Abstract
Based on physiological characteristics, we hypothesized that different strains of Gracilaria birdiae from two distinct geographical areas of the Brazilian coast (2500 km apart) would have different responses to long-term exposure to UV-B radiation (UV-B). The locations differ in their environmental conditions: one is a warmer area, Ceará State (CE), closer to the equator; the other is a colder area, Espirito Santo State (ES), closer to the Tropic of Capricorn. To test the hypothesis that the CE population is more resistant to UV-B than the ES population, apical segments of the red (RDCE, RDES), green (GRCE) and greenish-brown (GBCE) strains were cultivated in the laboratory under two treatments: control (PAR) and artificial UV-B (PAR + UV-B). Algal performance was evaluated by considering growth rates, pigment content and ultrastructural analysis. Compared with the control, all strains showed a decrease in growth rates after exposure to UV-B. Of all strains, RDES showed the greatest sensitivity to UV-B. However, a decrease in growth rate and morphological changes were observed to a lesser extent in the RDCE strain. Moreover, exposure to UV-B resulted in a decrease in the concentrations of phycobiliproteins in the RDCE strain. The GBCE strain showed an increase in phycoerythrin (PE)/allophycocyanin (APC) and phycocyanin (PC)/allophycocyanin (APC) ratios after exposure to UV-B, suggesting this strain had a higher tolerance to the radiation. No differences in the chlorophyll a and carotenoid content were found between the control and UV-B treated samples for all strains. Ultrastructural changes, such as damage to chloroplasts and mitochondria, were present in all strains after exposure to UV-B. In summary, our findings support the hypothesis that the population from Ceará State has adapted to the higher irradiation and is thus more resistant to increased UV-B. Additionally, of the strains tested, the GBCE and RDCE strains appear to be more resistant to this radiation.
Introduction
The deleterious effects of UV-B on photosynthetic organisms result from a combination of damage and repair, which may have consequences for growth, primary production, cell biology and ultrastructure (Franklin & Foster, Citation1997; Buma et al., Citation2003; Xue et al., Citation2005; Bischof et al., Citation2006; Barufi et al., Citation2011; Harley et al., Citation2012; Schemer et al., Citation2012). Intertidal macroalgae, being restricted to their place of growth, are exposed for extended periods during low tide and are therefore susceptible to the effects of enhanced UV-B (Cabrera et al., Citation1995; Altamirano et al., Citation2000). However, these organisms are also capable of optimizing their photosynthetic response to a given light regime and maintaining metabolic and enzymatic activity at a wide range of temperatures (Henley & Ramus, Citation1989). Furthermore, most of these algae have defence mechanisms that minimize the damage caused by UV-B (Fiscus & Booker, Citation1995; Franklin & Foster, Citation1997). Nonetheless, intertidal algae can still exhibit a variety of damaging responses when exposed to UV-B (Xue et al., Citation2005; Häder et al., Citation2011; Harley et al., Citation2012).
Growth is the best parameter to explain the ecophysiological characteristics of an organism (Roleda et al., Citation2004a). The combined effects of damage to essential molecules, such as proteins, pigments and DNA, will affect important cellular processes, such as nutrient uptake, photosynthesis and DNA transcription or replication (Buma et al., Citation2003), which, in turn, will result in a decrease in growth rate and reproduction (Franklin & Foster, Citation1997; Häder et al., Citation1998). These processes are directly affected by ultraviolet radiation, and the ability to repair or prevent damage determines the tolerance of the species to this radiation (van de Poll et al., Citation2001). In addition to physiological responses, there are also functional and ultrastructural changes in cells and organelles exposed to various forms and intensities of ultraviolet radiation (Somozy, Citation2000). Among the various organelles, chloroplasts seem to be the most sensitive to UV-B (Talarico & Maranzana, Citation2000). Chloroplasts of damaged cells lose their structural integrity (Navarro et al., Citation2010a) and the ordered pattern of both stromal grana and thylakoids disappears, with the latter becoming slightly dilated (Hollósy, Citation2002; Poppe et al., Citation2002, 2003; Holzinger & Lutz, 2006).
Photosynthetic pigments have different sensitivities when subjected to high levels of UV: phycobiliproteins are more susceptible than chlorophylls and carotenoids (Bischof et al., Citation2006). Generally, there is a decrease in phycobiliproteins when seaweeds are exposed to UV (Xu & Gao, Citation2007; Eswaran et al., Citation2001; Navarro et al., Citation2010a) and an increase in the concentration of chlorophyll a when exposure is prolonged (Kräbs & Wiencke, Citation2005; Navarro et al., Citation2010a; Barufi et al., Citation2011; Schemer et al., Citation2012).
Increasing levels of UVB radiation reaching the earth’s surface have been reported since the late 1980s. Recent research has predicted a decrease in the cloud cover at low latitudes (< ~ 30°), which should result in increases of between 3 and 6% of incident UV-B (McKenzie et al., Citation2011; Watanabe et al., Citation2011; Zepp et al., Citation2011), changes that could affect the vertical zonation, composition and diversity of macroalgal populations (Bischof et al., Citation2006; Häder et al., Citation2011; Harley et al., Citation2012).
Gracilaria birdiae, an economically important agarophyte, is the main source of agar in Brazil (Plastino & Oliveira, Citation2002). As the alga has a wide distribution along the intertidal Brazilian coast, populations occur at different latitudes and are exposed to distinctly different light, temperature and nutrient conditions. Besides the red wild type, some coloured strains have been found in natural populations or generated spontaneously in the laboratory (Plastino et al., Citation2004; Costa & Plastino, Citation2011). The red wild type and strains from populations closer to the equator have acquired adaptations to higher light intensities and higher water temperature, when compared with strains from southwest populations (Ursi et al., Citation2003, 2013). However, the effects of UV-B on this species and its coloured strains remain largely unknown. Based on previous results (Ursi et al., Citation2003, 2013), we can hypothesize that strains from populations closer to the equator may be more resistant to UV-B than strains from the southwest populations. Therefore, the aim of this study was to evaluate the effects of long-term exposure (28 days) to artificial UV-B on strains of G. birdiae from two distinct geographical areas of the Brazilian coast 2500 km apart, one warmer area closer to the equator and another colder area closer to the Tropic of Capricorn. Growth rates, pigment content and ultrastructure were evaluated.
Materials and methods
Biological material
Four strains of Gracilaria birdiae (in vitro unialgal and non-axenic cultures) obtained from the Gracilariaceae Germplasm Bank of the University of São Paulo (Costa et al., 2012) were selected for the experiments as follows. Apical branches of female gametophytes of the red (wild-type, RDCE) and greenish-brown (GBCE) strains were derived from algae collected in March 1994 from Paracuru Beach (03.40 °S 39.07 °W), Ceará State (CE), Brazil (Costa & Plastino, Citation2011); the red wild-type (RDES) strain was derived from algae collected in August 1994 from Anchieta Beach (20.80 °S 40.65 °W), Espírito Santo State (ES), Brazil (Plastino et al., Citation2004); and the green (GRCE) strain was established from tetraspores released by green apical segments that had spontaneously arisen on a red tetrasporophyte obtained in the laboratory in June 1997 (Costa & Plastino, Citation2011). Voucher specimens have been deposited in the herbarium of the Instituto de Biociências, at the University of São Paulo (SPF 56492–56945).
General culture conditions
Strains were maintained in sterile seawater (32 psu salinity, refractometer American Optical 10419, Leica, Wetzlar, Germany), enriched with 12.5% von Stosch solution (Ursi & Plastino, Citation2001), which was prepared without nitrate. The nitrate was added at a concentration of 0.250 mM (Costa, Citation2005). The algae were kept in a controlled environment at 25 ± 1°C with a photoperiod of 14 h. The photosynthetically active radiation (PAR) was 70 ± 10 µmol m–2 s–1 provided by Osram 40 W ‘Daylight’ fluorescent tubes (Osram, Berlin, Germany) and measured by a quantameter (Li-COR model L1-185, Lincoln, Nebraska, USA). Cultures were aerated for 30 min h–1 (radial compressor Ibram CR3, São Lourenço, Brazil), and the medium was renewed weekly.
Ultraviolet B radiation (UV-B)
The UV-B was provided by UVB Broadband lamps (20 W/12 RS, Philips TL, Amsterdam, the Netherlands) and measured by a radiometer (Vilber-Lourmat VLX – 3 W no. 2218, Eberhardzell, Germany). The UVB Broadband lamps emit radiation in the ‘B’ bandwidth of the UV spectrum (290–315 nm), with the peak at 302 nm. The light source was positioned above the shelves where the culture flasks were deposited. At intervals of 7 days, flasks were randomly repositioned so that all apices would receive the same irradiation during the experiment. The experimental space inside the culture room was isolated by layers of polyethylene HD, which prevented the ultraviolet radiation from dissipating into the environment. Putative ultraviolet-C radiation was filtered with cellulose diacetate foil (0.075 mm thick, Ultraphan URT, Digefra, Munich, Germany), which displayed 0% transmission below 286 nm. In order to prevent their degradation, these filters were replaced every 45 h (Adamse & Britz, Citation1992).
