Abstract
Benthic diatoms inhabiting intertidal flats face highly variable environmental conditions, due to changing water levels and exposure during low tide. The present study is the second part of a more extensive study of the adaptive potential of these species in response to varying UV radiations in the Solthörn tidal flat (Lower Saxony, southern North Sea). Five isolates (Achnanthes exigua, Amphora exigua, Cocconeis peltoides, Diploneis littoralis and Navicula digitoradiata), which were found in this area in high cell numbers in summer 2008, were used in semi-continuous cultures to study the physiological effects of UV-radiation (PAR [photosynthetically active radiation], PAR+UV-A, PAR+UV-B, PAR+UV-B+UV-A). For short- and long-term exposures (6 h, 30 days), the composition of intercellular carbohydrates, amino and fatty acids were analysed in exponential-phase cultures grown at a salinity of 30 in a 12 : 12 h light : dark cycle at 20 °C. Although all tested species showed distinct differences in their initial carbohydrate, amino and fatty acid compositions and in their responses to the different UV treatments, general response patterns could be identified. Overall physiological responses to short- and long-term UV treatments included the accumulation of proline as well as an increase in total carbohydrates and lipids, whereas significant differences in the composition of carbohydrates, amino and fatty acids occurred after long-term exposure to the UV treatments (P < 0.05). While UV-A exposure led to higher accumulations of phenylalanine, aspartic acid and saturated fatty acids, the response to UV-B long-term exposure included increases of galactose, mannose and unsaturated fatty acids in the cells. In both UV experiments there was a noteworthy accumulation of the amino acid tryptophan in most species. The combined UV-A+UV-B experiment showed a significant (P < 0.05) increase of aspartic acid, phenylalanine, galactose and saturated fatty acids in a majority of species. Overall, the results indicated significant differences in the physiological responses of the five diatom taxa during UV exposure, which suggests species-specific acclimation strategies that may explain the growth insensitivity towards at least short-term UV.
Introduction
There has been much effort to measure the effects of ultraviolet radiation (UV) on aquatic life (Arts et al., Citation2000), and in particular on phytoplankton (e.g. Häder et al., Citation1995), primarily because of the enhanced UV-B radiation (290–320 nm) resulting from stratospheric ozone depletion. UV-A radiation (320–400 nm), although not affected by ozone depletion, will nevertheless have strong effects on the physiological and ecological responses of organisms living near the surface in aquatic environments (Arts et al., Citation2000). UV radiation can damage DNA and proteins, oxidize membrane lipids and chlorophyll, and cause damage to photosystem II (Vincent & Roy, Citation1993; Hessen et al., Citation1997; Sundbäck et al., Citation1997; Odmark et al., Citation1998; Skerratt et al., Citation1998).
Intertidal mudflats represent a highly unstable environment, due to continuously changing water levels and currents as well as temporary exposure to the air (Reineck, Citation1983). These habitats often harbour extensive communities of benthic diatoms, which are responsible for a major part of the primary production (Underwood & Kromkamp, Citation1999). Such communities are mainly composed of multi-layered biofilms of epipelic species, which may perform vertical migrations, limiting the UV exposure time of the uppermost cells and offering photoprotection by self-shading (Underwood & Kromkamp, Citation1999). The depth to which these communities extend is limited, however, by the extent to which light can penetrate into intertidal sediments (2–4 mm: Jørgensen et al., Citation1983; Kühl et al., Citation1996; Garcia-Pichel et al., Citation1999). Diatom motility involves the production of polysaccharides (exopolymers or ‘extracellular polymeric substances’ [EPS]) including rhamnose, fucose, xylose, mannose, galactose or glucose. Thus, in cohesive sediments dominated by epipelic diatoms, sediment colloidal carbohydrate and EPS concentrations are closely related to algal biomass (Underwood & Smith, Citation1998; Underwood et al., Citation2004).
Besides migration, one mechanism to cope with the effects of solar UV radiation is to block potentially harmful wavelengths with UV-absorbing compounds. For example, mycosporine-like amino acids (MAAs) show absorption maxima from 310 to 360 nm and have been identified in a wide variety of marine organisms, including dinoflagellates (Carreto et al., Citation1990) and macroalgae (Sivalingam et al., Citation1974; Wood, Citation1989). However, pennate diatoms – and most benthic diatoms are pennate – have been shown to contain very low concentrations of MAAs (Helbling et al., Citation1996). Thus, since benthic diatoms can be very tolerant to UV-B radiation (Peletier, Citation1996), they must apparently rely on mechanisms other than UV-absorbing compounds to avoid UV damage.
In intertidal microphytobenthic diatoms, production of compatible solutes such as proline, carbohydrates, polyols, glycine betaine and β-dimethylsulphoniopropionate (DMSP) has been reported as a physiological response to high salinity (Scholz & Liebezeit, Citation2012a) and temperature fluctuation (Scholz & Liebezeit, Citation2013). It is not yet fully understood how these compounds are involved in stress tolerance, but it is assumed that most of them stabilize macromolecules against various forms of stress, protecting complex proteins and membranes and the transcriptional and translational machinery (e.g. Chen & Murata, Citation2002). Some organic osmolytes, such as the amino acid proline (Hare & Cress, Citation1997), also have been shown to scavenge reactive oxygen species (ROS). In eukaryote cells, proline is synthesized from glutamate via ∆1-pyrroline-5-carboxylate (P5C) in two successive reductions catalysed by ∆1-pyrroline-5-carboxylate synthase (P5CS) and ∆1-pyrroline-5-carboxylate reductase (P5CR), while proline synthesis via ornithine as a precursor is mediated by ornithine δ-aminotransferase (δ-OAT) (Krell et al., Citation2007). Thus assays of these enzymes in cells subjected to UV stress can provide insights into the possible significance of proline in limiting UV damage.
During a one-year investigation of the microphytobenthic community in the Solthörn tidal flat (southern North Sea) we observed significant differences in the composition of the benthic diatom community during the annual cycle (Scholz & Liebezeit, Citation2012b, c). Physiological acclimatization to fluctuating temperatures and salinities was found in several diatom taxa, indicating the ability of single taxa to tolerate such changes by altering their biochemical composition (Scholz & Liebezeit, Citation2012a, d, Citation2013). The present study is the second part of a study of five intertidal microphytobenthic diatoms, in which they were subjected to photosynthetically active radiation (PAR) supplemented with different UV combinations (UV-A, UV-B and UV-A+UV-B) during short- (6 h) and long-term experiments (30 days, with a daily dose of 4 h). The taxa were isolated from the Solthörn tidal flat in summer 2008, when relatively high levels of UV were recorded on the sediment surface (up to 118 Wm−2 in June 2008; Scholz & Liebezeit Citation2012c). Whereas the first part of our study (Scholz et al., Citation2014) dealt with the influence of UV on growth and antioxidative defence strategies, the main objectives of the present study were to determine (1) variations in carbohydrate and lipid content, which might give evidence on the overall physiological status of the cells; (2) carbohydrate composition, particularly with regard to EPS production and the possible role of particular carbohydrates as compatible solutes; (3) differences in fatty acid composition including likely changes in membrane composition; and (4) amino acid composition, with special emphasis on proline, including the activities of enzymes involved in proline metabolism.
Materials and methods
Organisms
The diatoms Achnanthes exigua, Cocconeis peltoides, Diploneis littoralis, Navicula digitoradiata and Amphora exigua were isolated from the Solthörn tidal flat. The taxa were isolated according to the method described in Scholz & Liebezeit (Citation2012a). Details about sampling stations and dates as well as purification of species are given in Scholz et al. (Citation2014).
Cultivation of monocultures prior to experiments
Axenic cultures of individual species were grown in 2-l Erlenmeyer flasks (approximately 500 ml culture volume) using modified f/2 medium (Guillard, Citation1975; pH 8.04, salinity = 30). To reflect Solthörn tidal flat conditions (Scholz & Liebezeit, Citation2012b, c), Guillard’s formulation was modified by (1) changing the N : P ratio to 17 : 1, and (2) adding NaHCO3 after autoclaving to a final concentration of 2 mM. The photon flux rate was 600 µmol photons m−2 s−1 (Philips, Germany, Master TL-D 18W/840) with a light : dark regime of 16 : 8 h; cultures were maintained at 20 ± 0.5 °C. Glass beads (Sartorius, Ø 300–700 µm) were placed on the bottom of culture vessels in a layer approximately 3 mm thick and used as a substratum. Salinity, pH and conductivity were measured using handheld probes (YK-31SA, YK-2001PH SI Model 33, Engineered Systems and Designs-Model 600, Philips W9424; Philips, Amsterdam, the Netherlands). Cultures were supplied with new medium every 7 days to maintain optimal growth. After biofilm development (2 weeks after inoculation) the culture broth was removed with a sterile sleeve until only a thin liquid film remained over the biofilm. Subsequently, the experiments were started by addition of fresh culture medium.
