Abstract
The diatom genus Diprora (Bacillariophyceae) was described from a cave on Kauaʻi, Hawaiʻi. Due to its lack of a raphe system and bilateral symmetry, the genus was first assigned to the Fragilariophycidae (‘araphid diatoms’), but its systematic affinities were undetermined. We present a three-gene phylogeny, based on nuclear (SSU rDNA) and chloroplast (rbcL, psbC) genes, to determine the phylogenetic relationships of Diprora in the diatom tree of life. The concatenated multi-gene phylogenies, and the individual genes trees show Diprora to nest deep within the raphid diatoms, and in different analyses (of one-, two- and three-gene sequences) this diatom is shown to be most closely allied with taxa referred currently to the Sellaphoraceae, including Sellaphora or Fallacia. Hypothesis testing of the likelihood of Diprora being an ‘araphid’ diatom indicates rejection of the hypothesis that the phylogenetic position of D. haenaensis is within the araphid diatoms. Loss of the raphe system and reduction of other features may be related to its cave-dwelling habit.
Introduction
The freshwater algal flora of Hawaiʻi has long been thought to be composed of cosmopolitan species, as demonstrated in the checklist produced by Sherwood (Citation2004). More recently the flora has been shown to contain many unique species, especially diatoms (Lowe et al., Citation2009; Kociolek et al., Citation2010; Lowe & Sherwood, Citation2010; Graeff & Kociolek, Citation2011; Ripple & Kociolek, Citation2013). Perhaps the most distinctive diatom species described from Hawaiʻi is Diprora haenaensis, a monotypic genus described from a cave on the island of Kauaʻi (Main, Citation2003).
Diprora has several distinct attributes. In his description of the genus with light and scanning electron microscopy, Main (Citation2003) documented a marginal row of striae, each composed of a single areola; the margin raised into what has been termed a prow (Main, Citation2003); a filamentous growth habit; and a lack of rimoportulae and pore fields. He also documented fimbriate perizonium strips around an initial valve and fimbriate girdle bands, as well as the formation of internal spores in this species, which also lacks a raphe. Main (Citation2003) explored the systematic placement of Diprora, discussing the possibility of creating a new family and order for this enigmatic diatom. He suggested that, due to its filamentous growth habit and araphid sternum, it could not be assigned to the centric diatoms sensu Round et al. (Citation1990), and he did not suggest any affiliation with the raphid diatoms, presumably due to its lack of a raphe. Main concluded Diprora was a member of the Fragilariophyceae, a taxon established for the ‘araphid’ diatoms by Round et al. (Citation1990) but later shown to be non-monophyletic (e.g. Sims et al., Citation2006).
Diprora is also unique in its distribution. It was originally described from a cave on the northernmost part of Kauaʻi. It forms mucilaginous masses near the entrance of caves, and grows in relatively low light. It had previously not been reported from other habitats or from caves on any of the other Hawaiian Islands.
Molecular approaches to understanding the phylogenetic relationships of diatoms have transitioned from using single genes (such as SSU and rbcL) to developing multi-gene trees (e.g. Ruck & Theriot, Citation2011; Souffreau et al., Citation2011). A large library of gene sequences has been developed from the pioneering work of several laboratories, and these include genes from several gene families. Three of the most widely utilized genes in developing phylogenies for diatoms have been nuclear SSU rDNA and the plastid rbcL and psbC genes (e.g. Theriot et al., Citation2010; Ruck & Theriot, Citation2011).
As part of the Hawaiian Freshwater Algal Biodiversity Survey (Sherwood et al., Citation2012), we had the opportunity to collect multiple samples of D. haenaensis from the Hawaiian Islands. In the present study, our aim was to investigate the phylogenetic relationships of Diprora using light and scanning electron microscope observations of valve and girdle band morphology, as well as analyses of SSU rDNA, rbcL and psbC gene sequences.
Materials and methods
Materials and microscopy
Material containing D. haenaensis was collected by J.R. Johansen from Maniniholo Dry Cave, located on the north shore of the island of Kauaʻi, Hawaiʻi, USA (22°12.192′N, 159°33.940′W), on 11 January 2012 (Kociolek Collection at the University of Colorado (JPK), accession #8296). Additional specimens of D. haenaensis were collected earlier by J.P. Kociolek from Haena Cave, on the island of O‘ahu in January 2011 (JPK #6800). The roof and sides of both caves were scraped from along wet seeps originating from crevices in the rock. Collections were split and part of the initial collection was preserved in 2.5% glutaraldehyde. From living material individual cells or short chains of D. haenaensis were isolated into clonal culture through serial dilution via micropipette. Cultures of D. haenaensis were grown in WC medium (Guillard & Lorenzen, Citation1972) at room temperature (c. 21°C) with a 12 : 12 h light : dark cycle at a photon flux density of approximately 50 µmol m−2 s−1. The culture is currently available from the Kociolek Laboratory. Cleaned material and voucher slides have been accessioned into the Kociolek Collection at the University of Colorado (8296-Dipr001).