Two treatments were performed (control and UV-B). The control treatment (PAR) was similar to general culture conditions. In the UV-B treatment (0.08 W m–2) strains were exposed to UV-B for 3 h per day in the middle of the light period. Neither of the treatments was aerated. Experiments were conducted for a period of 28 days. Three replicates per treatment were made for each coloured strain (RDCE, RDES, GRCE and GBCE).
Growth rates
One week before the beginning of the experiment (pre-treatment), 30 apical fragments of each strain (RDCE, RDES, GRCE and GBCE), each about 2 cm in length, were cultivated in six polyethylene flasks containing 500 ml of enriched seawater (about 50 mg of algae per flask, 5 apical segments), in the absence of UV-B. After that, the strains were cultivated in triplicate in each experimental condition for growth rate determination (control and UV-B). Growth was assessed weekly by measures of mass, using an A 200 Mettler analytical balance (Mettler Toledo, Columbus, USA), whereas the morphology of gametophytes was recorded at the beginning of the experiment and after 4 weeks (SONY Cyber-shot 8.1 megapixels). Growth rates were estimated by means of the following equation: GR= [(Mf/Mi) ^ (1/t) – 1] × 100%, where GR = growth rate, Mf = final mass, Mi = initial mass and t = time (Lignell & Pedersén, Citation1989).
Pigment analyses
Phycobiliproteins, chlorophyll a and carotenoids were quantified in all strains cultivated in the control and UV-B conditions at the end of the experiment (after 4 weeks). Pigment extractions were carried out at 4°C, according to Kursar et al. (Citation1983a, Citation1983b) with modifications (Plastino & Guimarães, Citation2001). Briefly, 200 mg of apical segments from each replicate (N = 3) were disrupted by grinding with liquid nitrogen and 50 mM phosphate buffer (pH 5.5). Crude extracts were centrifuged at 44 000 × g for 20 min. The supernatant containing the phycobiliproteins (phycoerythrin (PE), phycocyanin (PC) and allophycocyanin (APC)) was removed and kept in sealed vials at 4°C until a reading was taken using a spectrophotometer (HP 8452A, Palo Alto, California, USA). The extraction of chlorophyll a and carotenoids was carried out on samples of 40 mg fresh weight for each replicate (N = 3). Briefly, 2 ml of N, N-dimethylformamide (DMF 99.8%) was added to each sample and the samples were kept at 4°C in the dark for 12 h. Crude extracts were centrifuged at 19 000 × g for 20 min. The supernatant containing chlorophyll a and carotenoids was transferred to test tubes, which remained sealed in the dark at 4°C until spectrophotometry was carried out (Wellburn, Citation1994). We used the equation described by Inskeep & Bloom (Citation1985) to determine the concentration of chlorophyll a, and the equation described by Wellburn (Citation1994) to determine the concentration of total carotenoids.
Transmission electron microscopy
Apical segments 4 mm long from strains cultivated in control and UV-B conditions for four weeks were fixed in 6% glutaraldehyde, 0.1 M cacodylate buffer and 0.2 M sucrose (pH 7.0) for 12 h at 4ºC (Plastino & Costa, Citation1999). After three washes in the same buffer with decreasing concentrations of sucrose (10 min each), the samples were post-fixed in 1% osmium tetroxide (OsO4) for four hours at 4ºC. Afterwards, the samples were washed twice in fresh (0.1 M) cacodylate buffer and twice in (0.1 M) saline solution (NaCl), then maintained in (0.1 M) NaCl for 12 h at 4ºC. Dehydration was carried out in a graded acetone series before infiltration using Spurr’s medium (Plastino & Costa, Citation1999) (Low viscosity embedding media Spurr’s kit, Electron Microscopy Sciences cat. no. 14300, Hatfield, Pennsylvania, USA). Thin sections were stained with 2% uranyl acetate and lead citrate (Reynolds, Citation1963). The samples were observed under a transmission electron microscope (Zeiss EM900, Jena, Germany).
Statistical analyses
Time series measurements of the growth rates were subjected to repeated measures analysis of variance (RMANOVA), and different pigment concentrations were subjected to unifactorial analyses of variance (ANOVA) to determine the effects of treatments across the sampling days. In all cases, an a posteriori Newman–Keuls test was used to establish statistical differences. Statistical analyses were done using the Statistica 7 program (Statsoft, Tulsa, Oklahoma, USA, http://www.statsoft.com), considering P < 0.05.
Results
Growth rates
After a week of exposure to UV-B, the RDES strain of Gracilaria birdiae showed significant curling of the apical fragments and changes in colouration, acquiring a brownish hue. Apical segments of the other strains cultivated in UV-B showed similar changes, but only after the second week of exposure. At that time, segments of the RDES strain showed signs of necrosis and acquired a coiled appearance. These characteristics were more evident after 28 days of cultivation ().
Fig. 1. Apical segments of Gracilaria birdiae from the red (RDCE and RDES), green (GRCE) and greenish-brown (GBCE) strains, after 28 days of exposure to: photosynthetically active radiation, PAR (control); and PAR+UV-B (0.08 W m–2). A, RDCE. B, RDES. C, GRCE. D, GBCE. Scale = 1 cm.
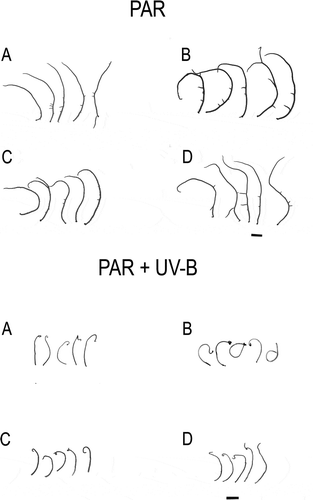
All strains cultivated in PAR+UV-B showed lower GRs than strains cultivated in PAR (F = 298.06; P < 0.01) (). However, when considering each of the test conditions separately, growth rates (GRs) were similar among the different strains (RDCE, RDES, GRCE and GBCE): PAR (F = 4.34; P = 0.95) and PAR+UV-B (F = 4.34; P = 0.98).
Fig. 2. Relative growth rates from red (RDCE and RDES), green (GRCE) and greenish-brown (GBCE) strains of Gracilaria birdiae cultivated in different conditions showing all data collected over 28 days. Samples exposed to: photosynthetically active radiation, PAR (control), P; and PAR+UV-B (0.08 W m–2), PB. Data presented as mean ± SD (N = 3). Treatments with different letters indicate significant differences according to one-way ANOVA and Newman–Keuls test (P <0.05).
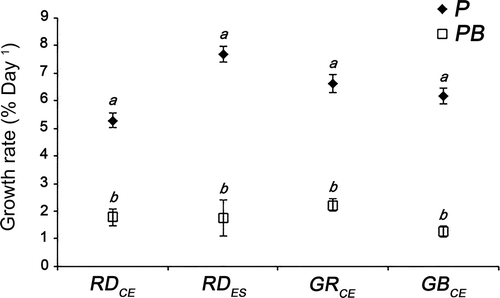
Analysis of the GRs from all dates on which data were collected (four occasions from days 7–28) showed that all strains exposed to PAR+UV-B showed a decrease in GR by the second week (F = 136.42; P = 0.00), whereas in control conditions, the GRs remained constant over time (GRCE, P > 0.11) or decreased only in the third (GBCE, P = 0.00) or fourth week (RDCE, P = 0.01) (). However, the RDES strain showed a decrease of GR for both PAR and PAR+UV-B from the second week (F = 136.42; P = 0.00).
Fig. 3. Growth rates of strains of Gracilaria birdiae calculated from the average of fresh mass (mg) obtained every week for 28 days. Samples exposed to: photosynthetically active radiation, PAR (control), P; and PAR+UV-B (0.08 W m–2), PB. Data presented as mean ± SD (N = 3). Treatments with different letters indicate significant differences according to one-way ANOVA and Newman–Keuls test (P < 0.05).
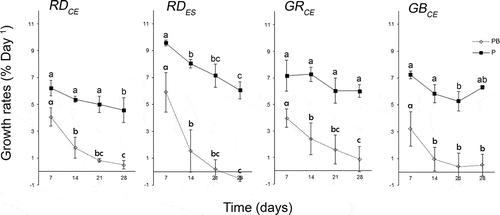
Phycobiliproteins
An analysis comparing each strain of G. birdiae under both experimental conditions (control and UV-B) showed that the RDCE strain presented lower concentrations of APC (F = 4.96; P = 0.02), PC (F = 0.00; P = 0.04) and PE (F = 0.92; P = 0.01) after exposure to UV-B (). However, the RDES strain had similar concentrations of APC (F = 4.96; P = 0.39), PC (F = 0.00; P = 0.80) and PE (F = 0.92; P = 0.72) under both conditions. No differences were found between control and UV-B for the GRCE [APC (F = 4.96; P = 0.97), PC (F = 0.00; P = 0.53), and PE (F = 0.92; P = 0.72)] and GBCE strains [APC (F = 4.96; P = 0.95), PC (F = 0.00; P = 0.64), and PE (F = 0.92; P = 0.31)].