Experimental design
Axenic isolates were cultivated in 2-l semi-continuous cultures under sterile conditions as described above, using sterilized, UV-transparent quartz bottles. Experiments were conducted in a dark chamber, without incoming light. Photosynthetic active radiation (PAR, 400–700 nm) and UV-A were provided for 14 h daily, supplemented with 4 h of UV-B. Care was taken to obtain realistic PAR+UV-A+UV-B ratios and relatively high values of PAR, such as found at the sediment surface in the Solthörn tidal flat during summer 2008 (Scholz & Liebezeit, Citation2012c). UV-A radiation was supplied from four Philips TLK09N 40 W fluorescent tubes (maximum emission at 354 nm) and UV-B from two Spectroline EB160C fluorescent tubes (Laboratory Products Sales, Rochester, New York, USA; maximum emission at 312 nm) pre-burned for c. 110 h to stabilize the lamp output. The lamps were suspended 60 cm above the samples and turned on for several hours prior to the experiment, again to stabilize output. Lamp radiation < 290 nm was eliminated by covering the UV-B tubes with 0.08 mm cellulose acetate sheet, replaced daily. PAR, UV-A and UV-B irradiances were adjusted with neutral density screens positioned in front of the fluorescent tubes. Irradiances were determined with a Biospherical QSL100 quantum scalar irradiance meter (Biospherical Instruments, San Diego, USA) for PAR, and with an International Light IL1700 radiometer (International Light Technologies, Massachusetts, USA) for UV-A and UV-B (photodetectors SUD033 and SUD240), resulting in 348 Wm−2 PAR, 41 Wm−2 UV-A, and 0.49 Wm−2 UV-B, corresponding to average UV values in the environment, as measured in 2008 with a MACAM SR9910 double monochromator scanning spectroradiometer (Macam Photometrics, Livingston, UK) with a cosine corrected sensor in air. PAR was 1510 µmol photons m−2 s−1 when measured with a LICOR LI-250 light meter and a flat sensor (LI-COR Biosciences, Bad Homburg, Germany). Due to lateral heterogeneities in the light field, PAR0 (photosynthetic active radiation at the point 0) was measured inside culture flasks containing medium but no algae. The first test series was the short-term experiment, which was run for 6 h. The second test series, the long-term experiment, was started concurrently and ran for 30 days. Six replicate flasks per treatment and species were deployed for the experiment. Taxa were grown in biofilms on a quartz-glass bead layer; in order to simulate environmental conditions. Once per day 60 ml of culture suspension was replaced with fresh medium (giving a dilution rate of 0.6 d−1), to avoid nutrient depletion in the cultures. The position of replicate flasks was changed randomly every other day to eliminate any location effects due to minor changes in external conditions.
Cell counts, harvesting and sample treatment
Culture checks and counts were performed microscopically, using a Neubauer Improved counting chamber with 0.1 mm depth (LO Laboroptik, Germany). Only cells that exhibited red fluorescence under UV radiation were counted. At least 1000 cells were counted in each sample at 400× magnification.
Generally, biofilms were allowed to grow without physical disturbance on the glass beads. Diatoms were easily removed for sampling by shaking on a rotating, shaking device (25 × g), dislodging the biofilm from the substrate and homogenizing the culture. Microscopical observation of the beads after shaking confirmed complete release of the cells. Biomass from the short-term (6 h) and the long-term experiments (30 days) were harvested by centrifugation at 680 × g for 10 min. The three replicates with the diatom biomass were each subdivided into seven aliquots and used for different extraction procedures as described below.
Biochemical analysis
Total carbohydrate and lipid contents
Total soluble carbohydrates were determined using the phenol sulphuric acid method of Kochert (Citation1978) and Ben-Amotz et al. (Citation1985), incorporating the modifications of Mercz (Citation1994) and Buttery (Citation2000). The samples were homogenized in 1 M H2SO4 (if not otherwise mentioned, all of the chemicals used in this study were of the highest purity from Sigma–Aldrich) and, after heating at 100 °C for 60 min, 0.3 ml of the supernatant was transferred into a fresh vial and made up to 2 ml with deionized water. Sets of glucose standards were prepared and 1 ml of 5% (w/v) phenol solution was added to all samples, after which 5 ml of concentrated H2SO4 was added. Absorbance was read at 485 nm.
The total lipid determination was based on the method of Bligh & Dyer (Citation1959), as modified by Kates & Volcani (Citation1966) and adapted by Mercz (Citation1994) and Buttery (Citation2000), using methanol : chloroform : deionized water (2 : 1 : 0.8, v/v/v). The extracts were dried under a stream of ultra-pure N2 gas and placed in a vacuum desiccator over silica gel overnight and then weighed.
Carbohydrates
The extraction of carbohydrates followed the method of Karsten et al. (Citation1991), using 70% aqueous ethanol. Mono- and disaccharides accumulated during the experiments were identified by thin layer chromatography (TLC Silica Gel 60 F254; Merck, Whitehouse Station, New Jersey, USA) according to the method described by Zhang et al. (Citation2009). Arabinose, galactose, glucose, fructose, fucose, mannose, ribose, rhamnose, sorbose, sucrose and xylose standards were used.
Free fatty acids
The analysis of lipids was conducted with the method described by Wöstmann & Liebezeit (Citation2008). Extracts from freeze-dried samples were prepared by ultrasonic extraction, using a solvent system of sequentially increasing polarity of (1) one treatment with n-hexane, (2) one treatment with n-hexane/dichlormethane (50 : 50 v/v), (3–5) three treatments with dichlormethane/methanol (90 : 10 v/v) corresponding to the polarity of hydrocarbons, alcohols and polar compounds, respectively. After a separation of fractions by column chromatography, polar compounds were analysed using an Agilent 5973 GCMS System (Agilent Technologies Sales & Services, Waldbronn, Germany) operating at 70 eV with a mass range of m/z 50–650 in the scan modus. The carrier gas was helium. Before measurement the polar compounds were derivatized to trimethylsilyl ethers by adding 50 µl of N-methyl-N-(trimethylsilyl)-trifluoracetamide (MSTFA) to each sample. Components were identified by comparison of their mass spectra and retention times with synthetic standards (e.g. Fatty Acid Kit EC10A-1KT Supelco, Fatty acid standards 1891 and 1896 Supelco). Quantification was accomplished by addition of defined quantities of erucic acid before derivatization.
Free amino acids
Proline concentration was determined and quantified spectrophotometrically with ninhydrin, according to the method described by Bates et al. (Citation1973) and modified by Nothnagel (Citation1995). For free amino acid analysis by HPLC, cold perchloric acid extraction was applied according to the method of Bligny et al. (Citation1989) as modified by Plettner (Citation2002). The supernatant from the extraction was removed, nitrogen fixed and lyophilized (Alpha 1-4 LSC lyophilization system, Christ, Germany). The samples were stored at −80 °C until analysis. HPLC analysis involved precolumn derivatization with o-phthaldialdehyde (OPA) and 2-mercaptoethanol, according to the method of Liebezeit & Behrends (Citation1999), using a 250 mm × 4.0 mm ID column Nucleosil 100 C18 with 5 μm particle diameters as stationary phase (AB, Bischoff Chromatography, Leonberg, Germany). In detail, 1 ml sample or standard and 100 µl sodium borate buffer (0.1 M, pH 10.5) were mixed for 50 s on a vortex. Then 50 µl of the OPA reagent (prepared fresh daily by dissolving 20 mg OPA and 200 µl 2-mercaptoethanol in 5 ml methanol) were added and mixed. After 2 min, 100 μl of this mixture was manually injected with a glass syringe (Hamilton 710, Rheodyne 8125, Wertheim-Mondfeld, Germany). The column was eluted using a ternary gradient consisting of solvent A (0.05 M sodium acetate, pH 8.4), solvent B (0.05 M sodium acetate, pH 5.4) and solvent C (100% MeOH) as follows: 0–70% B and 20–30% C for 13 min, then 70–56% B and 30–36% C for 9 min, 100% C for 28 min, back to 0% B and 20% C for 5 min, and finally 0% B and 20% C for 5 min. The flow rate was 1.0 ml min−1. The amino acids were detected fluorometrically at an excitation wavelength of 330 nm and an emission wavelength of 450 nm. For calibration and quantification of amino acids, a standard mixture of 18 amino acids (AA-S-18, Sigma) as well as L-ornithine was used. Quantification was carried out with the program EZChrom Elite 3.1.7 (Scientific Software, Agilent Technologies, USA).
Enzyme assays relating to proline metabolism
Extractions for the 6-h and 30-day assays were done at 4°C, following the method described by Plettner (Citation2002). Cells were homogenized in a pre-chilled mortar and pestle with 50 ml of 100 mM 2-(N-morpholino)ethanesulfonic buffer (MES, pH 6.5) containing 10 mM MgCl2.6H2O, 0.5% saccharose, 1 mM 1,1,1-trichloro-2,2-bis (4-chlorophenyl) ethane (DDT), 10% glycerol, 20 mg Polyclar AT, 15 mg Amberlite XAD-4, 1 tablet protease-inhibitor, 1 mM pepstatin and 2 mM Na-EDTA. The homogenates were ground in liquid nitrogen, incubated at room temperature for 15 min, and centrifuged (15 500 × g for 30 min). The enzymes were precipitated from the culture supernatant by adding ammonium sulphate to 80% saturation. The assay was left overnight and the precipitate collected by centrifugation at 3000 × g for 30 min, dissolved in resuspension buffer (100 mM MES buffer, pH 6.5, without Polyclar, Amberlite and Pepstatin), and frozen in 50 µl portions at −80 °C. Activities are expressed as units (U), which is defined as the amount of the enzyme that catalyses the conversion of 1 µmol of substrate per minute in relation to the protein concentration (mg). 100 µl deionized water was used as a blank in the following enzyme assays.