Material for light microscope (LM) observations was cleaned of organic matter by boiling in concentrated nitric acid, followed by multiple rinses with filtered water until a neutral pH was reached. For LM observations, cleaned material was air dried onto glass coverslips and permanently mounted in Naphrax® mounting medium (Brunel Microscopes, Chippenham, U.K.). Permanent slides were examined using an Olympus BX-51 LM outfitted with DIC optics and 60× (1.42 N.A.) and 100× (1.40 N.A.) objectives (Olympus America, Center Valley, Pennsylvania, U.S.A.) and images were taken using an Olympus DP 71 digital camera. For scanning electron microscope (SEM) observations, cleaned material was air-dried onto glass coverslips, sputter coated with 1 nm gold using a Cressington 108 sputter coater (Cressington Scientific Instruments, Watford, U.K.) and examined using a JEOL JSM 7401 field emission SEM (JEOL, Tokyo, Japan) at an acceleration voltage of 3 kV. Images were compiled with the freeware program Gimp 2.8 (http://www.gimp.org/).
DNA extraction and amplification
A Chelex 100® extraction method was used for DNA extraction from cultures of D. haenaensis. For the extraction 200 µL of a 10% Chelex 100® slurry was added to a small pellet of D. haenaensis. The Chelex–Diprora solution was agitated in a vortex mixer for 15 s at the highest setting, followed by centrifugation in a Fisher Scientific Accuspin Micro centrifuge (Thermo Fisher Scientific, Waltham, Massachusetts, U.S.A.) for 2 min at a force of 16 000 × g. The tube containing the centrifuged diatom cells and Chelex slurry was then heated at 95°C for 20 min, followed by an additional vortex and centrifugation cycle. After the final centrifugation, the supernatant was pipetted off the Chelex pellet into a new micro-centrifuge tube for storage.
For the phylogenetic analyses, three genetic markers were amplified: the nuclear marker SSU rDNA and two chloroplast markers, rbcL and psbC (). Markers were amplified through polymerase chain reaction (PCR) using GE Healthcare Illustra Ready-To-GoTM PCR beads (GE Healthcare Biosciences, Pittsburgh, Pennsylvania, U.S.A.) following the manufacturer’s instructions. lists the primers used for the amplification and sequencing of the three markers. The following PCR cycle was used for the amplification of all markers: an initial denaturing cycle of 94°C for 3 min 30 s, followed by 36 cycles of 94°C for 50 s, 52°C for 50 s, and 72°C for 50 s, with a final elongation stage of 72°C for 15 min. The amplified PCR products were purified using the PCR clean-up product ExoSAP-IT (Affymetrix, Santa Clara, California, U.S.A.) following the manufacturer’s instructions. Purified PCR products were sent to Functional Biosciences (Madison, Wisconsin, U.S.A.) for sequencing and returned sequences were assembled using Geneious 5.6 (Drummond et al., Citation2012).
Table 1. Taxa included in this study. Taxa are classified by informal groupings and include strain identifiers and GenBank accession numbers.
Table 2. Primers used to amplify and sequence the SSU rDNA, rbcL and psbC markers used in this study.
Sequence alignment and phylogenetic analyses
Diprora DNA sequences have been archived in GenBank (see for accession numbers). Sequence data for an additional 63 taxa, including representatives from ‘centric’, ‘araphid pennate’ and raphid pennate diatom lineages were obtained from GenBank and used in the phylogenetic analyses ( gives a full list of included taxa, strain IDs and GenBank accession numbers for the markers used).
Sequence alignments for the newly generated and GenBank sequences were performed using Muscle (Edgar, Citation2004), implemented in Geneious ver. 5.6 (Drummond et al., Citation2012) using default settings. After automatic alignment, the matrix was inspected visually and manually aligned as necessary. The ends of individual gene alignments were trimmed in order to minimize the amount of missing data due to differences in sequence length. Because of limited sequence availability, individual gene, two-gene (SSU and rbcL) and three-gene (SSU, rbcL and psbC) concatenated datasets were used to maximize the number of taxa included. The final trimmed lengths and taxa for the individual markers were 1481 bp and 55 taxa for SSU rDNA (Supplementary information, Alignment S1) and 1283 bp including 47 taxa for rbcL (Alignment S2). A 2752 bp concatenated two-gene matrix including 46 taxa (Alignment S3) and a 3712 bp concatenated three-gene matrix including 42 taxa (Alignment S4) were created from the trimmed single-marker alignments.
For the phylogenetic analyses, models of nucleotide substitution were selected for each marker individually and for the concatenated dataset as a whole using jModelTest 0.1.1 (Guindon & Gascuel, Citation2003; Posada, Citation2008) implementing the Akaike Information Criterion (AIC). The general time reversible (GTR) model with a gamma distribution (Γ) and a proportion of invariable sites (I) was chosen as the best model for each individual marker as well as for the concatenated matrix. Maximum likelihood (ML) analysis was conducted with RAxML 7.3.2 (Stamatakis, Citation2006) using the raxmlGUI 1.2 (Silvestro & Michalak, Citation2012) front end. The most likely tree for each individual marker, as well as for the combined datasets, was generated by conducting 20 independent ML tree searches using the GTR+Γ+I model with four gamma distribution rate classes, and branch support values were estimated using 500 bootstrap (BS) replicates. Bayesian analyses of the individual marker and combined datasets were conducted in Mr. Bayes 3.2.1 (Ronquist et al., Citation2012) using the GTR+Γ+I model with four gamma distribution rate classes. The Bayesian analyses were run for 10 million generations with a 1 million generation burn-in for the single marker dataset, and 20 million generations with a 2 million generation burn-in for the combined datasets. All Bayesian analyses used default priors and sampled trees and parameters every 1000 generations.
