Abstract
The first reports on the presence of zoochlorellae within the organelles of mesenchymal cells from freshwater sponges were published in the 19th century. Today, it is well-known that freshwater sponges can be found in association with different endosymbiotic algae. However, until now there has been no detailed information about the endosymbiotic chlorophyll-containing algae in the remarkable endemic green sponges from Lake Baikal. In our study we were able for the first time to isolate and identify endosymbionts from primmorphs cultivated in vitro, and to compare them with those from naturally occurring Lubomirskia baicalensis sponges. Structural as well as molecular biological investigations show that the endosymbiotic alga is a Mychonastes species closely related to M. huancayensis. Another novel aspect of our work was to show that it is possible to use primmorphs of endemic sponges for isolation and subsequent cultivation of their endosymbiotic algae. We employed a simple cold-water (3–4°C) approach for cultivating Mychonastes sp., both within sponge primmorphs and in culture.
Introduction
Sponges (Porifera) are probably the earliest multicellular animals (Philippe et al., 2009) and their fossil record dates back to the Precambrian (Reitner & Wörheide, Citation2002; Love et al., Citation2009). Demosponges are recorded before the end of the Marinoan glaciation (635 Myr ago) and still exist today (Love et al., Citation2009). They are sessile filter-feeding animals inhabiting both marine and freshwater environments, and often contain diverse symbiotic microorganisms. A variety of sponge–microbe associations have been described in tropical, temperate and Antarctic regions (Trautman & Hinde, Citation2002; Steindler et al., 2002) and involve a diverse range of heterotrophic bacteria (including facultative anaerobes), cyanobacteria, unicellular algae, fungi and archaea (Wilkinson & Garrone, Citation1980; Preston et al., Citation1996; Höller et al., Citation2000; Webster et al., 2001, 2004; Taylor et al., 2004, 2007). In some cases, these microbial communities comprise 40–60% or more of the volume of the sponge and can contribute significantly to host metabolism, e.g. due to photosynthesis and nitrogen flux (Davy et al., Citation2002; Hentschel et al., Citation2006; Bayer et al., Citation2007). Algal symbionts may comprise up to 75% of the cellular tissue in sponge–algal associations (Wilkinson, Citation1987; Trautman et al., Citation2000; Trautman & Hinde, Citation2002).
The first reports of the presence of zoochlorellae within organelles of mesenchymal cells of freshwater sponges were published by Potts (Citation1887) and Weltner (Citation1893). It is now known that freshwater sponges can also be found in association with yellow-green algae, cryptophytes, dinoflagellates and diatoms (Reisser & Hader, Citation1984; Frost et al., Citation1997; Trautman & Hinde, Citation2002; Pröschold et al., Citation2011; Annenkova et al., Citation2011). Although the symbiotic microalgal flora (mostly zoochlorellae) in both marine (Sara, Citation1971; Vacelet, Citation1971, Citation1981; Palumbi, Citation1985; Rützler, Citation1990; Steindler et al., Citation2002; Trautman & Hinde, Citation2002; CitationÁvila et al., 2007) and freshwater sponges (Schröder, Citation1926; Castro-Rodriguez, Citation1930; Brien, Citation1932; Agnes & Brondsted, Citation1953; Brondsted & Brondsted, Citation1953; Miller, Citation1964; Lewin, Citation1966; Muscatine et al., Citation1967; Gilbert & Allen, Citation1973a, b; Williamson, Citation1979; Frost & Williamson, Citation1980; Whitman, Citation1981; Kanayama & Kamishima, Citation1990) has been thoroughly investigated, there is still little detailed information on endosymbiotic chlorophyll-containing algae in endemic sponges from Lake Baikal. This contrasts with the phytoplankton of this ancient lake, for which there are a number of reports on biodiversity and ecology (e.g. Popovskaya, Citation1968; Nagata et al., Citation1994; Bondarenko, Citation1995; Belykh et al., Citation2000; Jewson et al., Citation2008).
Demosponges from the family Lubomirskiidae are well known representatives of the endemic fauna of the Lake Baikal. These sponges inhabit the littoral zone of Lake Baikal, which is situated in south-east Siberia (51–56°N, 104–110°E) and is the world’s largest (23 000 km2), deepest (1643 m) and oldest (> 24 million years) freshwater body (Kozhova & Izmest’eva, Citation1998). Sponges dominate the littoral zone of Lake Baikal, covering 47% of the available surfaces (Frost et al., Citation1982; Bailey et al., Citation1995; Pile et al., Citation1997).
The Lake Baikal demosponges are chlorophyll-containing freshwater organisms due to their association with various chlorophyll-producing microsymbionts (Latyshev et al., Citation1992; Bil et al., Citation1999). A distinctive feature of sponges from Lake Baikal is their ability to live in symbiosis with various kinds of microalgae, including both green algae and dinoflagellates (Bil et al., Citation1999; Müller et al., Citation2009; Annenkova et al., Citation2011). Furthermore, there is evidence of genetic diversity among the symbiotic dinoflagellates (Annenkova et al., Citation2011).
Adult demosponges are difficult and expensive to study in vivo in most cases. Therefore, since sponges grow comparatively slowly and sampling is often difficult, an increasing amount of attention worldwide has focused on the cultivation of sponges in vitro and the development of permanent cultures like primmorphs (Klautau et al., Citation1993; Ilan et al., Citation1996; Custodio et al., Citation1998; Müller et al., Citation1999; Rinkevich, Citation1999; De Rosa et al., Citation2001; Pomponi, Citation2006; Chernogor et al., Citation2011a). The discovery of primmorphs in freshwater sponges was made by Potts in the 19th century (Potts, Citation1887), when they were referred to as ‘reduction bodies’. It was observed that freshwater sponges lose their organization when placed in conditions that are unfavourable for their normal behaviour. By gradual contraction of their soft parts they are able to form small compact masses of cells – the reduction bodies or primmorphs – which, when placed in favourable conditions, will reform new sponges (Müller, Citation1911). Interestingly, it was already noted at that time that unicellular green algae are localized within vacuoles of archaeocytes inside the primmorphs (see the review of Penney, Citation1933). Today, primmorphs are accepted to be structures intermediate between single cells and sponges. They can serve as models in studies aimed at solving the many challenges in the physiology and cell and molecular biology of sponges (Custodio et al., Citation1998; Müller et al., Citation1999; Pomponi, Citation2006; Cao et al., Citation2007; Chernogor et al.,Citation2011a, b). In particular, long-term studies of cultivated primmorphs allow us to determine the composition of the autochthonous microflora of sponges: differentiating between the autochthonous and allochthonous microorganisms of sponges is difficult or impossible in the wild because sponges are active filter feeders.
Recently, we developed unique methods for the long-term cultivation of primmorphs isolated from the Lubomirskia baicalensis demosponge (Chernogor et al., 2011a), which is endemic to Lake Baikal. This has allowed creation of a model system in which the primmorphs can be grown under experimentally controlled conditions in the absence of any additional organic components (Chernogor et al., 2011a). Intriguingly, the primmorphs possess the same green colouration as freshly collected L. baicalensis sponge, even after months of cultivation under artificial conditions. This intriguing phenomenon inspired us to try to isolate the chlorophyll-containing endosymbionts and identify them. The goals of the present study were therefore (1) to carry out systematic screening for the presence of microalgal symbionts within freshly collected L. baicalensis sponges (Fig. 1), as well as in the primmorphs obtained from them; (2) to isolate the previously unknown endosymbionts; and (3) to identify them using rDNA sequence data.