The ∆1-pyrroline-5-carboxylate synthase (P5CS) assay, which is the ATP- and NADPH-dependent reduction of glutamate to γ-glutamic semialdehyde, was carried out in K3PO4 buffer (500 mM, pH 7.0), 100 mM glutamic acid, 100 mM MgCl26H2O, 5 mM ATP, 10 mM NADPH and 100 μl enzyme extract, at 20 °C. The reaction velocity was measured as the rate of consumption of NADPH, monitored as decrease in absorption at 340 nm as a function of time, as described by Stines et al. (Citation1999).
∆1-pyrroline-5-carboxylate reductase (P5CR) activity was determined spectrophotometrically at 20 °C according to the method of Treichel (Citation1986), using an extinction coefficient of 6.317 l−1 mM−1 cm−1 of NADPH at 340 nm, in a reaction mixture containing 75 mM DDT, 5 mM ∆1-pyrroline-5-carboxylate (P5C), 15 mM NADPH, 50 µl of enzyme solution, and 500 mM K3PO4 buffer (pH 7.0), in a total volume of 900 µl.
Ornithine-δ-aminotransferase (δ-OAT) activity was assayed according to Vogel & Kopac (Citation1960), as modified by Yang & Kao (Citation1999). The assay mixture contained 100 µl enzyme extract and 160 µl potassium phosphate buffer (500 mm, pH 7.5) containing 60 mM L-ornithine, 20 mM α-ketoglutarate and 10 mM pyridoxal-5′-phosphate. The reaction medium was incubated at 30°C for 30 min. The reaction was stopped by adding 200 µl trichloroacetic acid (10%) and the colour was developed by incubating the reaction mixture with 200 µl o-aminobenzaldehyde (0.5%) in ethanol (95%) for 30 min in the dark. After centrifugation at 14 200 × g for 30 min, the absorbance at 444 nm of the clear supernatant fraction was measured.
Statistical analysis
To assess the temporal variability of the five diatom taxa, analyses of variance (one-way ANOVA) were performed on logarithmically transformed data (to achieve normality), testing each species separately. The relationships between the growth rates obtained from the different UV treatments (given in Scholz et al., Citation2014) and physiological characteristics (e.g. concentrations of amino acids, glucose, mannose, glycerol, saturated (SFA) and unsaturated fatty acids (UFA)) were evaluated using non-parametric Spearman rank correlation (rs) to avoid problems associated with non-normal data distribution. Canonical correspondence analysis (CCA), with a forward selection of variables, was then applied to evaluate the relationship between the UV treatments used during the long-term experiments and the physiological responses and growth rates of the five diatom taxa. This analysis was done using a covariance matrix with logarithmic transformation [log (x + 1)] for the physiological data and variation range for the biological data. Altogether, 14 physiological variables (proline, aspartic acid, glutamic acid, cysteine, lysine, phenylalanine, threonine, tryptophan, tyrosine, glucose, galactose, mannose, SFA, UFA) and 15 biological variables were used (cell numbers of the five taxa, each grown at PAR+UV-A, PAR+UV-B and PAR+UV-A+UV-B). In order to verify the probability that the eigenvalues of the axes had been attributed by chance, a Monte Carlo test was applied (999 interactions; P ≤ 0.05). All tests were performed with the program XLSTAT 2011, version 2011.2.08 (Addinsoft, New York).
Results
Total carbohydrate and lipid contents
The initial gross composition of each species (i.e. the lipid and carbohydrate contents in cultures exposed only to PAR) showed significant differences between the individual species (). In detail, Diploneis littoralis had the highest carbohydrate content (2.91 µg cell−1, ), whereas two other species (Amphora exigua and Achnanthes exigua) had the highest lipid content (4.8 and 5.2 µg cell−1, respectively, ). Changes in total carbohydrate and lipid occurred with both the wavelength and duration of irradiance. Increases of total carbohydrate were observed in most species after 6 h UV exposure, being significant for D. littoralis in the UV-A assay (ANOVA F1,42 = 27.3, P < 0.0001, ) and Achnanthes exigua in the combined UV-A and UV-B treatment (ANOVA F1,36 = 29.5, P < 0.0001, ). Similarly, total lipid per cell also increased in most tested species after short-term UV-exposure. The total lipid content of Amphora exigua increased significantly after 6 h UV-B exposure (ANOVA F1,36 = 31.4, P < 0.0001: ). Furthermore, long-term exposure led only to an enhancement of the distribution pattern found before.
Fig. 1. Temporal variations in carbohydrate (A‒D) and lipid (E–H) concentrations for Achnanthes exigua (▀), Amphora exigua (▴), Cocconeis peltoides (◯), Diploneis littoralis () and Navicula digitoradiata (◊) at PAR, PAR + UV-A, PAR + UV-B and PAR + UV-A + UV-B, through initial, short- (6 h) and long-term experiments (30 days).
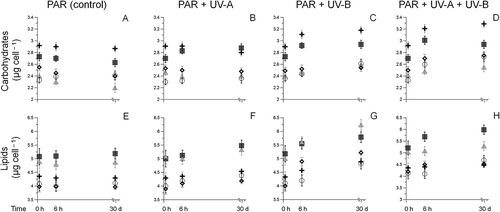
Composition of carbohydrates
With the exception of a higher glucose content in Achnanthes exigua (average difference 8.9%, ), the initial sugar composition of diatom taxa did not vary appreciably (). In general, glucose was the predominant sugar, ranging from 22.6% in D. littoralis to 25.9% in N. digitoradiata. Galactose and mannose (13.5–19.8%) were also common, with arabinose, ribose, xylose and rhamnose found in smaller varying proportions (5.8–13%).
Fig. 2. Variations in the main carbohydrate concentrations (mean ± SD) for (A) Achnanthes exigua, (B) Amphora exigua, (C) Cocconeis peltoides, (D) Diploneis littoralis and (E) Navicula digitoradiata at the start of the experiments at 20 °C (PAR = 600 µmol photons m−2 s−1).
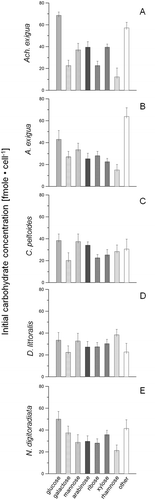
Differences occurred from 6 h onwards after UV exposure (). Relative carbohydrate compositions changed to a predominance of galactose in all tested species after short- and long-term UV experiments. This effect was more pronounced after UV-B and UV-A+UV-B treatments than under UV-A exposure alone, being significant for C. peltoides after short-term exposure (ANOVAPAR-control to UV-A/UV-B assay F1,6 = 28.2, P < 0.0001; ) and for D. littoralis after long-term exposure (ANOVAPAR-control to UV-A/UV-B assay F1,6 = 30.4, P < 0.0001; ).
Fig. 3. Temporal variations in relative carbohydrate compositions of (A, B) Achnanthes exigua, (C, D) Amphora exigua, (E, F) Cocconeis peltoides, (G, H) Diploneis littoralis and (I, J) Navicula digitoradiata at PAR, PAR + UV-A, PAR + UV-B and PAR + UV-A + UV-B, during 6-h (A, C, E, G, I) and 30-day (B, D, F, H, J) experiments.