Hypothesis testing
Two competing hypotheses concerning the phylogenetic position of D. haenaensis within the diatom tree of life were tested using tree likelihoods and the approximately unbiased (AU) test (Shimodaira, Citation2002). For this analysis, the outgroup taxa (Odontella aurita ECT3772 and Odontella aurita ECT3788) were removed from the three-gene matrix, creating an unrooted tree with monophyletic araphid and raphid branches. RAxML was used to generate ML trees from the unconstrained dataset and one constrained to have D. haenaensis and the araphid taxa forming a monophyletic group. These two ML trees represent the most likely tree topologies from the current study and under the hypothesis that D. haenaensis is most closely related to an araphid lineage. The probability that the araphid hypothesis was as likely as the ML tree reported here was tested by calculating per-site log likelihood values using RAxML and implementing the AU test in the program Consel using default settings (Shimodaira & Hasegawa, Citation2001). In the AU test, Consel compares the constrained tree topology to a set of trees generated through a multi-scale bootstrap technique of per site log likelihoods. If the constrained tree topology falls outside the 95% confidence interval of the generated trees the hypothesis can be rejected.
Results
Morphology
LM observations of living cells are presented in . In nature, the cells form straight colonies. Mucilage pads were not evident and this species lacks spines, so we are still unsure how chain formation is maintained. There is a large difference in the size of cells even after one cell division, so this species must go through size reduction in relatively few divisions. In glutaraldehyde-preserved specimens each cell has a single, H-shaped chloroplast (), which lies beneath the epivalve (), and the living, cultured cells contained few to many lipid droplets ().
Figs. 1–17. Diprora haenaensis from Maniniholo Dry Cave, Kauaʻi, Hawaiʻi, USA, LM. 1. Chain of living cells showing H-shaped chloroplast and lipid droplets. 2, 3. Valve view of field-fixed specimens, showing H-shaped chloroplasts. 4–17. Cleaned cells in valve (Figs 4–6, 8–10, 12–17) and girdle (Figs 7, 11) views, showing size diminution series. Initial valves are tumid at the centre and linear-elliptical; valves of vegetative cells become elliptical to round at the small end of the series. Scale bars = 10 µm.
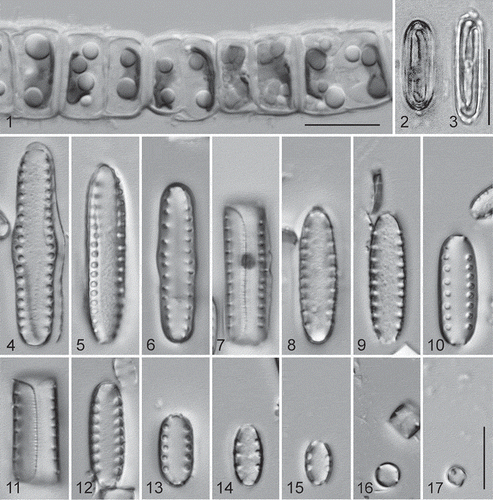
Cleaned valve and girdle band observations with LM are presented in . The linear to elliptical valves have a single row of relatively wide areolae down each side near the margin, which also extends onto the valve margin. The overlap of the epivalve on the hypovalve is distinct in this species (). In some cases the wide areolae may occur near the base of the valve, but no pore fields or other features have been identified for this species.
Observations made with SEM () reveal valves that have large, round to ovoid areolae (). The interstriae, as viewed internally, are relatively thick and the pores appear to have no internal occlusions (). Externally, however, occlusions can be seen at the valve poles (, , ). These occlusions are sunken into the areolae and hymenate, with a network of round openings in a single plane (). There are no processes present (). In girdle view the epivalve is shown to be much larger than the hypovalve (). Extensions from the epivalve fit over the mantle of the hypovalve at both apices (). The girdle bands adjoining the valve are half bands. The advalvar margin of each of these band is fimbriate, and at the base of the separation of the fimbriae the opening is rounded (); the abvalvar band margin is slightly undulate (, ). The half bands fit into the associated valve, fimbriate margin tucked under the mantle, one on each side of the valve (). A further narrow band is positioned between the half bands from each valve in the frustule (). An initial valve is shown in . The valve has transversely oriented ridges, impressions left from being covered by the perizonium (). The imprint indicates that the perizonium consists of a wide central band – the primary transverse perizonial band – with much narrower secondary bands on either side of it, which become somewhat wider away from the primary band. The secondary bands are inflected along the centre of the auxospore on the side visible in and apparently do not extend to the tips of the auxospore, since the initial cell has plain, rounded ends.
