Abstract
Arthrospira platensis is a cyanobacterium known for its nutritional value and secondary metabolites. Extracellular polymeric substances (EPS) are an important trait of most cyanobacteria, including A. platensis. Here, we extracted and analysed different fractions of EPS from a locally isolated strain of A. platensis. Three different fractions of EPS were distinguished. These were EPS released into the medium (REPS), EPS loosely bound to the organism (LEPS) and EPS tightly bound to the organism (TEPS), which were extracted by different procedures. The LEPS fraction was smaller than the other two fractions. The EPS of A. platensis exhibited high diversity. Total protein and carbohydrate content was determined in each of these fractions. The largest amount of total carbohydrates and total proteins was in the TEPS fraction. Eight sugar moieties were detected and analysed in all EPS fractions using HPAE-PAD. Fructose, mannose and ribose were rare sugar residues in all fractions of EPS. With the exception of fructose, all sugars tested for were detected in TEPS. The amount of sugars detected was significantly higher in TEPS compared with the two other fractions, especially for galactose, xylose and glucose. The EPS were localized by confocal laser scanning microscopy (CLSM) after staining with different fluorescent dyes and it was found that A. platensis possessed a thick and smooth layer of EPS around the spiral trichomes.
Introduction
Extracellular polymeric substances (EPS) are important compounds produced by many cyanobacteria; they surround the organisms as sheaths, capsules or mucilage. EPS provide protection from various biotic and abiotic stresses and facilitate the attachment of the organism to a solid substrate (Palmer et al., Citation2007; Pereira et al., Citation2009; Jittawuttipoka et al., Citation2013). Generally, EPS are categorized into three types: sheaths, capsules and mucilage, based on their location, structure and consistency. Capsules are considered to be highly structured, strictly delimited and tightly attached to the organism, whereas sheaths are less structured, not delimited, of varying thickness and not so intimately attached to the organism. Mucilage is unstructured and loosely associated with the organism or freely dispersed into the environment (Richert et al., Citation2005). In recent years, there has been great interest in the exploitation of EPS by various industrial sectors because of the chemical and physical properties of these polymers. For instance, EPS and EPS-producing microorganisms have been used for soil conditioning (Abed et al., Citation2009).
Arthrospira platensis (previously known as Spirulina platensis) belongs to the family Oscillatoriaceae and is a filamentous cyanobacterium with spiral trichomes. It is one of the best-studied and most widely used cyanobacteria in biotechnology because of its fast growth in open ponds under alkaline conditions that largely exclude contaminating organisms (Ahmed et al., Citation2010b). Arthrospira platensis has enormous potential for applications in a variety of fields such as food, cosmetics, environmental improvement, aquaculture and in the future perhaps therapeutics (Rossi et al., Citation2005; Trabelsi et al., Citation2009). Arthrospira platensis produces many metabolites that are either unique or found in higher concentrations in this organism than others and thus may be produced economically (Cohen, Citation1997). As is the case for many other cyanobacteria, Arthrospira secretes a variety of different EPS that can be extracted and used in different fields (Trabelsi et al., Citation2009), for instance, arthrospiral EPS has been reported to enhance human innate immunity (Lobner et al., Citation2008; Parages et al., Citation2012). The objective of the present work was (1) to extract different EPS fractions and characterize them biochemically and (2) to visualize the EPS of A. platensis without disturbing its structural integrity by using various lectins and confocal laser scanning microscopy.
Materials and methods
Strain and growth conditions
Arthrospira platensis MMG-9 (NCBI GenBank Accession number FJ839360 for the 16S rRNA gene sequence) was isolated from a rice field in Pakistan (Ahmed et al., Citation2010b). This Arthrospira strain was cultured and maintained in Spirulina medium (George, Citation1976) at 25°C at a 16 : 8 h light-dark cycle (200 µmol photons m−2 s−1). Cultures were harvested after 3 weeks of incubation for extraction of EPS.
Growth measurement
For estimation of growth, chlorophyll a was determined following the method of Tandeau de Marsac & Houmard (Citation1988). Cells were harvested by centrifugation at 10 000 × g for 10 min at 4°C. The pellets were extracted with 80% methanol for 2 h in the dark at 4°C. The extract was centrifuged at 10 000 × g for 10 min at 4°C and the absorbance of the supernatant was measured spectrophotometrically at 665 nm against 80% methanol. Chlorophyll content (µg ml−1) was calculated from the absorption at 665 nm (OD665nm) × 13.9.
