortical fascicles. Carpogonial branches borne on periaxial and distal fascicle cells and, when present, cells of adventitious filaments. Presumed fertilization resulting in multiple gonimoblast initials cut off directly from the carpogonium; fusion cell absent; carposporangia terminal at surfaces of compact carposporophytes.
Entwisleia F.J. Scott, G.W. Saunders & Kraft, gen. nov.
Description
Central-axial cells tightly jacketed by a lax layer of downwardly growing rhizoidal filaments derived from periaxial cells, the rhizoidal filaments giving rise to perpendicular adventitious cortical filaments that fill in between the nodal fascicles. Carpogonial branches linear, outwardly directed, bearing simple to richly branched lax filaments from subhypogynous cells; divisions of the fertilized carpogonium vertical, gonimoblast initials several, initially directed basipetally from the carpogonium; spermatangia borne on cells of cortical fascicles and on adventitious cortical filaments arising from rhizoidal filaments.
Etymology
Named in honour of Dr Timothy J. Entwisle, with our admiration. For years, the two junior authors enjoyed bantering with him, one of the world’s premier experts on the Batrachospermales, promising him that, through our diligence, we would one day surely provide him with the first marine representative of the order. For a time it appeared that we might actually have delivered on this audacious boast. Despite our failure, as indicated by molecular data (see below), we name this unexpected find for Prof. Entwisle in recognition of his outstanding contributions to the taxonomy of freshwater macroalgae, particularly in the Australasian flora, and for his services to botanical sciences generally in major leadership roles as past Executive Director of the Botanic Gardens Trust, Sydney; recent Director of Conservation, Living Collections and Estates, Kew Gardens, London; and newly appointed Director and Chief Executive of the Royal Botanical Gardens, Melbourne.
Pronunciation
Because accents in Latin, and particularly in botanical-Latin names, are usually placed on either the penultimate or ante-penultimate syllable, we regard the final consonant in ‘Entwisle’ as having a long-vowel’s pronunciation (‘ell’), as it more-or-less does when spoken. We thus advocate pronunciation of the genus name as ‘Ent-wiss-ELL′-ee-a’, despite the fact that no ‘e’ precedes the ‘l’ as the name is written. Correspondingly, ‘Ent-wiss-ell-ee-A′-cee-ee’ and ‘Ent-wiss-ell-ee-A′-lees’ are the intonations that we advocate for the family and order.
Type and only species
Entwisleia bella F.J. Scott, G.W. Saunders & Kraft, sp. nov.
Description
Thalli caespitose, erect, to 8.0 cm in length, the axes terete, mucoid, single or in clusters from fibrous discoid holdfasts. Axes cylindrical or nodulose, radially branched to 2–5 orders. Periaxial cells 3–8. Cystocarps globular to somewhat compressed, 80–105 µm in diameter; carposporangia undivided, 5.5–9.0 µm in length.
Etymology
Besides being appropriate because E. bella is a beautiful plant, the name of this species also pays homage to the first author’s Sicilian great-grandparent, who settled in Queenscliff, Victoria, on Bass Strait to the north of Tasmania. Early experiences at Queenscliff strongly influenced the senior author’s subsequent devotion to exploring and studying the biologically rich Australian marine environment.
Holotype
FJS #6512 (HO 569791) (; Supplementary ).
Figs 3–10. Entwisleia bella. Habit and vegetative features. The specimens illustrated are FJS 6512 (Figs 3, 4), FJS 5425 (Fig. 6), FJS 6119 (Figs 5, 8, 9, from slide B; Fig. 10, from slide D), and FJS 6120 (Fig. 7, from slide C). 3. In situ habit of the holotype (see also Supplementary Fig. 1). 4. The herbarium-pressed cystocarpic holotype specimen. 5. Pressed thallus fragment showing three to five orders of irregularly spaced lateral branching. 6. Habit of the liquid-preserved base of a robust female gametophyte, the primary axis anchored by a fibrous holdfast pad (arrow). 7. A transversely dividing apical cell (arrowhead) extending beyond enveloping cortical filaments. 8. Broadly rounded apices of primary laterals on a cystocarpic thallus (arrowheads indicate cystocarps), the apical cells of the central axes deeply immersed among cortical filaments ending in hairs. 9. A totally immersed central-axial filament (arrows) surrounded by cortical fascicles. 10. Whorl laterals of a distal axis showing early development of basipetal rhizoidal filaments from periaxial cells (arrowheads).
