Abstract
The main objective of this study was to determine the optimal concentrations of a wide spectrum of exogenous phytohormones for effective stimulation of cell division and production of maximum cell yield in Euglena gracilis Klebs cultured in vitro. Results indicate that two hormones combined exert more effective growth stimulation than a single hormone or three, four or five different hormones combined. Specifically, trans-zeatin at 10−7 M combined with abscisic acid at 10−9 M produced optimal conditions for growth, yielding the maximum cell concentration. High concentrations of exogenous phytohormones were toxic to Euglena. The addition of trans-zeatin, N6-isopentenyladenine, and benzylaminopurine to Euglena cultures resulted in dense, dark green chloroplasts, suggesting that exogenous phytohormones increased the production of chlorophyll. Given the response to exogenous growth regulators, the study identified and quantified the types of endogenous cytokinins (CKs) and abscisic acid (ABA) synthesized in vitro by Euglena gracilis. HPLC-(ESI) MS/MS analysis revealed that the algal cells produced and released into the medium a mixture of CKs and ABA. The main CKs identified in the cell pellets and supernatant samples were from a t-RNA degradation pathway and included: cis-zeatin (cZ) derivatives cZR, cZNT, MeSZ and MeSZR, and to a lesser extent, the free base N6-isopentenyladenine (iP) and its derivatives iPR, iPNT, MeSiP and MeSiPA. A positive response to ABA, and the relatively high levels detected in E. gracilis, suggest that this hormone is important for alleviating stress conditions of in vitro culture that might otherwise restrict cell division.
Abbreviations
2MeSZ | = | methylthiol-zeatin |
2MeSZR | = | methylthiol-zeatin riboside |
ABA | = | abscisic acid |
BAP | = | benzylaminopurine |
BAR | = | benzylaminopurine riboside |
cZ | = | cis-zeatin |
cZR | = | cis-zeatin 9-riboside |
CK | = | cytokinin |
DHZ | = | dihydrozeatin |
DHZR | = | dihydrozeatin 9-riboside |
FB | = | free bases (DHZ + tZ + cZ + iP) |
GA3 | = | gibberellin |
GLUC | = | glucosides (DZOG + tZOG + cZOG + DZROG + tZROG + cZROG + DZ9G + tZ9G + cZ9G) |
IAA | = | indole-3-acetic acid |
iP | = | N6-isopentenyladenine |
iPR | = | N6-isopentenyladenine 9-riboside |
LC-(ESI) MS/MS | = | liquid chromatography-electrospray ionization-tandem mass spectrometry |
MeSiP | = | methylthiol-isopentenyladenine |
MeSiPA | = | methylthiol-isopentenyladenosine |
MET | = | methylthiols (2MeSZ + 2MeSiP + 2MeSZR + 2MeSiPA) |
NT | = | nucleotides (DHZNT + tZNT + cZNT + iPNT) |
RB | = | ribosides (DHZR + tZR + cZR + iPR) |
tZ | = | trans-zeatin |
tZR | = | trans-zeatin 9-riboside |
Introduction
Phytohormones are organic substances which play a major role in regulating plant growth and development. These molecules individually or cooperatively direct the metabolism of individual cells and carry information between cells, thereby coordinating growth and development. Several groups of physiologically active hormone compounds are ubiquitous among higher plants, such as auxins, cytokinins, gibberellins and abscisic acid (Santner et al., Citation2009). These endogenous growth regulators have also been detected in various algal taxa in concentrations comparable to those of vascular plants. However, experimental data elucidating hormone metabolism and action in algae, and their role in algal growth, are limited (Bradley, Citation1991; Tarakhovskaya et al., Citation2007; Mekhalfi et al., Citation2012).
