Abstract
Limited molecular data for marine tube-forming diatoms are available currently and this study provides the first molecular survey of these taxa. To conduct this survey, we used a molecular-assisted alpha taxonomy (MAAT) approach that utilizes DNA barcode data. We used three DNA barcode markers: the 3´ end of the large subunit of RUBISCO (rbcL-3P); the variable D2/D3 region of the nuclear large subunit ribosomal DNA (LSU D2/D3); and the internal transcribed spacer 2 (ITS2) to assign marine tube-forming diatoms from Canada to genetic species groups. The rbcL-3P analysis uncovered 29 genetic groups including representatives of Haslea crucigera, Navicula bottnica, N. brunelii, N. ramosissima, Nitzschia fontifuga, N. tubicola, Parlibellus berkeleya and P. delognei f. elliptica, as well as a complex of 14 closely related groups morphologically consistent with Berkeleya rutilans. We sequenced ITS2 for representatives of the B. rutilans complex; these data were consistent with the rbcL-3P genetic clusters for 86% of the colonies tested. The remaining 14% were in conflict, possibly indicating that more than a single Berkeleya genetic species was present in each colony. To investigate this hypothesis further, we developed ‘species-specific’ ITS2 primers and confirmed heterogeneity of Berkeleya genetic species in 64% of the colonies tested (N = 91). Therefore, a taxonomic assessment of tube-forming species that were originally described on the basis of colony morphology (e.g. Berkeleya rutilans) can only proceed using clonal cultures or single-cell analysis.
Introduction
Diatoms are a diverse group of eukaryotic single-celled algae with an estimated 200 000 species (Mann & Droop, Citation1996) that live in a variety of freshwater and marine habitats on rocks, plants, soil, sand, etc. Diatoms are single-celled, but some species form colonies using specialized structures such as the linking spines between frustules of Aulacoseira, the mucilage pads that hold together the characteristic star-shaped colonies of Asterionella, or the fultoportulae of some Thalassiosira species that secrete chitinaceous threads to hold cells together (Round et al., Citation1990). Other diatom species form macroscopic mucilage tubes. One of these species, Berkeleya rutilans, was among the first to be classified with plants due to its macroscopic habit and resemblance to filamentous brown algae (Round et al., Citation1990).
While similar in habit, the marine diatoms that form macroscopic tubes are not a monophyletic group and belong to several unrelated genera including: Berkeleya, Gato, Gyrosigma, Haslea, Navicula, Nitzschia and Parlibellus (Round et al., Citation1990; Lobban & Navarro, 2013). Seaweed experts expecting Ectocarpales have often collected these diatoms, but they are not typically included in seaweed floras (Lobban, Citation1984). Most researchers describing floras of marine tube-forming diatoms have focused on them specifically (e.g. Aleem, Citation1949, 1950; Cox, Citation1977a, 1977b). Of particular interest for this study are the Atlantic and Pacific floras published for Canadian waters by Lobban (1984, 1985, 1990). For these floras, an extensive collection of herbarium and fresh material was analysed and the Atlantic Canadian tube-forming flora found to include Berkeleya rutilans, Haslea crucigera, Navicula bottnica, N. rusticensis, Parlibellus delognei, P. delognei f. elliptica and P. berkeleyi, with Navicula brunelii, N. mollis, N. perminuta, N. ramosissima, Nitzschia fontifuga and N. tubicola listed as cohabitants or ‘endophytes’ (i.e. diatoms that live within the tubes of other diatom species; Lobban, Citation1984). The Pacific flora (Lobban, Citation1985, 1990) included most of the species found in eastern Canada with a few exceptions: Navicula bottnica, N. brunelii, N. perminuta and Parlibellus delognei f. elliptica were absent; N. rusticensis did not form its own tubes; the additional tube-forming diatoms Berkeleya fragilis and B. micans were present; and a few new cohabitants were reported, namely Navicula ramosissima var. torquata, Nitzschia frustulum, and N. longissima. In addition to these, other diatoms and algae can also grow as epiphytes on the macroscopic tubes, including Cocconeis, Licmophora and Synedra, and a unicellular green alga, Chlorochytrium cohnii, has been reported to grow inside tube colonies in the UK (Cox, Citation1981). The most abundant taxon found on the Atlantic and Pacific coasts was B. rutilans (Lobban, Citation1984, 1985). Berkeleya rutilans has also been reported from Antarctica (Scott & Thomas, Citation2005), the Arctic (Lobban, Citation1984), Chile (Rivera, Citation1976), Europe (Ros et al., Citation2009) including the UK (Cox, Citation1977b), and Japan (Mizuno, Citation1977).
While the floras recorded by Lobban (1984, 1985, 1990) provide a starting point, species identification was based on colony and valve morphology. More recently, molecular surveys of Cyclotella meneghiniana (Beszteri et al., 2005), Eunotia bilunaris (Vanormelingen et al., Citation2008), Nitzschia palea (Trobajo et al., Citation2009, 2010) and Sellaphora pupula (Behnke et al., Citation2004) have revealed greater species diversity than expected based on morphology alone. In some cases, this unexpected diversity is congruent with changes in valve shape (e.g. in Sellaphora and Navicula cryptocephala: Mann et al., Citation2004; Poulíčková et al., Citation2010) or poroid morphology (e.g. in Pseudo-nitzschia: Amato et al., Citation2007). In other cases this diversity is cryptic and no morphological characters have been identified to distinguish between genetic species groups (e.g. in Cyclotella and Nitzschia: Beszteri et al., 2005; Trobajo et al., Citation2009, 2010).
Using molecular data to distinguish species of diatoms is becoming more common, but the best molecular marker(s) for this purpose is still debated (Evans et al., Citation2007; Moniz & Kaczmarska, Citation2009, 2010; Hamsher et al., Citation2011; Zimmerman et al., 2011). The most common markers are: the 5′ end of the cytochrome c oxidase I gene, COI-5P (Evans et al., Citation2007); the nuclear small subunit ribosomal DNA, SSU (Moniz & Kaczmarska, Citation2009; Zimmerman et al., 2011); the universal plastid amplicon, UPA (Sherwood & Presting, Citation2007; Hamsher et al., Citation2011); the large subunit of RUBISCO, rbcL (Evans et al., Citation2007, 2008; Trobajo et al., Citation2010; Hamsher et al., Citation2011); the LSU D2/D3 (Trobajo et al., Citation2010; Hamsher et al., Citation2011); and the internal transcribed spacers of rDNA (ITS) (Behnke et al., Citation2004; Amato et al., Citation2007; Moniz & Kaczmarska, Citation2009, 2010). The SSU and UPA markers have broad applicability across protistan taxa and can be easily used in environmental surveys (Godhe et al., Citation2008; Sherwood et al., Citation2008), but cannot distinguish between closely related species and therefore are not the best markers for uncovering diatom diversity (Moniz & Kaczmarska, Citation2009; Hamsher et al., Citation2011; Zimmerman et al., 2011). The COI-5P region is able to distinguish between diatom species, but cannot be easily amplified and sequenced for some diatom lineages (Hamsher et al., Citation2011), possibly due to introns in the region (Armbrust et al., Citation2004; Ravin et al., Citation2010). A region (780 bp) towards the 3′ end of the rbcL (rbcL-3P) and the variable D2/D3 region of the LSU (LSU D2/D3) have recently been suggested as species-level markers for diatoms (Hamsher et al., Citation2011). These markers display universality across diatom taxa as a single primer pair for each marker can amplify and sequence many genera and have the resolving power to differentiate between closely related species pairs (Hamsher et al., Citation2011). The ITS marker has adequate resolution to delineate diatom species, but can display intraclonal divergence (i.e. sequence divergence between copies of the ITS within a single clonal culture) that can make determining genetic species groups difficult (Behnke et al., Citation2004; Thornhill et al., Citation2007). However, intraclonal variability may not be problematic in all taxa (Moniz & Kaczmarska, Citation2009, 2010). The ITS2 region displays greater variation than ITS1 and its variability is congruent with delineation between closely related species (Amato et al., Citation2007).