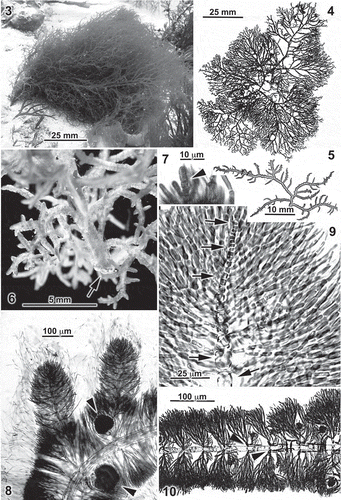
Type locality
(): –6 m at ‘The Blowhole’ (42° 58′ 19″ S, 147° 20′ 25″ E), Blackmans Bay, Derwent Estuary, Tasmania, (F.J. Scott & I.M. Mitchell; 9 February 2012).
Representative specimens examined
FJS 6118-6122 ‘The Blowhole’, Blackmans Bay (7.0–8.7 m; F.J. Scott & I.M. Mitchell, 17 Feb. 2011); FJS 6506–6509 paratypes (5.5–6.5 m; F.J. Scott & I.M. Mitchell, 26 Jan. 2012); FJS 6512 (K GEN-16141) holotype (6 m; F.J. Scott & I.M. Mitchell, 9 February 2012).
Distribution
Known only from the type locality, Blackmans Bay, Tasmania.
Habitat and community structure
Thalli occurred between 5.0–9.0 m depths and were scattered sparingly on Permian mudstone reef flats dusted or shallowly covered by sand. The site is located within the seaward margin of the Derwent Estuary and is subject to episodic high-rainfall plume events throughout the year and heavy swells during winter. The most conspicuous species with which E. bella was associated were the endemic fucoid Carpoglossum confluens and the native red alga Tsengia feredayae, other major occupants of the habitat being the brown algae Cladostephus spongiosus, Dictyopteris muelleri, Bellotia eriophorum, Ecklonia radiata, Cystophora moniliformis and species of Sargassum. Larger associated reds included Rhodoglossum gigartinoides and Hemineura frondosa. Year-round continuous monitoring suggests that plants appear in early summer, become reproductive and most abundant in January and February, and decline to small numbers or even total absence during autumn and winter.
Habit. Thalli are erect (), 1.5–8.0 cm in length, and grow singly or in clusters from a fibrous discoid holdfast (Fig. 6). Living plants are bright- to pale-red in colour and are flaccid and somewhat mucoid but not gelatinous in texture. Axes are terete throughout, slightly moniliform/torulose to cylindrical in outline, and radially branched to 2–5 orders ().
Vegetative structure. Thalli are uniaxial and the divisions of apical cells are transverse (), resulting in an initial series of rectilinear progeny before whorl branching of central-axial cells sets in (). Growth of determinant lateral filaments rapidly overtops the apical cells (Fig. 8), embedding them deeply within broadly rounded tips (). Apical cells are 2–3 µm in diameter, 5–8 µm in length. Axes are 100–130 µm wide distally, the second- and third-order laterals 200–270 µm wide and main axes 400–600 µm broad proximally. Plastids in the cortical cells are single and parietal. Pyrenoids are absent, as are secondary pit-connections.
Early development of lateral fascicles results in the cutting off of 3–8 periaxial cells in apparently no precise order around the distal poles of central-axial cells, which are 15–25 µm in diameter before the formation of periaxial cells and reach 35–110 µm in diameter by 100–450 µm in length further away from the apex. The fascicles of determinate filaments reach 220 µm in length with 8–10 elongate or ovoid cells, the terminal cells often ending in a colourless hair up to 300 µm long (Fig. 8); most interior cells subtend a pseudodichotomy (). Fascicles and adventitious filaments densely clothe mature central-axial filaments () but the stout central-axial cells remain prominently visible through the jacketing cortication ().