Figs. 18–26. Diprora haenaensis from Maniniholo Dry Cave, Kauaʻi, Hawaiʻi, USA, SEM. 18. External view of the valve face showing large areolae. 19. Internal view of valve. 20. Girdle view of the whole frustule showing overlap of the epi- and hypovalves. 21. Girdle band with fimbriate margin. 22. External view of an initial valve showing grooves imprinted from perizonial bands. 23, 24. Girdle view of two attached cells showing girdle band structure. 25. Detailed external view of marginal areolae showing them to lack occlusions. 26. High magnification external view of terminal areola showing recessed, hymenate occlusions. Scale bars = 1 µm (Figs 18–25) and 100 nm (Fig. 26).
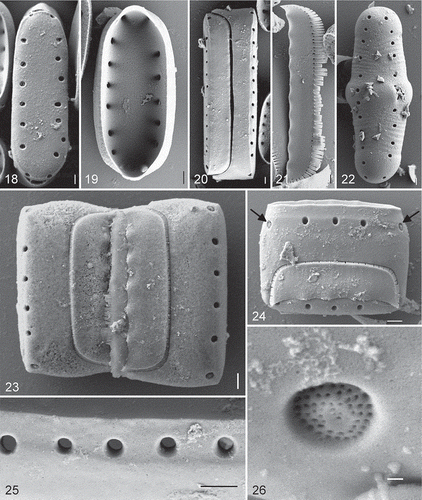
We examined an estimated 100,000 valves, including those that were interrupted in terms of their development, and no hint of a raphe branch or system was ever encountered.
Molecular analyses
Maximum likelihood and Bayesian analyses of the 3712 bp three-gene concatenated dataset returned largely congruent results, with log-likelihood values of −32014.32 and −31989.80 for the ML and Bayesian analyses, respectively. The incongruence in the trees occurred in the large raphid pennate diatom clade, where the Bayesian analysis, while fully resolving each individual clade, was unable to resolve the relationships between the Craticula–Stauroneis, Diprora–Fallacia–Caloneis–Pinnularia and Halamphora–Entomoneis–Rhopalodia clades (: unresolved Bayesian nodes are denoted with ‘–’). Trees inferred from both the ML and Bayesian analyses agreed in placing D. haenaensis within the raphid pennate diatoms () and provided strong support (100% bootstrap/1.00 Bayesian posterior probability) for the position of D. haenaensis as sister to Fallacia pygmaea and F. monoculata within a larger clade including the genera Pinnularia and Caloneis.
Fig. 27. Maximum likelihood phylogeny inferred from a concatenated dataset, including the nuclear marker SSU rDNA and the chloroplast markers rbcL and psbC. Node supports are shown above the branches as maximum likelihood bootstrap values (500 bootstrap replicates)/Bayesian posterior probabilities (both reported as per cent). Diprora haenaensis is highlighted in bold and a larger font.
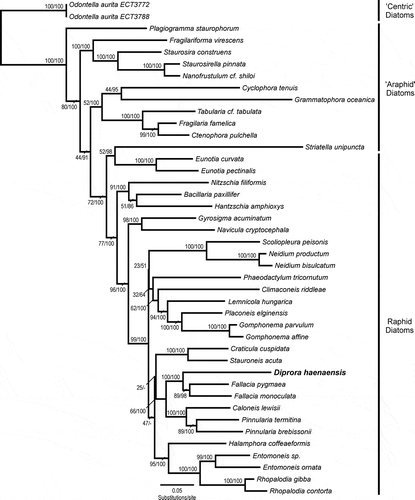
Maximum likelihood and Bayesian analysis performed on the 2752 bp two-gene concatenated dataset returned congruent results concerning the position of D. haenaensis and the topology of closely related taxa (). Log-likelihood values for the best trees were –21696.10 and –21712.98 for the ML and Bayesian analysis, respectively. Both analyses placed D. haenaensis within the raphid pennate diatoms and within a clade that included Rossia, Fallacia and Sellaphora. Within this clade, the analysis provided weak support (38/0.63) for D. haenaensis being most closely related to the genus Sellaphora, sister to S. cf. seminulum.
Fig. 28. Maximum likelihood phylogeny inferred from a concatenated dataset of the nuclear marker SSU rDNA and the chloroplast marker rbcL. Node supports are shown above the branches as maximum likelihood bootstrap values (500 bootstrap replicates)/Bayesian posterior probabilities (both reported as per cent). Diprora haenaensis is highlighted in bold and a larger font. Taxon sampling has been expanded (relative to the three-gene dataset) to include more members of the Sellaphoraceae.
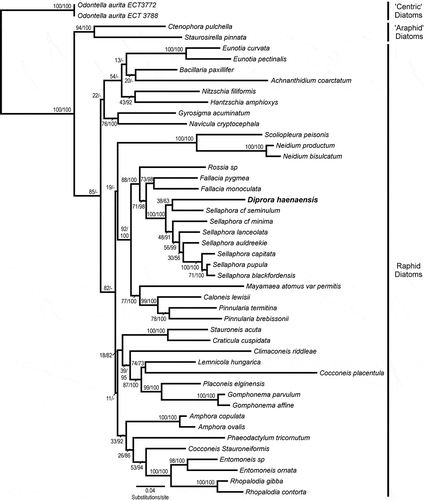
Maximum likelihood and Bayesian analyses performed on the single marker datasets returned results consistent with the analyses conducted on the concatenated dataset and indicated the phylogenetic position of D. haenaensis to be within the raphid pennate diatoms, most closely related to Fallacia or Sellaphora depending on the taxa included in the analysis. The analysis of SSU and rbcL single gene data provided moderate support (67/0.80) and (44/0.97) for the position of D. haenaensis as sister to S. cf. minima and S. lanceolata, respectively (Figs S1, S2). The analysis of psbC single gene data provided weak to moderate support (50/0.68) for D. haenaensis as sister to the genus Fallacia.