As a result of the considerations discussed above, our study used three molecular regions (rbcL-3P, LSU D2/D3 and ITS2) to investigate marine tube-forming diatom diversity from Canadian Atlantic and Pacific waters, following a typical molecular-assisted alpha taxonomic approach (Saunders, Citation2008), which Cianciola et al. (Citation2010) abbreviate as ‘MAAT’. Used successfully in many macroalgal studies (e.g. Le Gall & Saunders, Citation2010), MAAT involves targeted collecting of individuals from higher-level taxonomic or functional groups (e.g. tube-forming diatoms), which are then assigned to ‘genetic species groups’ using the appropriate molecular marker(s). All of the individuals thus assigned to a ‘genetic group’ are then subjected to morphological observations to generate a comprehensive understanding of that species’ phenotype, ultimately with the intention of assigning existing names or proposing new names where appropriate. For our MAAT survey of Canadian tube-forming diatoms, our initial definition of ‘individual’ equated to individual colonies. This proved to be somewhat naïve, since a comparison of the data from the three markers suggested that tube-forming diatom colonies are more often than not composed of more than a single genetic species group. To test this hypothesis further, we developed and used ‘species-specific’ ITS2 primers in a series of trials.
Materials and methods
Collection and morphological identification of colonies
Macroscopic colonies of tube-forming diatoms were collected from both the intertidal and subtidal along the Atlantic and Pacific shorelines of Canada and the USA (). Individual colonies were preserved in 95% ethanol or pressed on herbarium paper, with a subsample placed in silica gel for DNA extraction. Silica gel-preserved subsamples were stored at −20°C. In addition, for six colonies, a small section of the colony was removed and placed in a Petri dish with F/2+Si medium (Guillard & Ryther, Citation1962) in an attempt to culture the tube-forming diatoms. Cultures were maintained at 10°C with a 12 : 12 light : dark cycle.
A portion of each colony was removed from the 95% ethanol, re-hydrated with water on the herbarium sheet, or partially harvested by centrifugation for cultures. For identification, colony fragments were mounted in 50% (v/v) corn syrup with 4% (v/v) formaldehyde to prevent microbial growth. Following rbcL-3P analysis (see below), representative colonies for each genetic species group were selected for further morphological investigation. To make slide preparations of this material, an additional fragment of each colony was removed from 95% ethanol, re-hydrated, or harvested as above, placed in a beaker with 10 ml deionized H2O, and boiled with 10 ml H2O2 for 30 min to clean the frustules. The resulting suspensions of cleaned frustules were allowed to settle for 24 h, the supernatant removed, and deionized H2O added. This settling and washing process was repeated until neutral pH was achieved. Cleaned frustules were then dried on coverslips and mounted on slides with Naphrax (Brunel Microscopes, Chippenham, UK). Slides (corn syrup and Naphrax mounted) were observed under 1000× on either a Leica DM5000B (1.35 N.A., HCX PlanApo objective) or an Olympus Bx-51 outfitted with DIC (1.40 N.A., UPlanSApo objective) light microscope and photographed using either a Leica DFC480 or an Olympus DP-71 digital camera (Leica, Heidelberg, Germany or Olympus, Tokyo, Japan, respectively). All herbarium sheets, cleaned material, and slides have been deposited in the Connell Memorial Herbarium of the University of New Brunswick (UNB).
Molecular identification of colonies
Extraction of DNA from silica-preserved subsamples followed the brown algal protocol of Saunders & McDevit (Citation2012), with a few steps modified to compensate for the low specimen volume: subsamples were extracted in 200 µl of brown algal buffer, the acetone step was omitted, and DNA was eluted in 100 µl of TE buffer (10 mM Tris, pH 8 and 1 mM EDTA). The PCR of rbcL-3P and LSU D2/D3 followed Hamsher et al. (Citation2011). The ITS2 region was amplified initially using DiamITSF (5′–AATGCGAATTGCAAGACCTC–3′) and KG4 (Lane et al., Citation2006). The ITS2 PCR profile was: 95°C initial denaturation for 3 min; 38 cycles of 94°C for 30 s, 60°C for 45 s, and 72°C for 2 min; and a final extension of 72°C for 10 min. While fragments sequenced using the DiamITSF primer were readable, the KG4 primer resulted in mostly unreadable sequences. Therefore, in order to obtain readable ITS2 sequences from additional colonies, new primers SEHF1 (5′–CGGTAGCATTCTTGTCTG–3′) and SEHR1 (5′–AATACKACAWRAGGCTCC–3′) were developed from an alignment of Berkeleya sequences only. The ITS2 PCR profile used with the ‘Berkeleya primers’ was identical to the profile above, except the annealing temperature was lowered to 55°C.
For each marker, PCR products were visualized, cleaned when multiple bands were present, and sequenced following Hamsher et al. (Citation2011). The resulting sequences were edited in Sequencher™ 4.8 (Gene Codes, Ann Arbor, Michigan, USA). Sequences were aligned for each marker separately either by eye for rbcL-3P and LSU D2/D3 using MacClade 4 (Version 4.06) for OSX (Maddison & Maddison, Citation2003) or using default multiple alignment parameters for ITS2 in CLUSTALX (Version 2.0.5, Larkin et al., Citation2007). The rbcL-3P final alignment included 142 colonies (780 bp) with no indels, but ten colonies had ambiguities in their sequences, most likely due to contamination. These colonies are included in the rbcL-3P tree and treated as members of their closest rbcL-3P genetic species group in later analysis, but were not included in the divergence value calculations. The LSU D2/D3 alignment included sequences from 106 colonies (~700 sites). For ITS2, sequences from closely related species (Berkeleya spp. 1–9) could be aligned easily, but alignment of sequences between more distant taxa was more difficult. Thus, sequences for Berkeleya sp. 10 were acquired, but not included in the analyses because they could not be aligned unambiguously. The ITS2 final alignment included 36 colonies (661 sites). Final alignments of LSU D2/D3 and ITS2 are included here as supplementary files (Files S1 and S2, respectively). Clustering was performed for each marker (rbcL-3P, LSU D2/D3, ITS2) separately, using the neighbour-joining algorithm on uncorrected dissimilarities (p) in Geneious v5.4 (Drummond et al., Citation2011). All sequences and their trace files have been uploaded to the Barcode of Life Data System (BOLD; www.boldsystems.org) and GenBank (see for specimen identifiers and accession numbers).