Cytokinins (CK) are derivatives of the nitrogenous base adenine and are classified by their side chain as either isoprenoid or aromatic. Isoprenoid CKs are divided into four groups: trans-zeatin (tZ), cis-zeatin (cZ), dihydrozeatin (DHZ) and isopentenyladenine (iP), with aromatic CKs consisting of benzylaminopurine (BAP) and topolins (T) (Stirk & Van Staden, Citation2010; Spichal, Citation2012). In addition to stimulating cell division and differentiation in vascular plants, CKs also regulate shoot and root differentiation, growth of lateral buds and leaf expansion, floral transition, chloroplast development, seed germination, nutrient mobilization, biomass distribution and delay of senescence (Mok, Citation1994; Martin et al., Citation2001; Burkle et al., Citation2003; Tarakhovskaya et al., Citation2007). Endogenous CKs have also been described in numerous algal taxa. Both isoprenoid and aromatic CKs have been identified in green, red and brown macroalgae and in microalgae in the Chlorophyta, Cyanophyta and Chrysophyta. Despite these taxa belonging to diverse evolutionary lineages, their CK profiles are similar, in that derivatives of iP and cZ dominate (Stirk et al., Citation2003, Citation2009, Citation2011; Ordog et al., Citation2004; Piotrowska & Czerpak, Citation2009; Gupta et al., Citation2011; Mekhalfi et al., Citation2012).
The most characteristic response elicited by the application of exogenous CKs in higher plants and algae is stimulation of cell division. Although little is known about CK biosynthesis in algae, Chlorella vulgaris cultures showed a significant increase in cell number in the presence of exogenous CKs (Piotrowska & Czerpak, Citation2009), and exogenous CKs have been reported to stimulate cell division in both micro- and macroalgal species (Jennings et al., Citation1972; Pedersen, Citation1973; Buczek et al., Citation1975; Tatkowska & Buczek, Citation1980; Burkiewicz, Citation1987; De Nys et al., Citation1990; Bradley, Citation1991).
Abscisic acid (ABA) is a phytohormone that plays an important role in plant responses to environmental stress and plant pathogens (Jiang & Hartung, Citation2008; Santner et al., Citation2009). The general functions of ABA in vascular plants are to inhibit precocious germination and promote dormancy in seeds, and production of molecules that protect cells against desiccation (Nakashima & Yamaguchi-Shinozaki, Citation2013). ABA has also been implicated in induction of the synthesis of storage protein in seeds, heterophylly, initiation of secondary roots, flowering and senescence (Mauch-Mani & Mauch, Citation2005; Tuteja, Citation2007). GC-MS studies have shown the presence of ABA in several algal species, such as Dunaliella parva, Chlamydomonas reinhardtii, Ulva lactuca, Draparnaldia mutabilis and Chara foetida (Bradley, Citation1991). Furthermore, tests of 64 algal species revealed an accumulation of ABA under adverse environmental conditions (Hirsch et al., Citation1989). Similar effects are seen in bacteria (Cohen et al., Citation2008) and higher plants; ABA accumulates in the tissues of plants grown under water-, osmotic- or salt-stress conditions, and can act as an important stress-alleviating factor (Creelman & Zeevaart, Citation1985; LaRosa et al., Citation1985; Stewart et al., Citation1986; Bensen et al., Citation1988; Zeevaart & Creelman, Citation1988).
Among the other plant hormones, indole-3-acetic acid (IAA) is the most widely distributed natural auxin. IAA is primarily responsible for the stimulation of cell elongation in excised stem and coleoptile sections but is also involved in secondary root initiation, vascular differentiation, the development of axillary buds, flowers and fruits, and enabling roots and shoots to respond to gravity and unilateral light (Santner et al., Citation2009). Gibberellins (GA3) regulate cell elongation and the mobilization of endosperm reserves in early seed germination, and have been implicated in developmental responses such as flowering, root and fruit growth, seed development in fruits, and the development of leaf primordia in stems (Santner et al., Citation2009).
Previous investigations into the role of plant hormones as factors regulating algal growth have focused on the identification and exogenous application of phytohormones in seaweeds. However, there is an increasing interest in phytohormones in microalgae, especially those mass cultured for the production of biofuel, and for use in the pharmaceutical industry, as agrochemicals and animal feed (Stirk et al., Citation2011). The objective of this investigation was to better understand the effect of exogenously applied phytohormones on the growth of Euglena gracilis Klebs, a unicellular photosynthetic protist with unusual traits, such as motility and phototactic response to light, which can be exploited for harvesting strategies.