Figs 1–4. Lubomirskia baicalensis sponges and their endosymbionts. 1. Underwater image of green L. baikalensis sponges attached to rock at a depth of 15 m. 2. Primmorph obtained from dissociated L. baicalensis sponge cells after 48 h. 3, 4. Light microscope images showing green microalgae located within amoebocytes isolated from the primmorphs of L. baicalensis (Fig. 3) and the same microalgae isolated from the primmorphs using a syringe (Fig. 4). Scale bars = 0.5 mm (Fig. 2) and 5 µm (Figs 3, 4).
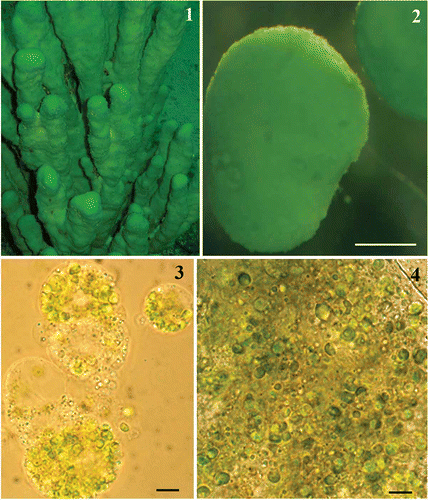
Materials and methods
Sample collection
Specimens of L. baicalensis were collected using SCUBA in Lake Baikal near Cape Listvennichny from 15–20 m depth (water temperature 3–4°C) in December 2010, during minimal growth of diatoms. The apical parts of specimens over 30–40 cm tall were collected, immediately placed in containers with Baikal water and ice, and transported to the Limnological Institute of the Siberian Branch of the Russian Academy of Sciences in Irkutsk after 1.2 h, at constant water temperature (3–4°C).
Formation and cultivation of primmorphs and isolation of microalgae
Primmorphs were obtained using the classical method of mechanical dissociation of the cells (Rezvoj, Citation1936): a clean sponge was crushed and the sponge cell suspension obtained was subsequently filtered through sterile 200-, 100- and 29-μm-mesh nylon to eliminate pieces of skeleton and spicules of the maternal sponge. The sponge cellular suspension was diluted to a concentration of 5 × 106 cells ml−1 and used as the primary material for primmorph formation. The L. baicalensis primmorphs (Fig. 2) were cultivated as described previously (Chernogor et al., 2011a) in natural Baikal water (NBW) at 3–6°С for >12 months, with 12 h mode of change of day and night. NBW was taken from a depth of 500 m in the lake and passed through sterilizing filters (Nalgene, Rochester, New York, U.S.A.).
Primmorphs were washed in sterile NBW to decrease casual contamination and filtered step-by-step through 10.0-, 5.0- and 2.0- µm filters (Sigma–Aldrich, St. Louis, Missouri, U.S.A.), consecutively. To obtain a suspension of the microalgae-containing amoebocytes from the primmorphs, the primmorphs were destroyed by pumping through a 5 ml syringe fitted with a 0.7 × 40 mm needle (Vogt Medical, Vertrieb, Germany). To monitor this process we used light and fluorescence microscopy. Microalgal cells were separated from the primmorph amoebocytes using consecutive filtering through a syringe with 0.33 cm2 polypropylene filters (Millipore, Germany) with pore sizes of 10, 5 and 2 µm. The microalgal cellular suspension obtained in this way was diluted in NBW up to a concentration of 1.2 × 103 cells ml−1 that was used for the cultivation of microalgae. Cell concentrations were determined using a haemocytometer (Steklopribor, Poltava, Ukraine) and light microscopy (Strober, Citation2001). Isolated microalgae were replaced into the 24-well plates (Nalgene, Nunc Nalge, Penfield, New York, U.S.A.) and cultivated aerobically using NBW and 1.5% Bacto-Agar (Difco, Detroit, Michigan, U.S.A.). They were kept for 6–12 months at 3–4°C with light from a 47 Lux lamp (LX 1330 B, Sanpometer, Moscow, Russia) and a 14 : 10 h light : dark cycle. Half of the cultivation medium was replaced once a week. In each experiment, the following sources were used for growth: 1.0% Dulbecco’s modified eagle medium (DMEM) (Sigma–Aldrich) (De Rosa et al., Citation2001, Citation2003), 1.5% fetal bovine serum (FBS) (HyClone Laboratories, Washington, U.S.A.), and different antibiotic groups: gentamycin 10 μg ml−1 (Sigma–Aldrich), a solution containing 1% penicillin (10 000 U ml−1) and streptomycin (10 000 μg ml−1) (Life Technologies, Paisley, U.K.), amphotericin 2.5 μg ml−1 (Life Technologies), and kanamycin 10 μg ml−1 (Life Technologies).
Light and electron microscopy
Living and formaldehyde-fixed (0.5%) samples of microalgae were investigated under an Axiovert 200 inverted microscope (Zeiss, Oberkochen, Germany) and fluorescence optics (lamp HBO 50W/AC ASRAM, blue filter, excitation 358/493 nm; emission 463/520 nm). Microalgal cells were stained using DAPI (4′,6-diamidino-2-phenylindole, Sigma-Aldrich). Photographs were taken with a Panasonic DMC-FZ3 digital camera.
Fixation for semi-thin sections and for scanning electron microscopy (SEM) and transmission electron microscopy (TEM) was performed according to the following procedure: pre-fixation in 1% OsO4 (Electron Microscopy Sciences, Hatfield, Pennsylvania, U.S.A.), 10 min; washing in cacodylate buffer (Electron Microscopy Sciences; 30 mM, pH 7.9), 10 min; fixation in 1.5% glutaraldehyde (Sigma–Aldrich) solution in cacodylate buffer (30 mM, pH 7.9), 1 h; washing in cacodylate buffer (30 mM, pH 7.9), 30 min; post-fixation in 1% OsO4 solution in cacodylate buffer (30 mM, pH 7.9), 2 h; washing in filtered Baikal water 3 × 15 min at room temperature; dehydration in increasing concentrations of ethanol (30%, 50%, 70%, 90% and 100%, 20 min in each) at room temperature; and finally embedding in Araldite resin (Electron Microscopy Sciences). For SEM, the specimens were critical point dried, mounted, coated with gold–palladium mixture, and examined in a Philips SEM 525M (Philips, Amsterdam, the Netherlands) microscope. Fixed specimens for TEM were dehydrated in a graded ethanol series, infiltrated, and finally embedded in Spurr's resin (Electron Microscopy Sciences), and polymerized for 24 h at 70°C (Spurr, Citation1969). Ultrathin sections were cut with a diamond knife, using an Ultracut EMUC6 microtome (Leica, Austria). The sections were sampled on uncoated 300 mesh grids (Electron Microscopy Sciences) and post-stained with uranyl acetate and Reynolds’ lead citrate (Electron Microscopy Sciences). The ultrastructure of cells in intracellular culture and in primmorphs was photographed using a Libra 120 TEM (Zeiss, Germany).
DNA extraction and sequencing of SSU and ITS rDNA
To isolate total DNA from the endosymbionts of primmorphs, they were suspended by pipetting in 2% Triton (Sigma–Aldrich) for 5 min. The cell suspension was washed twice with sterile Baikal water and treated with DNase (Sileks, Moscow, Russia) to remove extracellular DNA. Endosymbiont cells were concentrated by centrifugation and purified from the extracellular DNA fragments by agarose gel electrophoresis for 3 h. Genomic DNA from endosymbionts and microalgal cultures was extracted using AxyPrep Multi Genomic DNA Kits (Axygen, Corning Life Sciences, Union City, California, U.S.A.).