Extraction of EPS
EPS were extracted according to Staats et al. (Citation2000) with modifications. The EPS can be tightly bound and remain attached to the cells, or released into the medium as REPS which can be recovered from the supernatant. Tightly bound EPS were extracted from the cells in two steps. Firstly, the loosely bound EPS (LEPS) were obtained by shaking in lukewarm water and secondly, the remaining tightly bound EPS (TEPS) was extracted with 0.1 M EDTA.
Arthrospira platensis was grown for 3 weeks in 1 litre culture flasks. Cultures were harvested by centrifugation at 5000 × g for 20 min at 20°C. For REPS, the supernatant was filtered through membrane filters (Millipore, 0.45 µm), freeze-dried, weighed and stored at −20°C until further analysis. For LEPS, 4.5 ml of MilliQ water was added to the cell pellet, vortexed for 1 min and subsequently incubated in a shaking water bath (120 rpm) at 30°C for 1 h. The cells were checked microscopically to ensure that they were not damaged. Subsequently, the extracts were centrifuged at 5000 × g for 20 min at 20°C. The supernatant containing the LEPS was filtered through membrane filters (Millipore, 0.45 µm), freeze-dried, weighed and stored at −20°C until further analysis. For the extraction of TEPS, the pellets resulting from the LEPS extraction were re-suspended in 4.5 ml of 0.1 M EDTA and incubated for 4 h in a shaking (120 rpm) water bath at 25°C. The cells were checked microscopically to ensure that no cell lysis had occurred, in order to minimize contamination of the extracts with intracellular carbohydrate. The extracts were centrifuged for 20 min at 5000 × g at 20°C and the supernatant containing the TEPS was filtered through a membrane filter (Millipore, 0.45 µm), freeze-dried, weighed and stored at −20°C until further analysis.
Measurement of total carbohydrates, total proteins, uronic acids and glycosaminoglycans (GAGs)
Known amounts of the three fractions of EPS were dissolved in MilliQ water. Total sugar content was determined according to Dubois et al. (Citation1956); total protein content was measured with the Bradford Protein Assay (Bio Rad Laboratories, Veenendaal, the Netherlands); uronic acids were measured with carbazole in 80% sulphuric acid with borate ions added (0.8% sodium borate – tetrahydrate and concentrated sulphuric acid mixed in ratio of 17 : 83) (Taylor & Buchanan-Smith, Citation1992), and glycosaminoglycan was measured with the dimethyl methylene blue method (Chandrasekhar et al., Citation1987).
Monosaccharide analysis
Monosaccharide composition of the three EPS fractions was analysed qualitatively and quantitatively using High Performance Anion Exchange Chromatography with Pulsed Amperometric Detection (HPAE-PAD). Known quantities of EPS were suspended in 4.5 ml MilliQ water and subsequently hydrolysed by adding 0.5 ml of 11 M H2SO4 to 4.5 ml EPS solution in a glass vial and incubating for 1 h at 120°C. Immediately after hydrolysis the glass vials were put on ice, BaCO3 was added to neutralize the solution and the vials were vigorously shaken. The pH of the solution was measured and BaCO3 was added until the pH reached 5–6. The hydrolysed solution was centrifuged for 20 min at 10 000 × g and 4°C, then the supernatants were filtered through a 0.22 µm filter (Millex-GV4; Millipore, Bedford, MA, USA) and stored in 1 ml glass vials at −20°C until further analysis (Boschker et al., Citation2008).
HPAE-PAD was carried out on a Dionex System (Dionex, Breda, the Netherlands) consisting of an HPLC pump (Dionex, P580), an autoinjector (Dionex, ASI-100) and an electrochemical detector (Dionex, ED40) fitted with a CarboPac PA20 guard and narrow-bore analytical column (3×150 mm; Dionex, Breda, the Netherlands) and eluted isocratically with 300 µl min−1 of 1 mM NaOH. The column was regenerated regularly with 200 mM NaOH. All eluents were degassed with helium before NaOH was added and further degassed with helium during analysis. All pump heads were rinsed at least once a day to prevent crystallization. Peaks were analysed with ChromeleonC Dionex Version 6.80 SR5 Build 2413. Peak identification was based on retention times in comparison with external standards. Concentration measurements were based on peak areas of the separated compounds and calibrated against external standards.