Fig. 4. Phycobiliprotein concentrations of four strains of Gracilaria birdiae. Data were obtained from algae cultivated in different conditions: photosynthetically active radiation, PAR (control), P and PAR+UV-B (0.08 W m–2), PB. APC, allophycocyanin; PC, phycocyanin; PE, phycoerythrin. Data presented as mean ± SD (N = 3). Treatments with different letters indicate significant differences according to one-way ANOVA and Newman–Keuls test (P < 0.05).
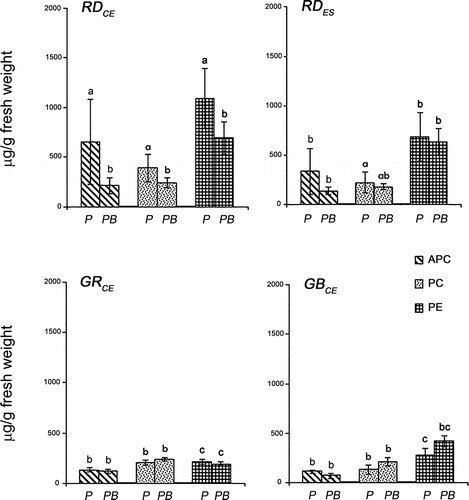
Considering the different strains cultivated under control conditions, higher concentrations of allophycocyanin (APC) (F = 4.80; P < 0.04) and phycoerythrin (PE) (F = 22.41; P < 0.02) were found for the RDCE strain when compared with the other strains (). The RDCE strain also presented the highest concentration of phycocyanin (PC) when compared with the GRCE (F = 3.89; P = 0.02) and GBCE strains (F = 3.89; P = 0.02), but these concentrations were similar to those observed for the RDES strain (F = 3.89; P = 0.86). High concentrations of PE were also observed for the RDES strain when compared with the GRCE (F = 22.41; P = 0.01) and GBCE strains (F = 22.41; P = 0.04). The GRCE strain had similar concentrations of APC (F = 4.80; P = 0.09), PC (F = 3.89; P = 0.95), and PE (F = 22.41; P = 0.59) when compared with the GBCE strain.
Considering the different strains exposed to PAR+UV-B, low concentrations of PE were verified for the GRCE strain when compared with the RDCE (F = 22.41; P = 0.02) and RDES strains (F = 22.41; P = 0.02) (). However, these concentrations were similar to those found in the GBCE strain (F = 22.41; P = 0.25). No differences were observed for concentrations of APC (F = 4.80; P > 0.62) and PC (F = 3.89; P > 0.86) among all strains, when exposed to UV-B.
An analysis comparing the control and UV-B conditions for the GBCE strain showed higher values for the PE/APC (F = 19.93; P = 0.00) and PC/APC (F = 23.21; P < 0.01) ratios after exposure to UV-B, whereas for the PE/PC ratio (F = 0.25; P = 0.59), no differences were observed (). The RDCE, RDES and GRCE strains showed similar concentrations of PE/PC (F = 0.25; P > 0.21), PE/APC (F = 19.93; P > 0.10) and PC/APC (F = 23.21; P > 0.19) in both conditions.
Fig. 5. Ratios of phycobiliproteins in four strains of Gracilaria birdiae. Data were obtained from algae cultivated in different conditions: photosynthetically active radiation, PAR (control), P; and PAR+UV-B (0.08 W m–2), PB. (PE/PC, phycoerythrin/phycocyanin; PE/APC, phycoerythrin/allophycocyanin; PC/APC, phycocyanin/allophycocyanin. Data presented as mean ± SD (N = 3). Treatments with different letters indicate significant differences according to one-way ANOVA and Newman–Keuls test (P < 0.05).
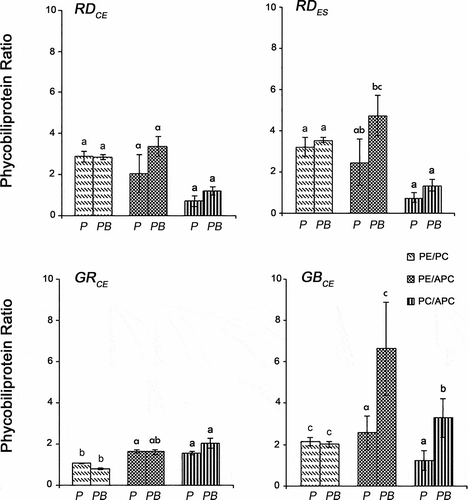
Chlorophyll a and carotenoids
No difference in chlorophyll a (Chl a) content was found between control and UV-B for all strains of G. birdiae (F = 0.14, P > 0.70). Similar concentrations of Chl a (F = 2.97, P > 0.06) were also observed among the different strains for both the PAR and PAR + UV-B conditions ().
Fig. 6. Chlorophyll a (Chl a) and carotenoid (Car) concentrations of four strains of G birdiae. Data were obtained from algae cultivated in different conditions: photosynthetically active radiation, PAR (control), P; and PAR+UV-B (0.08 Wm-2), PB. Data presented as mean ± SD (N = 3). Treatments with different letters indicate significant differences according to one-way ANOVA and Newman–Keuls test (P < 0.05).
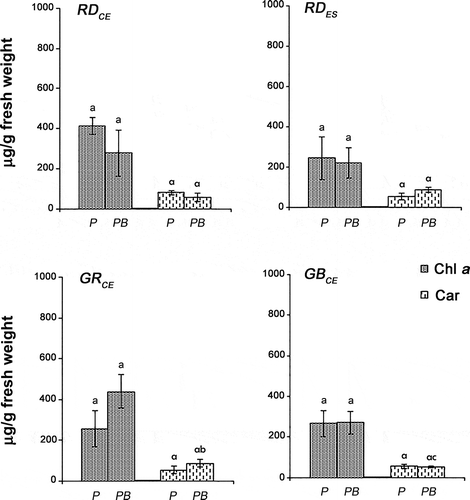
Comparing the different strains, similar concentrations of carotenoids (Car) were observed in the control (F = 3.55, P > 0.19), while for the PAR + UV-B condition, higher concentrations were observed for the GRCE strain when compared with the GBCE strain (F = 3.55, P = 0.04) (). However, the values of GRCE and GBCE were similar to the carotenoid content found for the RDCE (F = 3.55, P > 0.16) and RDES (F = 3.55, P > 0.18) strains. The RDCE and RDES strains showed similar values for carotenoids when exposed to UV-B (F = 3.55, P > 0.04). Considering each of the strains, similar concentrations of carotenoids (F = 0.04, P > 0.83) were found in PAR and PAR + UV-B.
Comparing the different strains in both experimental conditions, similar results were found for APC/Chl a (F = 4.46, P > 0.83) and PC/Chl a (F = 1.55, P > 0.97) ratios (). The RDCE strain showed a higher PE/Chl a ratio when compared with the GRCE (F = 7.21, P = 0.03) and GBCE (F = 7.21, P = 0.03) strains in the control. The RDES strain also showed a higher PE/Chl a ratio when compared with the GRCE (F = 7.21, P = 0.02) and GBCE (F = 7.21, P = 0.03) strains in the control, although these values were similar to those found for the RDCE (F = 7.21, P = 0.46) strain. No differences were found among the strains for the PE/Chl a ratio in the PAR + UV-B (F = 7.01, P > 0.98) condition.
Fig. 7. Phycobiliproteins/chlorophyll a ratio in four strains of G. birdiae. Data were obtained from algae cultivated in different conditions: photosynthetically active radiation, PAR (control), P; and PAR+UV-B (0.08 W m–2), PB. APC/Chl a, allophycocyanin/chlorophyll a; PC/Chl a, phycocyanin/chlorophyll a; PE/Chl a, phycoerythrin/chlorophyll a. Data presented as mean ± SD (N = 3). Treatments with different letters indicate significant differences according to one-way ANOVA and Newman–Keuls test (P < 0.05).