Hypothesis testing
The most likely tree topologies, inferred from the three-gene dataset by RAxML and used in the AU test, had log likelihood values of −30991.59 and −31106.84 for the unconstrained and constrained trees respectively. The results of the AU test returned P = 1.00 for the unconstrained topology and P < 0.001 for the constrained topology. The returned probability of P < 0.05 for the constrained tree, based on the current data, allows rejection of the hypothesis that the phylogenetic position of D. haenaensis is within the araphid diatoms.
Discussion
Our results from nuclear and plastid genes, individually and together, demonstrate that Diprora is part of a lineage of naviculoid diatoms, most closely related to members of the Sellaphoraceae, including F. pygmaea and Sellaphora taxa, and part of a monophyletic group that also includes Stauroneis, Craticula, Pinnularia and Caloneis. Thus, given that Diprora lacks a raphe system and shows no evidence of a vestigial raphe, molecular sequences from three genes strongly suggest that Diprora has secondarily lost its raphe system.
There are several morphological features that, despite its lack of a raphe system, are consistent with the placement of Diprora amongst the raphid diatoms. Within the pennate diatoms most ‘araphid’ diatoms (except a small number of genera) and members of the Eunotiales possess rimoportulae (Round et al., Citation1990; Kociolek et al., Citation2001), but this feature is absent in the raphid diatom group (Kociolek, Citation2000). The feature is also lacking in Diprora. Additionally, transverse perizonial bands, as in Diprora, are also found in many raphid pennates (Mann et al., Citation2011). The perizonium structure for some non-raphid pennates is quite different from the condition observed in Diprora (Sato et al., Citation2004, Citation2008). Finally, a close relationship between Diprora and members of the Sellaphoraceae is consistent with the presence in both of hymenate occlusions (Round et al., Citation1990) and a single H-shaped chloroplast lying against the epivalve (Mann, Citation1989).
Main (Citation2003) argued for the assignment of Diprora to the araphid diatoms because of its filamentous growth habit and lack of a raphe system. Loss of a raphe system has been hypothesized in several raphid lineages, including the Eunotiaceae (Kociolek & Rhode, Citation1998; Wetzel et al., Citation2012) and Naviculaceae (in the genera Diadesmis sensu lato, Hygropetra and Frankophila: see Granetti, Citation1978; Lange-Bertalot, Citation1997; Cox, Citation2006a; Mayama & Idei, Citation2009; Furey et al., Citation2012). Monoraphid diatoms have secondarily lost the raphe on one valve (Kociolek & Stoermer, Citation1986; Cox, Citation2006b), due to a filling in of the raphe slit with silica (Andrews, Citation1981; Boyle et al., Citation1984; Kociolek & Williams, Citation1987). This can sometimes be incomplete, leaving a vestige of the raphe slit (Lange-Bertalot & Le Cohu, Citation1985). Raphid diatoms with a filamentous growth habit are known in Eunotia (Pritchard, Citation1861; Steinman & Ladewski, Citation1987), Pinnularia (Hustedt, Citation1926), Diadesmis sensu lato (O’Meara, Citation1875; Hustedt, Citation1962), Fragilariopsis (Hustedt, Citation1958) and Bacillaria (Ussing et al., Citation2005; Jahn & Schmid, Citation2007).
The organization and structure of the cingulum of Diprora is quite unlike any other diatom. It has individual cingulum elements that fit in one side of the valve or the other, and do not loop around the periphery of the valve, as is common in both raphid and araphid diatoms (Round et al., Citation1990). We interpret this feature to be autapomorphic for the genus.
Diprora is known to occur only along wet fissures in caves from Kauaʻi and O‘ahu, two of the oldest high islands in the Hawaiian archipelago. The phylogenetic position of Diprora deep within the naviculoid diatoms suggests that its lack of a raphe is due to it being secondarily lost. Reduction of function in cave-dwelling organisms is well-known across a wide spectrum of organisms including arachnids (Jäger, Citation2001), crustaceans (Villacorta et al., Citation2008), insects (Juan & Emerson, 2010), fishes (Chakrabarty et al., Citation2012) and amphibians (Sket, Citation1997). In many of these cases, the mechanisms for the secondary loss of eyes and colouration are due to a loss of function in the cave environment (Culver et al., Citation1995; Porter & Crandall, Citation2003; Protas et al., Citation2011; Protas & Jeffery, Citation2012). The diatom Diadesmis gallica, which also can produce rapheless valves, is also known to occur in caves (Granetti, Citation1978; Cox, Citation2006a; Poulícková & Hašler, Citation2007). Given the narrow ecological niche of Diprora, the same mechanisms related to loss of function might apply in this diatom.