Localization of EPS by CLSM
EPS were localized using a confocal laser scanning microscope (Leica TCS-NT, Leica Lasertechnik Heidelberg, Germany) equipped with an Argon-Krypton laser and using the 564 and 488 nm lines. Leica 25 (NA 0.75) oil immersion and Leica 63 (NA 0.9) water immersion objectives were used (Ahmed et al., Citation2010a).
Autofluorescence of cyanobacterial chlorophyll was used for observation of the cells and the different components of EPS were stained using a variety of lectins and fluorescent dyes (). Multi-fluorochrome staining was performed using fluorescent dyes in series. Eighteen-day-old cultures were harvested by centrifugation for 10 min at 10 000 × g in Eppendorf tubes and the pellets were treated with the fluorescent dye according to the manufacturer’s instructions. Excitation wavelengths and emission filters of the CLSM were adjusted according to the specificity of fluorescent dye.
Table 1. Fluorescent dyes and conditions.
Results
Extraction of EPS
Extracted EPS appeared as a white material. A total of 561 mg EPS (g Chl a)−1 was extracted. Extraction and quantification of the three EPS fractions of A. platensis MMG-9 revealed that TEPS was the most abundant (49%) whereas the LEPS fraction was the least abundant: 15% of total EPS ().
Table 2. Biochemical characteristics of EPS fractions.
Measurement of total carbohydrates, total proteins, uronic acids and glycosaminoglycan
The quantity of total carbohydrate was highest (605 mg g−1) in TEPS and lowest (502 mg g−1) in REPS. Total protein content was significantly higher (ANOVA-DMRT at P = 0.05) in TEPS and REPS (). TEPS contain the highest amount of uronic acids and glycosaminoglycan (GAG), 21 mg g−1 and 16 mg g−1 of EPS dry weight, respectively ().
Monosaccharide analysis
Monosaccharide composition analysis revealed the heterogeneous nature of the EPS of A. platensis MMG-9. Of the eight sugars analysed by our method, seven were detected in the EPS (only fructose was not detected in any of the EPS fractions). Five sugars, i.e. fucose, galactose, glucose, rhamnose and xylose, were present in all three EPS fractions; ribose and mannose were only detected in TEPS. Neither ribose nor fructose have been reported to be common in cyanobacterial EPS.
Quantitative analysis of the three EPS fractions revealed that the different monosaccharides were present in varying amounts (). Among the seven monosaccharide residues measured in the three EPS fractions, glucose (198 µg (Chl a)−1) and galactose (237 µg (Chl a)−1) were present in significantly higher amounts in TEPS. Rhamnose was the most prominent fraction in REPS and LEPS, comprising 41% and 38%, respectively. Mannose and ribose were undetectable in REPS and LEPS. Xylose was present in abundance in TEPS (23%).
Table 3. Comparison of constituent monosaccharidic residues in different EPS fractions of A. platensis MMG-9.
Confocal laser scanning microscopy
EPS of A. platensis MMG-9 stained well with DTAF and the attached EPS were visible at high resolution along with details of the surface of the trichomes (–). CSA-TRITC stained EPS up to 1 µm distant from the cells (–). Con-A-FITC stained thin layers of EPS that seemed to be tightly associated with the cell and did not extend to the outer layers of the EPS (). Staining with Alexa Fluor 488 showed the presence of proteins attached to the trichomes but also in REPS, which is detached from the trichomes (). Lotus-FITC stains fucose in EPS and it seemed to stain the REPS in particular (–). A dense region consisting of a thick layer of EPS (up to 20 µm) was observed around the Arthrospira trichomes showing the presence of fucose-binding domains in this EPS ().
Figs 1–9. Localization of EPS from A. platensis MMG-9 using confocal laser scanning microscopy. Figs 1, 3. Overlaid images of autofluorescence and stained EPS with DTAF. Figs 2, 4. Spiral trichomes embedded in EPS stained with DTAF. Figs 5–6. Overlaid images of autofluorescence and stained EPS with CSA-TRITC. Fig. 7. Image of EPS stained with CSA-TRITC. Fig. 8. Staining with Con-A-FITC. Fig. 9. Staining with Alexa Fluor 488. Scale bars = 10 µm (Figs 1, 2), 15 µm (Figs 3, 4), 5 µm (Fig. 5), 10 µm (Figs 6–9). Red = cyanobacterial autofluorescence, green = EPS.