Colony heterogeneity testing
Owing to higher than expected contamination levels in the single marker sequences obtained from individual colonies (20% in this study compared with 0% reported in Hamsher et al., Citation2011) and conflicts between markers (see Results), we hypothesized that colonies were heterogeneous, i.e. contained more than one genetic species group. To assess this we chose colonies based on the rbcL-3P results for heterogeneity testing. The 91 colonies tested () included 12 representatives from the largest group (Berkeleya sp. 1), 48 representatives from Berkeleya spp. 2–11, four colonies that had rbcL-3P ambiguities (were treated as representatives of their closest related genetic species group; see Results), 27 colonies that had unreadable rbcL-3P sequences, and five negative PCR controls. The unreadable category included colonies for which the rbcL-3P sequences had multiple peaks (i.e. ambiguities), but which nonetheless had background sequences consistent with Berkeleya species. Each of these colonies was probed using ‘species-specific’ ITS2 primers () to determine whether the colony was heterogeneous; this involved nine PCR reactions per colony using distinct ‘species-specific’ primers. ITS2 was chosen because it was the most variable marker tested, making ‘species-specific’ primer design more feasible. Primer sequences, directions, annealing temperatures, and the compatible primers (for the opposite direction) are presented in . With the exception of the annealing temperature (), the PCR profile followed the ITS2 protocol for all primer pairs. All PCR products were visualized and sequenced following the protocol presented above for ITS2, except that sequencing was completed in only a single direction using the appropriate ‘species-specific’ primer. The resulting sequences were compared with the ITS2 sequences previously generated.
Table 1. ITS2 ‘species-specific’ primers used for Berkeleya rutilans. Primer sequences are presented 5′ to 3′.
To determine the extent of heterogeneity within a single colony, a second test was performed. Eight colonies were collected on 3 August 2011, from a site near Lepreau, New Brunswick, Canada (45.0722° N, 66.4690° W). Each colony was cut into ten pieces with a razor blade and each fragment placed in a separate vial with silica gel. Colony fragments were ground and extracted as described above [ten pieces from eight individuals = 80 colony fragments and 16 mock DNA extractions (control for cross-contamination)]. Initially, rbcL-3P was amplified and sequenced for each colony fragment (following Hamsher et al., Citation2011) to assign the colony fragment to a ‘genetic species group’ as in our initial tests. To test for heterogeneity within the colony fragments, four PCR reactions were conducted on each fragment with the ‘species-specific’ primers for Berkeleya spp. 1+11, 2, 6 and 9 (), using the protocol established for the first heterogeneity test. These particular ‘species-specific’ primers were selected to test for heterogeneity because they were either for one of the genetic species identified by the rbcL-3P analysis of the fragments (i.e. Berkeleya spp. 1+11, 2, and 6) or the most common cohabitant recognized in our initial survey (Berkeleya sp. 9). Twenty per cent of the amplicons were randomly sequenced in one direction as above to confirm that amplicons were consistent with the ‘species-specific’ primer combination used to generate it. Results were summarized for each colony fragment.
Results
Morphological species identification
Of the 182 colonies collected, a majority (~80%) were identified morphologically as Berkeleya rutilans. Some colonies of B. rutilans contained valves with a single morphotype ( and ), while other colonies contained individuals with differing valve morphologies (Figs 7–12 and 13–18). Other species forming tubes were Parlibellus delognei f. elliptica (17 colonies), P. berkeleyi (11), Haslea crucigera (1), Navicula brunelii (1), Navicula mollis (1) and Navicula ramosissima (1). In addition to the dominant tube-forming diatoms, Navicula bottnica, N. perminuta, Nitzschia fontifuga, N. tubicola and the green alga Chlorochytrium were identified as cohabitants (endophytes). Melosira and Licmophora species were found growing epiphytically on the tubes. Of the six colonies for which culturing was attempted, two (SEH004, SEH009) resulted in the growth of a tube-forming diatom (Parlibellus berkeleyi and Navicula ramosissima, respectively). Diatom cohabitants (Navicula bottnica, Nitzschia tubicola, N. fontifuga) or other diatom contaminants (Fragilaria sp.) dominated the cultures that were attempted from colonies of Berkeleya rutilans and P. delognei f. elliptica.
Figs 1–18. Cleaned valves of Berkeleya rutilans, LM. 1–3. Putative Berkeleya sp. 1 morphology from a colony (SEH156) for which we recorded only this genetic species group. 4–6. Putative Berkeleya sp. 9 morphology from a colony (GWS009393) for which we recorded only this genetic species group, including an initial valve (Fig. 4). 7–12. Putative Berkeleya sp. 1 (Figs 7–9) and Berkeleya sp. 9 (Figs 10–12) morphologies from a colony (GWS009756) recorded as containing both of the respective genetic species groups. 13–18. Representative valves from a single colony (GWS009341) containing Berkeleya sp. 6 (Fig. 13), sp. 8 (Figs 16–18), and sp. 9 (Figs 14, 15). Scale bar = 10 μm.
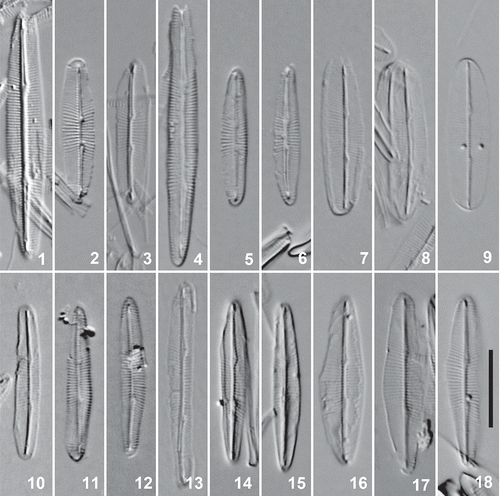
Molecular species identification
To assess the species diversity of marine tube-forming diatoms in Canada the rbcL-3P marker was amplified and sequenced from the colonies listed in , including six cultures. Within-species genetic divergence was defined to be less than 0.1%, based on Trobajo et al. (Citation2009). This threshold is slightly more conservative than that found by Hamsher et al. (Citation2011), who reported 0.1% divergence between the most closely related Sellaphora species. As a result, we may slightly underestimate the actual number of species present. Note that most of the genetic species recovered here are > 0.4% divergent.
Of the 182 colonies subjected to genetic identification using rbcL-3P, approximately 80% of the amplicons resulted in readable diatom sequences. The unreadable rbcL-3P sequences either contained a large number of ambiguities (indicating more than one closely related genetic species in a colony), or were entirely unreadable with multiple peaks at each site (indicating contamination from divergent diatom lineages). This level of ‘contamination’ (20%) is much higher than the 0% expected for this marker based on Hamsher et al. (Citation2011). The majority of the successful sequences (78%) were from Berkeleya rutilans colonies and formed a cluster of 14 genetic species groups, 11 of which were represented by more than one colony (). The B. rutilans genetic species groups differed by 0.2–9% (2–70 bp) when ambiguities were excluded from divergence estimates (, asterisks). Berkeleya spp. 5 and 6 were the closest related species groups, with only 0.2% divergence.
Fig. 19. Diversity of the marine tube-forming diatoms in Canadian waters. Genetic species groups displayed as a neighbour-joining tree inferred from the rbcL-3P data. An asterisk indicates sequences with ambiguities that were not included in divergence estimates as discussed in the text. Identifiers in bold are sequences from culture material.