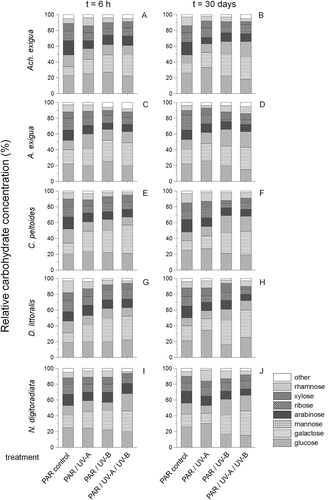
Composition of free fatty acids
The main fatty acids found in the tested five diatom taxa were the unsaturated 16:1 (palmitoleic acid = PLA, 16:1(n-7)), 16:4(n-1) and 20:5 (eicosapentaenoic acid = EPA, 20:5(n-3)) compounds, with the dominant saturated fatty acids being 14:0 (myristic acid = MA) and 16:0 (palmitic acid = PA) (, representing t = 0 h). From the 18-carbon fatty acids only the monosaturated oleic acid (18:1(n-9)) and stearidonic acid (SDA, 18:4(n-3)) were recorded in high amounts (3.4–7.5% and 8.6–10.2% of the total fatty acids, respectively). With the exception of significant variations in the 16:1(n-7) and 20:5(n-3) fatty acids (P < 0.05), this initial composition pattern varied only slightly between the five species (with differences of up to 8.8%, ANOVA: F1,86 = 4.6, P = 0.021) but changed both as a function of time and irradiance (). While UV-A short-term exposure seemed not to affect the free fatty acid compositions (P > 0.05), the UV-B and combined UV-A+UV-B treatments exhibited significant increases of EPA in Achnanthes exigua and Amphora exigua (11.8 and 13.9%, respectively, ANOVA F1,12 = 21.8, P < 0.0001, , C). There was also a noteworthy decrease of MA and 18:0 stearic acid (SA) in all taxa after UV-B and UV-A+UV-B short-term exposure (ANOVA: F1,93 = 30.4, P < 0.0001; , C, E, G, I). During the course of the experiment, only a marginal decrease of PUFAs was observed in the control cultures (, D, F, H, J). As in the short-term experiments, however, after 30 days the amounts of saturated and monounsaturated fatty acids (MUFAs) decreased while PUFAs increased in most UV-B and UV-A+UV-B-treatments. The increase in PUFAs concerned mainly the 16:4 acids, PLA and EPA in most tested species. Significant increases of EPA in D. littoralis (ANOVA F1,6 = 25.9, P < 0.0001, ) and PLA in Amphora exigua (ANOVA F1,6 = 30.7, P < 0.0001, ) were observed in the combined UV-A+UV-B-assay. In contrast to the observations for the short-term treatment, the long-term UV-A-treatments showed a general reverse development as compared with the short-term ones. Here significantly higher amounts of EPA were found in C. peltoides (ANOVA: F1,6 = 26.7, P < 0.0001 ), D. littoralis (ANOVA: F1,6 = 29.2, P < 0.0001, ) and N. digitoradiata (ANOVA: F1,6 = 25.8, P < 0.0001, ), showing an average difference compared with the long-term PAR-control of up to 19.3%.
Fig. 4. Variations in the free fatty acid concentrations (mean ± SD) for Achnanthes exigua, Amphora exigua, Cocconeis peltoides, Diploneis littoralis and Navicula digitoradiata at the start of the experiments at 20 °C (PAR = 600 µmol photons m–2 s–1).
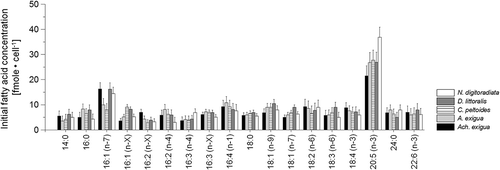
Fig. 5. Temporal variation in relative free fatty acid compositions of (A, B) Achnanthes exigua, (C, D) Amphora exigua, (E, F) Cocconeis peltoides, (G, H) Diploneis littoralis and (I, J) Navicula digitoradiata at PAR, PAR+UV-A, PAR+UV-B and PAR+UV-A+UV-B, during 6-h (A, C, E, G, I) and 30-day (B, D, F, H, J) experiments.
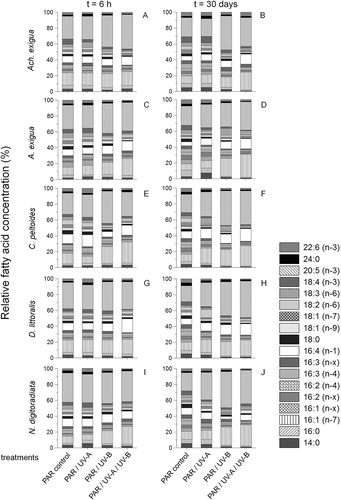
Free amino acid composition
Generally, the essential amino acids (aspartic acid, histidine, isoleucine, leucine, lysine, threonine, phenylalanine and valine) represented nearly one half of the total amino acid pool (~ 48.2%). As only slight variations and low final concentrations of the amino acids histidine, ornithine, arginine, methionine, alanine, serine, glycine, valine, isoleucine and leucine were observed during and after the conducted experiments; we suggest that these amino acids are not involved in UV acclimatization. Therefore, these amino acids have not been considered in the following analyses in detail; they were added up and designated as ‘other’ in and .
Fig. 6. Variations in the main free amino acid concentrations (mean ± SD) for (A) Achnanthes exigua, (B) Amphora exigua, (C) Cocconeis peltoides, (D) Diploneis littoralis and (E) Navicula digitoradiata at the start of the experiments at 20 °C (PAR = 600 µmol photons m−2 s−1).
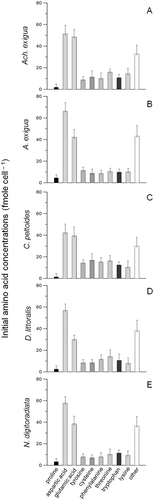
Fig. 7. Temporal variations in relative free amino acid compositions of (A, B) Achnanthes exigua, (C, D) Amphora exigua, (E, F) Cocconeis peltoides, (G, H) Diploneis littoralis and (I, J) Navicula digitoradiata at PAR, PAR+UV-A, PAR+UV-B and PAR+UV-A+UV-B during 6-h (A, C, E, G, I) and 30-day (B, D, F, H, J) experiments.
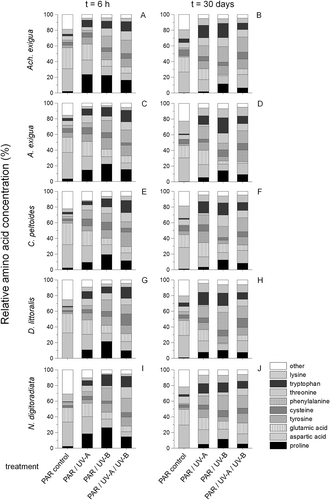
With the exception of significantly higher values of glutamic acid in Achnanthes exigua compared with the other four isolates at t = 0 (ANOVA, F1,15 = 26.9, P < 0.0001, ), the initial free amino acid compositions of the five benthic diatom taxa () as well as in the PAR-controls during the experiments varied only slightly (), showing insignificant variations of serine, arginine and glycine (P > 0.05). Differences occurred mainly in the UV-B and the combined UV-A+UV-B treatments after 6 h exposure. In Amphora exigua the levels of free proline rose significantly from initial values of 4.1–6.5 fmole cell–1 in the PAR-control to 93.8–130.6 fmole cell–1 in the UV-B-assay (ANOVA: F1,27 = 32.6, P < 0.0001, ). Tryptophan showed the highest relative concentration in the UV-B-treatment of N. digitoradiata (14.4%; ANOVA: F1,27 = 36.8, P < 0.0001, ), whereas in the combined UV-treatment relative values ranged from 13.7% (Achnanthes exigua, ) to 15.3% (C. peltoides, ) of the total amino acids (ANOVA: F1,27 = 31.2, P < 0.0001). In addition, there were significant differences in the proportions of tyrosine (11.2–18.6%), threonine (9.2–15.2%) and cysteine (2.3–15.8%) after short-term exposure to the combined UV-treatment (P < 0.05). As time advanced, the UV-induced levels of free proline decreased in all tested species (t = 30 days, , D, F, H, J). In contrast, after 30 days there were high relative concentrations of phenylalanine in all UV treatments, being significant for D. littoralis in the UV-B assay (ANOVA: F1,27 = 29.0, P < 0.0001, ). Finally, the proportions of lysine showed small but significant increases in all treatments of the long-term assays (P < 0.05).
The specific activities of the enzymes involved in proline metabolism (P5CS, P5CR and δ-OAT) showed distinct differences over time (). Generally, the highest enzyme activities were recorded in the short-term UV-B treatments, not after 30 days, confirming the observation that proline was not significantly involved in the long-term adaptation process in the diatom taxa (P > 0.05, , D, F); this agrees with the decreasing relative proline concentrations in the long term in all taxa tested (cf. ). Moreover, distinct differences were detected in comparison of the accumulated enzymes between the five tested species. For example, D. littoralis displayed the highest P5CS activity after 6 h UV-B exposure, indicating that the glutamate pathway may contribute here significantly to the UV-induced accumulation of free proline (ANOVA: F1,25 = 27.8, P < 0.0001, ). In contrast, P5CR was more important in UV-assays with Amphora exigua (ANOVA: F1,25 = 29.3, P < 0.0001, ), whereas C. peltoides showed the highest δ-OAT activities (ANOVA: F1,25 = 30.8, P < 0.0001, ). Finally, a small but significant increase of δ-OAT activities was also observed in assays of Achnanthes exigua and Amphora exigua after short-term UV-B and UV-B+UV-A exposure (ANOVA: F1,24 = 26.3 and F1,25 = 25.9, P < 0.0001, ).
Fig. 8. Temporal variations in proline dependent enzyme activities (U) (mean ± SD) of, (A-B) P5CS, (C-D) P5CR and (E-F) δ-OAT for Achnanthes exigua, Amphora exigua, Cocconeis peltoides, Diploneis littoralis and Navicula digitoradiata at PAR, PAR+UV-A, PAR+UV-B and PAR+UV-A+UV-B, during 6-h (A, C, E) and 30-day (B, D, F) experiments.