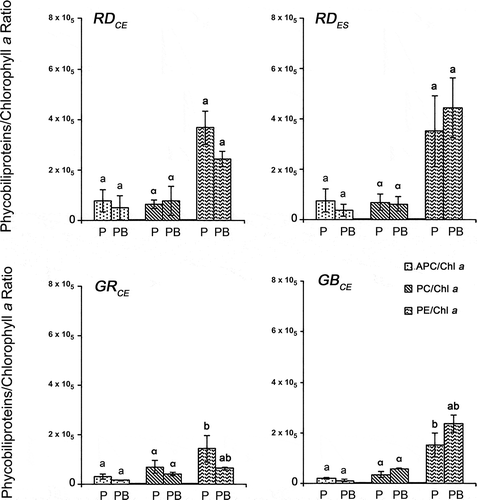
When comparing the carotenoids/chlorophyll a ratio, similarities were observed among the different strains, for both PAR (F = 1.58, P > 0.99) and PAR + UV-B (F = 1.58, P > 0.98) conditions (). Considering each strain, no differences were observed in both tested conditions (F = 0.38, P > 0.20).
Fig. 8. Carotenoid/chlorophyll a ratio of four strains of G birdiae. Data were obtained from algae cultivated in different conditions: photosynthetically active radiation, PAR (control), P; and PAR+UV-B (0.08 W m–2), PB. Data presented as mean ± SD (N = 3). Treatments with different letters indicate significant differences according to one-way ANOVA and Newman–Keuls test (P < 0.05).
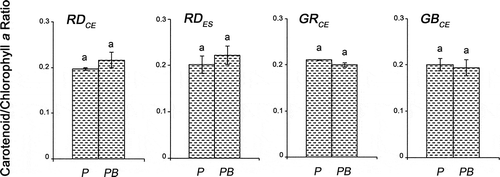
The proportions of the three groups of photosynthetic pigments (phycobiliproteins, chlorophyll a and carotenoids) showed changes after 28 days of exposure to UV-B when compared with the control. However, the similarities between the RDCE and RDES strains remained (). Phycobiliproteins were the most abundant group of pigments in both experimental conditions, with percentages ranging from 51.14% (GRCE) to 81.19% (RDCE) of total pigments. Chlorophyll a was present at between 15.71% (RDCE) and 40.70% (GRCE), and carotenoids at between 3.09% (RDCE) and 8.14% (GRCE) in the control. Higher percentages of Chl a and total carotenoids (Car) were observed for all strains after prolonged exposure to UV-B, except for the GBCE strain, whose values showed a decrease of 11.91% (Chl a) and 2.49% (Car) when compared with the control. Higher percentages of phycobiliproteins were also observed, with the exception of allophycocyanin (APC), whose values were lower for all strains after exposure to UV-B when compared with the control.
Fig. 9. Percentage of photosynthetic pigments present in four strains of G. birdiae. Data were obtained from algae cultivated in different conditions: photosynthetically active radiation, PAR (control), P; and PAR+UV-B (0.08 W m–2), PB. Car, carotenoids; Chl a, chlorophyll a; APC, allophycocyanin; PC, phycocyanin; PE, phycoerythrin.
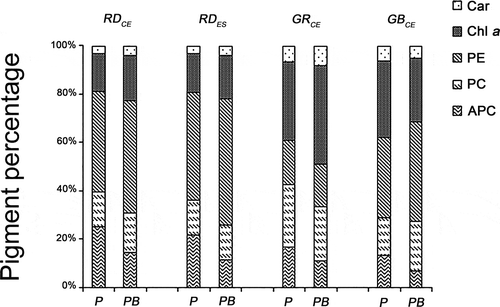
Transmission electron microscopy
Specimens of Gracilaria birdiae from the red (RDCE and RDES), green and greenish-brown strains presented similar characteristics when cultivated in control conditions (, , , ). The cell wall consisted of microfibrils immersed in an electron-transparent amorphous matrix (). Cytoplasmic connections (‘pit-connections’) between the adjacent cells were commonly observed. Pit plugs presented a central structure (plug core) which was surrounded by a membranous layer with no additional associated membrane. Cortex cells contained dense and compact cytoplasm with a large number of chloroplasts and no obvious vacuoles (). In contrast, medullary cells had a large central vacuole and other organelles arranged peripherally, adjacent to the plasma membrane. Chloroplasts contained a single peripheral thylakoid, while the other thylakoids were evenly spaced across the organelle (, , ). Plastoglobuli were observed between adjacent thylakoids (, ). Electron-transparent regions, also called fibrillar regions or genophores (0.16 ± 0.05 µm), were observed in the central region of chloroplasts (, , ). Other organelles, such as Golgi bodies () and mitochondria (, ), were scattered in the cytoplasm. Starch grains were also observed (, ). Nuclei with dense contents were observed in both cortical and medullary cells (, ).
Figs 10–15. Transmission electron microscopy of the RDCE strain of Gracilaria birdiae. Figs 10, 11. Individuals exposed to PAR (control). Fig. 10. Nucleus surrounded by double membrane (arrow) and chloroplast with a single peripheral thylakoid (arrowhead); Fig. 11. Chloroplast with genophore (arrows). Figs 12–15. Individuals exposed to PAR+UV-B (0.08 W m–2) during 28 days (3 hours per day): cortical cells () and medullary cell (). Fig. 12. Disorganized cellular content; Fig. 13. Physodes in the cytoplasm; Fig. 14. Chloroplasts with separated thylakoids (arrows). Fig. 15. Chloroplast with separated thylakoids (arrows) associated with mitochondria with disintegrated cristae (arrowhead). Chloroplast (cl), Golgi bodies (c), physodes (ce), cell wall (PC), mitochondria (m), nucleus (n) and plastoglobuli (pg). Scale bars = 0.5 µm (); 2 µm ().
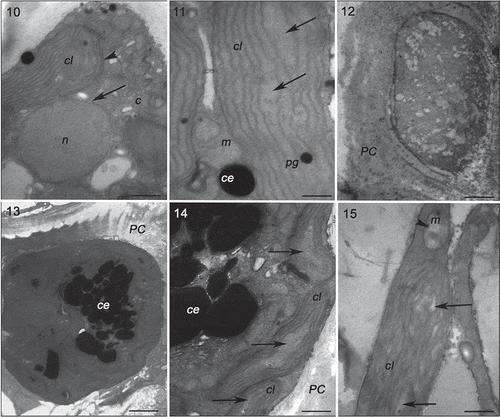
Figs 16–21. Transmission electron microscopy of the RDES strain of Gracilaria birdiae. Figs 16–18. Individuals exposed to PAR (control). Fig. 16. Cortical cell: chloroplasts arranged around the nucleus. Fig. 17. Chloroplast with genophore (arrow). Fig. 18. Detail of mitochondria. Figs 19–21. Cortical cells of individuals exposed to PAR+UV-B (0.08 W m–2) during 28 days (3 h per day). Fig. 19. Starch grains scattered on the cytoplasm. Fig. 20. Mitochondria with disintegrated cristae (arrows). Fig. 21. Cell wall-producing vesicles (arrowheads) and chloroplast with separated thylakoids (arrow). Chloroplast (cl), starch grain (a), mitochondria (m), nucleus (n), cell wall (PC) and plastoglobuli (pg). Scale bars = 0.5 µm (); 0.2 µm (); 2 µm ().
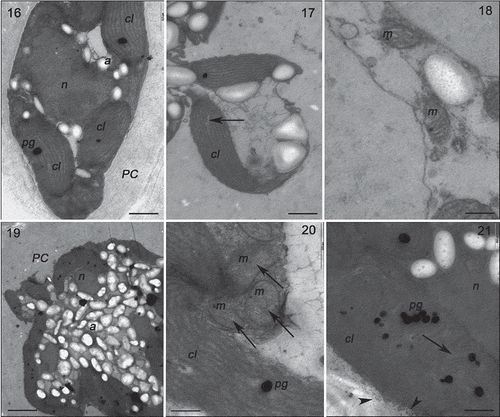
Figs 22–26. Transmission electron microscopy of the GRCE strain of Gracilaria birdiae. Figs 22–23. Individuals exposed to PAR (control). Fig. 22. Cortical cell: chloroplasts with genophore (arrows). Fig. 23. Medullary cells: chloroplasts with starch grains adjacent to the cell wall. Figs 24–26. Individuals exposed to PAR+UV-B (0.08 W m–2) during 28 days (3 h a day). Fig. 24. Cortical cell: Starch grains scattered on the cytoplasm. Fig. 25. Medullary cell: with irregular contours showing vesicle formation (arrowhead), organelle disorganization and separation of thylakoids in chloroplasts (arrows). Fig. 26. Chloroplast with separated thylakoids (arrow) and cell wall producing vesicles (arrowheads). Chloroplast (cl), cell wall (PC), plastoglobuli (pg), starch grains (a) and vacuole (v). Scale bars = 2 µm (); 0.5 µm ().