Supplementary information
The following supplementary data is accessible via the Supplementary Content tab on the article's online page at http://dx.doi.org/10.1080/09670262.2013.860239
Supplementary Figure S1. Maximum likelihood phylogeny inferred from nuclear SSU rDNA.
Supplementary Figure S2. Maximum likelihood phylogeny inferred from chloroplast encoded rbcL.
Supplementary files: Alignments S1 (SSU), S2 (rbcL), S3 (SSU+rbcL) and S4 (SSU+rbcL+psbC).
Supplementary material
Download Zip (356.2 KB)Acknowledgements
The constructive comments of two anonymous reviewers, the Associate Editor and Editor-in-Chief are greatly appreciated. This research was supported in part by grant 0841774 of the National Science Foundation.
References
- Alverson, A.J., Jansen, R.K. & Theriot, E.C. (2007). Bridging the Rubicon: phylogenetic analysis reveals repeated colonizations of marine and fresh waters by thalassiosiroid diatoms. Molecular Phylogenetics and Evolution, 45: 193–210.
- Andrews, G.W. (1981). Achnanthes linkei and the origin of monoraphid diatoms. Bacillaria, 4: 29–40.
- Boyle, J.A., Pickett-Heaps, J.D. & Czarnecki, D.B. (1984). Valve morphogenesis in the pennate diatom Achnanthes coarctata. Journal of Phycology, 20: 563–573.
- Chakrabarty, P., Davis, M.P. & Sparks, J.S. (2012). The first record of a trans-oceanic sister-group relationship between obligate vertebrate troglobites. PLoS ONE, 7: e44083. doi:10.1371/journal.pone.0044083.
- Cox, E.J. (2006a). Raphe loss and spine formation in Diadesmis gallica (Bacillariophyta) – an intriguing example of phenotypic polymorphism in a diatom. Nova Hedwigia, Beiheft 130: 163–176.
- Cox, E.J. (2006b). Achnanthes sensu stricto belongs with genera of the Mastogloiales rather than with other monoraphid diatoms (Bacillariophyta). European Journal of Phycology, 41: 67–81.
- Culver, D.C., Kane, T.C. & Fong, D.W. (1995). Adaptation and natural selection in caves. The evolution of Gammarus minus. Harvard University Press, Cambridge, MA.
- Daugbjerg, N. & Andersen, R.A. (1997). A molecular phylogeny of the heterokont algae based on analysis of chloroplast-encoded rbcL sequence data. Journal of Phycology, 33: 1031–1041.
- Drummond, A.J., Ashton, B., Buxton, S., Cheung, M., Cooper, A., Duran, C., Heled, J., Kearse, M., Markowitz, S., Moir, R., Stones-Havas, S., Sturrock, S., Swidan, F., Thierer, T. & Wilson, A. (2012). Geneious v 5.6. Available from http://www.geneious.com.
- Edgar, R.C. (2004). MUSCLE: multiple sequence alignment with high accuracy and high throughput. Nucleic Acids Research, 32: 1792–1797.
- Edgar, S.M. & Theriot, E.C. (2004). Phylogeny of Aulacoseira (Bacillariophyta) based on molecules and morphology. Journal of Phycology, 40: 772–788.
- Furey, P.C., Mayama, S., Lowe, R.L. & Catenazzi, A. (2012). Frankophila wayqechae sp. nov., a new aerophilic diatom species from the Peruvian Andes, South America. Diatom Research, 27: 165–175.
- Graeff, C.L. & Kociolek, J.P. (2011). The ultrastructure of Frustulia weinholdii Hustedt and F. neomundana Lange-Bertalot & Rumrich, with comparisons to two new Frustulia species from Hawaii and South Carolina. Proceedings of the Academy of Natural Sciences of Philadelphia, 161: 43–59.
- Granetti, B. (1978). Struttura di alcune valve teratologische di Navicula gallica (W. Smith) Van Heurck. Giornale Botanico Italiano, 112: 1–12.
- Guillard, R.R.L & Lorenzen, C.J. (1972). Yellow-green algae with chlorophyllide c. Journal of Phycology, 8: 10–14.
- Guindon, S. & Gascuel, O. (2003). A simple, fast and accurate method to estimate large phylogenies by maximum-likelihood. Systematic Biology, 52: 696–704.
- Hustedt, F. (1926). Untersuchungen über den Bau der Diatomeen, II–III. Berichte der Deutschen Botanischen Gesellschaft, 44: 394–402.
- Hustedt, F. (1958). Diatomeen aus der Antarktis und dem Südatlantik. Deutsche Antarktische Expedition 1938/1939. Geographie-kartographie Anstalt Mundus, Hamburg, 2: 103–191.
- Hustedt, F. (1962). Die Kieselalgen Deutschlands, Österreichs und der Schweiz unter Berücksichtigung der übrigen Länder Europas sowie der angrenzenden Meeresgebiete. In Dr L. Rabenhorst’s Kryptogamen Flora von Deutschland, Österreich und der Schweiz, vol. 7 (3). Akademische Verlagsgesellschaft, Leipzig.