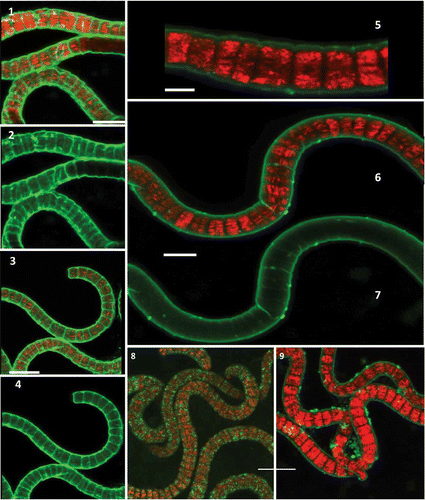
Figs 10–14. Localization of EPS from A. platensis MMG-9 using confocal laser scanning microscopy. Figs 10–12. Spiral filaments embedded in EPS stained with Lotus-FITC. Fig. 13. EPS clouds from A. platensis MMG-9 using confocal laser scanning microscopy and three dyes (Lotus-FITC, CSA-TRITC and Alexa Fluor 488). Fig. 14. z-stacks showing the depth of the image (numbers show depth in µm). Scale bars = 15 µm (Fig. 10), 10 µm (Fig. 11), 50 µm (Fig. 12), 10 µm (Fig. 13). Red = cyanobacterial autofluorescence, green = EPS.
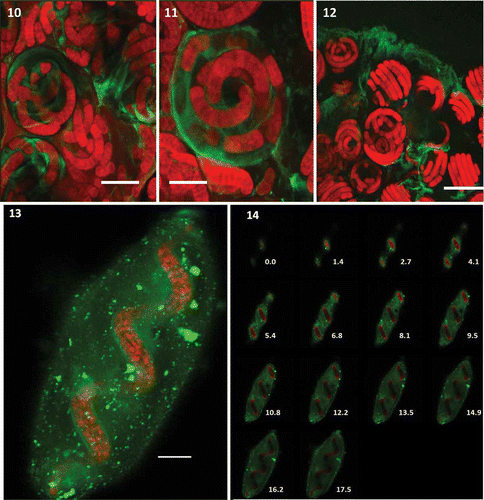
Staining with individual lectins resulted in the visualization of EPS, but in order to obtain a detailed insight into the EPS matrix around the cyanobacterial trichomes, three lectins with different dyes were used simultaneously (Lotus-FITC, CSA-TRITC and Alexa Fluor 488). Visualization after combined staining resulted in the detection of a cloud of EPS around the spiral trichome (). Analysis of 3D stacks showed the trichome spiral width to be 15–20 µm whereas the length of the trichome was 100 µm. EPS covering the trichomes was 10–20 µm in thickness showing EPS granules of various sizes that are more densely stained (); 1–2 µm thickness of TEPS was visible around the trichome.
Discussion
Cyanobacteria produce diverse exopolysaccharides in terms of their physical and chemical properties. Because of the large amounts of EPS produced by cyanobacteria and their potential industrial applications, cyanobacterial EPS have been studied extensively (Li et al., Citation2001). In the present study, EPS from A. platensis strain MMG-9 were the subject of investigation. They were extracted as three operationally defined fractions, of which REPS was the most abundant. Arthrospira platensis strain MMG-9 produced copious amounts of EPS composed of carbohydrates and protein in equal proportions in TEPS and LEPS. The latter fraction is composed of mucilage and its presence indicated that A. platensis strain MMG-9 continuously releases EPS. The proportions of released and bound EPS in various studies show a high variability (Gloaguen et al., Citation1995; Nicolaus et al., Citation1999); such variability may be strain dependent or the result of the cultivation conditions. Virtually any environmental condition could influence the production of the different fractions of EPS, which makes it difficult, if not impossible, to compare our results with other published data.
Protein is an integral component of cyanobacterial EPS that determines in part the physicochemical properties of these polymers (Kawaguchi & Decho, Citation2000). Uronic acids enhance the stickiness of cyanobacterial EPS which aids, for instance, biofilm or microbial mat formation (Pereira et al., Citation2009; Stal, Citation2012). Glucosaminoglycan (GAG), in combination with uronic acids, contributes to the anionic nature of EPS; GAG and uronic acids bind calcium and magnesium ions forming ionic bridges between EPS molecules and charged minerals in sediments. This property of EPS is particularly important in biofilms and microbial mats as it forms the matrix in which the organisms are embedded, helping them to attach to a surface and stabilize their environment (Kawaguchi & Decho, Citation2000; Gao & Zou, Citation2001). It is therefore not surprising that most (90%) of the cyanobacterial EPS studied thus far contain uronic acid (Pereira et al., Citation2009).