To our knowledge, this is the first study where Euglena was used as a model to analyse the role of CKs and ABA, as well as the effect of selected auxins and gibberellins on algal growth. This study combines CK/ABA profiling and examination of the response to different CKs, ABA, IAA and GA3 applied exogenously, both as single treatments and multiple hormone combinations. Our work identified important hormonal interactions which play a role in algal growth. Microscopic examination of Euglena morphology qualified the effects of the exogenously applied growth regulators at the cellular level. Endogenous production of CKs and ABA during Euglena gracilis in vitro culture was confirmed by LC-MS/MS.
Materials and methods
Experimental material and growth conditions
The Euglena gracilis Klebs culture was purchased from Boreal Laboratory Supplies Ltd (St. Catharines, Ontario, Canada). 100 ml of the culture was added to 2.9 l of Euglena gracilis medium consisting of CaCl2 (0.01 g l−1), CH3COONa.3H2O (1.0 g l−1) and ‘Lab-Lemco’ powder (LP0029) (1.0 g l−1), tryptone (LP0042) (2.0 g l−1) and yeast extract (LP0021) (2.0 g l−1) (Oxoid LDD, Basingstoke, Hampshire, England). Euglena was cultured non-axenically, in an environmental chamber (Controlled Environments Ltd, Winnipeg, Manitoba, Canada) at 25°C and pH 7.5 for 7 days, and was aerated with 160 ml min−1 of filtered compressed air to form the stock culture. Illumination was supplied during a 16-h photoperiod by fluorescent lights (200 μmol photon m−2 s−1).
500 μl of Euglena stock culture was collected daily and cells were counted using Vi-Cell XR Viability Analyzer (Beckman Coulter Canada, Inc., Mississauga, Ontario, Canada).
Determination of optimum phytohormone concentrations for Euglena growth
To prepare test sub-cultures for single and multiple hormone treatments, 25 ml of 7-day-old Euglena stock culture was centrifuged (4000 rpm) for 5 minutes, the pellet was washed and re-suspended in 25 ml of DI water, and incubated at 25°C and pH 7.5 for 48 h. All test cultures were grown under the same environmental conditions as stock culture.
Single hormone treatment
In order to examine the effect of ABA, CKs (tZ, iP, BAP), IAA and GA3 (Sigma-Aldrich Co., LLC) on Euglena growth, the phytohormone solutions were applied to 2-day-old sub-cultures in the early exponential phase (4.5×104 cells ml−1) at 5 concentrations: 10−9, 10−8, 10−7, 10−6 and 10−5 M, in replicates of five. An equivalent amount of Milli-Q™ water was added to control cultures.
The hormone concentrations yielding the maximum cell counts were consequently applied to analyse the effect of multiple hormone treatment. These same hormones were also selected as important metabolites for MS profiling of their endogenous levels.
Multiple hormone treatment
Results from the first part of these experiments provided the basis for multiple hormone treatments. Phytohormones dissolved in Milli-Q™ water were prepared in 26 different combinations of ABA, tZ, iP, BAP and IAA, at their optimum concentration for Euglena growth and applied to Euglena test cultures at the early exponential phase (2.7×104 cells ml−1), in replicates of five. An equivalent amount of Milli-Q™ water was added to control cultures.
Determination of cell concentration
For growth profiles of Euglena gracilis cultures, 500 µl samples were collected from the single and multiple hormone treatments after 144 h growth and the number of cells determined by direct counting using a Vi-Cell XR Viability Analyzer (Beckman Coulter Canada, Inc., Mississauga, Ontario) and compared with the control.
Statistical differences between treatments were evaluated with Duncan’s range test in conjunction with an analysis of variance. Due to the differences in initial cell concentrations of cultures used in single and multiple hormone treatment experiments, results were converted to a percentage of the cell concentration in control cultures (100%).
Microscopic examination of Euglena gracilis cells with single and multiple hormone treatment
The single phytohormones, in concentrations that maximized culture growth, were applied to sub-cultures of Euglena gracilis: ABA, iP, BAP, IAA and GA3 at 10−9 M, and tZ at 10−7 M. The same phytohormones were applied to the cultures at 10−5 M concentration, as at this concentration culture growth was inhibited and minimized. For the combined hormone treatments, the phytohormone combination which maximized culture growth was applied to test sub-cultures of Euglena gracilis: ABA at 10−9 M and tZ at 10−7 M. The combination that resulted in minimal culture growth, ABA, iP, BAP and IAA at 10−9 M, and tZ at 10−7 M, was also tested. All treated cultures were incubated for 144 hours as detailed under growth conditions.