Figs 11–16. Entwisleia bella. Vegetative features. The specimens illustrated are FJS 5425 (Figs 11, 12, 16), FJS 6119 (Fig. 13, from slide D; Fig. 14, from slide B), and FJS 6120 (Fig. 15, from slide B). 11. Surface view of an axis with mature cystocarps (arrowheads). 12. The axis of Fig. 11, the focus on the stout cells of the central-axial filament. 13. Basipetal rhizoids arising from periaxial cells (arrowheads) and bearing adventitious cortical filaments between successive cystocarpic (arrows) whorl-laterals. 14. Periaxial cells (arrows) and a lax monolayer of acropetal and basipetal rhizoids that produce adventitious cortical filaments, one of which supports a carpogonial branch and early gonimoblast (arrowhead). 15. The basal periaxial cell (arrow) of an indeterminate lateral, the cell surrounded by basipetal rhizoids (arrowheads) from its own periaxial cells. 16. Cross-section of a central-axial filament, its cuticle surrounded by closely appressed rhizoids bearing adventitious cortical filaments (arrowheads).
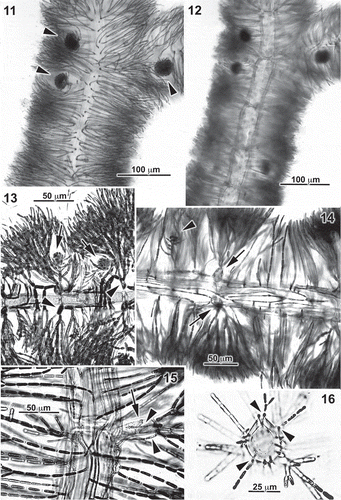
Within a few millimetres of the tips of axes, the periaxial cells initiate somewhat widely spaced, proximally directed rhizoids (), which are 5–8 µm in diameter. These adhere tightly to the surfaces of the central-axial cells and initiate adventitious perpendicular laterals () that corticate the axes between the periaxial fascicles (). Down-growing rhizoids predominate, but are later supplemented by distally directed rhizoids arising on the same periaxial cells (). Adventitious corticating filaments tend to be sparingly and distally branched (), although they eventually reach the same length as periaxial fascicles and are composed of similarly shaped or somewhat more rectilinear cells (). When periaxial cells subtend an indeterminate lateral (), this is signalled by the issuing of rhizoidal filaments from the bases of the lateral’s own periaxial cells (). Cross-sections of axes show the rhizoidal filaments closely appressed to the cuticle of the central-axial cells and producing perpendicular filaments with cells initially about half the diameter of fascicle cells ().
Spermatangial reproduction. Gametophytes are dioecious; the spermatangial thalli are constructed and branch like female gametophytes, although some are more moniliform in outline (). The distal 2–4 cells of assimilatory filaments, both those of the fascicles and those of adventitious filaments derived from rhizoids, bear sessile spermatangia () in pairs or threes on axial and lateral parent cells (). The spermatangia are lateral and apical on their bearing cells (), there being no intercalated spermatangial mother cells.
Figs 17–21. Entwisleia bella. Male gametophytes. Specimens illustrated are FJS 6120 (Fig. 17; Figs 19–21 from slide A) and FJS 5425 (Fig. 18, from slide B). 17. A liquid-preserved portion of an annulate spermatangial thallus. 18. Cross-section of a spermatangial frond, the spermatangia distal on both primary and adventitious cortical filaments. 19, 20. Surface (Fig. 19) and interior (Fig. 20) views of spermatangial axes. 21. Sessile spermatangia borne laterally and apically (arrowheads) on the terminal two or three cells of cortical filaments.