Cyanobacterial EPS are complex molecules (Pereira et al., Citation2009); while many bacteria produce EPS containing less than four sugar moieties, cyanobacterial EPS may be composed of up to 12 monosaccharides (Pereira et al., Citation2009). One speciality of cyanobacterial EPS is the presence of one to three pentose sugars, which differentiate cyanobacterial EPS from other microbial EPS (Sutherland, Citation1994). The composition of EPS of A. platensis is complex (Trabelsi et al., Citation2009). Quantitative analysis of EPS fractions considered in this study, i.e. REPS, LEPS and TEPS, revealed the ratio in which the component monosaccharides were present in the three operational fractions to be different, with seven out of eight tested monosaccharide residues detected in the TEPS fraction. Although a wide range of analytical techniques have been used to study the monosaccharide composition of EPS, including TLC, HPLC, GC and GC-MS, these techniques can only detect four–eight residues in a single run (Saravanan & Jayachandran, Citation2008; Jindal et al., Citation2013; Ohki et al., Citation2014). Glucose is the predominant monosaccharide in the majority of cyanobacterial released polysaccharides (REPS); an exception is the REPS of Anabaena sphaerica which contains galactose as the main sugar (Li et al., Citation2001). The EPS fractions of A. platensis strain MMG-9 were rich in glucose, galactose and rhamnose, particularly in REPS, confirming the findings of Majdoub et al. (Citation2009) who reported that rhamnose made up to 49.7% of the EPS of Arthrospira platensis. Rhamnose and fucose are deoxy sugars that make EPS hydrophobic and contribute significantly to the emulsifying properties of these polysaccharides (Pereira et al., Citation2009). A combination of the hydrophobic and hydrophilic features of cyanobacterial EPS, along with adhesion to substrates, contributes to water storage, thus conferring desiccation resistance (Rossi et al., Citation2012). Fucose is not an unusual component in EPS. It occurs in the glycoconjugates of many microorganisms and is an important component of the cell wall and capsule structures of Gram-negative and Gram-positive bacteria (Maki & Renkonen, Citation2004). The relative proportion of fucose in EPS of Nostoc has been associated with the salt resistance of this cyanobacterium (Yoshimura et al., Citation2012). Xylose has also been reported as an important component of EPS (Filali Mouhim et al., Citation1993; Trabelsi et al., Citation2009), supporting our finding of the large proportion of this sugar in the TEPS of A. platensis (23%), although previous studies of TEPS have not reported the rare sugar ribose as was found in this study.
Confocal laser scanning microscopy provided information with regard to the localization of EPS and supported the view that the different operational EPS fractions could indeed be related to recognizable structures (Ruas-Madiedo & de los Reyes-Gavilan, Citation2005). The use of various fluorescently labelled lectins and fluorescent stains gave insight into the localization of the EPS (Strathmann et al., Citation2002) and auto-fluorescence of the cyanobacteria visualized the cells (Ahmed et al., Citation2011). DTAF stains the EPS of cyanobacteria and plant cells (Ahmed et al., Citation2011) and showed nicely the cross-walls between adjacent cells, which is a typical feature of Arthrospira trichomes (Waterbury, Citation2006). CSA-TRITC, a lectin specific for N-acetylgalactoamine, depicted the galactose richness in Arthrospira EPS. Both DTAF and CSA-TRITC stained the TEPS but to variable degrees; CSA-TRITC stained only the outer EPS covering of the trichomes whereas DTAF stained particularly the regions between two cells with a strong fluorescence (, ). Besides carbohydrates, DTAF shows also affinity for proteins and this may be the reason for the more intense staining of the EPS (Schumann & Rentsch, Citation1998). Alexa is a sensitive dye for protein (Huang et al., Citation2004) and allowed us to detect and locate protein in the EPS. Con-A has affinity for mannose, which is present in TEPS in small amounts but also binds to the glucose in EPS (Strathmann et al., Citation2002). Con-A staining confirmed the presence of mannose in the TEPS as there was no glucose found in this fraction. Some fucose-specific lectins, including Lotus tetragonolobus (Lotus), which shows higher affinity for (α-1,2)-linked fucose residues, have been reported to be less suitable for detection of the sheath or TEPS (Zippel & Neu, Citation2011). Lotus-FITC stains fucose in EPS and it seemed to stain particularly the REPS.