Euglena cells taken from maximal and minimal growth cultures were compared with control samples for differences in cell size and morphology. 100 µl of each Euglena culture was removed and placed on a depression slide for examination with an Omax V434 Series stereoscopic microscope (Omax Co. Ltd, Korea).
Measurements of endogenous abscisic acid and cytokinins levels in Euglena gracilis
Extraction and purification of ABA and CKs
A modified protocol described by Quesnelle & Emery (Citation2007) and Farrow & Emery (Citation2012) was used. To prepare test cultures, 15 ml of Euglena stock culture was centrifuged (4000 rpm) for 5 min, the pellet was washed and re-suspended in 5 ml of DI water, and grown at 25°C and pH 7.5 for 48 hours. Samples were centrifuged (4000 rpm) for 5 min and the supernatant was decanted. The supernatant and pellet were dried in a rotary vacuum at 35°C, re-suspended in extraction buffer (CH3OH:H2O:HCOOH [15:4:1, v/v/v]) and then homogenized (ball mill, Retsch MM300) at 4°C over ice (−20°C). The tissue samples were spiked with internal standards (149.8 ng of 2H4-ABA (PBI, Saskatchewan, Canada) and 10 ng of each of the deuterated internal standard CKs (OlChemim Ltd, Olomouc, Czech Republic; ), vortexed thoroughly and sonicated for 1 min. The samples were allowed to extract passively overnight (approximately 12 hours) at −20°C. Pellets were re-extracted with 1 ml extraction buffer at −20°C. The pooled supernatants were dried in a speed vacuum concentrator at 35°C.
Table 1. Cytokinins (CKs) scanned by liquid chromatography-positive electrospray ionization tandem mass spectrometry (HPLC (ESI)-MS/MS) in Euglena cells and supernatants.
Extraction residues were reconstituted in 0.2 ml of 1 M formic acid (pH 1.4) to ensure complete protonation of all CKs. Each extract was purified on a mixed mode, reverse-phase, cation-exchange cartridge (Oasis MCX 2cc; Waters, Mississauga, Ontario, Canada). Cartridges were activated using 1 ml of HPLC grade methanol and equilibrated using 1 ml of 1 M formic acid (pH 1.4). After equilibration, each sample was loaded and washed with 1 ml of 1 M formic acid (pH 1.4). ABA and CKs were eluted based on their chemical properties. ABA was eluted first using 1 ml HPLC grade methanol. The nucleotide fraction (CKNTs) was eluted using 1 ml 0.35 M ammonium hydroxide, free bases (CKFBs) and ribosides (CKRBs) were retained based on charge and hydrophobic properties and, thus, these were eluted last using 1 ml 0.35 M ammonium hydroxide in 60% methanol. All samples were evaporated to dryness in a speed vacuum concentrator at 35°C and immediately stored at −20°C. CKNTs were dephosphorylated using 3 units of bacterial alkaline phosphatase in 1 ml 0.1 M ethanolamine-HCl (pH 10.4) for 12 hours at 37°C (Emery et al., 1998). The resulting CKRs were brought to dryness in a speed vacuum concentrator at 35°C. Samples were re-constituted in 0.3 ml double-distilled water (DDW) for further purification on a reversed-phase C18 column (Canadian Life Sciences; C18 2cc, 96-well plate; Peterborough, Ontario, Canada). Columns were activated using 0.6 ml HPLC grade methanol and equilibrated with 1.2 ml double-distilled water. The samples were loaded onto the C18 cartridge and allowed to pass through the column by gravity. The sorbent was washed with 0.6 ml DDW and analytes were eluted using 1 ml HPLC grade methanol. All sample eluents were dried in a speed vacuum concentrator at 35°C and stored at −20°C until further processing. Prior to LC-MS/MS analysis, all dried samples were re-constituted in 1.5 ml buffer (CH3COOH:C2H3N:ddH2O [0.08:5.0:94.92, vol/vol/vol] for CK, and CH3COOH:CH3OH:ddH2O [0.08:5.0:94.92, vol/vol/vol] for ABA).