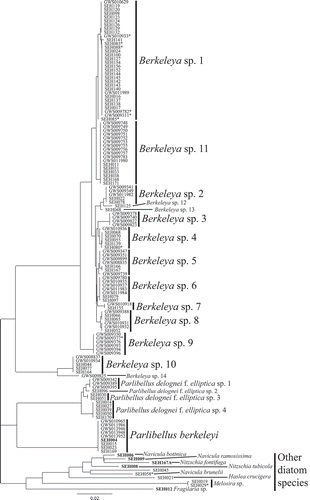
The rbcL-3P analysis also resolved four Parlibellus delognei f. elliptica genetic species (), with 0.4–1.1% (3–9 bp) divergence between them, and one P. berkeleyi genetic species group (0% divergence). Ten additional diatom sequences were positioned on long branches. Five of these ten sequences were from our cultures and represented an additional tube-forming diatom (Navicula ramosissima), common cohabitants (N. bottnica, Nitzschia fontifuga and N. tubicola), and a diatom ‘contaminant’ (Fragilaria). Of the remaining five sequences, two were additional tube-forming diatom species (Haslea crucigera, Navicula brunelii), two were epiphytes on P. delognei f. elliptica (a Melosira species), and one (SEH045) was identified morphologically as B. rutilans, but a contaminant must have been sequenced instead (SEH045; BLAST indicated it represents a Navicula species).
The LSU D2/D3 marker results were similar to those for rbcL-3P (). From the colonies collected, 70% of amplicons resulted in clean, readable diatom sequences. The clustering analysis (tree not shown) of these sequences confirmed one large group (71% of sequences) of closely related Berkeleya genetic species and two Parlibellus species clusters. The divergence between genetic species of the P. delognei f. elliptica complex (0.4–1.6% divergence), or individuals of P. berkeleya (0% divergence), and the cohabitant or contaminant diatoms were similar to rbcL-3P divergence values. However, the resolution of the LSU D2/D3 marker was much lower for the Berkeleya genetic groups, indicating only three genetic species with 0.08% divergence between them. One additional tube-forming diatom (Navicula mollis) was sequenced using LSU D2/D3 (the rbcL-3P sequence for this colony was messy). Two colonies (SEH155, SEH050) that clustered in known groups in the rbcL-3P analysis (Berkeleya sp. 7 and P. delognei f. elliptica, respectively) were on long branches in the LSU D2/D3 analysis; BLAST indicated they were contaminant species of Nitzschia and Navicula, respectively.
ITS2 was sequenced to investigate further the B. rutilans species complex. Of the colonies tested (N = 57), 65% of amplicons resulted in clean, readable diatom sequences. The remaining sequences were unreadable (N = 19) or represented contamination (one fungal sequence). Clean diatom sequences were generated from representatives of 11 of the rbcL-3P genetic species groups (), including three of the colonies with ambiguities ( and , asterisk). The three colonies with ambiguities in their rbcL-3P sequence fell within the Berkeleya sp. 1 genetic species group based on ITS2 data, which was consistent with their placement in the rbcL-3P analysis. The sequence from Berkeleya sp. 10 was not included in the ITS2 tree because it could not be unambiguously aligned with other Berkeleya spp. sequences. The remaining Berkeleya genetic species exhibited 0–0.6% (0–4 sites) within-species divergence and 1.1–11.2% (7–75 sites) between species. Two potential genetic species groups in the rbcL-3P analysis (Berkeleya sp. 1 and 11) were in a single cluster in the ITS2 analysis (, contrast ), indicating a single genetic species with high variation in both markers (0.7% for rbcL-3P and 0.4% for ITS2).
Fig. 20. Diversity of Berkeleya species in Canadian waters. Genetic species groups displayed as a neighbour-joining tree inferred from the ITS2 data. An asterisk indicates ITS2 sequences for colonies that exhibited ambiguities in their rbcL-3P sequences. Identifiers in bold are sequences that did not match their expected rbcL-3P genetic species group. Berkeleya sp. X indicates an ITS2 sequence that could not be linked to an rbcL-3P genetic species group. A representative of Berkeleya sp. 10 was sequenced, but was not included in the tree for reasons discussed in the text.
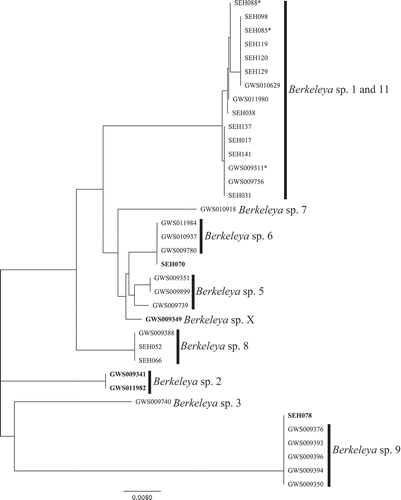
Of the ITS2 sequences acquired, 86% showed the same genetic species group assignment as rbcL-3P. The remaining 14% of colonies sequenced for ITS2 (N = 5) were in conflict with the rbcL-3P analysis. The first conflict involved Berkeleya sp. 2 (), whose colonies were distributed into three groups in the ITS2 analysis (, bold): one colony, SEH078, was identical to the ITS2 of Berkeleya sp. 9; GWS009341 and GWS011982 grouped together and are assumed here to be Berkeleya sp. 2 (); and GWS009349, which we have named Berkeleya sp. X (), did not match any other sequence. This unmatched Berkeleya genetic species group was not detected in the rbcL-3P analysis and was not pursued further. The second conflict involved Berkeleya sp. 4. The only ITS2 sequence obtained for colonies tested from this group (SEH070; , bold) was identical to sequences from Berkeleya sp. 6. Although introgression may explain some of these conflicting results between the rbcL-3P and ITS2 analyses, they also are consistent with the hypothesis that each Berkeleya colony could be composed of more than one Berkeleya genetic species (i.e. some colonies are heterogeneous).
Colony heterogeneity testing
To determine whether the Berkeleya colonies were heterogeneous, ‘species-specific’ ITS2 primers were designed and used to probe 91 colonies () and determine whether more than a single genetic species was present in each colony. In addition to the ITS2 sequences expected for the nine ‘specific’ primer combinations, eight new ITS2 types were uncovered (). These novel genetic groups were within the Berkeleya cluster indicating as many as 22 possible genetic species groups, but could not be related to the rbcL-3P groups and were not analysed further. Only 31 of the colonies tested (36%) had a single ITS2 type (). This is probably an overestimate, as we tested for the presence of only nine of the 22 possible genetic species groups and a negative PCR result is not necessarily equivalent to the absence of a genetic species. The remaining 60 colonies had more than one ITS2 type, with approximately 30% of colonies (N = 26) containing at least four ITS2 types (). Four of the ten rbcL-3P species groups tested had no colonies with a single ITS2 type (Berkeleya spp. 2, 3, 4 and 10; ). The only rbcL-3P genetic species that appeared to form colonies of a single genetic group was Berkeleya sp. 7 (), but this may be an artefact of sample size as only two colonies tested using ITS2 ‘species-specific’ primers had the rbcL-3P sequence of Berkeleya sp. 7. Interestingly, colonies that yielded unreadable rbcL sequences (suggesting the presence of more than one genetic species group of Berkeleya) also displayed the highest ITS2 diversity (), an observation consistent with our hypothesis of colony heterogeneity.
Fig. 21. Histogram of the number of ITS2 types per individual colony. The ITS2 types that could not be tied to rbcL-3P genetic species group were not included in the analysis, as discussed in the text.