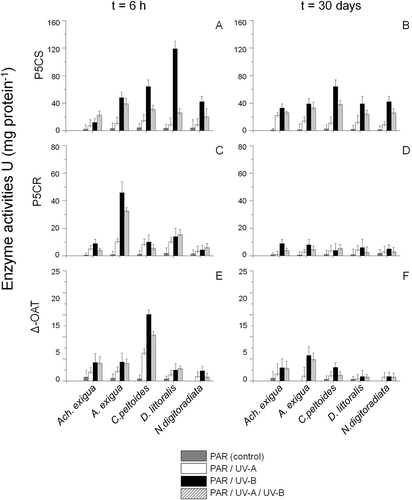
Non-parametric Spearman rank correlations and canonical correspondence analysis of physiological responses to different UV treatments
Analysis of Spearman rank correlations showed distinct differences between the physiological responses of the five tested diatom taxa to the long-term UV treatments (). While all five diatom species showed a significant positive response to long-term UV-B and UV-A+UV-B exposure with respect to the amino acid proline (P < 0.05), only three out of the five species showed a significant positive relationship to this peculiar amino acid in the UV-A treatment. Furthermore, phenylalanine seemed to be the second most important amino acid in the acclimatization process to different UV treatments, being significant for four out of the five tested taxa in the UV-A treatment and three of them in the UV-B treatment. In contrast, in the combined UV treatment all tested taxa showed a significant positive relationship with respect to phenylalanine. Besides phenylalanine, aspartic acid, mannose and galactose were significant for most of the tested species in the combined UV-A+UV-B treatment. In contrast, the UV-B treated species were predominantly significantly correlated with the accumulation of tryptophan, glucose, galactose and mannose. The highest positive correlation between the accumulation of glucose and the diatom taxa was displayed by D. littoralis (rs > 0.7) in the UV-A assay. Finally, saturated fatty acids were significantly correlated to all tested taxa in the UV-A treatment, whereas only three out of the five tested species in the UV-B assay and two in the combined UV assay showed significant correlations to unsaturated fatty acids.
Table 1. Non-parametric Spearman rank correlations (rs-values) for physiological variables measured for five diatom species after 30 days’ exposure to PAR/UV-A, PAR/UV-B and PAR/UV-A/UV-B. Significance level (P values) * < 0.05, ** < 0.01, *** < 0.001. Abbreviations: ARA: arabinose, ASP: aspartic acid, CYS: cysteine, GAL: galactose, GLU: glutamic acid, GLUC: glucose, LYS: lysine, MAN: mannose, RAH: rhamnose, PHE: phenylalanine, PRO: proline, UFA: unsaturated fatty acids, RIB: ribose, SFA: saturated fatty acids, THR: threonine, TRY: tryptophan, TYR: tyrosine, UFA: unsaturated fatty acids, XYL: xylose, n.s.: not significant (α < 0.05).
Several physiological variables were closely correlated with the growth data of the tested diatom species according to the canonical correspondence analysis (), where the first two CCA axes explained 80.4% of the variance. In particular, the first CCA axis was positively correlated with phenylalanine, followed by tryptophan, proline and the unsaturated fatty acids (including mono- and polyunsaturated ones). In addition, the first CCA axis was negatively associated with tyrosine, threonine and cysteine. Phenylalanine and glucose accounted for 14% of the overall variance among the physiological variables. With the second CCA axis only cysteine showed a strong negative correlation. The taxa were distributed according to the degree of physiological response to the different UV treatments. Species arranged towards the border of the plot showed specializations regarding certain physiological variables, such as N. digitoradiata to phenylalanine in the UV-B assay. In this context, the strong positive correlation between the first CCA axis and UV-B treated species is noteworthy.
Fig. 9. Canonical correspondence analysis plot of Axes 1 and 2 showing species groupings of Achnanthes exigua (Ach. ex.), Amphora exigua (A. ex.), Cocconeis peltoides (C. pel.), Diploneis littoralis (D. lit.) and Navicula digitoradiata (N. dig.) in relation to 14 physiological variables measured at □ PAR + UV-A, ○ PAR+UV-B and ∆ PAR+UV-A+UV-B during a 30-day experiment. The lengths of the arrows represent the relative importance of different variables in explaining species distributions, while the angles of the arrows relative to the axes and to other variables indicate the strength of their correlations. Abbreviations: ASP: aspartic acid, CYS: cysteine, GAL: galactose, GLU: glutamic acid, GLUC: glucose, LYS: lysine, MAN: mannose, PHE: phenylalanine, PRO: proline, SFA: saturated fatty acids, THR: threonine, THY: tryptophan, TYR: tyrosine, UFA: unsaturated fatty acids.
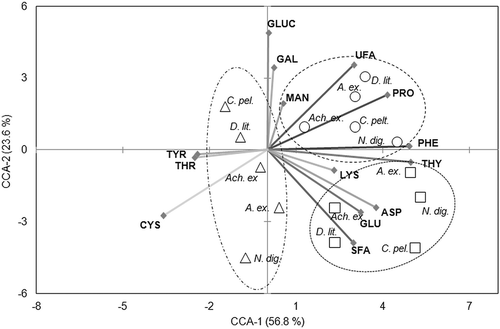
Discussion
UV effects on total carbohydrate and lipid content
Accumulation of intracellular photosynthetic products (lipids or carbohydrates) is a common, although not unique, property of UV-stressed algae (Shifrin & Chisholm, Citation1981; Harrison et al., Citation1990; de Madariaga & Joint, Citation1992). In the present study, carbohydrate and lipid contents seemed to be affected by UV, particularly UV-B, while in the control treatment, the biochemical compositions of the five diatom taxa were similar to those published for other diatoms (e.g. Rousch et al., Citation2003; Pistocchi et al., Citation2005). Karentz et al. (Citation1991) and Veen et al. (Citation1997) stated that a general increase in cell dry weight of UV-B stressed algae reflects storage of proteins and carbohydrates due to delayed cell division. In this context, it is necessary to distinguish between short- and long-term UV effects in our results. In the first part of the study (short-term exposure) the specific growth rates of the five diatom taxa did not show significant differences in comparison to the PAR control (P > 0.05), whereas 4 hours of daily exposure to UV-B and UV-A+UV-B for 30 days led to decreasing cell numbers in all tested species (Scholz et al., Citation2014). Thus, the observed increase in carbohydrates after long-term UV exposure in our data may be linked to the decline in cell division found for the five taxa in the first part of the study. In addition, our carbohydrate analysis did not allow differentiation between intra- and extracellular monosaccharides. Consequently, the increased carbohydrate contents might also be derived from the exopolysaccharide fractions of the tested diatom taxa. Generally, the excretion of EPS can create a boundary between cells and the surrounding environment and sometimes fulfils a protective role against desiccation (De Caiola et al., Citation1996; De Philippis et al., Citation2001); it allows heterotrophic metabolism during prolonged periods of detrimental conditions (Gross et al., Citation1998). Some studies also have revealed that UV-B induces the synthesis of exopolysaccharides in cyanobacteria, providing a matrix for mycosporine amino acids (Ehling-Schulz et al., Citation1997; Quesada et al., Citation1999). Finally, Chen et al. (2009) suggested that exopolysaccharides protect against DNA strand breaks and lipid peroxidation in a cyanobacterium by effectively eliminating reactive oxygen species induced by UV-B radiation.
UV effects on carbohydrate composition
In the present study, glucose accounted for > 34% of the carbohydrate fraction, which is slightly higher than the 20–30% recorded in other studies (Hama, Citation1988). This greater amount of glucose does not seem to be uncommon, however, because results for an inshore population of phytoplankton dominated by diatoms also revealed that glucose could account for 65–85% of total monosaccharides contents in the carbohydrate pool (Hama & Handa, Citation1992). Percival et al. (Citation1980), showed that p-1,3-D-glucan, the major storage carbohydrate in phytoplankton, yielded mainly glucose upon hydrolysis. Studies on biosynthetic pathways of p-1,3-D-glucan also support this observation and have revealed that D-glucose is the starting compound for storage glucan and is first incorporated into UDP-a-D-glucose and then into p-1,3-D-glucan (Mackie & Preston, Citation1974). Among the major constituents of the carbohydrate fraction, the relative concentrations of galactose in the UV-treated species represented the most profound differences from the PAR-controls. Similarly, in response to UV-B treatments galactose was found as the predominant fraction, besides glucose and arabinose, in EPS from bacteria (Wang et al., Citation2007) and Goes et al. (Citation1996) stated that higher values for mannose, galactose and glucose in their UV-treated samples indicate conclusively that synthesis rates of structural carbohydrates are accelerated in the presence of UV. In addition to galactose, mannose and glucose also contributed significantly to the overall increase in the monosaccharide content of the carbohydrate pool in the UV-treated taxa in the present investigation.