CKs and ABA quantification and analysis
Hormones were identified and quantified by electrospray ionization, liquid chromatography-tandem mass spectrometry, HPLC-(ESI) MS/MS (Agilent HPLC connected to Sciex Applied Biosystem 5500 API Mass Spectrometer). A 20 µl sample volume was injected on a Luna reversed-phase C18 column (3 µm, 150 × 2.1 µm; Phenomex, Torrance, California, USA). CKs were eluted with an increasing gradient of 0.08% acetic acid in acetonitrile (A) mixed with 0.08% acetic acid in DDW (B) at a flow rate of 0.2 ml min−1. The initial conditions were 5% A and 95% B, changing linearly to 95% A and 5% B for 5 min, and then linearly returning back to initial conditions for 20 min. ABA was eluted with an increasing gradient of 0.08% acetic acid in methanol (A) mixed with 0.08% acetic acid in DDW (B) at a flow rate of 0.2 ml min−1. The initial conditions were 50% A and 50% B, changing linearly to 95% A and 5% B for 1 min, and then linearly returning back to initial conditions for 20 min. The effluent was introduced into the electrospray source (source block temperature of 700°C), using conditions specific for each CK, with multiple reaction monitoring (MRM) of the protonated intact CK molecule [M+H]+ and the specific product ion.
Data were analysed using Analyst (v 1.5) software (AB Sciex, Framingham, Massachusetts, USA), to calculate peak area. Quantification was achieved through isotope dilution analysis based on recovery of 2H-labelled internal standards.
Results
Single hormone treatments
All hormones showed biphasic responses, firstly promotive and secondly inhibitory. Preliminary experiments showed that ABA, iP, BAP, IAA and GA3 at 10−9 M and tZ at 10−7 M were most effective at inducing cell division as cell number reached maximum density (), so these concentrations were used in the multiple hormone treatments. All phytohormones tested at 10−5 M, with the exception of BAP, suppressed culture development and inhibited cell proliferation. Cultures treated with BAP 10−5 M were not significantly different from the control.
Fig. 1. Dose–response growth curve of hormone-treated Euglena gracilis cells. The phytohormone solutions were applied to Euglena cultures in their early exponential phase (4.5×104 cells ml−1) at 5 concentrations: 10−9, 10−8, 10−7, 10−6 and 10−5 M (five replicates). The number of cells in the growth medium was determined after 144 h by direct counts with a Vi-Cell XR Viability Analyzer) and compared with the control. The cell concentration of control cultures is represented by the dotted line (100%); ns, no significant differences between the applied concentrations of the same hormone according to Duncan’s test (P = 0.05), NS, no significant difference from the control.
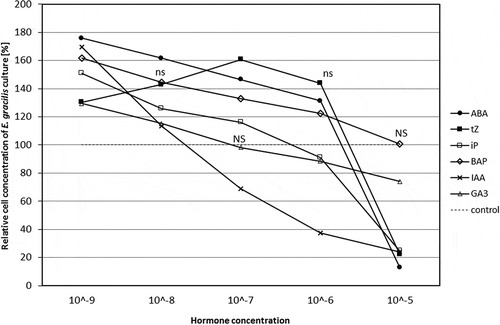
Multiple hormone treatments
tZ at 10−7 M combined with ABA at 10−9 M enhanced cell division and yielded the maximum cell concentration (). The number of cells in the culture treated with the most effective hormone combination increased by over 140% after 144 h of cultivation compared with the control. Generally, increasing the number of different hormones in a single combination decreased cell count; lowest cell counts were observed in cultures treated with a combination of five hormone types.
Fig. 2. Stimulation of Euglena gracilis growth by multiple hormone treatments prepared in 26 different combinations of ABA, tZ, iP, BAP and IAA at their optimum concentrations for Euglena growth and applied to cultures at the early exponential growth phase (2.7×104 cells ml−1; five replicates). The number of cells in the growth medium was determined after 144 h by direct counts and compared with the control (100%); bars labelled with different letters are significantly different according to Duncan’s test (P = 0.05), NS, no significant difference from the control.