- Jäger, P. (2001). A new species of Heteropoda (Araneae, Sparassidae, Heteropodinae) from Laos, the largest huntsman spider? Zoosystema, 23: 461–465.
- Jahn, R. & Schmid, A-M.M. (2007). Revision of the brackish-freshwater diatom genus Bacillaria Gmelin (Bacillariophyta) with the description of a new variety and two new species. European Journal of Phycology, 42: 295–312.
- Juan, C. & Emerson, B.C. (2010). Evolution underground: shedding light on the diversification of subterranean insects. Journal of Biology, 9: 17.
- Kociolek, J.P. (2000). Valve ultrastructure of some Eunotiaceae (Bacillariophyceae), with comments on the evolution of the raphe system. Proceedings of the California Academy of Sciences, 52: 11–21.
- Kociolek, J.P. & Rhode, K. (1998). Raphe vestiges in “Asterionella” species from Madagascar: evidence for a polyphyletic origin of the araphid diatoms? Cryptogamie, Algologie, 19: 57–74.
- Kociolek, J.P. & Stoermer, E.F. (1986). Phylogenetic relationships and classification of monoraphid diatoms based on phenetic and cladistic methodologies. Phycologia, 25: 297–303.
- Kociolek, J.P. & Williams, D.M. (1987). Unicell ontogeny and phylogeny: examples from the diatoms. Cladistics, 3: 274–284.
- Kociolek, J.P., Lyon, D. & Spaulding, S. (2001). Revision of the South American species of Actinella Lewis. In Lange-Bertalot Festschrift. Studies on diatoms. Dedicated to Prof. Dr. Dr. h.c. Horst Lange-Bertalot on the occasion of his 65th birthday (Jahn, R., Kociolek, J.P., Witkowski, A. & Compere, P., editors), 131–166. A.R.G. Gantner, Ruggell, Liechtenstein.
- Kociolek, J.P., Graeff, C.L. & Lowe, R.L. (2010). A new freshwater Gyrosigma Hassall species from Hawaii: implications for understanding sources of freshwater diatoms in the Hawaiian archipelago. Polish Botanical Journal, 55: 65–71.
- Lange-Bertalot, H. (1997). Frankophila, Mayamaea und Fistulifera: drei neue Gattungen der Klasse Bacillariophyceae. Archiv für Protistenkunde, 148: 65–75.
- Lange-Bertalot, H. & Le Cohu, R. (1985). Raphe-like vestiges in the pennate diatom suborder Araphidinae? Annales de Limnologie, 21: 213–220.
- Lowe, R.L. & Sherwood, A.R. (2010). Distribution and morphological variability of Cosmioneis (Bacillariophyceae) in Hawaii. Proceedings of the Academy of Natural Sciences of Philadelphia, 160: 21–28.
- Lowe, R.L., Sherwood, A.R. & Ress, J.R. (2009). Freshwater species of Achnanthes Bory (Bacillariophyta) from Hawaii. Diatom Research, 24: 327–340.
- Main, S.P. (2003). Diprora haenaensis gen. et sp. nov., a filamentous, pseudoaerial, araphid diatom from Kauaʻi (Hawaiian Islands). Diatom Research, 18: 259–272.
- Mann, D.G. (1989). The diatom genus Sellaphora: separation from Navicula. British Phycological Journal, 24: 1–20.
- Mann, D.G., Poulícková, A., Sato, S. & Evans, K.M. (2011). Scaly incunabula, auxospore development, and girdle polymorphism in Sellaphora marvanii sp. nov. (Bacillariophyceae). Journal of Phycology, 47: 1368–1378.
- Mayama, S. & Idei, M. (2009). Fine structure of two Hygropetra species, Hygropetra gelasina sp. nov. and Hygropetra balfouriana (Bacillariophyceae), and the taxonomic position of the genus with special reference to Frankophila. Phycological Research, 57: 290–298.
- Medlin, L.K., Elwood, H.J., Stickel, S. & Sogin, M.L. (1988). The characterization of enzymatically amplified eukaryotic 16S-like rRNA-coding regions. Gene, 71: 491–499.
- O’Meara, E. (1875). Report on the Irish Diatomaceae. Proceedings of the Royal Irish Academy, series 2, 2: 235–425.
- Porter, M.L. & Crandall, K.A. (2003). Lost along the way: the significance of evolution in reverse. Trends in Ecology and Evolution, 18: 541–547.
- Posada, D. (2008). jModelTest: phylogenetic model averaging. Molecular Biology and Evolution, 25: 1253–1256.
- Poulícková, A. & Hašler, P. (2007). Aerophytic diatoms from caves in central Moravia (Czech Republic). Preslia, 7: 185–204.
- Pritchard, A. (1861). A history of infusoria, living and fossil: arranged according to Die Infusionsthierchen of C.G. Ehrenberg; containing coloured engravings, illustrative of all the genera, and descriptions of all the species in that work, with several new ones; to which is appended an account of those recently discovered in the chalk formations. Edition IV, revised and enlarged by J.T. Arlidge, W. Archer, J. Ralfs, W.C. Williamson and the author. Whittaker, London.