Small-subunit (SSU) rRNA gene fragments from L. baicalensis primmorph endosymbionts (see Supplementary Information) were PCR-amplified using the universal eukaryotic primers NS1 and NS2 (White et al., Citation1990). To amplify SSU gene fragments and the internal transcribed spacer (ITS) rRNA from microalgae, universal eukaryotic primers were used in pairs: NS1 (5′-GTAGTCATATGCTTGTCTC-3′) with NS4 (5′-CTTCCGTCAATTCCTTTAAG-3′), NS3 (5′-GCAAGTCTGGTGCCAGCAGCC-3′) with/NS8 (5′-TCC GCAGGTTCACCTACGGA-3′), and NS5 (5′-AACTTAA AGGAATTGACGGAAG-3′) with ITS-4 (5′-TCCTCCGC TTATTGATATGC-3′), as described by White et al. (Citation1990). Amplicons were separated in a 1% agarose gel and ligated into a commercial vector from a CloneJETTM PCR Cloning Kit (Fermentas, Lithuania) following the manufacturer’s instructions. Raw lysates of bacterial cells were used as the templates for PCR amplification using the pJET1.2 Forward Sequencing Primer and pJET1.2 Reverse Sequencing Primer. Nucleotide sequences were determined using an Applied Biosystems 3130xl Genetic Analyzer (Life Technologies, Carlsbad, California, U.S.A.).
Phylogenetic analysis of sequences
The raw sequence data were edited with Bioedit 7.0. 9.0 (Hall, Citation1999) and aligned using MAFFT version 7 (mafft.cbrc.jp/alignment/server/) (Katoh & Toh, Citation2008). The sequences obtained have been deposited in GenBank (EMBL) as accession HG532016. In order to identify the sponge symbiont and determine its position among the green algae, 28 18S rDNA sequences of chlorophyte algae were obtained from GenBank (http://www.ncbi.nlm.nih.gov). These were aligned with the L. baicalensis endosymbiont sequence using MAFFT (the alignment is available as Supplementary file 1) and the alignment was analysed by distance [neighbour-joining (NJ) (Van de Peer & De Wachter, Citation1994), using maximum likelihood-corrected distances based on the J2:G:4 model] and maximum likelihood, using Treefinder, version of October 2008 (Jobb et al., Citation2004). The Bootstrap analyses were performed using distance (NJ, 1000 replicates) and maximum likelihood (ML 1000 replicates) criteria. The ITS-1, 5.8S and ITS-2 rRNA gene sequence of the L. baicalensis endosymbiont was aligned with those of 21 strains of Mychonastes from GenBank (alignment in Supplementary information, Fig. 4) and phylogenetic analyses performed as described above.
Results and discussion
Isolation, cultivation and morphology of the sponge endosymbiont
Lubomirskia baicalensis (class Demospongiae, order Haplosclerida, family Lubomirskiidae) is a sponge with an encrusting base, which bears erect axes (generally 30–60 cm high but up to 1 m) that branch dichotomously and have rounded apices (Fig. 1). The branches vary from 1 to 4 cm in diameter and are cylindrical to flattened. The colour of live specimens is a brilliant green, which is due to the symbionts inhabiting the outer layer of the sponge (Efremova, Citation1981). Lubomirskia baicalensis is common on rocks, boulders and wood along the entire shoreline of Lake Baikal, at depths of 3–4 m to more than 50 m. The optimal habitat for flourishing dense populations occurs at depths of 5–8 m.
Primmorphs from L. baicalensis (Fig. 2), obtained as described above, were green, suggesting the presence of chlorophyll-producing organisms. Microalgae were easily seen in LM (Figs 3, 4) and were localized within amoebocytes isolated from the primmorphs (see Materials and methods). Comparisons of the morphology of the microalgae observed within amoebocytes of an adult L. baicalensis sponge and those in amoebocytes from primmorphs (Figs 3–6) confirmed good survival of microalgae after the isolation procedure. Moreover, the isolation technique we used was successful in the subsequent cultivation of algal cells on both NBW and 1.5% Bacto-Agar-based medium (Figs 10–12, S1–S3).
Figs 5–9. Electron microscopy of the Mychonastes endosymbiont of L. baicalensis. 5, 6. Transmission electron micrographs of cross-sections from microalgal endosymbionts within amoebocytes obtained from the L. baicalensis sponge (Fig. 5) and within amoebocytes isolated from primmorph culture of the same sponge (Fig. 6). 7, 8. Transmission electron microscopy of endosymbiotic algae isolated from primmorphs of L. baicalensis after 6 months’ cultivation: individual cells on NBW (Fig. 7) and on 1.5% Bacto-agar (Fig. 8). Abbreviations: N, – nucleus, M – mitochondrion; S – starch grains, C – chloroplast, L – lipid globule. 9. A single symbiont cell, SEM, showing the characteristic rib-like ornamentation on the cell wall and polysaccharide-like threads. Scale bars = 1 µm.
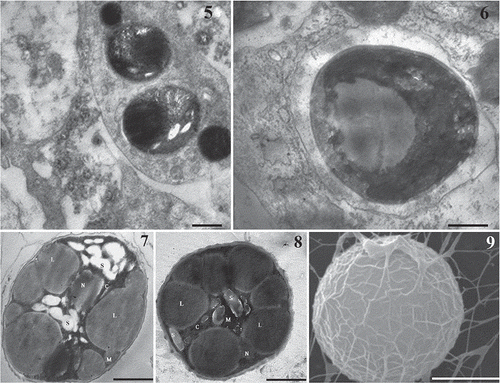
The morphology and ultrastructure of the algae isolated from primmorphs and cultivated under different conditions are shown in Figs 7 and 8. The alga was unicellular and solitary. Cells were spheroidal and 2.0–3.5 μm in diameter. Light microscopy showed that the cell wall was thick, with an irregular network of ribs on the surface, which were seen more clearly in SEM (Fig. 9). The cell wall was two-layered (Figs 7, 8). Each cell had a single chloroplast, which was parietal and cup-shaped, and lacked a pyrenoid. Starch grains were also clearly visible, scattered among the thylakoids. Using TEM, we observed a great number of large lipid globules inside the microsymbiont cells during extended cultivation in both Baikal water and 1.5% Bacto-Agar (Figs 7, 8). The composition and origin of these lipids are as yet unknown. Microalgal cells showed typical chloroplast autofluorescence (Fig. 11) and the small nuclei were revealed by DAPI staining (Fig. 12).
Figs 10–12. Light and epifluorescent microscopic pictures of the symbiotic microalgae isolated from primmorphs of L. baicalensis and cultivated in natural Baikal water. 10. Cells viewed with bright field optics. 11. Epifluorescence microscopy, showing chloroplast autofluorescence. 12. DAPI staining, showing microalgae cells containing chloroplasts and nuclei (blue). Scale bars: 10 µm.
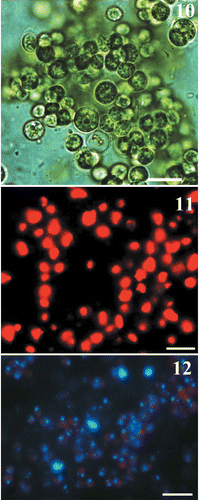
The cellular morphology of the isolate suggested that the endosymbiont is related to the genus Mychonastes, due to its characteristic two-layered cell wall (Fig. 6) with a network of ribs (Fig. 7), and the absence of a pyrenoid, which is one of the characteristic properties of Mychonastes (Krienitz et al., Citation2011). A similar cellular morphology was described for M. homosphaera from a freshwater lake by Hanagata et al. (Citation1999). However, the morphology of the endosymbionts (Figs 3–12) did not offer enough characteristics to determine their relationships within Mychonastes, nor even to confirm that they belong to this genus. Moreover, in the light microscope it is also difficult to discriminate these green algae from the freshwater picoplanktonic eustigmatophyte Nanno-chloropsis limnetica (Krienitz et al., Citation2000), which is also present in Lake Baikal (Fietz et al., Citation2005). Therefore, it was necessary to use more effective methods for the identification of the microalga – via molecular markers – which are described below.