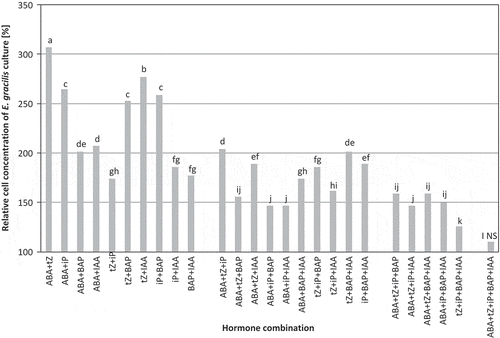
Microscopic examination of Euglena gracilis cells with different hormone treatments
The results of microscopic analysis of Euglena gracilis cell size and morphology after single and multiple hormone treatments are presented in and Figs 3–18. Euglena control cultures had elongated cells with numerous small chloroplasts that were not possible to differentiate individually (Figs 3–4). Inhibitory hormone concentrations applied to Euglena most often resulted in characteristic, spherical or teardrop-shaped cells. Additionally, algal cells treated with different hormone types and concentrations exhibited significant differences in size, colour and density of chloroplasts, as well as altered morphology of cell membrane.
Table 2. Cell size of Euglena gracilis after phytohormone treatment of the cultures by concentrations best promoting growth (single treatments: tZ at 10−7 M, and iP, ABA, BAP, IAA, GA3 at 10−9 M; combination of tZ at 10−7 M and ABA at 10−9 M), and most inhibitory treatments (single treatments: tZ, iP, ABA, BAP, IAA and GA3 at 10−5 M; combination of tZ at 10−7 M and iP, ABA, BAP, IAA at 10−9 M). Results are means of the diameter of 50 cells, recorded by a cell counter for each of the tested hormone treatments. Control cells mean diameter 14.66 μm.
Figs 3–18. Euglena gracilis cell morphology. Euglena cells from maximal (A) and minimal (B) growth cultures were compared with control samples and examined for differences in cell morphology. Figs 3, 4. Algae growing in control media. Fig. 5. tZ at 10−7 M. Fig. 6. tZ at 10−5 M. Fig. 7. iP at 10−9 M. Fig. 8. iP at 10−5 M. Fig. 9. ABA at 10−9 M. Fig. 10. ABA at 10−5 M. Fig. 11. BAP at 10−9 M. Fig. 12. BAP at 10−5 M. Fig. 13. IAA at 10−9 M. Fig. 14. IAA at 10−5 M. Fig. 15. GA3 at 10−9 M. Fig. 16. GA3 at 10−5 M. Fig. 17. tZ at 10−7 and ABA at 10−9 M. Fig. 18. tZ at 10−7, iP, ABA, IAA, and BAP at 10−9 M.
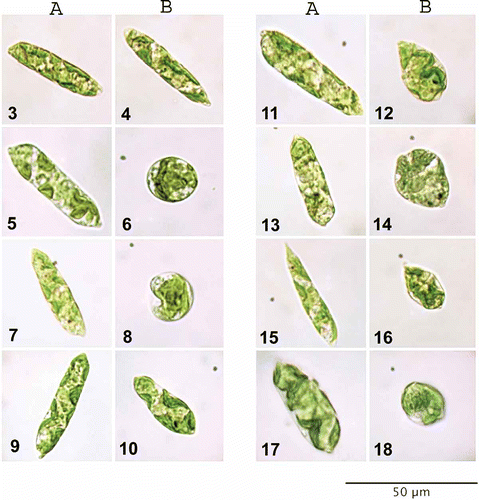
Endogenous ABA and CKs measurements
HPLC-(ESI) MS/MS analysis showed that Euglena gracilis can produce and release ABA and a mixture of CKs to the media. The endogenous hormone profiles of the tested algal cells and supernatants are presented in . Although Euglena cultures were grown non-axenically, no visible bacterial contamination was detected, either by microscope observations or cell counter scanning. For this reason, all the observed hormone profiles were considered as of algal origin.
Table 3. Abscisic acid and cytokinins quantified in Euglena gracilis cell pellets and supernatants. After centrifugation, Euglena cell pellets and supernatants were analysed by liquid chromatography tandem mass spectrometry separately for ABA and CK. Concentrations are given in picomoles per g fresh weight ± standard error for the pellets, and picomoles per ml culture media ± standard error for supernatants. Results shown are the mean values of four replicates.