Regardless of the complications involved with the use of multiple fluorochromes, multifluorochrome staining is a valuable technique that allows the detailed investigation of microbial samples and is becoming increasing popular for the study of EPS-producing microbes (Chen et al., Citation2007). Visualization after combined staining resulted in the detection of a thick EPS matrix surrounding the spiral trichome (). The inner core of the spiral trichome seemed to be stained densely suggesting a higher amount of EPS in that region.
EPS that surround microbes are involved in the attachment of cells to a substrate and also in the formation of a matrix in which microbes are embedded (de los Rios et al., Citation2004). In terrestrial cyanobacteria, a thick EPS envelope also serves as a reservoir to hold water in order to avoid desiccation (Yoshimura et al., Citation2012). This matrix of mucilage could enhance the biotechnological importance of Arthrospira as it efficiently traps metal ions and other toxic compounds (Garcia-Meza et al., Citation2005; De Philippis et al., Citation2011). The ability of cyanobacterial EPS to chelate metal ions enables cells to accumulate these trace nutrients for growth and/or to prevent them from direct contact with toxic metals (Pereira et al., Citation2009).
The use of lectins is an elegant method to visualize different types of microbial EPS (Zippel & Neu, Citation2011). Furthermore, the use of optical sectioning with confocal laser scanning microscopy enables 3D imaging of undamaged cyanobacterial trichomes along with their surrounding EPS (Barranguet et al., Citation2004). The thick heterogeneous layer of EPS covering the cyanobacterial trichomes illustrates how these polymeric substances could act as a physical barrier preventing direct contact between the cell and its environment.
Acknowledgements
This research was part of the PhD thesis of Dr Mehboob Ahmed. The Higher Education Commission of Pakistan is acknowledged for providing funding to Mehboob Ahmed (IRSIP No.1-8 ⁄HEC⁄HRD⁄ 2007⁄923) to visit the Netherlands Institute of Ecology (NIOO-KNAW) and use Confocal Laser Scanning Microscopy and analyses of EPS.
References
- Abed, R.M.M., Dobretsov, S. & Sudesh, K. (2009). Applications of cyanobacteria in biotechnology. Journal of Applied Microbiology, 106: 1–12.
- Ahmed, M., Stal, L.J. & Hasnain, S. (2010a). Association of non-heterocystous cyanobacteria with crop plants. Plant and Soil, 336: 363–375.
- Ahmed, M., Stal, L.J. & Hasnain, S. (2010b). Production of indole-3-acetic acid by the cyanobacterium Arthrospira platensis strain MMG-9. Journal of Microbiology and Biotechnology, 20: 1259–1265.
- Ahmed, M., Stal, L.J. & Hasnain, S. (2011). DTAF: an efficient probe to study cyanobacterial–plant interaction using confocal laser scanning microscopy (CLSM). Journal of Industrial Microbiology and Biotechnology, 38: 249–255.
- Barranguet, C., Van Beusekom, S.A.M., Veuger, B., Neu, T.R., Manders, E.M.M., Sinke, J.J. & Admiraal, W. (2004). Studying undisturbed autotrophic biofilms: still a technical challenge. Aquatic Microbial Ecology, 34: 1–9.
- Boschker, H.T.S., Moerdijk-Poortvliet, T.C.W., Van Breugel, P., Houtekamer, M. & Middelburg, J.J. (2008). A versatile method for stable carbon isotope analysis of carbohydrates by high-performance liquid chromatography/isotope ratio mass spectrometry. Rapid Communications in Mass Spectrometry, 22: 3902–3908.
- Chandrasekhar, S., Esterman, M.A. & Hoffman, H.A. (1987). Microdetermination of proteoglycans and glycosaminoglycans in the presence of guanidine hydrochloride. Analytical Biochemistry, 161: 103–108.
- Chen, M.Y., Lee, D.J., Tay, J.H. & Show, K.Y. (2007). Staining of extracellular polymeric substances and cells in bioaggregates. Applied Microbiology and Biotechnology, 75: 467–474.
- Cohen, Z. (1997). The chemicals of Spirulina. In Spirulina platensis (Arthrospira): Physiology, Cell-biology, and Biotechnology (Vonshak, A., editor), 175–204. CRC Press, London.
- de los Rios, A., Ascaso, C., Wierzchos, J., Fernandez-Valiente, E. & Quesada, A. (2004). Microstructural characterization of cyanobacterial mats from the McMurdo Ice Shelf, Antarctica. Applied and Environmental Microbiology, 70: 569–580.