We continued to perform daily observations on the dynamics of the growth of isolated endosymbionts, using haemocytometer technique on both NBW and 1.5% Bacto-agar, for a period of 12 months (Fig. 13). It seemed that the microalga needed some time for adaptation to these artificial conditions at temperatures of 3–4°C, as growth was slow and there was initially no visible increase in biomass. Significant growth and biomass increase were observed only after 6 months of cultivation. Interestingly, these cultures have subsequently continued to grow for a further 2 years.
Molecular characterization of the endosymbiont
After isolation of its total DNA, we amplified, cloned and sequenced the SSU and ITS rRNA gene fragments and showed that microalgal endosymbionts isolated from amoebocytes are related to Mychonastes. The results of the gene sequencing from the 18S rRNA using universal eukaryotic probes showed genetic affinity of the cultivated intracellular eukaryotic algae from primmorphs of Baikal sponge L. baicalensis to the coccoid green algal genus, Mychonastes. The full sequence of the 18S rRNA gene fragments, internal transcribed spacer 1, 5.8S RNA gene, internal transcribed spacer 2, and part of 28S rRNA gene had a length of 2660 bp. The initial BLAST search suggested that M. huancayensis strain SAG 89.81 was the best hit with 96% identity and 96% coverage. Mychonastes jurisii strain CCAP 260/1 was the second hit with 96% identity and 89% coverage. These preliminary results indicated that the cultures isolated belong to Mychonastes sp. (Chlorophyceae). We therefore made a phylogenetic analysis of 18S rDNA sequences from 29 strains of chlorophycean algae, which confirmed that the sponge symbiont does indeed belong to the genus Mychonastes (Fig. 14; we corrected ‘Mychonastes’ zofingensis to Chromo- chloris in the 18S rDNA gene sequences, following Fučíková & Lewis, Citation2012). Subsequent analyses of ITS sequences – this marker was used by Krienitz et al. (Citation2011) in their recent revision of the genus – from 21 strains of Mychonastes confirmed that the Mychonastes symbiont of L. baicalensis is a close relative of M. huancayensis and M. jurisii (Fig. 15). These three species grouped together with high bootstrap support. The overall topology of the phylogenetic tree was similar to the maximum-likelihood tree of the genus Mychonastes previously published by Krienitz et al. (Citation2011), based on 5.8S and ITS-2 rRNA gene sequences.
Fig. 14. Maximum-likelihood (ML) phylogenetic tree based on SSU rRNA gene sequences showing the position of Mychonastes among chlorophycean green algae, and the position of Mychonastes sp. sponge symbiont within the Mychonastes clade. Numbers at branches indicate ML/NJ bootstrap support (< 90%) from maximum likelihood (1000 replicates) and neighbour joining (1000 replicates).
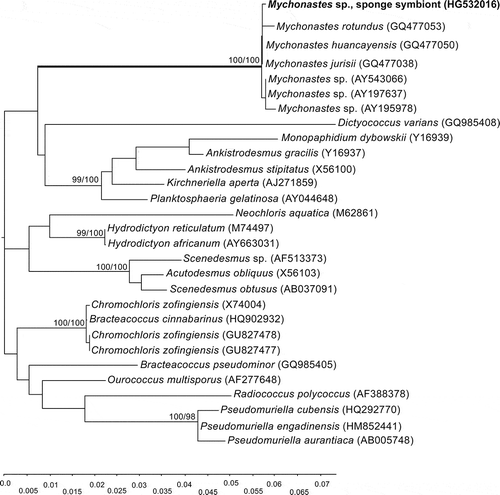
Fig. 15. Maximum-likelihood phylogenetic tree based on ITS-1, 5.8S and ITS-2 rRNA gene sequences (unrooted). Numbers above branches indicate ML/NJ bootstrap support from maximum likelihood (1000 replicates) and neighbour joining (1000 replicates).
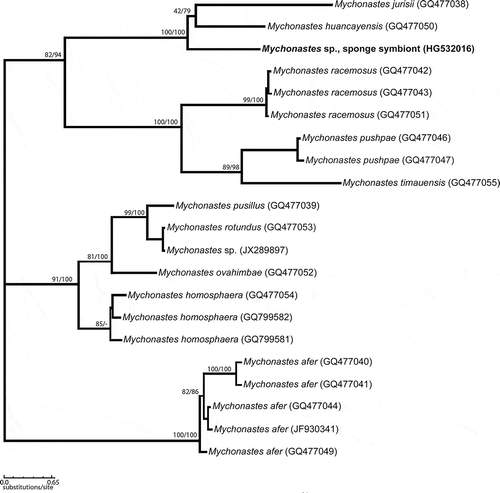
Mychonastes: both free-living and symbiotic
The widespread occurrence of eukaryotic autotrophic picoplankton in Lake Baikal has been established using LM and epifluorescence microscopy (reviewed by Fietz et al., Citation2005). A chlorophycean strain from Lake Baikal was identified as Choricystis minor by 18S rRNA gene sequencing (Belykh et al., Citation2000), and green algae have been suggested to dominate the eukaryotic picoplankton of Lake Baikal (Nagata et al., Citation1994; Belykh et al., Citation2000). In 2005, Fietz and co-authors reported Nannochloropsis limnetica (Eustigmatophyceae) from the lake. However, to date, very few other picoplanktonic species have been identified from Baikal, and the sponge endosymbionts have also remained uncharacterized. To our knowledge, there have been no previous reports of Mychonastes from the free-living plankton of Baikal or from any sponge, here or elsewhere. Therefore, the isolation and identification of Mychonastes sp. as a picophyte symbiont of L. baicalensis is unexpected and interesting.
The chlorophycean green alga Mychonastes (usually living as a solitary organism) has been excellently characterized using SSU and ITS rRNA gene sequence analyses and microscopical observations (Krienitz et al., Citation2011). Members of this genus are among the most common chlorophycean algae (Hanagata, Citation1998) occurring in the picoplankton and smaller nanoplankton of fresh and brackish waters (Krienitz & Bock, Citation2012). Some species, like M. elegans (Bachmann et al., Citation1985; Krienitz et al., Citation2011), are marine, while the optimum growth of M. ruminatus is at salinities of around 15 ppt (Simpson et al., Citation1978). Another species, M. desiccatus, grows optimally in concentrations corresponding to full-strength seawater (Margulis et al., Citation1988) and, intriguingly, upon desiccation this species forms an achlorophyllous, lipid-filled cyst in which no plastid is evident. The formation of such cysts allows the alga to survive the frequent and intermittent dry periods in its natural habitat (Margulis et al., Citation1988). In freshwater lakes, Mychonastes species probably play an important role in biomineralization. For example, Dittrich et al. (Citation2004) reported precipitation of calcium carbonate (calcite) on the surface of a Mychonastes isolated from Lake Lucerne (Switzerland). Numerous Mychonastes species have been described from the inland waters of Europe, Africa, Asia and South America. Some morphological criteria are useful for distinguishing species, such as the shape and size of the cells and the organization of mucilaginous strands among the cells of colonies, but the main diagnostic features are genetic, particularly ITS sequences (Krienitz et al., Citation2011; Krienitz & Bock, Citation2012). However there are few reports of Mychonastes as symbionts: for example, none of the species studied by Krienitz et al. (Citation2011) are listed as symbiotic. Only recently has it been shown that a Mychonastes species is the symbiont of green hydra, living in gastrodermal myoepithelial cells (Kovačević et al., Citation2010).