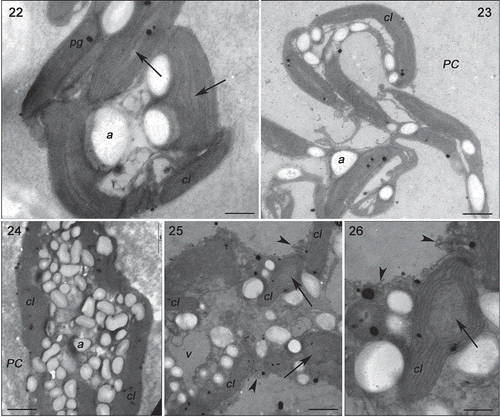
Figs 27–31. Transmission electron microscopy of the GBCE strain of Gracilaria birdiae. Figs 27–29. Individuals exposed to PAR (control). Fig. 27. Chloroplasts of cortical cell with genophore (arrows). Fig. 28. Medullary cells: note nuclear envelope (arrow). Fig. 29. Physodes in the cytoplasm of cortical cell. Figs 30–31. Medullary cells of individuals exposed to PAR+UV-B (0.08 W m–2) during 28 days (3 h per day). Fig. 30. Chloroplasts with separated thylakoids (arrows) and irregular contours (arrowhead). Fig. 31. Chloroplast with separated thylakoid (arrow) and cell wall-producing vesicles (arrowhead). Physodes (ce), chloroplast (cl), mitochondria (m), nucleus (n) and plastoglobuli (pg). Scale bars = 0.5 µm (); 2 µm (); 0.2 µm (Fig. 31).
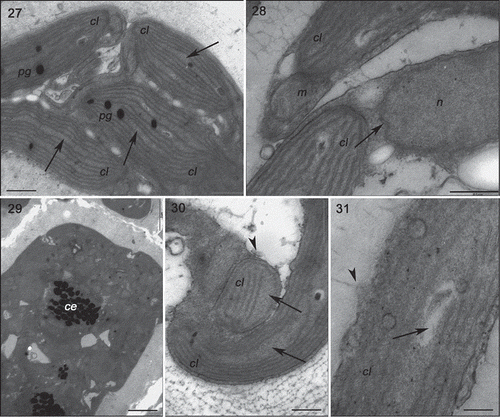
Strains grown in UV-B (0.08 Wm−2) for a period of 28 days showed ultrastructural changes (, , , ). The RDCE strain showed a total disorganization of some cortical cells, specifically the most superficial ones directly exposed to the radiation (), where no internal structures or organelles could be distinguished. All strains showed cortical cells with irregular contours and a great number of cell wall-producing vesicles (, , ). In these cells, there were also more starch grains (, ); however, this increase varied according to the strain, with more expression in the RDES (180%) and GRCE (110%) strains. The remaining strains also contained more starch grains: RDCE (37.5%) and GBCE (24%). There was also structural damage to chloroplasts, including wider separation of adjacent thylakoids (0.35 ± 0.25 µm) (, , , ), and the disintegration of mitochondrial internal cristae, irrespective of strain colour (, ). ‘Electron dense particles’ or physodes were observed in some cortical cells of RDCE () exposed to UV-B; however, they were on one occasion also observed in GBCE strains () cultivated in control condition.
Discussion
Growth rates
Although all strains showed decreased growth rates after exposure to UV-B when compared with the control, it was evident that the RDES strain showed the greatest sensitivity, presenting signs of necrosis and curling of the apical fragments after the second week of exposure. These accentuated curlings may be an adaptive response to decrease the exposure of the apical fragment. The curling was least obvious in the RDCE strain, which seems to indicate a greater resistance to increased levels of incident UV-B. These results support the hypothesis that individuals of G. birdiae from populations near the equator, where they are exposed to high irradiances and temperatures, would be more resistant to increased levels of UV-B when compared with individuals from higher latitudes. These observations also corroborate data reported in the literature, indicating that the RDCE strain is more resistant to high irradiance compared with the RDES strain and other coloured strains (Ursi & Plastino, Citation2001; Ursi et al., Citation2003). Deformation and curling of the thalli have also been described for other red algae after long-term exposure to UV-B, subsequent to a decrease in growth rates (Michler et al., Citation2002; Roleda et al., Citation2004a, Mansilla et al., Citation2006; Schmidt et al., Citation2009; Navarro et al., Citation2010b). However, yet other red algae, such as Mastocarpus stellatus and Chondrus crispus, did not undergo changes in growth when exposed to UV-B radiation (Roleda et al., Citation2004b).
The similarity in growth rates among different strains of G. birdiae cultivated in control conditions conflicts with data from previous studies in which the RDES strain showed higher growth rates when compared with other strains (Ursi & Plastino, Citation2001; Ursi et al., Citation2003). However, it should be noted that those authors used higher irradiance and constant aeration during the cultivation period, whereas the current work did not use aeration and the irradiance was lower. Such small changes in abiotic conditions could have acclimatized the photosynthetic system, thus minimizing possible differences among strains. The GRCE strain was the only one whose growth rate remained constant throughout the experiment when cultured in PAR alone (control), suggesting a greater capability to acclimatize than other strains.
Pigment composition
Phycobiliproteins play an important role in the mechanism of acclimation to light in red algae (Talarico, Citation1996; Figueroa et al., Citation1997; Roleda et al., Citation2004a) and UV-B has already been demonstrated to be effective in reducing these pigments (Eswaran et al., Citation2002; Schmidt et al., Citation2009, Citation2010a). The decrease in the concentrations of phycoerythrin (PE), phycocyanin (PC) and allophycocyanin (APC) in the RDCE strain of G. birdiae when exposed to UV-B corroborated observations of bleaching of the thalli. Similar results have been found in G. domingensis (Schmidt et al., Citation2010c), Iridaea cordata (Navarro et al., Citation2010b), and Kappaphycus alvarezii (Eswaran et al., Citation2001; Schmidt et al., Citation2010a, Citation2010b). Therefore, the presence of low concentrations of these pigments in the RDCE strain after exposure to UV-B suggests a possible use of these substances as a source of nutrients, since phycobiliproteins (mainly phycoerythrin) can act as nitrogen reserves when the demand for nitrogen is greater than the cellular and/or environmental availability (Gantt, Citation1990). In contrast to the RDCE strain, RDES, GRCE and GBCE maintained values of phycobiliproteins similar to the control. A similar result was observed in Porphyra umbilicalis, in which concentrations of these pigments remained stable after long-term exposure to radiation (Aguilera et al., Citation2008).
Long-term exposure to UV-B also promoted an increase in the PE/APC and PC/APC ratios for the GBCE strain, indicating an increase in the proportion of PE and PC in relation to APC. This increase supports the hypothesis that PE and PC act as part of a protective mechanism, suggesting, in turn, that the GBCE strain would be resistant to changes in UV-B. Indeed, GBCE only showed a decrease in growth rates in the third week under UV-B, with recovery in the fourth week.
Besides phycobiliproteins, Chl a was the most abundant pigment in the different strains of Gracilaria birdiae, regardless of treatment, confirming results previously obtained with the species (Costa & Plastino, Citation2011). Apices of different strains of G. birdiae grown in PAR condition had similar concentrations of Chl a, in disagreement with previous studies in which higher concentrations of this pigment were found for the RDCE strain when compared with the wild strain of Espirito Santo (RDES) (Ursi et al., Citation2003) and the coloured variants of the Ceará State (GRCE and GBCE) (Costa & Plastino, Citation2011). Similar concentrations of carotenoids (Car) found for all strains in this work do not support previous studies with G. birdiae, where the RDES strain showed higher carotenoid content than the other strains (Ursi et al., Citation2003). It is important to highlight again that the results obtained in this study may have been influenced by the low irradiance levels used.
Similar concentrations of Chl a in the different strains of G. birdiae after prolonged exposure to UV-B corroborated results obtained in Porphyra umbilicalis, which recorded no change in Chl a content after prolonged exposure to UV-B (Aguilera et al., Citation2008). Due to its internal location, when compared with phycobiliproteins, this pigment would be more protected from the effects of UV-B radiation (Aguilera et al., Citation1999). However, in other algae, such as Ulva expansa (Grobe & Murphy, Citation1998) and Porphyridium cruentum (Villafañe et al., Citation2005), exposure to UV-B resulted in an increase in Chl a concentration.