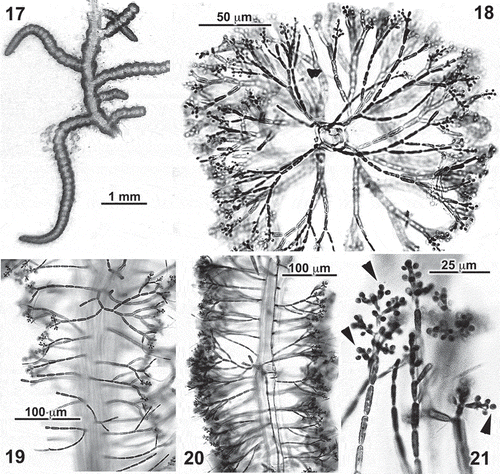
Carpogonial and cystocarp development
Carpogonial branches occur on both nodal fascicles and adventitious filaments. The cells are directed to the thallus surface () or curve laterally to various degrees () and number 4–8 proximal to the terminal carpogonium and trichogyne. Adventitious lateral cells issue from the hypogynous, subhypogynous () and even more proximal carpogonial-branch cells (), although they grow out into uniseriate unbranched or distally branched filaments primarily on subhypogynous cells (). Cells borne on hypogynous cells tend to arch toward the thallus interior (), in contrast to the more proximal filaments on the carpogonial branch, which bend toward the frond surface (). Presumed fertilization of the carpogonium is signalled by a lateral bulge or protrusion at the base (), followed by the cutting off of the first gonimoblast initial by a vertical septum (). This is followed by a second () and ultimately three or more gonimoblast initials as the trichogyne withers and disappears. The gonimoblast initial elongates before dividing () and is at first directed towards the thallus interior (). The early gonimoblast forms a flat-bottomed dense crown of cells on the hypogynous cell (). With further development, the outwardly growing gonimoblast filaments and subtending vegetative filaments from the lower carpogonial-branch cells become difficult to distinguish (), and no fusion cell forms at any stage of growth.
Figs 22–32. Entwisleia bella. Carpogonia, zygote and early carposporophytes. Specimens illustrated are FJS 6120 (Figs 22, 24, from slide C; Fig. 27, from slide A), FJS 6119 (Fig. 23, from slide B; Figs 25, 26, 28, 29, 32, from slide D; Fig. 30, from slide C; Fig. 31, from slide A). 22. An immature eight-celled, carpogonial branch borne on the basal cell of an adventitious cortical filament, the four cells subtending the carpogonium each bearing a single-celled lateral (arrowheads). 23. Single globose laterals (arrowheads) on the hypogynous and subhypogynous cells of an unfertilized carpogonial branch, with unbranched filaments of rectilinear cells borne proximally. 24. A carpogonial branch with lateral cells or filaments borne on each of the seven cells proximal to the carpogonium. 25, 26. Paired reflexed sterile laterals (arrowheads) on the hypogynous cells of mature carpogonial branches. 27. A lateral protrusion (arrowhead) of a presumably fertilized carpogonium prior to the cutting off of the first gonimoblast initial. 28. Formation of the first gonimoblast initial by longitudinal division (arrowhead) of a fertilized carpogonium. 29. Protrusion of a second gonimoblast initial from a carpogonium (arrow) following septation producing the first gonimoblast initial (arrowhead). 30. A two-celled primary gonimoblast (arrowheads) and septation (arrow) of the carpogonium to form the second gonimoblast initial. 31. A horizontally spreading cluster of gonimoblast cells (arrows) borne on the unfused (arrowhead) hypogynous cell. 32. Early development of gonimoblasts (arrowheads) on a carpogonium subtended by a hypogynous cell with a basipetal two-celled lateral (arrow) and sterile filaments from subhypogynous carpogonial-branch cells.
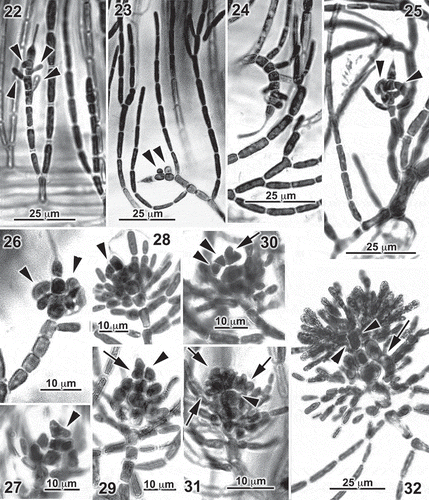
With maturation of the gonimoblast, the subtending filaments borne on carpogonial-branch cells branch pseudodichotomously or unilaterally (, ) or may remain relatively simple (). The carposporophyte expands into a tight cluster () and differentiates across the surface into an outer layer of lachrymose carposporangia 5.5–9.0 µm in length (). Regeneration of new sporangia within walls vacated by shed carpospores was not seen.