- De Philippis, R., Colica, G. & Micheletti, E. (2011). Exopolysaccharide-producing cyanobacteria in heavy metal removal from water: molecular basis and practical applicability of the biosorption process. Applied Microbiology and Biotechnology, 92: 697–708.
- Dubois, M., Gilles, K.A., Hamilton, J.K., Rebers, P.A. & Smith, F. (1956). Colorimetric method for determination of sugars and related substances. Analytical Chemistry, 28: 350–356.
- Filali Mouhim, R., Cornet, J.F., Fontane, T., Fournet, B. & Dubertret, G. (1993). Production, isolation and preliminary characterization of the exopolysaccharide of the cyanobacterium Spirulina platensis. Biotechnology Letters, 15: 567–572.
- Gao, K. & Zou, D. (2001). Photosynthetic bicarbonate utilization by a terrestrial cyanobacterium, Nostoc flagelliforme (cyanophyceae). Journal of Phycology, 37: 768–771.
- Garcia-Meza, J.V., Barrangue, C. & Admiraal, W. (2005). Biofilm formation by algae as a mechanism for surviving on mine tailings. Environmental Toxicology and Chemistry, 24: 573–581.
- George, E.A. (1976). Culture Centre of Algae and Protozoa. List of Strains 1976, 3rd edition. Cambridge.
- Gloaguen, V., Morvan, H. & Hoffmann, L. (1995). Released and capsular polysaccharides of Oscillatoriaceae (Cyanophyceae, Cyanobacteria). Archiv für Hydrobiologie, 109: 53.
- Huang, S.J., Wang, H.Y., Carroll, C.A., Hayes, S.J., Weintraub, S.T. & Serwer, P. (2004). Analysis of proteins stained by Alexa dyes. Electrophoresis, 25: 779–784.
- Jindal, N., Pal Singh, D. & Singh Khattar, J. (2013). Optimization, characterization, and flow properties of exopolysaccharides produced by the cyanobacterium Lyngbya stagnina. Journal of Basic Microbiology, 53: 902–912.
- Jittawuttipoka, T., Planchon, M., Spalla, O., Benzerara, K., Guyot, F., Cassier-Chauvat, C. & Chauvat, F. (2013). Multidisciplinary evidences that Synechocystis PCC6803 exopolysaccharides operate in cell sedimentation and protection against salt and metal stresses. PloS One, 8: e55564.
- Kawaguchi, T. & Decho, A.W. (2000). Biochemical characterization of cyanobacterial extracellular polymers (EPS) from modern marine stromatolites (Bahamas). Preparative Biochemistry and Biotechnology, 30: 321–330.
- Li, P., Harding, S.E. & Liu, Z. (2001). Cyanobacterial exopolysaccharides: their nature and potential biotechnological applications. Biotechnology and Genetic Engineering Reviews, 18: 375–404.
- Lobner, M., Walsted, A., Larsen, R., Bendtzen, K. & Nielsen, C.H. (2008). Enhancement of human adaptive immune responses by administration of a high-molecular-weight polysaccharide extract from the cyanobacterium Arthrospira platensis. Journal of Medicinal Food, 11: 313–322.
- Majdoub, H., Ben Mansour, M., Chaubet, F., Roudesli, M.S. & Maaroufi, R.M. (2009). Anticoagulant activity of a sulfated polysaccharide from the green alga Arthrospira platensis. Biochimica et Biophysica Acta (BBA) – Bioenergetics, 1790: 1377–1381.
- Maki, M. & Renkonen, R. (2004). Biosynthesis of 6-deoxyhexose glycans in bacteria. Glycobiology, 14: 1R–15R.
- Nicolaus, B., Panico, A., Lama, L., Romano, I., Manca, M.C., De Giulio, A. & Gambacorta, A. (1999). Chemical composition and production of exopolysaccharides from representative members of heterocystous and non-heterocystous cyanobacteria. Phytochemistry, 52: 639–647.
- Ohki, K., Le, N., Yoshikawa, S., Kanesaki, Y., Okajima, M., Kaneko, T. & Thi, T. (2014). Exopolysaccharide production by a unicellular freshwater cyanobacterium Cyanothece sp. isolated from a rice field in Vietnam. Journal of Applied Phycology, 26: 265–272.
- Palmer, J., Flint, S. & Brooks, J. (2007). Bacterial cell attachment, the beginning of a biofilm. Journal of Industrial Microbiology and Biotechnology, 34: 577–588.