- Protas, M.E. & Jeffery, W.R. (2012). Evolution and development in cave animals: from fish to crustaceans. Wiley Interdisciplinary Reviews: Developmental Biology, 1: 823–845.
- Protas, M.E., Trontelj, P. & Patel, N.H. (2011). Genetic basis of eye and pigment loss in the cave crustacean, Asellus aquaticus. Proceedings of the National Academy of Sciences of the United States of America, 108: 5702–5707.
- Ripple, H. & Kociolek, J.P. (2013). The diatom (Bacillariophyceae) genus Actinella Lewis from Hawaiʻi. Pacific Science, 67: 609–621.
- Ronquist, F., Teslenko, M., van der Mark, P., Ayres, D.L., Darling, A., Höhna, S., Larget, B., Liu, L., Suchard, M.A. & Huelsenbeck, J.P. (2012). MrBayes 3.2: efficient Bayesian phylogenetic inference and model choice across a large model space. Systematic Biology, 61: 539–542.
- Round, F.E., Crawford, R.M. & Mann, D.G. (1990). The diatoms. Biology and morphology of the genera. Cambridge University Press, Cambridge.
- Ruck, E.C. & Theriot, E.C. (2011). Origin and evolution of the canal raphe system in diatoms. Protist, 162: 723–737.
- Sato, S., Nagumo, T. & Tanaka, J. (2004). Auxospore formation and the morphology of the initial cells of the marine araphid diatom Gephyria media (Bacillariophyceae). Journal of Phycology, 40: 684–691.
- Sato, S., Mann, D.G., Matsumoto, S. & Medlin, L.K. (2008). Pseudostriatella pacifica gen. et sp. nov. (Bacillariophyta); a new araphid diatom genus and its fine-structure, auxospore and phylogeny. Phycologia, 47: 371–391.
- Sherwood, A.R. (2004). Bibliographic checklist of the non-marine algae of the Hawaiian islands. Bishop Museum, Occasional Papers Series, 80: 1–23.
- Sherwood, A.R., Wang, N., Carlile, A.L., Neumann, J.M., Wolfgruber, T.K. & Presting, G.G. (2012). The Hawaiian Freshwater Algal Database (HfwADB): a laboratory LIMS and online biodiversity resource. BMC Ecology, 12: 22.
- Shimodaira, H. (2002). An approximately unbiased test of phylogenetic tree selection. Systematic Biology, 51: 492–508.
- Shimodaira, H. & Hasegawa, M. (2001). CONSEL: for assessing the confidence of phylogenetic tree selection. Bioinformatics, 17: 1246–1247.
- Silvestro, D. & Michalak, I. (2012). raxmlGUI: a graphical front-end for RAxML. Organisms Diversity and Evolution, 12: 335–337.
- Sims, P.A., Mann, D.G. & Medlin, L.K. (2006). Evolution of the diatoms: insights from fossil, biological and molecular data. Phycologia, 45: 361–402.
- Sket, B. (1997). Distribution of Proteus (Amphibia: Urodela: Proteidae) and its possible explanation. Journal of Biogeography, 24: 263–280.
- Souffreau, C., Verbruggen, H., Wolfe, A.P., Vanormelingen, P., Siver, P.A., Cox, E.J., Mann, D.G., Van de Vijver, B., Sabbe, K. & Vyverman, W. (2011). A time-calibrated multi-gene phylogeny of the diatom genus Pinnularia. Molecular Phylogenetics and Evolution, 61: 866–879.
- Stamatakis, A. (2006). RAxML-VI-HPC: maximum likelihood-based phylogenetic analyses with thousands of taxa and mixed models. Bioinformatics, 22: 2688–2690.
- Steinman, A.D. & Ladewski, T.B. (1987). Quantitative shape analysis of Eunotia pectinalis (Bacillariophyceae) and its application to seasonal distribution patterns. Phycologia, 26: 467–477.
- Theriot, E.C., Ashworth, M., Ruck, E., Nakov, T. & Jansen, R.K. (2010). A preliminary multigene phylogeny of the diatoms (Bacillariophyta): challenges for future research. Plant Ecology and Evolution, 143: 278–296.
- Ussing, A.P., Gordon, R., Ector, L., Buczkó, K., Desnitski, A.G. & VanLandingham, S.L. (2005). The colonial diatom “Bacillaria paradoxa”: chaotic gliding motility, Lindenmeyer model of colonial morphogenesis, and bibliography, with translation of O.F. Müller (1783), “About a peculiar being in the beach water”. Diatom Monographs, 5: 1–140.
- Villacorta, C., Jaume, D., Oromi, P. & Juan, C. (2008). Under the volcano: phylogeography and evolution of the cave-dwelling Palmorchestia hypogaea (Amphipoda, Crustacea) at La Palma (Canary Islands). BMC Biology, 6: 7 doi:10.1186/1741-7007-6-7.
- Wetzel, C.E., Lange-Bertalot, H., Morales, E., Bicudo, D.C., Hoffmann, L. & Ector, L. (2012). Bicudoa amazonica gen. nov. et sp. nov. (Bacillariophyta) – a new freshwater diatom from the Amazon basin with a complete raphe loss in the Eunotioid lineage. Phytotaxa, 75: 1–18.