In microalgae, sugars and polyols are commonly the most important osmoprotectants in response to salinity change (Hu, Citation2004). In diatoms, the only carbohydrate reported to accumulate as a response to salinity increases is mannose, in Cylindrotheca fusiformis (Paul, Citation1979). Mannose accumulation was also recorded in relation to temperature fluctuations in our previous studies on microphytobenthic diatoms (Scholz & Liebezeit, Citation2013). In the present investigation, mannose accumulation was predominantly a response to long-term UV exposure (UV-B and combined UV-A+UV-B) and detected in all five species; during short-term treatments increases were recorded only in Amphora exigua and N. digitoradiata, in the UV-A and combined UV-A+UV-B assays respectively. Although the basis of any UV protection by galactose, mannose and glucose (e.g. by scavenging free radicals) is unclear, our data indicate a direct correlation between UV exposure and accumulation of these sugars. For example, the highest antioxidant capacity was found in Achnanthes exigua in the first part of this study (Scholz et al., Citation2014), correlating in the present study with relatively high amounts of mannose and galactose, at least in the combined UV-A+UV-B assay (). Other diatom taxa in this study also contained relatively high concentrations of these monosaccharides in the UV treatments. However, further investigations of the scavenging properties of carbohydrates are needed.
UV effects on fatty acid compositions
Lipids are major components of all biological membranes and can be destroyed by UV-B in the presence of oxygen. Hence peroxidation of unsaturated fatty acids has a direct effect on membrane structure and the formation of lipid peroxy radicals can induce further damage (Murphy, Citation1983; Bischof et al., Citation2007). Several studies have reported that UV-B has qualitative effects on the distribution of fatty acids (FA) in algae (e.g. Goes et al., Citation1994; Wang & Chai, Citation1994; De Lange & Van Donk, Citation1997). Besides others, the relative increase of short-chained fatty acids and a decrease of polyunsaturated fatty acids (PUFAs) are described as common responses in UV-stressed phytoplankton cells (e.g. Garcia-Pichel et al., 1992). Our data suggest that the impact of UV depends on the quality of the irradiance. In the UV-A assays, saturated fatty acids (SFAs) such as palmitic and myristic acid seemed to be more important than unsaturated ones. In contrast, 60% of the tested species accumulated unsaturated fatty acids such as palmitoleic and eicosapentaenoic acid in response to UV-B. So UV-B radiation resulted in an increase of PUFAs and a reduction of SFAs in most of the five tested diatoms. This is in line with a study by Hessen et al. (Citation1997), where important membrane fatty acids like eicosapentaenoic acid and docosahexaenoic acid seemed susceptible to UV radiation, owing to lipid peroxidation or reduced biosynthesis. Interestingly, in two of the species we studied (C. peltoides and D. littoralis) PAR+UVA+UVB induced an increase in the unsaturated fatty acids, whereas the other three taxa showed significant levels of saturated ones (P < 0.05). Here, the increased production of SFA at the expense of PUFAs could be an important strategy, as SFAs can serve as an important energy source for adaptation to UV-B stress (Teoh et al., Citation2005).
UV effects on amino acid compositions
Proline is considered to be involved in the osmotic adjustment of benthic marine diatoms (Scholz & Liebezeit, Citation2012a). That is, it acts as a compatible osmolyte and functions as a protector of macromolecules such as proteins and membranes, and as a nitrogen-storage compound and energy source after stress release (Aspinall & Paleg, 1981; Delauney & Verma, Citation1993). Furthermore, proline accumulation has been reported during nitrate deficiency (Miflin & Lea, Citation1977). In the present study, the short-term response to UV included an increase in proline levels. The consistency in the time-course changes of δ-OAT and P5CR activities and free proline levels during the early period of UVR-treatments suggests that the UV-stress-induced free proline accumulation may be due to a stimulation of synthesis via the ornithine pathway, at least in Amphora exigua. Only in D. littoralis did the glutamate pathway seem to be preferred (). In addition, it is evident that both the glutamate and the ornithine pathways for proline synthesis co-exist in C. peltoides, due to the different specific enzyme activities during exposure to UV-radiation. However, the stimulation of enzyme synthesis (δ-OAT, PC5S and P5CR) is a factor contributing to the UV-stress-induced free proline accumulation.
Besides proline, several other free amino acids have been detected in marine diatoms with possible functions as organic osmolytes, such as glutamic acid (Fujii et al., Citation1995; Nothnagel, Citation1995), alanine (Nothnagel, Citation1995) and taurine (Jackson et al., 1992). All these compounds have been mentioned in relation to salinity stress (Fujii et al., Citation1995; Nothnagel, Citation1995). The role of these and other amino acids in UV-acclimation in benthic diatoms has apparently not been considered so far. While taurine was present only in marginal concentrations, the five analysed diatom taxa showed significant variations of phenylalanine and tryptophan in relation to UV-exposure. In the presence of UV, the decrease in the relative concentrations of glutamic acid and aspartic acid, and the increase in phenylalanine, represented the most conspicuous changes within the amino acid pools of the five diatom taxa. We know of no published examples of algae using phenylalanine as a UV acclimation agent, but several reports exist of higher plants producing UV-absorptive secondary products derived from phenylalanine, such as flavonoids, in response to UV-B stress (e.g. Li et al., Citation1993). Interestingly, the highest flavonoid concentrations were detected in Achnanthes exigua and N. digitoradiata after long-term UV-exposure (UV-B and UV-B+UV-A) in the first part of the study (Scholz et al., Citation2014), corresponding in the present study to higher relative concentrations of phenylalanine (). Similarly, Amphora exigua and D. littoralis in the short-term UV-B assay, as well as Amphora exigua and C. peltoides in the long-term one, showed higher concentrations of flavonoids (Scholz et al., Citation2014), which might also be related to the increasing phenylalanine concentrations detected in the present study. Although we did not conduct further investigations on flavonoid synthesis in marine benthic diatoms, there are some studies showing the presence of flavonoids in planktonic species such as Skeletonema costatum in connection with antioxidant activities (e.g. Shanmugapriya et al., Citation2010).
As pointed out above, changes in the relative concentrations of the amino acid tryptophan were significant in Achnanthes exigua, Amphora exigua and N. digitoradiata during short-term UV-A+UV-B-exposure, as well as for all tested diatom taxa in the long-term combined treatment. Especially tryptophan, but also tyrosine, cysteine and glutathione, show absorption maxima in the UV region (200–300 nm) and hence may protect the cells by absorbing a substantial amount of UV-B radiation (Xue et al., Citation2005). Indeed, the protective effects of cysteine, reduced glutathione, tryptophan and sodium pyruvate against UV-B-induced damage have been studied in the nitrogen-fixing cyanobacterium Nostoc muscorum (Tyagi et al., Citation2003). We did not analyse for glutathione, but tyrosine and cysteine were also detected in small but significant concentrations in our study, varying among species; they may be actively involved in UV acclimation. Generally, the removal of singlet oxygen, as well as direct absorption of UV-B by these amino acids, suggests that they may help limit UV-B damage (Xue et al., Citation2005), functioning like other UV-B protective compounds present in the cells of various algae (Sinha et al., Citation1998; Quesada et al., Citation1999).
Conclusions
The present study suggests that benthic marine Wadden Sea diatoms have considerable potential to change their biochemical composition in response to different UV irradiances, but not all diatoms respond equally. All species enhanced the production of several compounds, including proline, phenylalanine, tryptophan, glucose, mannose and galactose. Besides carbohydrates, lipids were found to be the most important compounds in acclimatization to UV-B radiation. The accumulation of saturated and monounsaturated fatty acids and the decline in PUFA synthesis amply demonstrate that UV radiation can induce changes in benthic diatom lipid biochemistry through its effect on fatty acid biosynthesis. The intracellular concentrations of the accumulated compounds depend on the physiological potential of each diatom species. Some diatoms are able to produce more protectants and scavenging compounds and should therefore be able to grow at higher UV irradiances. These different acclimation potentials may be important for niche separation in intertidal flats.
Acknowledgements
We are grateful to Dr Daniel Ziehe and Professor Dr Ken Ryan for their really helpful comments on the manuscript.
References
- Arts, M.T., Rai, H. & Tumber, V.P. (2000). Effects of artificial UV-A and UV-B radiation on carbon allocation in Synechococcus elongatus (cyanobacterium) and Nitzschia palea (diatom). Verhandlungen Internationale Vereinigung für Limnologie, 27: 1–8.
- Aspinall, D. & Paleg, L.G. (1981). Proline accumulation: physiological aspects. In Physiology and biochemistry of drought resistance in plants (Aspinall, D. & Paleg, L.G., editors), 206‒241. Academic Press, Australia.
- Bates, L.S., Waldren, R.P. & Teare, I.D. (1973). Rapid determination of free proline for water-stress studies. Plant and Soil, 39: 205–207.
- Ben-Amotz, A., Tornabene, T.G. & Thomas, W.H. (1985). Chemical profile of some selected species of microalgae with emphasis on lipids. Journal of Phycology, 21: 72‒81.
- Bischof, K., Gómez, I., Molis, M., Hanelt, D., Karsten, U., Lüder, U., Roleda, M.Y., Zacher, K. & Wiencke, C. (2007). Ultraviolet radiation shapes seaweed communities. Reviews in Environmental Sciences and Biotechnology, 5: 141–166.
- Bligh, E.G. & Dyer, W.J. (1959). A rapid method of total lipid extraction and purification. Canadian Journal of Biochemistry and Physiology, 37: 911–917.