The Lubomirskia–Mychonastes symbiosis
Endosymbiosis ‘generally leads to enhanced ecosystem production and greater accumulation of algal biomass, due to hosts providing a refuge from predation and the efficient recycling of metabolites’ (Johnson, Citation2011). Indeed, among metazoa in aquatic habitats, like sponges, endosymbiosis is far more common in low nutrient environments (Stoecker et al., Citation2009), and therefore it is not surprising to see this in representatives of poriferan fauna of Lake Baikal. As in other lower animals, such as green hydra, the symbiotic role of zoochlorellae in Porifera has been studied mainly in the context of being a nutrient supply to the animal cells, or having an influence on the hatching of gemmules in freshwater sponges (Kanayama & Kamishima, Citation1990; Okuda et al., Citation2002). Symbiosis between algae and freshwater sponges is mainly understood as mutual benefits of photosynthesis, i.e. photosynthetic products such as oxygen and nutrients passing from algae to sponge, and metabolic wastes such as carbon dioxide and phosphate from sponge to algae (Wilkinson, Citation1980); symbiotic algae are even contained in some amphibian eggs laid in poorly aerated waters, to ensure sufficient oxygen (Bachmann et al., Citation1985). We suggest that this phenomenon could play a crucial role in L. baicalensis. It is well known that freshwater sponges usually flourish during warm seasons and disappear in winter (Okuda et al., Citation2002). However, L. baicalensis, as well as other representatives of the family Lubomirskidae, are perennial and retain their green colouring (see Fig. 1) even during the harsh Siberian winter, when they are located under a layer of ice about a metre thick. These sponges have no gemmules and so the suggestion about the possible role of Mychonastes sp. in stimulating hatching is irrelevant in this case. However, possibly the endosymbiont may be involved in the ‘larval explosion’ that can be observed in the lake during February–March each year.
Our experimental results show that both L. baicalensis primmorphs (Chernogor et al., 2011a) and Mychonastes sp. cells grow at temperatures between 3 and 4°C in vitro over long periods of cultivation (see Fig. 13). Primmorphs died at temperatures over 15°C and this may reflect the temperature limit for growth of the endosymbiont algae, something that can be tested with the culture approach described here. Temperature limitation may also occur in other Mychonastes species. For example, the growth rate of M. homosphaera (Lake Kinneret, Israel) is maximal at 14°C, whereas at higher temperatures, or after transferring cultures from low to high temperatures, growth was slower (Malinsky-Rushansky et al., Citation2002); correspondingly, picoeukaryotes were found in Lake Kinneret in winter and spring when temperatures were low (14–18°C), but not in summer and autumn when temperatures were higher (~28°C) (Malinsky-Rushansky et al., Citation1995).
Primmorphs generated from dissociated cells of different sponges are well-known model systems for studies of spiculogenesis, cell proliferation and cell death (for a review, see Valisano et al., Citation2012). Here, we show for the first time that it is possible to use the primmorphs of sponges for the isolation and subsequent cultivation of their endosymbiotic algae. Our results indicate that there is no need to use fetal bovine serum (FBS) or classical nutrient medium (e.g. Dulbecco's modified eagle's medium) containing antibiotics (De Rosa et al., Citation2001, Citation2003) for cultivation of either the Baikal sponge primmorphs or their Mychonastes endosymbionts. The data obtained confirm our previously published suggestion (Chernogor et al., 2011a, b) that specific symbiotic algae provide the sponge, as well as the primmorphs, with necessary nutrition.
Concluding remarks
Strains of Chlorophyceae, Eustigmatophyceae and Haptophyceae are used to produce biofuels and other industrial organic chemicals (Georgianna & Mayfield, Citation2012). The coccoid green algae are the most diverse group of freshwater planktonic algae and provide a wide range of experimental opportunities for studying the genesis and ecological advantages of these organisms (Krienitz & Bock, Citation2012). Mychonastes has already attracted the attention of biotechnologists. Some Mychonastes species, like Mychonastes afer, have been used as an economic feedstock for biodiesel production (Yuan et al., Citation2011), and comparisons of the fatty acid contents of green (Choricystis, Mychonastes) and eustigmatophycean (Nannochloropsis) freshwater picoplanktonic algae have revealed significant differences in the composition of polyunsaturated fatty acids (Krienitz & Wirth, Citation2006). Earlier studies showed that a number of polyunsaturated fatty acids are found among the lipids of Baikal sponges (Latyshev et al., Citation1992) and our discovery of a way to isolate the Mychonastes endosymbiont will allow detailed studies of the amounts and spectrum of the lipids that are accumulated under different culture conditions, and of lipid metabolism.
Supplementary information
The following supplementary material is available for this article, accessible via the Supplementary Content figures on the article's online page at http://dx.doi.org/10.1080/09670262.2013.862306
Supplementary Figs S1–S3. Light and epifluorescent microscopic pictures of the symbiotic microalgae isolated from primmorphs of L. baicalensis and cultivated using 1.5% Bacto-agar. S1. Living microalgae. S2. Epifluorescent microscopic pictures showing chloroplast autofluorescence. S3. DAPI stained cells showing chloroplasts and nuclei. Scale bars = 10 µm.
Supplementary Fig. S4. Alignment of ITS-1, 5.5S, ITS-2 and part of LSU of Mychonastes species.
Supplementary file. Alignment of 18S rDNA sequences in Mychonastes and other chlorophycean algae (fasta format).
Supplementary material
Download Zip (2.8 MB)Acknowledgements
This work was supported by the Basic project ‘Sponges’, the Program 6.1.1., the Integration Project SB RAS № 38–2009, by the DFG (grant EH 394/1-1) as well as by the BHMZ Programme of the Dr.-Erich-Krüger Foundation (Germany) at TU Bergakademie Freiberg. We thank D. Fomin for technical assistance in scanning electron microscopy and transmission electron microscopy. The authors are indebted to the service staff of the Microscopy Centre at the A.V. Zhirmunsky Institute of Marine Biology, FEB RAS. We cordially thank Allison L. Stelling for technical support.
References
- Agnes, B. & Brondsted, H.V. (1953). The effect of symbiotic zoochlorellae on the germination rate of gemmules of Spongilla lacustris (L.). Videnskabelige Meddelelser Naturhistorisk Forening i København, 115: 133–144.
- Annenkova, N.V., Lavrov, D.V. & Belikov, S.I. (2011). Dinoflagellates associated with freshwater sponges from the ancient Lake Baikal. Protist, 162: 222–236.
- Ávila, E., Carballo, J.L., José, A. & Cruz-Barraza, J.A. (2007). Symbiotic relationships between sponges and other organisms from the Sea of Cortes (Mexican Pacific coast): same problems, same solutions. In Porifera research: biodiversity, innovation and sustainability (Custódio, M.R., Lôbo-Hajdu, G., Hajdu, E. & Muricy, G., editors), 147–155. Série Livros 28. Museu Nacional, Rio de Janeiro.
- Bachmann, M.D., Carton, R.G., Buckholden, J.M. & Wetzel, R.G. (1985). Symbiosis between salamander eggs and green algae: microelectrode measurements inside eggs demonstrate effect of photosynthesis on oxygen concentration. Canadian Journal of Zoology, 64: 1586–1588.
- Bailey, R.C., Day, K.E., Norris, R.H. & Reynoldson, T.B. (1995). Macroinvertebrate community structure and sediment bioassay results from nearshore areas of North American Great Lakes. Journal of Great Lakes Research, 21: 42–52.