The different strains of G. birdiae showed similar concentrations of Car, in both experimental conditions (control and UV-B). The lack of an increase in concentration of Car as a result of exposure to UV-B, as observed in other species (Altamirano et al., Citation2000; Roleda et al., Citation2004b; Kräbs & Wiencke, Citation2005), suggests the presence of other mechanisms of protection such as the synthesis of UV-B absorbing compounds observed in some macroalgae (Karsten et al., Citation1998, 2005; Figueroa et al., Citation2003). It is important to note that ‘mycosporine-like amino acids’ (MAAs) have already been reported in G. birdiae (Cardozo et al., Citation2008). In addition, some of the carotenoids of the xanthophyll subgroup (oxygenated carotenoids) are also able to neutralize the excited form of chlorophyll by the xanthophyll cycle, protecting it from damage caused by excessive radiation (Demming-Adams & Adams, Citation1993). The occurrence of the violaxanthin cycle, an important protective mechanism against photoinhibition, has been suggested for G. birdiae (Ursi et al., Citation2003), as well as for other red macroalgae (Aihara & Yamamoto, Citation1968; Brown & McLachlan, Citation1982). Thus, although there was no increase in the carotenoid content in G. birdiae exposed to UV-B, it is possible that there was a change in the proportion of carotenoids which could not be detected with the methods we used. In a study with the diatom Thalassiosira weissflogii, higher carotenoid content occurred at an early stage of UV-B exposure, simultaneous to an increase in the pigments of the violaxanthin cycle; the synthesis of MAAs occurred, followed by a decrease in the carotenoid level (Zudaire & Roy, Citation2001). It is possible that the pigment extraction and quantification in G. birdiae, after 28 days of exposure to UV-B, occurred at the final stage of this process, preventing the detection of an increase of the carotenoid content.
Transmission electron microscopy
The ultrastructural characteristics of G. birdiae have previously been elucidated for the wild type strains of the Ceará (RDCE) and Espírito Santo (RDES) States (Plastino & Costa, Citation1999, Citation2001). This study represents the first time that ultrastructural analysis has been performed for the coloured strains (GRCE and GBCE). Coloured strains of G. birdiae exposed to PAR (control) showed characteristics similar to those observed in the wild type strains. However, some features observed in the current work, such as cytoplasmic connections, have not previously been fully described for the species. Their characteristics are consistent with previous descriptions for the Gracilariales (Pueschel, Citation1990).
Ultrastructural changes were observed after exposure to UV-B. All strains showed cortical cells with irregular contours and a large number of cell wall-producing vesicles, when compared with those grown in control conditions. Similar observations were made in Iridaea cordata (Navarro et al., Citation2010a), Palmaria palmata and P. decipiens (Poppe et al., Citation2002, Citation2003) when exposed to UV-B.
Damage to the chloroplasts in strains of G. birdiae exposed to UV-B might have contributed to the inhibition of growth. The separation of adjacent thylakoids and the formation of internal vesicles in chloroplasts have been recorded in other red algae (Poppe et al., Citation2002, Citation2003; Holzinger et al., Citation2004), indicating a greater sensitivity of chloroplasts to UV-B when compared with other cellular structures. In contrast, in the green alga Prasiola crispa, 24 hours of exposure to UV-B led to only a slight change in the chloroplasts and dilation of the thylakoids, with an apparent reduction in the number of plastoglobuli (Holzinger et al., Citation2006). Moreover, in the red alga Iridaea cordata, no changes were observed in chloroplasts, even after prolonged exposure to UV-B (Navarro et al., Citation2010a). Disintegration of the mitochondrial cristae induced by UV-B, observed in G. birdiae, has also been documented in other species, such as Palmaria palmata (Holzinger et al., Citation2004), P. decipiens (Poppe et al., Citation2003), and, to a lesser degree, Prasiola crispa (Holzinger et al., Citation2006). The lack of apparent damage to other cell structures, such as the nucleus and Golgi bodies, observed in this work and in I. cordata (Navarro et al., Citation2010a), G. domingensis (Schmidt et al., Citation2010c) and Kappaphycus alvarezii (Schmidt et al., Citation2009, Citation2010a, Citation2010b), suggests the existence of an efficient defence mechanism, preventing the destructive action of reactive oxygen species (ROS). These ROS may be responsible for the loss of cell membrane stability observed in chloroplasts and mitochondria exposed to UV-B by the action of lipid peroxidation (Kramer et al., Citation1991; Bowler et al., Citation1992; Jansen et al., Citation1996).
The protective function of the ‘electron dense particles’, or physodes, observed in some cortical cells of the RDCE strain of G. birdiae as a result of exposure to UV-B, may be attributed to the production and storage of phenolic compounds. Higher numbers of physodes have also been reported for Saccharina latissima (Holzinger et al., Citation2011) and Zygnema (Pichrtová et al., Citation2013) after exposure to UV-B.
Obvious structural changes observed in G. birdiae, such as the increase in the number of starch grains, especially in the RDES and GRCE strains after long-term exposure, have also been found in G. domingensis (Schmidt et al., Citation2010c) and Prasiola crispa (Holzinger et al., Citation2006) after exposure to UV-B. The increased number of storage compounds suggests damage to the cellular metabolism, indicating the inability to utilize these substances and maintain the mechanisms of synthesis and repair otherwise required for maintenance under adverse conditions such as UV-B exposure. This displacement of energy would, in turn, mean a reduction in other physiological processes, such as growth, as observed in G. birdiae for all the strains studied.
UV-B, absorbed mainly by nucleic acids and proteins, causes ultrastructural changes that will influence physiological processes (Karentz et al., Citation1991; Buma et al., Citation1995, Citation2003), as observed in G. birdiae. These changes were expressed as reductions in the growth rates of all strains, mainly in RDES. The RDCE strain showed the fewest ultrastructural and morphological changes, followed by the GBCE strain, suggesting that both strains are more resistant to increases in the levels of incident UV-B.
In summary, this study has used statistical and ultrastructural analyses to confirm changes in growth rates and pigment content associated with long-term exposure to UV-B in strains of G. birdiae collected from sites in lower (Ceará State) and higher (Espírito Santo State) latitudes. Our studies support the hypothesis that the population of G. birdiae and its variants growing closer to the equator (Ceará State) have adapted to higher irradiation and are thus more resistant to possible increases in UV-B, when compared with individuals from higher latitudes. Additionally, among the different strains, the RDCE and GBCE strains appear to be more resistant to changes in the current levels of UVB radiation. The presence of coloured strains in natural populations provides an additional survival mechanism, in which selection against one or more specific strains due to a sudden increase in UVB radiation will be less likely to affect the survival and abundance of the species in the environment.
Acknowledgements
This research was supported by the São Paulo Research Foundation (FAPESP: 2010/50175-3; 2011/10189-8), Brazilian National Council for Scientific and Technological Development (CNPq: 300148/93-3) and Coordination of Improvement of Higher Education Personnel (Capes). The authors acknowledge Rosário Petti for his assistance with cultivation and Waldir Caldeira for ultrastructural technical assistance. We also thank Professor Dr Carlos Frederico Martins Menck for providing the radiometer during the course of this work.
References
- Adamse, P. & Britz, S. (1992). Spectral quality of two fluorescent UV sources during long-term use. Journal of Photochemistry and Photobiology, 56: 641–644.
- Aguilera, J., Jiménez, C., Figueroa, F.L., Lebert, M. & Häder, D.P. (1999). Effect of ultraviolet radiation on thallus absorption and photosynthetic pigments in the red alga Porphyra umbilicalis. Journal of Photochemistry and Photobiology B: Biology, 48: 75–82.
- Aguilera, J., Figueroa, F.L., Häder, D.P. & Jiménez, C. (2008). Photoinhibition and photosynthetic pigment reorganization dynamics in light/darkness cycles as photoprotective mechanisms of Porphyra umbilicalis against damaging effects of UV radiation. Scientia Marina, 72: 87–97.
- Aihara, M.S. & Yamamoto, H.Y. (1968). Occurrence of antheraxanthin in two Rhodophyceae Acanthophora spicifera and Gracilaria lichenoides. Photochemistry, 7: 497–499.
- Altamirano, M., Flores-Moya, A. & Figueroa, F.L. (2000). Long-term effects of natural sunlight under various ultraviolet radiation conditions on growth and photosynthesis of intertidal Ulva rigida (Chlorophyceae) cultivated in situ. Botanica Marina, 43: 119–126.
- Barufi, J.B., Korbee, N., Oliveira, M.C. & Figueroa, F.L. (2011). Effects of N supply on the accumulation of photosynthetic pigments and photoprotectors in Gracilaria tenuistipitata (Rhodophyta) cultured under UV radiation. Journal of Applied Phycology, 23: 457–466.
- Bischof, K., Gómez, I., Molis, M., Halnet, D., Karsten, U., Lüder, U., Roleda, M.Y., Zacher, K. & Wiencke, C. (2006). Ultraviolet radiation shapes seaweed communities. Reviews in Environmental Science and Biotechnology, 5: 141–166.
- Bowler, C., van Montagu, M. & Inzé, D. (1992). Superoxide dismutase and stress tolerance. Annual Review of Plant Physiology and Plant Molecular Biology, 43: 83–116.
- Brown, L.M. & McLachlan, J. (1982). Atypical carotenoids for the Rhodophyceae in the genus Gracilaria (Gigartinales). Phycologia, 21: 9–16.