- Bligny, R., Roby, C. & Douce, R. (1989). Phosphorus-31 nuclear magnetic resonance studies in higher plant cells. In Nuclear magnetic resonance in agriculture (Pfeffer, P.E. & Gerasimowicz, W.V., editors), 72–87. CRC Press, Boca Raton.
- Buttery, M.J. (2000). Culture studies of two toxic dinoflagellate species, Alexandrium minutum and Gymnodinium catenatum. Ph.D. thesis, Murdoch University, Perth, Western Australia.
- Carreto, J.I., Carignan, M.O., Daleo, G. & De Marco, S.G. (1990). Occurrence of mycosporine-like amino acids in the red tide dinoflagellate Alexandrium excavatum: UV-photoprotective compounds? Journal of Plankton Research, 12: 909–921.
- Chen, T.H.H. & Murata, N. (2002). Enhancement of tolerance of abiotic stress by metabolic engineering of betaines and other compatible solutes. Current Opinion in Plant Biology, 5: 250–257.
- Chen, L.Z., Wang, G.H., Hong, S., Liu, A., & Liu, Y.A. (2009). UV-B-induced oxidative damage and protective role of exopolysaccharides in desert cyanobacterium, Microcoleus vaginatus. Journal of Integrative Plant Biology, 51: 194–200.
- De Caiola, M.G., Billi, D. & Friedmann, E.I. (1996). Effect of desiccation on envelopes of the cyanobacterium Chroococcidiopsis sp. (Chroococcales). European Journal of Phycology, 31: 97–105.
- De Lange, H.J. & Van Donk, E. (1997). Effects of UV-B irradiated algae on life history traits of Daphnia pulex. Freshwater Biology, 38: 711–720.
- Delauney, A.J. & Verma, D.P.S. (1993). Proline biosynthesis and osmoregulation in plants. Plant Journal, 4: 215–223.
- De Madariaga, J. & Joint, I. (1992). A comparative study of phytoplankton physiological indicators. Journal of Experimental Marine Biology and Ecology, 158: 149–165.
- De Philippis, R., Sili, C., Paperi, R. & Vincenzini, m. (2001). Exopolysaccharide producing cyanobacteria and their possible exploitation: a review. Journal of Applied Phycology, 13: 293–299.
- Ehling-Schulz, M., Bilger, W. & Scherer, S. (1997). UV-B-induced synthesis of photoprotective pigments and extracellular polysaccharides in the terrestrial cyanobacterium Nostoc commune. Journal of Bacteriology, 179: 1940–1945.
- Fujii, S., Nishimoto, N., Notoya, N. & Hellebust, J.A. (1995). Growth and osmoregulation of Chaetoceros muelleri in relation to salinity. Plant Cell Physiology, 36: 759–764.
- Garcia-Pichel, F., Sherry, N.D. & Castenholz, R.W. (1992). Evidence for an ultraviolet sunscreen role of the extracellular pigment scytonemin in the terrestrial cyanobacterium Chlorogleopsis sp. Phytochemistry & Photobiology, 56: 17–23.
- Garcia-Pichel, F., Kühl, M., Nübel, U. & Muyzer, G. (1999). Salinity-dependent limitation of photosynthesis and oxygen exchange in microbial mats. Journal of Phycology, 35: 227–238.
- Goes, J.I., Handa, N., Taguchi, S. & Hama, T. (1994). Effect of UV-B radiation on the fatty acid composition of the marine phytoplankton Tetraselmis sp.: relationship to cellular pigments. Marine Ecology Progress Series, 114: 259–274.
- Goes, J.I., Handa, N., Taguchi, S., Hama, T. & Saito, H. (1996). Metabolism of neutral monosaccharide constituents of storage and structural carbohydrates in natural assemblages of marine phytoplankton exposed to ultraviolet radiation. Limnology and Oceanography, 41: 1478–1489.
- Gross, W., Küver, J., Tischendorf, G., Bouchaala, N. & Büsch, W. (1998). Cryptoendolithic growth of the red alga Galdieria sulphuraria in volcanic areas. European Journal of Phycology, 33: 25–31.
- Guillard, R.R. (1975). Culture of phytoplankton for feeding marine invertebrates. In Culture of marine invertebrate animals (Smith, W.L. & Chanley, M.H., editors), 26–60. Plenum Press, New York.
- Häder, D.-P., Worrest, R.C., Kumar, H.D. & Smith, R.C. (1995). Effects of increased solar ultraviolet radiation on aquatic ecosystems. Ambio, 24: 174–180.
- Hama, T. (1988). I3C GC-MS analysis of photosynthetic products of the phytoplankton population in the regional upwelling area around the Izu Islands, Japan. Deep Sea Research, 35: 91–110.
- Hama, J, & Handa, N. (1992). Diel variation of water-extractable carbohydrate composition of natural phytoplankton populations in Kinu-ura Bay. Journal of Experimental Marine Biology and Ecology, 162: 159–176.
- Hare, P.D. & Cress, W.A. (1997). Metabolic implications of stress induced proline accumulation in plants. Plant Growth Regulation, 21: 79–102.
- Harrison, P.J., Thompson, P.A. & Calderwood, G.S. (1990). Effects of nutrient and light limitation on the biochemical composition of phytoplankton. Journal of Applied Phycology, 2: 45–55.
- Helbling, E.W., Chalker, B.E., Dunlap, W.C., Holm-Hansen, O. & Villafañe, V.E. (1996). Photoacclimation of Antarctic marine diatoms to solar ultraviolet radiation. Journal of Experimental Marine Biology and Ecology, 204: 85–101.
- Hessen, D.O., De Lange, H.J. & Van Donk, E. (1997). UV-induces changes in phytoplankton cells and its effects on grazers. Freshwater Biology, 38: 513–524.
- Hu, Q. (2004). Environmental effects on the cell composition. In Handbook of microalgal culture. Biotechnology and applied phycology (Richmond, A., editor), 83–93. Blackwell Publishing, Oxford.
- Jackson, A.E., Ayer, S.W. & Laycock, M.V. (1992). The effect of salinity on growth and amino acid composition in the marine diatom Nitzschia pungens. Canadian Journal of Botany, 70: 2189–2201.
- Jørgensen, B.B., Revsbech, N.P. & Cohen, Y. (1983). Photosynthesis and structure of benthic microbial mats: microelectrode and SEM studies of four cyanobacterial communities. Limnology and Oceanography, 28: 1075–1093.
- Karentz, D., McEuen, F.S., Land, M.C. & Dunlap, W. C. (1991). Survey of mycosporine-like amino acids compounds in Antarctic marine organisms: potential protection from ultraviolet exposure. Marine Biology, 108: 157–166.
- Karsten, U., Wiencke, C. & Kirst, G.O. (1991). Growth pattern and β-dimethylsulphonio-propionate (DMSP) content of green macroalgae at different irradiance. Marine Biology, 108: 151–155.
- Kates, M. & Volcani, B.E. (1966). Lipid composition of diatoms. Biochemica et Biophysica Acta, 116: 264–278.
- Kochert, G. (1978). Carbohydrate determination by the phenolsulfuric acid method. In Handbook of phycological methods: Physiological and biochemical methods (Hellebust, J.A. & Craigie, J.S., editors), 95–97. Cambridge University Press, Cambridge.
- Krell, A., Funck, D., Plettner, I., John, U. & Dieckmann, G. (2007). Regulation of proline metabolism under salt stress in the psychrophilic diatom Fragilariopsis cylindrus (Bacillariophyceae). Journal of Phycology, 43: 753–762.
- Kühl, M., Glud, R.N., Plough, H. & Ramsing, N.B. (1996). Microenvironmental control of photosynthesis and respiration and photosynthesis-coupled respiration in an epilithic cyanobacterial biofilm. Journal of Phycology, 32: 799–812.
- Li, J., Ou-Lee, T.A., Raba, R., Amundson, R.G. & Last, R.L. (1993). Arabidopsis flavonoid mutants are hypersensitive to UV-B irradiation. Plant Cell, 5: 171–179.
- Liebezeit, G. & Behrends, B. (1999). Determination of amino acids. In Methods of seawater analysis. 3rd ed. (Grasshoff, K., Kremling, K. & Ehrhardt, M., editors), 541–546. Wiley–VCH, Weinheim.
- Mackie, W. & Preston, R.D. (1974). Cell wall and intercellular region polysaccharides. In Algal physiology and biochemistry (Stewart, W.D.P., editor), 58–64. Blackwell Scientific Publications, Oxford.
- Mercz, T.I. (1994). A study of high lipid yielding microalgae with potential for large-scale production of lipids and polyunsaturated fatty acids. Ph.D. thesis, Murdoch University, Perth.
- Miflin, B.L. & Lea, P.J. (1977). Amino acid metabolism. Annual Review of Plant Physiology, 28: 299‒329.
- Murphy, T.M. (1983). Membranes as targets of ultraviolet radiation. Physiologia Plantarum, 58: 381–388.
- Nothnagel, J. (1995). The effects of salinity and light intensity on the osmolyte concentrations, cell volumes and growth rates of the Antarctic sea-ice diatoms Chaetoceros sp. and Navicula sp. with emphasis on the amino acid proline. PhD thesis, University of Bremen [Reports on Polar Research, 161]. Kamloth, Bremen.