Figs 33–38. Entwisleia bella. Mature cystocarps. Specimens illustrated are FJS 6119 (Figs 33–37, from slide D) and FJS 6120 (Fig. 36, from slide A). 33. A mature cystocarp terminating a carpogonial branch borne on a periaxial cell (arrowhead) to which both a rhizoid and two unilaterally branched cortical filaments (arrows) are attached, the proximal laterals on carpogonial-branch cells more regularly subdichotomous throughout and forming a lax involucre around the compact carposporophyte. 34, 35. Early gonimoblasts on carpogonial branches with unbranched laterals. 36. Terminal carposporangia (arrowheads) on gonimoblast filaments dislodged from the cystocarp of Fig. 35. 37. A pair of equally developed mature cystocarps borne with involucral filaments (arrowheads) on a common periaxial cell (arrow). 38. Mature and young cystocarps borne on carpogonial branches attached to separate periaxial cells (arrowheads) at an axial node.
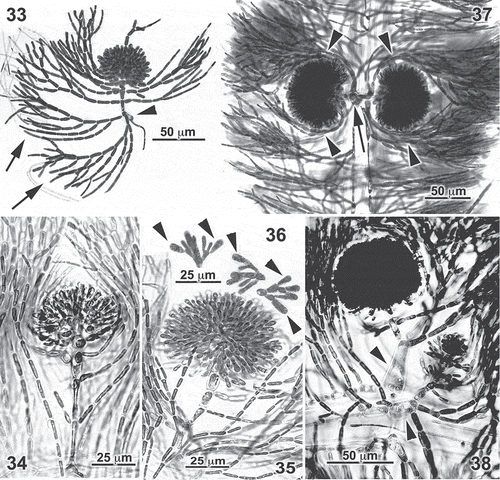
Mature cystocarps are globular to somewhat compressed and measure 80–105 µm in diameter (). Carpogonial branches subtending mature cystocarps are often borne directly on periaxial cells, either in roughly equal-sized pairs () or singly, and with adjacent carposporophytes at very different stages of development ().
Ultrastructure. TEM sections of primary pit-connections clearly indicate two cap layers (). The plasma membrane () branches to cross both the inner and outer surfaces of the pit plug between the inner and outer cap membranes (i.e. making the pit plug ‘extracellular’).
Discussion
The new species bears a strong resemblance in the field to Dudresnaya australis (Robins & Kraft, Citation1985, ), with which it was sometimes initially confused. Dudresnaya australis, however, differs greatly from Entwisleia both internally and reproductively, as well as in producing isomorphic gametophytes and tetrasporophytes, Dudresnaya producing zonate tetrasporangia. Another only distantly related genus that contains species that are similar in habit and texture to Entwisleia is Ganonema (Huisman, Citation2006, ), and indeed the new collections would have strongly suggested alliances with the Liagoraceae were it not for the fact that all members of the Liagoraceae are multiaxial. This leaves the freshwater genus Batrachospermum and its allies as the taxa that most closely resemble our plants. Entwisle et al. (Citation2007, , ) have shown that Australian members of Batrachospermum have many features resembling those described here for Entwisleia, namely very similar axial, fascicle and corticating rhizoidal structures, carpogonial branches borne on periaxial cells and bearing numerous sterile laterals from hypogynous and subhypogynous cells (Entwisle et al., Citation2007, ), and stalked, embedded globular cystocarps (Entwisle et al., Citation2007, , ). The uncanny resemblance even extends to the initiation of the gonimoblasts, which Kylin (Citation1917, reprinted in Kylin, Citation1956, ) showed arise in Batrachospermum directly on the carpogonium by vertical cross-walls and are initially bent toward the thallus interior. Furthermore and again like Entwisleia, the spermatangia in Batrachospermum are produced directly on cells of cortical fascicles, without the intermediary of special bearing cells (Kumano, Citation2002, p. 46, pl. 88(1)).