- Bayer, K., Schmitt, S. & Hentschel, U. (2007). Microbial nitrification in Mediterranean sponges: possible involvement of ammonia-oxidizing Betaproteobacteria. In Porifera research: biodiversity, innovation and sustainability (Custódio, M.R., Lôbo-Hajdu, G., Hajdu, E. & Muricy, G., editors), 165–171. Série Livros 28. Museu Nacional, Rio de Janeiro.
- Belykh, O.I., Semenova, E.A., Kuznedelov, K.D., Zaika, E.I. & Guselnikova, N.E. (2000). A eukaryotic alga from picoplankton of Lake Baikal: morphology, ultrastructure and rDNA sequence data. Hydrobiologia, 435: 83–90.
- Bil, K., Titlyanov, E., Berner, T., Fomina, I. & Muscatine, L. (1999). Some aspects of the physiology and biochemistry of Lubomirskia baicalensis, a sponge from Lake Baikal containing symbiotic algae. Symbiosis, 26: 179–191.
- Bondarenko, N.A. (1995). Taxonomic list of planktonic algae. In Guide and key to pelagic animals of Baikal with ecological notes (Timoshkin, O.A., editor), 621–630. Nauka, Novosibirsk. [In Russian.]
- Brien, P. (1932). Contribution a l‘étude de la régénération naturelle chez les Spongillidae. Spongilla lacustris (L.), Ephydatia fluviatilis (L.). Archives de Zoologie Expérimentale et Générale, 74: 461–506.
- Brondsted, A. & Brondsted, H.V. (1953). The effect of symbiontic zoochlorellae on the germination rate of gemmules of Spongilla lacustris (L.). Videnskabelige Meddelelser Dansk Naturhistorisk Forening, 115: 133–145.
- Cao, X., Fu, W., Yu, X. & Zhang, W. (2007). Dynamics of spicule production in the marine sponge Hymeniacidon perlevis during in vitro cell culture and seasonal development in the field. Cell & Tissue Research, 329: 595–608.
- Castro-Rodriguez, G. (1930). De la symbiose entre Spongilla lacustis et les zoochlorelles. Annales de la Société Royale Zoologique de Belgique, 61: 113–121.
- Chernogor, L.I., Denikina, N.N., Belikov, S.I. & Ereskovsky, A.V. (2011a). Long-term cultivation of primmorphs from freshwater Baikal sponges Lubomirskia baicalensis. Marine Biotechnology, 13: 782–792.
- Chernogor, L.I., Denikina, N.N., Belikov, S.I. & Ereskovsky, A.V. (2011b). Formation of spicules during the long-term cultivation of primmorphs from the freshwater Baikal sponge Lubomirskia baicalensis. Organic Chemistry: Current Research, S:2:001. doi:10.4172/2161-0401.S2–001.
- Custodio, M.R., Prokic, I., Steffen, R., Koziol. C., Borojevic, R., Brummer, F., Nikel, M. & Müller, W.E.G. (1998). Primmorphs generated from dissociated cells of the sponge Suberites domuncula: a model system for studies of cell proliferation and cell death. Mechanisms of Ageing and Development, 105: 45–59.
- Davy, S.K., Trautman, D.A., Borowitzka, M.A. & Hinde, R. (2002). Ammonium excretion by a symbiotic sponge supplies the nitrogen requirements of its rhodophyte partner. Journal of Experimental Biology, 205: 3505–3511.
- De Rosa, S., De Caro, S., Tommonaro, G., Slantchev, K., Stefanov, K. & Popov, S. (2001). Development in a primary cell culture of the marine sponge Ircinia muscarum and analysis of the polar compounds. Marine Biotechnology, 3: 281–286.
- De Rosa, S., De Caro, S., Iodice, C., Tommonaro, G., Stefanov, K. & Popov, S. (2003). Development in primary cell culture of demosponges. Journal of Biotechnology, 100: 119–125.
- Dittrich, M., Kurz, P. & Wehrli, B. (2004). The role of autotrophic picocyanobacteria in calcite precipitation in an oligotrophic lake. Geomicrobiology Journal, 21: 45–53.
- Efremova, S.M. (1981). The structure and embryogenesis of the Baikal sponge Lubomirskia baicalensis (Pallas) and relations of Lubomirskiidae with other sponges. In Morphogenesis in sponges (Korotkova, G.P., editor), 93–107. Leningrad University, Leningrad.
- Fietz, S., Bleiß, W., Hepperle, D., Koppitz, H., Krienitz, L. & Nicklisch, A. (2005). First record of Nannochloropsis limnetica (Eustigmatophyceae) in the autotrophic picoplankton from Lake Baikal. Journal of Phycology, 41: 780–790.
- Frost, T.M. & Williamson, C.E. (1980). In situ determination of the effect of symbiotic algae on the growth of the freshwater sponge Spongilla lacustris. Ecology, 61: 1361–1370.
- Frost, T.M., De Nagy, G.S. & Gilbert, J.J. (1982). Population dynamics and standing biomass of the freshwater sponge Spongilla lacustris. Ecology, 63: 1203–1210.
- Frost, T.M., Graham, L.E., Elias, J.E., Haase, M.J., Kretchmer, D.W. & Kranzfelder, J.A. (1997). A yellow-green algal symbiont in the freshwater sponge, Corvomeyenia everetti: convergent evolution of symbiotic associations. Freshwater Biology, 38: 395–399.
- Fučíková, K. & Lewis, L.A. (2012). Intersection of Chlorella, Muriella and Bracteacoccus: resurrecting the genus Chromochloris Kol et Chodat (Chlorophyceae, Chlorophyta). Fottea, 12: 83–93.
- Georgianna, D.R. & Mayfield, S.P. (2012). Exploiting diversity and synthetic biology for the production of algal biofuels. Nature, 488: 329–335.
- Gilbert, J.J. & Allen, H.L. (1973a). Studies on the physiology of the green freshwater sponge Spongilla lacustris: primary productivity, organic matter, and chlorophyll content. Verhandlungen. Internationale Vereinigung für Theoretische und Angewandte Limnologie, 18: 1413–1420.
- Gilbert, J.J. & Allen, H.L. (1973b). Chlorophyll and primary productivity of some green, freshwater sponges. Internationale Revue der gesamten Hydrobiologie, 58: 633–658.
- Hall, T.A. (1999). BioEdit: a user-friendly biological sequence alignment editor and analysis program for Windows 95/98/NT. Nucleic Acids Symposium Series, 41: 95–98.
- Hanagata, N. (1998). Phylogeny of the subfamily Scotiellocystoideae (Chlorophyceae, Chlorophyta) and related taxa inferred from 18S ribosomal RNA gene sequence data. Journal of Phycology, 34: 1049–1054.
- Hanagata, N., Malinsky-Rushansky, N. & Dubinsky, Z. (1999). Eukaryotic picoplankton, Mychonastes homosphaera (Chlorophyceae, Chlorophyta), in Lake Kinneret, Israel. Phycological Research, 47: 263–269.
- Hentschel, U., Usher, K.M. & Taylor, M.W. (2006). Marine sponges as microbial fermenters. FEMS Microbiology Ecology, 55: 167–177.
- Höller, U., Wright, A.D., Matthee, G.F., König, G.M., Draeger, S., Aust, H.J. & Schulz, B. (2000). Fungi from marine sponges: diversity, biological activity and secondary metabolites. Mycological Research, 104: 1354–1365.
- Ilan, M., Contini, H., Carmeli, S. & Rinkevich, B. (1996). Progress towards cell cultures from a marine sponge that produces bioactive compounds. Journal of Marine Biotechnology, 4: 145–149.