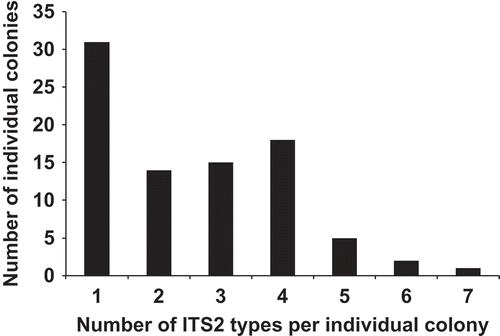
To determine the degree of heterogeneity within a single colony, eight colonies were cut into ten fragments (N = 80) and analysed using rbcL-3P data and the ITS2 ‘species-specific’ primers. RbcL-3P analysis of these colony fragments indicated that Berkeleya sp. 2, 4, 6 and 11 were present and that five of the eight colonies tested contained more than a single rbcL-3P genetic species (). To examine this heterogeneity further, ITS2 ‘species-specific’ primers for Berkeleya sp. 1+11, 2, 6 and 9 were used to probe the 80 colony fragments and 20% of the amplicons were sequenced. Of the amplicons sequenced, 74% were the target sequence, 19% were unreadable and 7% were ‘Unknown sp. 3’ (see ). Due to the high percentage (74%) of target sequence, all positive amplifications were treated as confirmation of a genetic group’s presence. A majority of the colony fragments (70%) contained all four genetic species groups that were tested and only 5% of the colony fragments contained a single ITS2 genetic group (), suggesting that the heterogeneity uncovered here is not limited only to a few cells in restricted areas of a colony, but rather is distributed widely throughout each colony. Again, the degree of heterogeneity found here is probably an underestimate, as the presence of only four of the 22 possible genetic species was tested for.
Table 2. Heterogeneity of Berkeleya colonies according to rbcL-3P genetic species diversity. Eight colonies were cut into 10 fragments and their (dominant) rbcL-3P determined by PCR and Sanger sequencing. Genetic species groups are as labelled in Fig. 1. ‘NAm’ indicates fragments that did not amplify and ‘unreadable’ indicates sequence that was messy.
Morphological evidence of colony heterogeneity
Because of the unexpected richness of genetic species groups and the genetic heterogeneity apparently present within colonies, we examined colonies in further detail to determine whether there was a corresponding morphological diversity within them. First, colonies presumably containing only (or at least dominated by) a single genetic species group were assessed for morphological uniformity and type. Two colonies, one of Berkeleya sp. 1 (SEH156: N = 20 valves; Figs 1–3) and one of Berkeleya sp. 9 (GWS009393: N = 20 valves; Figs 4–6), appeared to be morphologically homogeneous. In the Berkeleya sp. 1 colony, valves were elliptical to lanceolate in shape and measured 17–25 µm in length and 4–5 µm in width, with 26–28 striae in 10 µm (measured in the middle of the valve). The striae were parallel in the centre of the valve and radiate towards the apices. In the colony of Berkeleya sp. 9, valves were more linear and, while similar in length (17–34 µm) to those of the sp. 1 colony, were narrower (2–3 µm in width), with 22–28 striae in 10 µm. The striae were radiate in the centre of the valve (and coarser than in sp. 1) and parallel (and finer) towards the apices. In addition, the ratio of the distance between proximal raphe endings to the valve length was measured, due to its use in distinguishing B. obtusa from B. rutilans (Mizuno, Citation1979). The average ratio was slightly greater for Berkeleya sp. 1 (18%) than for Berkeleya sp. 9 (16%), but their ranges (14–26% and 10–19%, respectively) overlapped. We then studied a colony (GWS009756) for which our ITS2 results had indicated that both Berkeleya sp. 1 and Berkeleya sp. 9 were present and successfully identified cells with the two corresponding morphologies (e.g. Figs 7–9 and 10–12, respectively).
We also associated morphologies with three other genetic species, namely Berkeleya sp. 6 (Fig. 13), sp. 7 (not shown) and sp. 8 (Figs 16–18). The valves of these species differed with respect to shape, width, stria density and pattern, and the ratio of the distance between the central raphe endings to valve length (). A colony shown by molecular data to contain Berkeleya spp. 2, 6, 8 and 9 was then studied, as in the test described above involving Berkeleya spp. 1 and 9. As expected, the genetically heterogeneous colony was shown to contain cells morphologically consistent with the ‘pure’ species, including Berkeleya sp. 6 (Fig. 13), Berkeleya sp. 8 (Figs 16–18) and Berkeleya sp. 9 (Figs 14, 15). Thus in each case the valve morphologies found in each colony were consistent with the ITS2 ‘species-specific’ primer results.
Table 3. Morphological characteristics associated with five Berkeleya genetic species groups as inferred from individual colonies that were dominated by a single genetic type in our molecular analyses. Minimum–maximum (mode) values are presented for 20 valves. PRE/L = ratio of the distance between proximal raphe endings to the valve length.
Discussion
Identification of diatoms based on morphological features requires expensive light and scanning electron microscopy, a high degree of skill to differentiate species, and a vast expensive literature (Mann et al., Citation2010). Even with the acquired skill and the appropriate literature, different interpretations of descriptions are often encountered, and type material can be difficult to access or fail to provide the resolution necessary for identification using modern techniques (Mann et al., Citation2010). The use of molecular sequence data to resolve closely related species has increased our knowledge and appreciation of the diversity of diatoms and their biogeography.
The universality of the rbcL-3P marker allows for quick identification of genetic species and its discriminatory power highlights species that need further examination. Among our collections, the rbcL-3P marker uncovered unexpected diversity within P. delognei f. elliptica (four genetic species groups) and B. rutilans (14 genetic species groups). Whereas the presence of pseudocryptic or cryptic species is likely to be the explanation for our observations, there are other possible sources of the variation we uncovered in rbcL-3P and these must be addressed. For example, Amato et al. (Citation2007) and Levialdi Ghiron et al. (2008) highlighted cases of rbcL polymorphism in Pseudo-nitzschia. While this may be a problem with Pseudo-nitzschia, it has not been shown in studies of other taxa (e.g. Evans et al., Citation2008; Trobajo et al., Citation2010; Hamsher et al., Citation2011). Among our collections, Berkeleya sp. 1 and sp. 11 formed two rbcL-3P genetic species groups but a single ITS2 cluster. However, both markers showed a greater degree of variation in this group than in other species groups and will therefore need further examination, using culture material, to determine the cause of this pattern.
The LSU D2/D3 marker was able to differentiate Parlibellus genetic species but could not distinguish between most of the Berkeleya genetic groups. These results are similar to those of Hamsher et al. (Citation2011), with rbcL-3P and LSU D2/D3 showing equivalent divergence for more distantly related species, but less divergence of LSU D2/D3 between closely related genetic groups. The LSU D2/D3 data revealed that two colonies (SEH155, SEH050) contained at least two genetic species, with one of the genetic species in each sample being identified as a tube-forming diatom (rbcL-3P data) and the other a possible diatom cohabitant or a contaminant (LSU D2/D3 data). This pattern would not have been detected without the use of multiple markers for identification.