- Parages, M.L., Rico, R.M., Abdala-Diaz, R.T., Chabrillon, M., Sotiroudis, T.G. & Jimenez, C. (2012). Acidic polysaccharides of Arthrospira (Spirulina) platensis induce the synthesis of TNF-alpha in RAW macrophages. Journal of Applied Phycology, 24: 1537–1546.
- Pereira, S., Zille, A., Micheletti, E., Moradas-Ferreira, P., De Philippis, R. & Tamagnini, P. (2009). Complexity of cyanobacterial exopolysaccharides: composition, structures, inducing factors and putative genes involved in their biosynthesis and assembly. FEMS Microbiology Reviews, 33: 917–941.
- Richert, L., Golubic, S., Le Guedes, R., Ratiskol, J., Payri, C. & Guezennec, J. (2005). Characterization of exopolysaccharides produced by cyanobacteria isolated from polynesian microbial mats. Current Microbiology, 51: 379–384.
- Rossi, F., Micheletti, E., Bruno, L., Adhikary, S.P., Albertano, P. & De Philippis, R. (2012). Characteristics and role of the exocellular polysaccharides produced by five cyanobacteria isolated from phototrophic biofilms growing on stone monuments. Biofouling, 28: 215–224.
- Rossi, N., Petit, I., Jaouen, P., Legentilhomme, P. & Derouiniot, M. (2005). Harvesting of cyanobacterium Arthrospira platensis using inorganic filtration membranes. Separation Science and Technology, 40: 3033–3050.
- Ruas-Madiedo, P. & De los Reyes-Gavilan, C.G. (2005). Methods for the screening, isolation, and characterization of exopolysaccharides produced by lactic acid bacteria. Journal of Dairy Science, 88: 843–856.
- Saravanan, P. & Jayachandran, S. (2008). Preliminary characterization of exopolysaccharides produced by a marine biofilm-forming bacterium Pseudoalteromonas ruthenica (SBT 033). Letters in Applied Microbiology, 46: 1–6.
- Schumann, R. & Rentsch, D. (1998). Staining particulate organic matter with DTAF-a fluorescence dye for carbohydrates and protein: a new approach and application of a 2D image analysis system. Marine Ecology Progress Series, 163: 77–88.
- Staats, N., Stal, L.J. & Mur, L.R. (2000). Exopolysaccharide production by the epipelic diatom Cylindrotheca closterium: effects of nutrient conditions. Journal of Experimental Marine Biology and Ecology, 249: 13–27.
- Stal, L.J. (2012). Cyanobacterial mats and stromatolites. In Ecology of Cyanobacteria II: Their Diversity in Time and Space (Whitton, B.A., editor), 65–125. Springer, Dordrecht.
- Strathmann, M., Wingender, J. & Flemming, H.C. (2002). Application of fluorescently labelled lectins for the visualization and biochemical characterization of polysaccharides in biofilms of Pseudomonas aeruginosa. Journal of Microbiological Methods, 50: 237–248.
- Sutherland, I.W. (1994). Structure-function-relationships in microbial exopolysaccharides. Biotechnology Advances, 12: 393–448.
- Tandeau de Marsac, N. & Houmard, J. (1988). Complementary chromatic adaptation: physiological conditions and action spectra. Methods in Enzymology, 167: 318–328.
- Taylor, K.A. & Buchanan-Smith, J.G. (1992). A colorimetric method for the quantitation of uronic acids and a specific assay for galacturonic acid. Analytical Biochemistry, 201: 190–196.
- Trabelsi, L., M’sakni, N., Ouada, H., Bacha, H. & Roudesli, S. (2009). Partial characterization of extracellular polysaccharides produced by cyanobacterium Arthrospira platensis. Biotechnology and Bioprocess Engineering, 14: 27–31.
- Waterbury, J. (2006). The cyanobacteria—isolation, purification and identification. In The Prokaryotes (Dworkin, M., Falkow, S., Rosenberg, E., Schleifer, K.H. & Stackebrandt, E., editors), 1053–1073. Springer, Dordrecht.
- Yoshimura, H., Kotake, T., Aohara, T., Tsumuraya, Y., Ikeuchi, M. & Ohmori, M. (2012). The role of extracellular polysaccharides produced by the terrestrial cyanobacterium Nostoc sp. strain HK-01 in NaCl tolerance. Journal of Applied Phycology, 24: 237–243.
- Zippel, B. & Neu, T.R. (2011). Characterization of glycoconjugates of extracellular polymeric substances in tufa-associated biofilms by using fluorescence lectin-binding analysis. Applied and Environmental Microbiology, 77: 505–516.