- Jewson, D.H., Granin, N.G., Zhdarnov, A.A., Gorbunova, L.A., Bondarenko, N.A. & Gnatovsky, R.Yu (2008). Resting stages and ecology of the planktonic diatom Aulacoseira skvortzowii in Lake Baikal. Limnology and Oceanography, 53: 1125–1136.
- Jobb, G., von Haeseler, A. & Strimmer, K. (2004). TREEFINDER: A powerful graphical analysis environment for molecular phylogenetics. BMC Evolutionary Biology, 4: 18, doi:10.1186/1471-2148-4-18.
- Johnson, M.D. (2011). The acquisition of phototrophy: adaptive strategies of hosting endosymbionts and organelles. Photosynthesis Research, 107: 117–132.
- Kanayama, M., & Kamishima, Y. (1990). Role of symbiotic algae in hatching of gemmules of the freshwater sponge, Radiospongilla cerebellata. Zoological Science, 7: 649–655.
- Katoh, K. & Toh, H. (2008). Improved accuracy of multiple ncRNA alignment by incorporating structural information into a MAFFT-based framework. BMC Bioinformatics, 9: 212, doi: 10.1186/1471-2105-9-212.
- Klautau, M., Custodio, M.R. & Borojevic, R. (1993). Cell cultures of sponges Clathrina and Polymastia. In Vitro Cellular and Developmental Biology, 29: 97–99.
- Kovačević, G., Franjević, D., Jelenčić, B. & Kalafatić, M. (2010). Isolation and cultivation of endosymbiotic algae from Green Hydra and phylogenetic analysis of 18S rDNA sequences. Folia Biologica (Kraków), 58: 135–143.
- Kozhova, O.M. & Izmest’eva, L.R. (1998). Lake Baikal: evolution and biodiversity. Backhuys Publishers, Leiden.
- Krienitz, L. & Bock, C. (2012). Present state of the systematics of planktonic coccoid green algae of inland waters. Hydrobiologia, 698: 295–326.
- Krienitz, L. & Wirth, M. (2006). The high content of polyunsaturated fatty acids in Nannochloropsis limnetica (Eustigmatophyceae) and its implication for food web interactions, freshwater aquaculture and biotechnology. Limnologica, 36: 204–210.
- Krienitz, L., Hepperle, D., Stich, H.-B. & Weiler, W. (2000). Nannochloropsis limnetica (Eustigmatophyceae), a new species of picoplankton from freshwater. Phycologia, 39: 219–227.
- Krienitz, L., Bock, C., Dadheech, P.K. & Pröschold, T. (2011). Taxonomic reassessment of the genus Mychonastes (Chlorophyceae, Chlorophyta) including the description of eight new species. Phycologia, 50: 89–106.
- Latyshev, N.A., Zhukova, N.V., Efremova, S.M., Imbs, A.B. & Glysina, O.I. (1992). Effect of habitat on participation of symbionts in formation of the fatty acid pool of freshwater sponges of Lake Baikal. Comparative Biochemistry and Physiology Part B: Comparative Biochemistry, 102: 961–965.
- Lewin, R.A. (1966). Kultivo de zoochlorella apartigita el spongo. Scienca Revuo Internacia Sciencia Asocio Esperantista, 17: 33–36.
- Love, G.D., Grosjean, E., Stalvies, C., Fike, D.A., Grotzinger, J.P., Bradley, A.S., Kelly, A.E., Bhatia, M., Meredith, W., Snape, C.E., Bowring, S.A., Condon, D.J. & Summons, R.E. (2009). Fossil steroids record the appearance of Demospongiae during the Cryogenian period. Nature, 457: 718–721.
- Malinsky-Rushansky, N., Berman, T. & Dubinsky, Z. (1995). Seasonal dynamics of picophytoplankton in Lake Kinneret, Israel. Freshwater Biology, 34: 241–254.
- Malinsky-Rushansky, N., Berman, T., Berner, T, Yacobi, Y.Z. & Dubinsky, Z. (2002). Physiological characteristics of picophytoplankton, isolated from Lake Kinneret: responses to light and temperature. Journal of Plankton Research, 24: 1173–1183.
- Margulis, L., Hinkle, G., McKhann, H. & Moynihan, B. (1988). Mychonastes desiccatus Brown sp. nova (Chlorococcales, Chlorophyta) – an intertidal alga forming achlorophyllous desiccation-resistant cysts. Archiv für Hydrobiologie, 78 (Algological Studies, 49): 425–446.
- Miller, S.A. (1964). The effect of symbiotic algae on the growth of Spongilla lacustris. Master’s Thesis, University of Washington, Seattle, USA.
- Müller, K. (1911). Das Regenerationsvermögen der Süsswasserschwämme, insbesondere Untersuchungen über die bei ihnen vorkommende Regeneration nach Dissociation and Reunition. Archiv für Entwicklungsmechanik der Organismen, 32: 397–446.
- Müller, W.E.G., Wiens, M., Batel, R., Steffen, R., Schröder, H.C., Borojevic, R. & Custodio, M.R. (1999). Establishment of a primary cell culture from a sponge: primmorphs from Suberites domuncula. Marine Ecology Progress Series, 178: 205–219.
- Müller, W.E.G., Belikov, S.I., Kaluzhnaya, O.V., Chernogor, L., Krasko, A. & Schröder, H.C. (2009). Symbiotic interaction between dinoflagellates and the demosponge Lubomirskia baicalensis: aquaporin-mediated glycerol transport. In Biosilica in evolution, morphogenesis, and nanobiotechnology (Müller, W.E.G. & Grachev, M.A., editors), 145–170. Springer, Heidelberg.
- Muscatine, L., Karakashian, S.J. & Karakashian, M.W. (1967). Soluble extracellular products of algae symbiotic with a ciliate, a sponge and mutant hydra. Comparative Biochemistry and Physiology, 20: 1–12.
- Nagata, T.K., Takai, K., Kawanobe, D.-S., Kim, R., Nakazato, N., Guselnikova, N., Bondarenko, O., Mologawaya, T., Kostrnova, V., Drucker, V., Satoh, Y. & Watanabe, Y. (1994). Autotrophic picoplankton in southern Lake Baikal: abundance, growth and grazing mortality during summer. Journal of Plankton Research, 16: 945–959.
- Okuda, N., Yamamoto, A., Satoh, Y., Fujimoto, Y. & Kamishim, Y. (2002). Role of symbiotic algae on gemmule Germination of a freshwater sponge, Radiospongilla cerebellata. Chugokugakuen Journal, 1: 7–12.
- Palumbi, S.R. (1985). Spatial variation in an algal-sponge commensalism and the evolution of ecological interactions. American Naturalist, 126: 267–274.
- Penney, J.T. (1933). Reduction and regeneration in freshwater sponges (Spongilla discoides). Journal of Experimental Zoology, 65: 475–497.
- Philippe, H., Derelle, R., Lopez, P., Pick, K., Borchiellini, C., Boury-Esnault, N., Vacelet, J., Renard, E., Houliston, E., Quéinnec, E., Da Silva, C., Wincker, P., Le Guyader, H., Leys, S., Jackson, D.J., Schreiber, F., Erpenbeck, D., Morgenstern, B., Wörheide, G. & Manuel, M. (2009). Phylogenomics revives traditional views on deep animal relationships. Current Biology, 19: 706–712.
- Pile, A.J., Patterson, M.R., Savarese, M., Chernykh, V.I. & Fialkov, V.A. (1997). Trophic effects of sponge feeding within Lake Baikal’s littoral zone. Sponge abundance, diet, feeding efficiency, and carbon flux. Limnology and Oceanography, 42: 178–184.