ITS2 was also used to investigate the Berkeleya rutilans species complex. This marker can be problematic due to intraclonal polymorphisms that can render sequences difficult to interpret (Behnke et al., Citation2004). However, we do not feel this accounts for the messy sequences generated here, or the heterogeneity uncovered within individual colonies. If these were due to intraclonal polymorphism within this multi-copy nuclear region, we would have expected to obtain fewer clean ITS2 sequences and uncover lower congruence between ITS2 and rbcL-3P genetic species groups. In fact, clean ITS2 sequences were recovered from ten of the 11 rbcL-3P genetic species groups tested and the ITS2 data were consistent with the rbcL-3P data in 86% of cases. In addition, if we accept ITS2 intraclonal polymorphisms as the sole explanation for unreadable sequences and the apparent heterogeneity of colonies, then we would also have to apply similar arguments to explain these same two patterns in the rbcL-3P data. Although slight intraclonal variation has been reported in rbcL in diatoms (Amato et al., Citation2007; Levialdi Ghiron et al., 2008), we are not aware of reports consistent with the levels of variation uncovered here. The conflicts in genetic species group assignments between the rbcL-3P and ITS2 groups led us to the hypothesis that individual colonies were composed of more than a single Berkeleya species and the ITS2 region was sufficiently variable to allow the design of ‘species-specific’ primers so that we could further test this hypothesis. However, while the evidence suggests multiple genetic species groups are present within a single colony, the existence of ITS polymorphism cannot be ruled out and should be assessed in more detail in future studies.
The results from testing our heterogeneity hypothesis indicated that 64% of colonies contain two or more Berkeleya genetic groups and that the heterogeneity can be found throughout the colony, rather than being restricted to a small or localized portion of the colony, as might be expected if one colony acted as a substratum for other tube-formers growing epiphytically. This generates a few problems for the taxonomy of tube-forming diatom species.
Traditionally, gross colony morphology, together with the shape of the cells contained within the tubes, was used to separate species of tube-forming diatoms in form genera such as Schizonema or Homoeocladia (Kützing, Citation1844) and the type specimens of these species are the colonies themselves, not the cells they contain. One of the problems in doing this is that colony morphology does not provide many features when compared with frustule morphology (which considers the individual cells rather than the colonies) and this has led to a complex taxonomic history of tube-forming diatom species (Lobban, Citation1984). For example, Berkeleya rutilans was originally described by Roth (Citation1806) as Conferva rutilans. Roth’s (1806) description focused on colony morphology and the valve structure is only a minor detail (ellipsoid cells). Later, C. rutilans was transferred through a number of genera (Bangia, Hydrolinum, Schizonema, Girodella, Monema, Amphipleura and Carrodoria). The transfers to Bangia, Hydrolinium, Schizonema, Girodella and Monema were probably based on colony morphology alone. Even the transfer to Berkeleya by Grunow (Citation1880) maintains an emphasis on gross morphology, since Grunow described ~30 varieties of Berkeleya rutilans based on colony characteristics including colour.
A second problem with using colonies for describing and typifying species of tube-forming diatoms can be illustrated via the type collection of Berkeleya rutilans, presumably a colony (so far type material has not been located). Only 36% of the colonies tested in this study had a single ITS2 type and this is probably an overestimate, as only nine of 22 possible genetic species were tested for their presence. Hence our data indicate that multiple Berkeleya genetic groups commonly live in a single colony and therefore, the type specimen of B. rutilans is most likely a heterogeneous colony. If the genetic groups we detected are found to be distinct species, taxonomic revision of B. rutilans will be necessary and, if the original type collection of B. rutilans consists of a heterogeneous colony, any taxonomic revisions will require extensive morphological analyses and the creation of epitypes to clarify which element of the holotype or lectotype is to be considered to represent ‘true’ B. rutilans. Our initial morphological identification of colonies for this study revealed a variety of valve morphologies for B. rutilans and these may be congruent with some of the genetic species groups (for example, the morphologies depicted in Figs 1–3 seem to correspond to Berkeleya sp. 1). Mizuno (1979, 1981) acknowledged that there is morphological variation within B. rutilans (Cleve, Citation1894) and recognized two species (B. obtusa and B. sparsa) to account for this diversity. While some of the variants observed here may be congruent with B. obtusa and B. sparsa, further molecular identification and subsequent taxonomic revisions will be needed, based on clonal cultures or single-cell analysis (Edvardsen et al., Citation2003) with species described following the protocol outlined by Evans & Mann (Citation2009).
A number of interesting questions regarding colony construction and ecology of B. rutilans are prompted by our study. Does a single cell initiate the formation of the macroscopic colonies as Lobban (Citation1989) hypothesized, or are they initially formed by several or many cells, which in light of our evidence may or may not be derived from a single genetic species? Diatoms are reported to migrate in and out of the tubes (Lobban, Citation1989), so are individual tubes composed of different genetic species groups depending on the season? Are younger tubes less heterogeneous than older tubes, and are tubes even predominantly composed of a single dominant genetic type? Sexual reproduction of B. rutilans has been documented (as Amphipleura rutilans: Tschermak-Woess, Citation1973), but do all genetic groups in this complex reproduce sexually or are some ‘species’ asexual lineages?
If the genetic groups described here do correspond to species, what is the mechanism of speciation, given that the species seem to occupy the same niche; are there pre- or post-zygotic boundaries between the genetic groups? Berkeleya rutilans was described from Germany. Are the genetic species within the type collection (assuming the collection is a colony) similar to the ones studied here or does this collection include different species and where are these species found? Are the genetic species groups described in this study geographically restricted to North America or are they also found in other places where B. rutilans has been reported, which include the Arctic (Lobban, Citation1984), the Antarctic (Scott & Thomas, Citation2005), Chile (Rivera, Citation1976), Europe (Ros et al., Citation2009) and Japan (Mizuno, Citation1977)?
All of these questions can now be investigated because genetic species groups can be reliably identified using molecular tools. Without these tools, researchers have mistakenly assumed that B. rutilans was a single genetic species and underestimated the diversity of these unusual diatoms, at global to individual colony scales, although detailed morphological analyses could perhaps have detected some of the diversity uncovered here. However, some species within the B. rutilans complex are probably truly cryptic, highlighting the importance of combining morphological and molecular tools to reveal the full extent of species diversity in the genus.
Acknowledgements
We would like to thank members of the Saunders lab for assistance in collecting colonies and advice on this manuscript, and we are grateful to two anonymous reviewers for their valuable comments. This research was supported through funding to the Canadian Barcode of Life Network from Genome Canada (through the Ontario Genomics Institute), National Sciences and Engineering Research Council of Canada (NSERC), and other sponsors listed at www.BOLNET.ca. Additional funding was provided by NSERC and the Canada Research Chair Program to G.W. Saunders, as well as infrastructure support from the Canada Foundation for Innovation and the New Brunswick Foundation for Innovation.
Supplementary information
The following supplementary material is available for this article, accessible via the Supplementary Content tab on the article’s online page at http://dx.doi.org/10.1080/09670262.2014.885582
Supplementary . Samples used in this study.
Supplementary . Heterogeneity of individual Berkeleya colonies as determined by ITS2 ‘species-specific’ primers.
Supplementary File S1. LSU D2/D3 rDNA alignment (fasta file).
Supplementary File S2. ITS2 rDNA alignment (fasta file).
Table S1. Samples used in this study.