- Buma, A.G.J., Zemmelink, H.J., Sjollema, K. & Gieskes, W.W.C. (1995). UVB radiation modifies protein and photosynthetic pigment content, volume and ultrastructure of marine diatoms. Marine Ecology Progress Series, 142: 47–54.
- Buma, A.G.J., Boelen, P. & Jeffrey, W.H. (2003). UVR-induced DNA damage in aquatic organisms. In UV Effects in Aquatic Organisms and Ecosystems (Horacio, W.B. & Zagarese, E., editors), 291–320. Royal Society of Chemistry, London.
- Cabrera, S., Bozzo, S. & Fuenzalida, H. (1995). Variation in UV radiation in Chile. Journal of Photochemistry and Photobiology B: Biology, 28: 137–142.
- Cardoso, K.H.M., Vessecchi, R., Carvalho, V.M., Pinto, E., Gates, P.J., Colepicolo, P., Galembeck, S.E. & Lopes, N.P. (2008). A theoretical and mass spectrometry study of the fragments of mycosporine-like amino acids. International Journal of Mass Spectrometry, 273: 11–19.
- Costa, V.L. (2005). Diversidade intraespecífica em gametófitos de Gracilaria birdiae (Gracilariales, Rhodophyta): efeitos fisiológicos da concentração de nitrato no meio de cultura. Tese de Doutorado, Universidade de São Paulo, São Paulo. 100p.
- Costa, V.L. & Plastino, E.M. (2011). Color inheritance and pigment characterization of red (wild-type), greenish-brown, and green strains of Gracilaria birdiae (Gracilariales, Rhodophyta). Journal of Applied Phycology, 23: 599–605.
- Costa, E., Plastino, E.M., Petti, R., Oliveira, E.C. & Oliveira, M.C. (2012). The Gracilariaceae Germplasm Bank of the University of São Paulo, Brazil - a DNA barcoding approach. Journal of Applied Phycology, 24: 1643–1653.
- Demming-Adams, B. & Adams III, W.W. (1993). The xanthophyll cycle, protein turnover, and the high light tolerance of sun-acclimated leaves. Plant Physiology, 103: 1413–1420.
- Eswaran, K., Subba Rao, P.V. & Mairh, O.P. (2001). Impact of ultraviolet-B radiation on Kappaphycus alvarezii (Solieraceae, Rhodophyta). Indian Journal of Marine Sciences, 30: 105–107.
- Eswaran, K., Mairh, O.P. & Subba Rao, P.V. (2002). Inhibition of pigments and phycocolloid in a marine red alga Gracilaria edulis by ultraviolet-B radiation. Biologia Plantarum, 45: 157–159.
- Figueroa, F.L., Salles, S., Aguilera, J., Jiménez, C., Mercado, J., Viñegla, B., Flores-Moya, A. & Altamirano, M. (1997). Effects of solar radiation on photoinhibition and pigmentation in the red alga Porphyra leucosticta. Marine Ecology Progress Series, 151: 81–90.
- Figueroa, F.L., Escassia, L., Pérez-Rodríguez, E., Korbee, N., Giles, A.D. & Johnsen, G. (2003). Effects of short-term irradiation on photoinhibition and accumulation of mycosporine-like amino acids in sun and shade species of the red algal genus Porphyra. Journal of Photochemistry and Photobiology B: Biology, 69: 21–30.
- Fiscus, E.L. & Booker, F.L. (1995). Is increased UV-B a threat to crop photosynthesis and productivity? Photosynthesis Research, 43: 81–92.
- Franklin, L. & Foster, R.M. (1997). The changing irradiance environment: consequences for marine macrophyte physiology, productivity and ecology. European Journal of Phycology, 32: 207–232.
- Gantt, E. (1990). Pigmentation and photoacclimation. In Biology of the Red Algae (Cole, K.M. & Sheath, R.G., editors), 203–221. Cambridge University Press, Cambridge.
- Grobe, C.W. & Murphy, T.M. (1998). Solar ultraviolet-B radiation effects on growth and pigment composition of the intertidal algae Ulva expansa (Setch.) S. & G. (Chlorophyta). Journal of Experimental Marine Biology and Ecology, 225: 39–51.
- Häder, D.P., Kumar, H., Smith, R. & Worrest, R. (1998). Effects on aquatic ecosystems. Journal of Photochemistry and Photobiology B: Biology, 46: 53–68.
- Häder, D.P., Helbling, E.W., Williamson, C.E. & Worrest, R C. (2011). Effects of UV radiation on aquatic ecosystems and interactions with climate change. Photochemical and Photobiological Sciences, 10: 242–260.
- Harley, C.D., Anderson, K.M., Demes, K.W., Jorve, J.P., Kordas, R.L., Coyle, T.A. & Graham, M.H. (2012). Effects of climate change on global seaweed communities. Journal of Phycology, 48: 1064–1078.
- Henley, W.J. & Ramus, J. (1989). Optimization of pigment content and limits of photoacclimation for Ulva rotundata (Chlorophyta). Marine Biology, 103: 267–274.
- Hollósy, F. (2002). Effects of ultraviolet radiation on plant cells. Micron, 33: 179–197.
- Holzinger, A. & Lütz, C. (2006). Algae and UV irradiation: effects on ultrastructure and related metabolic functions. Micron, 37: 190–207.
- Holzinger, A., Lütz, C., Karsten, U. & Wiencke, C. (2004). The effect of ultraviolet radiation on ultrastructure and photosynthesis in the red macroalgae Palmaria palmata and Odonthalia dentata from arctic waters. Plant Biology (Stuttgart), 6: 568–577.
- Holzinger, A., Karsten, U., Lütz, C. & Wiencke, C. (2006). Ultrastructure and photosynthesis in the supralittoral green macroalga Prasiola crispa from Spitsbergen (Norway) under UV exposure. Phycologia, 45: 168–177.
- Holzinger, A., Di Piazza, L., Lütz, C. & Roleda, M. (2011). Sporogenic and vegetative tissues of Saccharina latissima (Laminariales, Phaeophyceae) exhibit distinctive sensitivity to experimentally enhanced ultraviolet radiation: photosynthetically active radiation. Phycological Research, 59: 221–235.
- Inskeep, W.P. & Bloom, P.R. (1985). Extinction coefficients of chlorophyll a and b in N,N-dimethylformamide and 80% acetone. Plant Physiology, 77: 483–485.
- Jansen, M.A.K., Gaba, V., Greenberg, B.M., Mattoo, A.K. & Edelman, M. (1996). Low threshold levels of ultraviolet-B in a background of photosynthetically active radiation trigger rapid degradation of D2 protein of photosystem-II. The Plant Journal, 9: 693–699.
- Karentz, D., Cleaver, J.E. & Mitchell, D.L. (1991). Cell survival characteristics and molecular responses of Antarctic phytoplankton to ultraviolet-B radiation. Journal of Phycology, 27: 326–341.
- Karsten, U., Franklin, L.A., Lüning, K. & Wiencke, C. (1998). Natural ultraviolet radiation and photosynthetically active radiation induce formation of mycosporine-like amino acids in the marine macroalga Chondrus crispus (Rhodophyta). Planta, 205: 257–262.
- Karsten, U., Friedl, T., Schumann, R., Hoyer, K. & Lembcke, S. (2005). Mycosporine-like amino acids and phylogenies in green algae: Prasiola and its relatives from the Trebouxiophyceae (Chlorophyta). Journal of Phycology, 41: 557–566.
- Kräbs, G. & Wiencke, C. (2005). Photosynthesis, photosynthetic pigments and mycosporine-like amino acids after exposure of the marine red alga Chondrus crispus (Gigartinales, Rhodophyta) to different light qualities. Phycologia, 44: 95–102.
- Kramer, G.F., Norma, H.A., Krizek, D.T. & Mirecki, R.M. (1991). Influence of UV-B radiation on polyamides, lipid peroxidation and membrane lipids in cucumber. Phytochemistry, 30: 2102–2108.
- Kursar, T.A., van der Meer, J. & Alberte, R.S. (1983a). Light-harvesting system of the red algae Gracilaria tikvahiae. I. Biochemical analyses of pigment mutations. Plant Physiology, 73: 353–360.
- Kursar, T.A., van der Meer, J. & Alberte, R.S. (1983b). Light-harvesting system of the red algae Gracilaria tikvahiae. II. Phycobilisome characteristics of pigment mutants. Plant Physiology, 73: 361–369.
- Lignell, A. & Pedersén, M. (1989). Agar composition as a function of morphology and growth rate. Studies on some morphological strains of Gracilaria secundata and Gracilaria verrucosa (Rhodophyta). Botanica Marina, 32: 219–227.
- Mansilla, A., Werlinger, C., Palácios, M., Navarro, N.P. & Cuadra, P. (2006). Effects of UV-B radiation on the initial stages of growth of Gigartina skottsbergii, Sarcothalia crispata and Mazzaella laminarioides (Gigartinales, Rhodophyta). Journal of Applied Phycology, 18: 451–459.