- Pomponi, S.A. (2006). Biology of the Porifera: cell culture. Canadian Journal of Zoology, 84: 167–174.
- Popovskaya, G.I. (1968). Novyj vid roda Synechocystis Sauv, v planktone ozera Bajkal [A new species of the genus Synechocystis Sauv. in Lake Baikal plankton]. Novosti sistematiki nizshikh rastenij, 1968: 3–5.
- Potts, E. (1887). Freshwater sponges. A monograph. Proceedings of the Academy of Natural Sciences of Philadelphia, 1887: 157–279.
- Preston, C.M., Wu, K.Y, Molinski, T.F. & DeLong, E.F. (1996). A psychrophilic crenarchaeon inhabits a marine sponge: Cenarchaeum symbiosum gen. nov. sp. nov. Proceedings of the National Academy of Sciences of the United States of America, 93: 6241–6246.
- Pröschold, T., Darienko, T., Silva, P.C., Reisser, W. & Krienitz, L. (2011). The systematics of Zoochlorella revisited employing an integrative approach. Environmental Microbiology, 13: 350–364.
- Reisser, W. & Hader, D.P. (1984). Role of endosymbiotic algae in photokinesis and photophobic responses of ciliates. Photochemistry and Photobiology, 39: 673–678.
- Reitner, J. & Wörheide, G. (2002). Non-lithistid fossil Demospongiae? Origins of their palaeobiodiversity and highlights in history of preservation. In Systema Porifera: a guide to the classification of sponges (Hooper, J.N.A. & van Soest, R.W.M., editors), 52–70. Kluwer Academic and Plenum Publishers, New York.
- Rezvoj, P.D. (1936). Freshwater sponges Fam. Spongillidae & Lubomirskiidae, Fauna URSS. Vol. 2. Academie Sciences URSS, Moscow.
- Rinkevich, B. (1999). Cell cultures from marine invertebrates: obstacles, new approaches and recent improvements. Journal of Biotechnology, 70: 133–153.
- Rützler, K. (1990). Associations between Caribbean sponges and photosynthetic organisms. In New perspectives in sponge biology (Rützler, K., editor), 455–466. Smithsonian Institution Press, Washington, DC.
- Sara, M. (1971). Ultrastructural aspects of the symbiosis between two species of the genus Aphanocapsa (Cyanophyceae) and Ircinia (Demospongiae). Marine Biology, 11: 214–221.
- Schröder, K. (1926). Carterius stepanowi (Dyb.) im Osten Deutschlands. Zugleich ein Überblick über die Süsswasserschwammfauna Schlesiens. Zoologischer Anzeiger, 67: 240–250.
- Simpson, P.D., Karlander, E. P. & Van Valkenburg, S.D. (1978). The growth rate of Mychonastes ruminatus Simpson et Van Valkenburg under various light, temperature and salinity regimes. British Phycological Journal, 13: 291–298.
- Spurr, A.R. (1969). A low-viscosity epoxy resin embedding medium for electron microscopy. Journal of Ultrastructural Research, 26: 31–43.
- Steindler, L., Beer, S. & Ilan, M. (2002). Photosymbiosis in intertidal and subtidal tropical sponges. Symbiosis, 33: 263–273.
- Stoecker, D.K., Johnson, M.D., de Vargas C & Not, F. (2009). Acquired phototrophy in aquatic protists. Aquatic Microbial Ecology, 57: 279–310.
- Strober, W. (2001). Monitoring cell growth. Current Protocols in Immunology, 21: A.3A.1–A.3A.2.
- Taylor, M.W., Schupp, P.J., Dahllof, I., Kjelleberg, S. & Steinberg, P.D. (2004). Host specificity in marine sponge-associated bacteria, and potential implications for marine microbial diversity. Environmental Microbiology, 6: 121–130.
- Taylor, M.W., Radax, R., Steger, D. & Wagner, M. (2007). Sponge-associated microorganisms: evolution, ecology and biotechnological potential. Microbiology and Molecular Biology Reviews, 71: 295–347.
- Trautman, D.A. & Hinde, R. (2002). Sponge/algal symbioses: a diversity of associations. In Symbiosis: mechanisms and model systems (Seckbach, J., editor). Kluwer Academic Publishers, the Netherlands.
- Trautman, D.A., Hinde, R. & Borowitzka, M.A. (2000). Population dynamics of an association between a coral reef sponge and a red macroalga. Journal of Experimental Marine Biology and Ecology, 244: 87–105.
- Vacelet, J. (1971). Étude en microscopie électronique de l‘association entre une cyanophycée chroococcale et une éponge du genre Verongia. Journal of Microscopy, 12: 363–380.
- Vacelet, J. (1981). Algal-sponge symbioses in the coral reefs of New Caledonia: a morphological study. In Proceedings of the 4th International Coral Reef Symposium, vol. 2 (Gomez, E.D. & Birkeland, C.E., editors), 713–719. Marine Sciences Center, University of the Philippines, Manila.
- Valisano, L., Pozzolini, M., Giovine, M. & Cerrano, C. (2012). Biosilica deposition in the marine sponge Petrosia ficiformis (Poiret, 1789): the model of primmorphs reveals time dependence of spiculogenesis. Hydrobiologia, 687: 259–273.
- Van de Peer, Y. & De Wachter, Y. (1994). TREECON for Windows: a software package for the construction and drawing of evolutionary trees for the Microsoft Windows environment. Computer Applications in the Biosciences, 10: 569–70.
- Webster, N.S., Watts, J.E.M. & Hill, R.T. (2001). Detection and phylogenetic analysis of novel crenarchaeote and euryarchaote 16S ribosomal RNA gene sequences from a Great Barrier Reef sponge. Marine Biotechnology, 3: 600–608.
- Webster, N.S., Negri, A.P., Munro, M.M.H.G & Battershill, C.N. (2004). Diverse microbial communities inhabit Antarctic sponges. Environmental Microbiology, 6: 288–300.
- Weltner, W. (1893). Spongillidenstudien 11. Archiv für Naturgeschichte, 3: 209–274.
- White, T.J., Bruns, T, Lee, S. & Taylor, J.W. (1990). Amplification and direct sequencing of fungal ribosomal RNA genes for phylogenetics. In PCR Protocols. A guide to methods and applications (Innis, M.A., Gefland, D.H., Sninsky, J.J. & White, T.J., editors), 315–322. Academic Press, San Diego, California.
- Whitman, D.L. (1981). Affects of symbiotic zoochlorella on the respiration and deterioration of the freshwater sponge, Ephydatia fluviatilis. http://hdl.handle.net/2027.42/53473.
- Wilkinson, C.R. (1980). Nutrient translocation from green algal symbionts of the freshwater sponge, Ephydatia fluviatilis. Hydrobiologia, 75: 241–250.
- Wilkinson, C.R. (1987). Interocean differences in size and nutrition of coral reef sponge populations. Science, 236: 1654–1657.
- Wilkinson, C.R. & Garrone, R. (1980). Nutrition of marine sponges. In Nutrition in the lower Metazoa (Smith, D.C. & Tiffon, Y., editors), 157–161. Pergamon Press, Oxford.
- Williamson, C.E. (1979). An ultrastructural investigation of algal symbiosis in white and green Spongilla lacustris (L.) (Porifera: Spongillidae). Transactions of the American Microscopical Society, 98: 59–77.
- Yuan, C., Liu, J., Fan, Y., Ren, X., Hu, G. & Li, F. (2011). Mychonastes afer HSO-3-1 as a potential new source of biodiesel. Biotechnology and Biofuels, 4: 47.