Table S2. Heterogeneity of individual Berkeleya colonies as determined by ITS2 ‘species-specific’ primers. Columns list the individual colonies tested (see for details), their rbcL-3P ‘species’ assignment (‘Unreadable’ indicates colonies that had unreadable rbcL-3P sequences and thus could not be assigned to one of the rbcL-3P genetic groups) and the ITS2 ‘species-specific’ primer combinations trialed (rbcL-3P genetic groups Berkeleya spp. 1+11 were combined as they formed a single ITS2 genetic species group). ‘Unknown’ 1–8 are ITS2 sequences that were not uncovered in our initial colony survey. The symbols + and – indicate whether target sequence was acquired for that colony and ITS2 primer combination. Values in bold red indicate expected matches, i.e. where the rbcL-3P ‘species’ matches the ITS2 ‘species-specific’ primer.
Supplementary material
Download Zip (167.6 KB)References
- Aleem, A.A. (1949). Distribution and ecology of marine littoral diatoms. Consideration of the littoral diatom-flora with special reference to forms living in gelatinous tubes. Botaniska Notiser, 1949: 414–440.
- Aleem, A.A. (1950). Distribution and ecology of British marine littoral diatoms. Journal of Ecology, 38: 75–106.
- Amato, A., Kooistra, W.H.C.F., Levialdi Ghiron, J.H., Mann, D.G., Pröschold, T. & Montresor, M. (2007). Reproductive isolation among sympatric cryptic species in marine diatoms. Protist, 158: 193–207.
- Armbrust, E.V., Berges, J.A., Bowler, C., Green, B.R., Martinez, D., Putnam, N.H., Zhou, S., Allen, A.E., Apt, K.E., Bechner, M., Brzezinski, M.A., Chaal, B.K., Chiovitti, A., Davis, A.K., Demarest, M.S., Detter, J.C., Glavina, T., Goodstein, D., Hadi, M.Z., Hellsten, U., Hildebrand, M., Jenkins, B.D., Jurka, J., Kapitonov, V.V., Kröger, N., Lau, W.W.Y., Lane, T.W., Larimer, F.W., Lippmeier, J.C., Lucas, S., Medina, M., Montsant, A., Obornik, M., Parker, M. S., Palenik, B., Pazour, G.J., Richardson, P.M., Rynearson, T.A., Valentin, K., Vardi, A., Wilkerson, F.P. & Rokhsar, D.S. (2004). The genome of the diatom Thalassiosira pseudonana: ecology, evolution, and metabolism. Science, 306: 79–86.
- Behnke, A., Friedl, T., Chepurnov, V.A. & Mann, D.G. (2004). Reproductive compatibility and rDNA sequence analyses in the Sellaphora pupula species complex (Bacillariophyta). Journal of Phycology, 40: 193–208.
- Beszteri, E., Ács, É., & Medlin, L.K. (2005). Ribosomal DNA sequence variation among sympatric strains of Cyclotella meneghiniana complex (Bacillariophyceae) reveals cryptic diversity. Protist, 156: 317–333.
- Cianciola, E.N., Popolizio, T.R., Schneider, C.W. & Lane, C.E. (2010). Using molecular-assisted alpha taxonomy to better understand red algal biodiversity in Bermuda. Diversity, 2: 946–958.
- Cleve, P.T. (1894). Synopsis of naviculoid diatoms. Part I. Kongliga Svenska VetenskapsAkademiens Handlingar, series 4, 26: 1–194.
- Cox, E.J. (1977a). The distribution of tube-dwelling diatom species in the Severn Estuary. Journal of the Marine Biological Association of the United Kingdom, 57: 19–27.
- Cox, E.J. (1977b). The tube-dwelling diatom flora at two sites in the Severn Estuary. Botanica Marina, 20: 111–119.
- Cox, E.J. (1981). Mucilage tube morphology of three tube-dwelling diatoms and its diagnostic value. Journal of Phycology, 17: 72–80.
- Drummond, A.J., Ashton, B., Buxton, S., Cheung, M., Cooper, A., Duran, C., Field, M., Heled, J., Kearse, M., Markowitz, S., Moir, R., Stones-Havas, S., Sturrock, S., Thierer, T. & Willson, A. (2011). Geneious v5.4. Available from http://www.geneious.com.
- Edvardsen, B., Shalchian-Tabrizi, K., Jakobsen, K.S., Medlin, L.K., Dahl, E., Brubak, S. & Paasche, E. (2003). Genetic variability and molecular phylogeny of Dinophysis species (Dinophyceae) from Norwegian waters inferred from single cell analyses of rDNA. Journal of Phycology, 39: 395–408.
- Evans, K.M. & Mann, D.G. (2009). A proposed protocol for nomenclaturally effective DNA barcoding of microalgae. Phycologia, 48: 70–74.
- Evans, K.M., Wortley, A.H. & Mann, D.G. (2007). An assessment of potential diatom “barcode” genes (cox1, rbcL, 18S and ITS rDNA) and their effectiveness in determining relationships in Sellaphora (Bacillariophyta). Protist, 158: 349–364.
- Evans, K.M., Wortley, A.H., Simpson, G.E., Chepurnov, V.A. & Mann, D.G. (2008). A molecular systematic approach to explore diversity within the Sellaphora pupula species complex (Bacillariophyta). Journal of Phycology, 44: 215–231.
- Godhe, A., Asplund, M.E., Harnstrom, K., Saravanan, V., Tyagi, A. & Karunasagar, I. (2008). Quantification of diatom and dinoflagellate biomasses in coastal marine seawater samples by real-time PCR. Applied and Environmental Microbiology, 74: 7174–7182.
- Grunow, A. (1880). Vorläufige Bemerkungen zu einer systematischen Anordung der Schizonema- und Berkeleya-Arten, mit Bezug auf die in Van Heurck’s Diatomeenflora von Belgien veröffentlichten Abbildungen der Frusteln auf Taf. XV, XVI und XVII. Part II. Botanisches Centralblatt, 4: 1585–1598.
- Guillard, R.R.L. & Ryther, J.H. (1962). Studies of marine planktonic diatoms. I. Cyclotella nana Hustedt and Detonula confervacea Cleve. Canadian Journal of Microbiology, 8: 229–239.
- Hamsher, S.E., Evans, K.M., Mann, D.G., Poulíčková, A. & Saunders, G.W. (2011). Barcoding diatoms: exploring alternatives to COI-5P. Protist, 162: 405–422.
- Kützing. F.T. (1844). Die kieselschaligen Bacillarien oder Diatomeen. W. Köhne, Nordhausen.
- Lane, C.E., Mayes, C., Druehl, L.D. & Saunders, G.W. (2006). A multigene molecular investigation of the kelp (Laminariales, Phaeophyceae) supports substantial taxonomic re-organization. Journal of Phycology, 42: 493–512.
- Larkin, M.A., Blackshields, G., Brown, N.P., Chenna, R., McGettigan, P.A., McWilliam, H., Valentin, F., Wallace, I.M., Wilm, A., Lopez, R., Thompson, J.D., Gibson, T.J. & Higgins, D.G. (2007). Clustal W and Clustal X version 2.0. Bioinformatics, 23: 2947–2948.
- Le Gall, L. & Saunders, G.W. (2010). DNA barcoding is a powerful tool to uncover algal diversity: a case study of the Phyllophoraceae (Gigartinales, Rhodophyta) in the Canadian flora. Journal of Phycology 46: 374–389.
- Levialdi Ghiron, J.H., Amato, A., Montresor, M. & Kooistra, W.H.C.F. (2008). Plastid inheritance in the planktonic raphid pennate diatom Pseudo-nitzschia delicatissima (Bacillariophyceae). Protist, 159: 91–98.