The closest associate of Entwisleia among fellow members of the Nemaliophycidae has been shown by our molecular analyses to be the Colaconematales, with its single genus Colaconema. For an alpha taxonomist whose experience of the families now included in the Nemaliophycidae largely predates the molecular era, no finding could be more startling or counterintuitive, for the gametophytes of Colaconema could hardly look less like those of Entwisleia. Originally created for diminutive and exclusively monosporangial acrochaetiaceous endophytes and epiphytes of European macroalgae (Dixon & Irvine, Citation1977, , ), Colaconema was subsequently regarded by Woelkerling (Citation1971) as a form-genus –analogous to the ‘fungi imperfecti’ of the mycological world – for acrochaetioids in which sexual reproduction was unknown. When, as a result of culture studies or field discovery, particular populations finally yield gametophytes and tetrasporophytes, Colaconema species would then be transferred to the genus Audouinella [which included all of the sexual species in the Acrochaetiaceae under Woelkerling’s (Citation1971) system], much as fungi imperfecti are sometimes moved as a result of new knowledge of reproductive processes, usually to the Ascomycetes. With time and the application of molecular methods, the Acrochaetiales has been returned to a multiple-genus order and various former members removed to a number of different orders. These include Colaconema itself, which, following the studies of Harper & Saunders (Citation2002), now contains reproductively, as well as molecularly, defined species such as Audouinella (Colaconema) daviesii and Audouinella (Colaconema) thuretii, for which typically acrochaetioid gametophytic and/or tetrasporangial stages are well documented (Woelkerling, Citation1971; Dixon & Irvine, Citation1977, , 43; Stegenga, Citation1985; Gabrielson et al., Citation2000). In weighing the merits of including Entwisleia in the Colaconematales versus elevating it to a separate order, the disparities in life cycles, vegetative architectures and reproductive anatomies have strongly influenced our choice of a separate order, abetted by the strong implications of the molecular analyses.
In addition to resolving the affinities of Entwisleia as a lineage of the Nemaliophycidae that is sister to the Colaconematales, our analyses are likely the most robust to date that address interordinal relationships in this subclass. Although support varied significantly, depending on the type of analysis and which gene regions were included and excluded, we did largely resolve consistent relationships among the major orders (, nodes a–g), which have until now largely defied phylogenetic resolution (see Verbruggen et al., Citation2010). Some of our results mirror those in the comprehensive analyses of Verbruggen et al. (Citation2010), including an association of the Colaconematales with the Acrochaetiales–Palmariales complex rather than the Nemaliales (node f, ) and the sister association of the Colaconematales+Acrochaetiales–Palmariales (node c, ) with the moderately supported Balbianiales and Balliales (node d, ). Our analyses differ from those of Verbruggen et al. (Citation2010) with regard to the relative positions of the Batrachospermales, Rhodachlyales and Thoreales (nodes a and b, ), but our results, as do theirs, present hypotheses for further phylogenetic testing within what is possibly the most anatomically diverse subclass of florideophytes.
Supplementary information
The following supplementary material is available for this article, accessible via the Supplementary Content tab on the article’s online page at http://dx.doi.org/10.1080/09670262.2013.849359
Table S1. Taxonomy, voucher information, and GenBank accession numbers of gene sequence used in the phylogenetic analysis.
Supplementary file 1. SSU alignment (nexus format).
Supplementary file 2. LSU alignment (nexus format).
Supplementary Figure 1. Colour photograph of the holotype of Entwisleia bella growing in situ at 6 m depth at ‘The Blowhole’, Blackmans Bay, Derwent Estuary, Tasmania.