- Odmark, S., Wängberg, S.Å., Sundbäck, K., Wulff, A. & Nilsson, C. (1998). Effects of UVB radiation on shallow-water marine microbenthic communities from a sandy sediment. Marine Biology, 132: 335–345.
- Paul, J.S. (1979). Osmoregulation in the marine diatom Cylindrotheca fusiformis. Journal of Phycology, 15: 280–284.
- Peletier, H. (1996). Long-term changes in intertidal estuarine diatom assemblages related to reduced input of organic waste. Marine Ecology Progress Series, 137: 265–271.
- Percival, E., Rahman, M.A. & Weigel, H. (1980). Chemistry of the polysaccharides of the diatom Coscinodiscus nobilis. Phytochemistry, 19: 809–811.
- Pistocchi, R., Trigari, G., Serrazanetti, G.P., Taddei, P., Monti, G., Palamidesi, S., Guerrini, F., Bottura, G., Serratore, P., Fabbri, M., Pirini, M., Ventrella, V., Pagliarani, A., Boni, L. & Borgatti, A.R. (2005). Chemical and biochemical parameters of cultured diatoms and bacteria from the Adriatic Sea as possible biomarkers of mucilage production. Science of the Total Environment, 353: 287–299.
- Plettner, I. (2002). Stressphysiologie bei antarktischen Diatomeen – ökophysiologische Untersuchungen zur Bedeutung von Prolin bei der Anpassung an hohe Salinitäten und tiefe Temperaturen. PhD thesis, University of Bremen, Germany.
- Quesada, A., Vincent, W. F. & Lead, D.R.S. (1999). Community and pigment structure of arctic cyanobacterial assemblages: the occurrence and distribution of UV-absorbing compounds. FEMS Microbiology Ecology, 28: 315–323.
- Reineck, H.-E. (1983). Sediment and dynamical processes. In Ecology of the Wadden Sea (Wolff, W.J., editor), 6–49. A.A. Balkema, Rotterdam, Holland.
- Rousch, J.M., Bingham, S.E. & Sommerfeld, M.R. (2003). Changes in fatty acid profiles of thermo-intolerant and thermo-tolerant marine diatoms during temperature stress. Journal of Experimental Marine Biology and Ecology, 295: 145–156.
- Scholz, B. & Liebezeit, G. (2012a). Composition of compatible solutes in marine intertidal microphytobenthic Wadden sea diatoms exposed to different salinities. European Journal of Phycology, 47: 393–407.
- Scholz, B. & Liebezeit, G. (2012b). Microphytobenthic dynamics in a Wadden Sea intertidal flat – Part I: Seasonal and spatial variations of diatom communities in relation to macronutrient availability. European Journal of Phycology, 47: 105–119.
- Scholz, B. & Liebezeit, G. (2012c). Microphytobenthic dynamics in a Wadden Sea intertidal flat – Part II: Seasonal and spatial variation of non-diatom community components in relation to abiotic parameters. European Journal of Phycology, 47: 120–137.
- Scholz, B. & Liebezeit, G. (2012d). Growth responses of 25 benthic marine Wadden Sea diatoms isolated from the Solthörn tidal flat (southern North Sea) in relation to varying culture conditions. Diatom Research, 27: 65–73.
- Scholz, B. & Liebezeit, G. (2013). Compatible solutes and fatty acid compositions of five marine intertidal microphytobenthic Wadden Sea diatoms exposed to different temperatures (southern North Sea). Diatom Research, 28: 337–358.
- Scholz, B., Rúa, A. & Liebezeit, G. (2014). Effects of UV radiation on five marine microphytobenthic Wadden Sea diatoms, isolated from the Solthörn tidal flat (Lower Saxony, southern North Sea) – Part I: Growth and antioxidative defence strategies. European Journal of Phycology, 49: 68–82.
- Shanmugapriya, R., Ramanathan, T., Satyavani, K. & Gurudeeban, S. (2010). Antioxidant and radical scavenging effect of a marine diatom species – Skeletonema costatum. Plant Archives, 10: 471–473.
- Shifrin, N.S. & Chisholm, S.W. (1981). Phytoplankton lipids: interspecific differences and effects of nitrate, silicate and light-dark cycles. Journal of Phycology, 17: 374–384.
- Sinha, R.P., Klisch, M., Groniger, A. & Hader, D.-P. (1998). Ultraviolet absorbing/screening substances in cyanobacteria, phytoplankton and macroalgae. Journal of Photochemistry and Photobiology B: Biology, 47: 83–94.
- Sivalingam, P.M., Ikawa, T., Yokohama, Y. & Nisizawa, K. (1974). Distribution of a 334 UV-absorbing-substance in algae, with special regard of its possible physiological roles. Botanica Marina, 17: 23–29.
- Skerratt, J.H., Davidson, A.D., Nichols, P.D. & McMeekin, T.A. (1998). Effect of UV-B on lipid content of three Antarctic marine phytoplankton. Phytochemistry, 49: 999–1007.
- Stines, A.P., Naylor, D.J., Høj, P.B. & van Heeswijck, R. (1999). Proline accumulation in developing grapevine fruit occurs independently of changes in the levels of Δ1-pyrroline-5-carboxylate synthetase mRNA or protein. Plant Physiology, 120: 923–931.
- Sundbäck, K., Odmark, S., Wulff, A., Nilsson, C. & Wängberg, S.Å. (1997). Effects of enhanced UV-B radiation on a marine benthic diatom mat. Marine Biology, 128: 171–179.
- Teoh, M.L., Chu, W.L., Marchant, H. & Phang, S.M. (2005). Influence of culture temperature on the growth, biochemical composition and fatty acid profiles of six Antarctic microalgae. Journal of Applied Phycology, 16: 421–430.
- Treichel, S. (1986). The influence of NaCl on Δ1-pyrroline-5-carboxylate reductase in proline-accumulating cell suspension cultures of Mesembryanthemum nodiflorum and other halophytes. Plant Physiology, 67: 173–181.
- Tyagi, R., Kumar, A., Tyagi, M.B., Jha, P.N., Kumar, H.D., Sinha, R.P. & Hader, D.P. (2003). Protective role of certain chemicals against UV-B-induced damage in the nitrogen-fixing cyanobacterium, Nostoc muscorum. Journal of Basic Microbiology, 43: 137–147.
- Underwood, G.J.C. & Kromkamp, J. (1999). Primary production by phytoplankton and microphytobenthos in estuaries. Advances in Ecological Research, 29: 93–153.
- Underwood, G.J.C. & Smith, D.J. (1998). Predicting epipelic diatom exopolymer concentrations in intertidal sediments from sediment chl. a. Microbial Ecology, 35: 116–125.
- Underwood, G.J.C., Boulcott, M., Raines, C.A. & Waldron, K. (2004). Environmental effects on exopolymer production by marine benthic diatoms – dynamics, changes in composition and pathways of production. Journal of Phycology, 40: 293–304.
- Veen, A., Reuvers, M. & Roncak, P. (1997). Effects of acute and chronic UV-B exposure on a green alga: a continuous culture study using a computer-control dynamic light regime. Plant Ecology, 128: 28–40.
- Vincent, W.F. & Roy, S. (1993). Solar ultraviolet-B radiation and aquatic primary production: damage, protection, and recovery. Environmental Reviews, 1: l–12.
- Vogel, R.H. & Kopac, M.J. (1960). Some properties of ornithine δ-transaminase from Neurospora. Biochimica et Biophysica Acta, 37: 539–540.
- Wang, H., Jiang, X., Mu, H., Liang, X. & Guan, H. (2007). Structure and protective effect of exopolysaccharide from P. agglomerans strain KFS-9 against UV radiation. Microbiological Research, 162: 124–129.
- Wang, K.S. & Chai, T.J. (1994). Reduction in omega-3 fatty acids by UV-B irradiation in microalgae. Journal of Applied Phycology, 6: 415–421.
- Wood, W.F. (1989). Photoadaptive responses of the tropical red alga Eucheuma striatum Schmitz (Gigartinales) to ultraviolet radiation. Aquatic Botany, 33: 41–51.
- Wöstmann, R. & Liebezeit, G. (2008). Allochthonous organic matter as carbon, nitrogen and phosphorus source on a sandbank island (Kachelotplate, Lower Saxonian Wadden Sea, Germany). Marine Biodiversity, 38: 153‒161.
- Xue, L., Zhang, Y., Zhang, T. & Wang, X. (2005). Effects of enhanced ultraviolet-B radiation on algae and cyanobacteria. Critical Reviews in Microbiology, 31: 79–89.
- Yang, C.W. & Kao, C.H. (1999). Importance of ornithine-δ-aminotransferase to proline accumulation caused by water stress in detached rice leaves. Plant Growth Regulation, 27: 189‒192.
- Zhang, Z., Xiao, Z. & Linhardt, R.J. (2009). Thin layer chromatography for the separation and analysis of acidic carbohydrates. Journal of Liquid Chromatography and Related Technologies, 32: 1711–1732.