- McKenzie, R.L., Aucamp, P.J., Bais, A.F., Björn, L.O., Ilyas, M. & Madronich, S. (2011). Ozone depletion and climate change: impacts on UV radiation. Photochemical and Photobiological Sciences, 10: 182–298.
- Michler, T., Aguillera, J., Halnet, D., Bischof, K. & Wiencke, C. (2002). Long-term effects of ultraviolet radiation on growth and photosynthetic performance of polar and cold-temperate macroalgae. Marine Biology, 140: 1117–1127.
- Navarro, N.P., Mansilla, A. & Plastino, E.M. (2010a). Iridaea cordata (Gigartinales, Rhodophyta): responses to artificial UVB radiation. Journal of Applied Phycology, 22: 385–394.
- Navarro, N.P., Mansilla, A. & Plastino, E.M. (2010b). UVB radiation induces changes in the ultra-structure of Iridaea cordata. Micron, 41: 899–903.
- Pichrtová, M., Remias, D., Lewis, L.A. & Holzinger, A. (2013). Changes in phenolic compounds and cellular ultrastructure of Arctic and Antarctic strains of Zygnema (Zygnematophyceae, Streptophyta) after exposure to experimentally enhanced UV to PAR ratio. Microbial Ecology, 65: 68–83.
- Plastino, E.M. & Costa, V.L. (1999). Ultrastructure of vegetative branches of the red macroalga Gracilaria sp. (Gracilariales). Acta Microscopica, 8: 793–794.
- Plastino, E.M. & Costa, V.L. (2001). Anomalous plastids in a light green strain of the red macroalga Gracilaria sp. (Gracilariales). Acta Microscopica, 3: 315–316.
- Plastino, E.M. & Guimarães, M. (2001). Diversidad intraespecifica. In Sustentabilidad de la biodiversidad, un problema actual. Bases cientifico-tecnicas, teorizaciones y proyecciones (Alveal, K. & Antezana, T., editors), 19–27. Universidad de Concepción, Concepción.
- Plastino, E.M. & Oliveira, E.C. (2002). Gracilaria birdiae (Gracilariales, Rhodophyta), a new species from the tropical South American Atlantic with a terete frond and deep spermatangial conceptacles. Phycologia, 41: 389–396.
- Plastino, E.M., Ursi, S. & Fujii, M.T. (2004). Color inheritance, pigment characterization, and growth of a rare light green strain of Gracilaria birdiae (Gracilariales, Rhodophyta). Phycological Research, 52: 45–52.
- Poppe, F., Hanelt, D. & Wiencke, C. (2002). Changes in ultrastructure, photosynthetic activity and pigments in the Antarctic red alga Palmaria decipiens during acclimation to UV radiation. Botanica Marina, 45: 253–261.
- Poppe, F., Schmidt, R.A.M., Hanelt, D. & Wiencke, C. (2003). Effects of UV radiation on the ultrastructure of several red algae. Phycological Research, 51: 11–19.
- Pueschel, C.M. (1990). Cell structure. In Biology of Red Algae (Cole, K.M. & Sheath R.G., editors), 7–42. Cambridge University Press, Cambridge.
- Reynolds, E.S. (1963). The use of lead citrate at high pH as an electron-opaque stain in electron microscopy. Journal of Cell Biology, 17: 208–212.
- Roleda, M.Y., Halnet, D., Kräbs, G. & Wiencke, C. (2004a). Morphology, growth, photosynthesis and pigments in Laminaria ochroleuca (Laminariales, Phaeophyta) under ultraviolet radiation. Phycologia, 43: 603–613.
- Roleda, M.Y., van der Poll, W.H., Halnet, D. & Wiencke, C. (2004b). PAR and UV-B effects on photosynthesis, viability, growth and DNA in different life stages of two coexisting Gigartinales: implications for recruitment and zonation pattern. Marine Ecology Progress Series, 281: 37–50.
- Schemer, F., Ventura, R., Barufi, J.B. & Horta, P.A. (2012). Salinity critical threshold values for photosynthesis of two cosmopolitan seaweed species: providing baselines for potential shifts on seaweed assemblages. Marine Environmental Research. http://dx.doi.org/10.1016/j.marenvres.2012.05.007.
- Schmidt, E.C., Scariot, L.A., Rover, T. & Bouzon, Z.L. (2009). Changes in ultrastructure and histochemistry of two red macroalgae strains of Kappaphycus alvarezii (Rhodophyta, Gigartinales), as a consequence of ultraviolet B radiation exposure. Micron, 40: 860–869.
- Schmidt, E.C., Maraschin, M. & Bouzon, Z.L. (2010a). Effects of UVB radiation on the carragenophyte Kappaphycus alvarezii (Rhodophyta, Gigartinales): changes in ultrastructure, growth, and photosynthetic pigments. Hydrobiologia, 649: 171–182.
- Schmidt, E.C., Nunes, B.G., Maraschin, M. & Bouzon, Z.L. (2010b). Effects of ultraviolet-B radiation on growth, photosynthetic pigments, and cell biology of Kappaphycus alvarezii (Rhodophyta, Gigartinales) macroalgae brown strain. Photosynthetica, 48: 161–172.
- Schmidt, E.C., Santos, R., Horta, P.A., Maraschin, M. & Bouzon, Z.L. (2010c). Effects of UVB radiation on the agarophyte Gracilaria domingensis (Rhodophyta, Gracilariales): changes in cell organization, growth and photosynthetic performance. Micron, 41: 919–930.
- Somozy, Z. (2000). Radiation response of cell organelles. Micron, 31: 165–181.
- Talarico, L. (1996). Phycobiliproteins and phycobilisomes in red algae: adaptive responses to light. Scientia Marina, 60: 205–222.
- Talarico, L. & Maranzana, G. (2000). Light and adaptive responses in red macroalgae: an overview. Journal of Photochemistry and Photobiology B: Biology, 56: 1–11.
- Ursi, S. & Plastino, E.M. (2001). Crescimento in vitro de linhagens de coloração vermelha e verde clara de Gracilaria sp. (Gracilariales, Rhodophyta) em dois meios de cultura: analise de diferentes estádios reprodutivos. Revista Brasileira de Botânica, 24: 587–594.
- Ursi, S., Pedersén, M., Plastino, E.M. & Snoeijs, P. (2003). Intraspecific variation of photosynthesis, respiration and photoprotective carotenoids in Gracilaria birdiae (Gracilariales: Rhodophyta). Marine Biology, 142: 997–1007.
- Ursi, S., Costa, V.L., Hayashi, L., Pereira, R.T.L., Paula, E.J. & Plastino, E.M. (2013). Intraspecific variation in Gracilaria birdiae (Gracilariales, Rhodophyta): growth, and agar yield and quality of color strains under aquaculture. Botanica Marina, 56: 241–248.
- van de Poll, W.H., Eggert, A., Buma, A.G.J. & Breeman, A.M. (2001). Effects of UV-B induced DNA damage and photoinhibition on growth of temperate marine red macrophytes: habitat related differences in UV-B tolerance. Journal of Phycology, 37: 30–37.
- Villafañe, V.E., Gao, K. & Helbling, E.W. (2005). Short- and long- term effects of solar ultraviolet radiation on the red algae Porphyridium cruentum (S.F.Gray) Nägeli. Photochemical and Photobiological Sciences, 4: 376–382.
- Xu, J. & Gao, K. (2007). Growth, pigments, UV-absorbing, compounds and agar yield of economic red seaweed Gracilaria lemaneiformis (Rhodophyta) grown at different depths in the coastal waters of the South China Sea. Journal of Applied Phycology, 20: 681–686.
- Xue, L., Zhang, Y., Zhang, T., An, L. & Wang, X. (2005). Effects of enhanced ultraviolet-B radiation on algae and cyanobacteria. Critical Reviews in Microbiology, 31: 79–89.
- Watanabe, S., Sudo, K., Nagashima, T., Takemura, T., Kawase, H. & Nozawa, T. (2011). Future projections of surface UV-B in a changing climate. Journal of Geophysical Research, 116: D16118, doi:10.1029/2011JD015749.
- Wellburn, A.R. (1994). The spectral determination of chlorophylls a and b, as well as total carotenoids, using various solvents with spectrophotometers of different resolution. Journal of Plant Physiology, 144: 307–313.
- Zepp, R.G., Erickson III, D.J., Paul, N.D. & Sulzberger, B. (2011). Effects of solar UV radiation and climate change on biogeochemical cycling: interactions and feedbacks. Photochemical and Photobiological Sciences, 10: 261–279.
- Zudaire, L. & Roy, S. (2001). Photoprotection and long-term acclimation to UV radiation in the marine diatom Thalassiosira weissflogii. Journal of Photochemistry and Photobiology B: Biology, 62: 26–34.