Supplementary material
Download Zip (38.7 KB)Acknowledgements
The first author wishes to thank her PhD supervisors, Prof. James Kirkpatrick, Prof. Graham Edgar and Dr Neville Barrett, for their generous encouragement of her ecological research in the subtidal marine habitats of southeastern Tasmania, and to her diving partners and field team Iona Mitchell, Amelia Fowles and Robert Wills for dedicated assistance throughout the years. Logistic support was provided by University of Tasmania and the Australian Antarctic Division (Electron Microscope Unit). Financial support to FJS was provided by the Australian Commonwealth Environmental Research Facilities (CERF/NERP, Marine Biodiversity Hub) and the Winifred Violet Scott Trust, and to G. Edgar through an Australian Research Council (ARC) linkage project. GWS thanks Tanya Moore for generating most of the sequence data used in this project, Drs Daryl Lam and Heroen Verbruggen for assistance with the program gDam, and Kyatt Dixon for the introduction to RAxML. Bridgette Clarkson, Susan Clayden, Kyatt Dixon, Suzanne Fredericq, David Garbary, Susan Loiseaux de Goër, Katy Hind, Robert Hooper, John Huisman, Lesleigh Kraft, Line Le Gall, Enrique Martinez, Dan McDevit, Iona Mitchell, Ruth Nielsen, D. Pascoaloto, Curt Pueschel, Brian Rudolph, Hector Ruiz, Robin Sheath, Anne Stewart, Melissa Tesche, Jose Utge, Heroen Verbruggen, Morgan Vis and John West all contributed to the collection of samples used in the molecular aspects of this project. The research was supported through funding to GWS from the Canadian Barcode of Life Network from Genome Canada through the Ontario Genomics Institute, Natural Sciences and Engineering Research Council of Canada and other sponsors listed at www.BOLNET.ca. Additional support to GWS was provided by the Canada Research Chair Program, the Canada Foundation for Innovation and the New Brunswick Innovation Foundation, as well as NSF through the RedToL project. GTK gratefully acknowledges the Australian Biological Resources Study for longstanding research support, Ms Rebecca Herrington for vital organization of fieldwork logistics, and the Botany Departments of the Universities of Melbourne and Hawaii for major financial assistance. We thank Dr Tom Schils and an anonymous referee for many helpful suggestions for improving the manuscript.
References
- Dixon, P.S. & Irvine, L.M. (1977). Seaweeds of the British Isles. Volume 1. Rhodophyta. Part 1. Introduction, Nemaliales, Gigartinales (British Museum [Natural History], London).
- Drummond, A.J., Ashton, B., Cheung, M., Heled, J., Kearse, M., Moir, R., Stones-Havas, S., Thierer, T. & Wilson, A. (2009). Geneious v4.7. Available from http://www.geneious.com/
- Entwisle, T.J., Skinner, S., Lewis, S.H. & Foard, H.J. (2007). Algae of Australia: Batrachospermales, Thoreales, Oedogoniales and Zygnemaceae. ABRS, Canberra; CSIRO Publishing, Melbourne.
- Gabrielson, P.W., Widdowson, T.B., Lindstrom, S.C., Hawkes, M.W. & Scagel, R.F. (2000). Keys to the benthic marine algae and seagrasses of British Columbia, southeast Alaska, Washington and Oregon. Phycological Contribution from the Department of Botany, University of British Columbia, 5: 1–189.
- Guindon, S. & Gascuel, O. (2003). A simple, fast, and accurate algorithm to estimate large phylogenies by maximum likelihood. Systematic Biology, 52: 696–704.
- Harper, J.T. & Saunders, G.W. (2001). Molecular systematics of the Florideophyceae (Rhodophyta) using nuclear large and small subunit rDNA sequence data. Journal of Phycology, 37: 1073–1082.
- Harper, J.T. & Saunders, G.W. (2002). A re-classification of the Acrochaetiales based on molecular and morphological data, and establishment of the Colaconematales ord. nov. European Journal of Phycology, 37: 463–476.
- Holmgren, P.K., Holmgren, N.H. & Barnett, L.C. (Eds). (1990). Index Herbariorum: Part 1: The Herbaria of the world (8th Ed. ). New York Botanical Garden, Bronx, New York.
- Huelsenbeck, J.P. & Ronquist, F. (2001). MRBAYES: Bayesian inference of phylogenetic trees. Bioinformatics, 17: 754–755.