- Lobban, C.S. (1984). Marine tube-dwelling diatoms of eastern Canada: descriptions, checklist, and illustrated key. Canadian Journal of Botany, 62: 778–794.
- Lobban, C.S. (1985). Marine tube-dwelling diatoms of the Pacific coast of North America. I. Berkeleya, Haslea, Nitzschia, and Navicula sect. Microstigmaticae. Canadian Journal of Botany, 63: 1779–1784.
- Lobban, C.S. (1989). Environmental factors, plant responses, and colony growth in relation to tube-dwelling diatom blooms in the Bay of Fundy, Canada with a review of the biology of tube-dwelling diatoms. Diatom Research, 4: 89–109.
- Lobban, C.S. (1990). Marine tube-dwelling diatoms of the Pacific coast of North America. II. Navicula subg. Navicula and a key to the tube-dwelling diatoms of the region. Canadian Journal of Botany, 68: 707–712.
- Lobban, C.S. & Navarro, J.N. (2013). Gato hyalinus, gen. et sp. nov., an unusual araphid tube-dwelling diatom from Western Pacific and Caribbean islands. Phytotaxon, 127: 22–31.
- Maddison, W.P. & Maddison, D.R. (2003). MacClade, version 4.06. Sinauer, Sunderland, MA.
- Mann, D.G. & Droop, S.J.M. (1996). Biodiversity, biogeography and conservation of diatoms. Hydrobiologia, 336: 19–32.
- Mann, D.G., McDonald, S.H., Bayer, M.M, Droop, S.J.M., Chepurnov, V.A., Loke, R.E., Ciobanu, A. & Du Buf, J.M.H. (2004). The Sellaphora pupula species complex (Bacillariophyceae): morphometric analysis, ultrastructure, and mating data provide evidence for five new species. Phycologia, 43: 459–482.
- Mann, D.G., Sato, S., Trobajo, R., Vanormelingen, P. & Souffreau, C. (2010). DNA barcoding for species identification and discovery in diatoms. Cryptogamie Algologie, 31: 557–577.
- Mizuno, M. (1977). On the tube-dwelling diatom Berkeleya rutilans (Trentepohl) Grun. Bulletin of the Japanese Society of Phycology, 25: 143–149.
- Mizuno, M. (1979). Taxonomic study on Berkeleya obtusa (Grev.) Grunow (Bacillariophyceae) from Hokkaido, Japan. Japanese Journal of Phycology (Sôrui) 27: 175–181.
- Mizuno, M. (1981). Berkeleya sparsa sp. nov., a tube-dwelling diatom from Hokkaido, Japan. Japanese Journal of Phycology (Sôrui) 29: 95–99.
- Moniz, M.B.J. & Kaczmarska, I. (2009). Barcoding diatoms: is there a good marker? Molecular Ecology Resources 9: 65–74.
- Moniz, M.B.J. & Kaczmarska, I. (2010). Barcoding of diatoms: nuclear encoded ITS revisited. Protist, 161: 7–34.
- Poulíčková, A., Veselá, J., Neustupa, J. & Škaloud, P. (2010). Pseudocryptic diversity versus cosmopolitanism in diatoms: a case study on Navicula cryptocephala Kütz. (Bacillariophyceae) and morphologically similar taxa. Protist, 161: 353–369.
- Ravin, N.V., Galachyants, Y.P., Merdanov, A.V., Beletsky, A.V., Petrova, D.P., Sherbakova, T.A., Zakharova, Y.R., Likhoshway, Y.V., Skryabin, K.G. & Grachev, M.A. (2010). Complete sequence of the mitochondrial genome of a diatom alga Synedra acus and comparative analysis of diatom mitochondrial genomes. Current Genetics, 56: 215–223.
- Rivera, P. (1976). Diatomeas de la Bahía de Concepción, Chile. III. Amphipleura rutilans (Trentepohl) Cleve, una diatomea muy poco conocida para la costa chilena. Boletin de Sociedad Biologia de Concepcion, 49: 125–130.
- Ros, M.D., Marín-Murcia, J.P. & Aboal, M. (2009). Biodiversity of diatom assemblages in a Mediterranean semiarid stream: implications for conservation. Marine and Freshwater Research, 60: 14–24.
- Roth, A.G. (1806). Catalecta botanica quibus plantae novae et minus cognitae describuntur atque illustrantur. Fasciculus tertius cum tabulis aenaeis XII. pp. [i–viii], [1]–350, [1–2, index pi.], [1-6, index] [1, err.], pls I–XII. Bibliopolio Io. Fr. Gleditschiano, Lipsiae [Leipzig].
- Round, F.E., Crawford, R.M. & Mann, D.G. (1990). The diatoms. Biology and morphology of the genera. Cambridge University Press, Cambridge.
- Saunders, G.W. (2008). A DNA barcode examination of the red algal family Dumontiaceae in Canadian waters reveals substantial cryptic species diversity. 1. The foliose Dilsea–Neodilsea complex and Weeksia. Botany, 86: 773–789.
- Saunders, G.W. & McDevit, D. (2012). Methods for DNA barcoding photosynthetic protists emphasizing the macroalgae and diatoms. Methods in Molecular Biology, 858: 207–222.
- Scott, F.J. & Thomas, D.P. (2005). Diatoms. In Antarctic marine protists (Scott, F.J. & Marchant, H.J., editors), 13–201. CSIRO Publishing, Canberra, Australia.
- Sherwood, A.R. & Presting, G.G. (2007). Universal primers amplify a 23S rDNA plastid marker in eukaryotic algae and Cyanobacteria. Journal of Phycology, 43: 605–608.
- Sherwood, A.R., Chan, Y.L. & Presting, G.G. (2008). Application of universally amplifying plastid primers to environmental sampling of a stream periphyton community. Molecular Ecology Resources, 8: 1011–1014.
- Thornhill, D.J., LaJeunesse, T.C. & Santos, S.R. (2007). Measuring rDNA diversity in eukaryotic microbial systems: how intragenomic variation, pseudogenes, and PCR artifacts confound biodiversity estimates. Molecular Ecology, 16: 5326–5340.
- Trobajo, R., Clavero, E., Chepurnov, V.A., Sabbe, K., Mann, D.G., Ishihara, S. & Cox, E.J. (2009). Morphological, genetic, and mating diversity within the widespread bioindicator Nitzschia palea (Bacillariophyta). Phycologia, 48: 443–459.
- Trobajo, R., Clavero, E., Evans, K.M., Vanormelingen, P., McGregor, R.C. & Mann, D.G. (2010). The use of partial cox1 rbcL and LSU rDNA sequences for phylogenetics and species identification within the Nitzschia palea complex (Bacillariophyceae). European Journal of Phycology, 45: 413–425.
- Tschermak-Woess, E. (1973). Die geschlechtliche Fortpflanzung von Amphipleura rutilans und das verschiedene Verhalten der Erstlingszellen von Diatomeen in Gallertschläuchen. Österreichische botanische Zeitschrift, 122: 21–34.
- Vanormelingen, P., Verleyen, E. & Vyverman, W. (2008). The diversity and distribution of diatoms: from cosmopolitanism to narrow endemism. Biodiversity and Conservation, 17: 393–405.
- Zimmermann, J., Jahn, R. & Gemeinholzer, B. (2011). Barcoding diatoms: evaluation of the V4 subregion on the 18S rRNA gene, including new primers and protocols. Organisms Diversity and Evolution, 11: 173–192.