- Huisman, J.M. (2006). Algae of Australia: Nemaliales. ABRS, Canberra; CSIRO Publishing, Melbourne.
- Kass., R.E. & Raftery., A.E. (1995). Bayes factors. Journal of the American Statistical Association, 90: 773–795.
- Kumano, S. (2002). Freshwater red algae of the world. Biopress, Bristol.
- Kylin, H. (1917). Über die Entwicklungsgeschichte von Batrachospermum moniliforme. Berichte der Deutschen Botanischen Gesellschaft, 35: 155–164.
- Kylin, H. (1956). Die Gattungen der Rhodophyceen. C.W.K. Gleerups, Lund.
- Le Gall, L. & Saunders, G.W. (2007). A nuclear phylogeny of the Florideophyceae (Rhodophyta) inferred from combined EF2, small subunit and large subunit ribosomal DNA: establishing the new red algal subclass Corallinophycidae. Molecular Phylogenetics and Evolution, 43: 1118–1130.
- Maddison, D.R. & Maddison, W.P. (2003). MacClade: analysis of phylogeny and character evolution. Version 4.08. Sinauer Associates, Sunderland, MA.
- Posada, D. & Crandall, K.A. (1998). Modeltest: Testing the model of DNA substitution. Bioinformatics, 14: 817–818.
- Robins, P.A. & Kraft, G.T. (1985). Morphology of the type and Australian species of Dudresnaya (Dumontiaceae, Rhodophyta). Phycologia, 24: 1–34.
- Saunders, G.W. & Hommersand, M.H. (2004). Assessing red algal supraordinal diversity and taxonomy in the context of contemporary systematic data. American Journal of Botany, 91: 1494–1507.
- Saunders, G.W. & McDevit, D.C. (2012). Methods for DNA barcoding photosynthetic protists emphasizing the macroalgae and diatoms. DNA barcodes: methods and protocols. Methods of Molecular Biology, 858: 207–222.
- Saunders, G.W. & Moore, T.E. (2013). Refinements for the amplification and sequencing of red algal DNA barcode and RedToL phylogenetic markers: a summary of current primers, profiles and strategies. Algae, 28: 31–43.
- Scott, F.J., Wetherbee, R. & Kraft, G.T. (1982). The morphology and development of some prominently stalked southern Australian Halymeniaceae (Cryptonemiales, Rhodophyta). I. Cryptonemia kallymenioides (Harvey) Kraft comb. nov. and C. undulata Sonder. Journal of Phycology, 18: 245–257.
- Spurr, A.R. (1969). A low viscosity epoxy resin embedding medium for electron microscopy. Journal of Ultrastructural Research, 26: 31–43.
- Stamatakis, A., Hoover, P. & Rougemont. J. (2008). A rapid bootstrap algorithm for the RAxML web-servers. Systematic Biology, 57: 758–771.
- Stegenga, H. (1985). The marine Acrochaetiaceae (Rhodophyta) of southern Africa. South African Journal of Botany, 51: 279–330.
- Swofford, D.L. (2003). PAUP: phylogenetic analysis using parsimony (and other methods), version 4. Sinauer Associates, Sunderland, MA.
- Verbruggen, H., Maggs, C.A., Saunders, G.W., Le Gall, L., Yoon, H-S. & De Clerck, O. (2010). Red algal tree of life: data mining approach identifies certainties and future research priorities and data requirements. BMC Evolutionary Biology, 10: 16.
- Vis, M.L., Harper, J.T. & Saunders, G.W. (2007). Large subunit rDNA and rbcL gene sequence data lace Petrohua bernabei gen. et sp. nov. in the Batrachospermales (Rhodophyta), but do not provide further resolution among taxa in this order. Phycological Research, 55: 103–112.
- Woelkerling, W.J. (1971). Morphology and taxonomy of the Audouinella complex (Rhodophyta) in southern Australia. Australian Journal of Botany, Supplement 1: 1–91.
- Womersley, H.B.S. (2004). The marine benthic flora of southern Australia. Part IIID: Ceramiales - Delesseriaceae, Sarcomeniaceae, Rhodomelaceae. Flora of Australia Supplementary Series No. 18. ABRS, Canberra.