Four CK fractions (FB, RB, NT and MET) were detected in Euglena pellet and supernatant samples, with cis-zeatin riboside prevailing in the pellet. Analysis of 2-day-long release of growth regulators to the culture medium showed that the hormone fractions secreted most abundantly outside the cells were methylthiols, with the exception of MeSiP, which was present in higher amounts in the pellet samples. Interestingly, relatively high levels of ABA were noted for the Euglena pellet and the media, when compared with the levels of most of the CK derivatives detected in the tested samples.
Detailed analysis of isoprenoid CKs revealed that for zeatin forms, the trans isomer of the free base fraction was present in the supernatant but the cis isomer was not detected. For riboside conjugates, the cis isomer was present whereas tZR was neither detected in the pellet nor supernatant. Both nucleotide forms, tZNT and cZNT were present in minute quantities. DHZ and its riboside were not detected and its nucleotide conjugate was present in negligible quantities only in Euglena supernatant. For isopentenyl derivatives, the free base, iP, was present as was its nucleotide precursor. In the pellet, iP nucleotide appeared at a higher concentration than the free base.
Finally, BAP was observed both in the pellets and supernatants, whereas no glycosyl-conjugated CKs were detected in any of the samples.
Discussion
Exogenous phytohormones can significantly enhance in vitro growth of Euglena gracilis. Euglena exhibited sensitivity to the following phytohormones, listed in order of their stimulating properties at optimal concentrations: ABA, IAA, BAP, tZ, iP and GA3. Euglena showed a dose-dependency in its sensitivity to exogenous phytohormones. tZ at 10−7 and ABA, iP, BAP, IAA and GA3 at 10−9 M caused the greatest increase in cell concentration, while inhibition of algal growth was observed in the presence of ABA, tZ, iP, IAA and GA3 at the highest applied concentration (10−5 M). The phytotoxic effect of high concentrations of phytohormones on plant growth has been previously reported (Carimi et al., Citation2004; Synkova et al., Citation2004). Our results correspond to these investigations, where high concentrations of CKs had cytotoxic effects on vascular plant growth by blocking cell proliferation and inducing programmed cell death. Results of the hormone combination studies indicate that two hormones combined exert a stronger growth-stimulating effect than one, three, four or five different hormones applied together. Furthermore, of all the multiple hormone treatments, tZ at 10−7 M combined with ABA at 10−9 M produced the most effective conditions for growth, yielding the maximum cell concentration.
Exogenous ABA, applied alone and in combination with tZ, produced the highest cell densities. Huang (Citation1991) found that the influx of phosphate, the efflux of protons and the release of proteins from Anabaena cells decreased in ABA-treated cultures by reducing the permeability of the plasmalemma; ABA provides a means of adapting to stress conditions. Exogenous ABA may enable Euglena gracilis cells in culture to mitigate abiotic and/or biotic stress conditions developing in vitro, thereby producing high cell concentrations, similar to beneficial effects on Arabidopsis plants of inoculation with ABA-producing bacteria under stress conditions (Cohen et al., Citation2008). In Euglena, ABA may play a promotive role and enhance growth, as in vascular plant systems where ABA is not only a hormone that regulates stress-signalling and controls stress responses, but often is critical for seed filling and tumour development (Efetova et al., Citation2007; Tuteja, Citation2007; Santner et al., Citation2009).
Euglena gracilis cultures showed a significant increase in cell numbers in response to exogenous CKs, tZ, iP and BAP at their optimal concentrations. This corresponds to earlier results where exogenous CKs stimulated cell division in micro- and macroalgal species (Jennings et al., Citation1972; Buczek et al., Citation1975; Tatkowska & Buczek, Citation1980; Burkiewicz, Citation1987; De Nys et al., Citation1990; Stirk et al., Citation1999). Piotrowska and Czerpak (Citation2009) have demonstrated that CK treatment in darkness stimulates cell division, suggesting a relationship between CK and cell division in green algae, but this relationship is not yet fully understood.
Production of chlorophyll a and b is increased in response to adenine-type CKs in Chlorella vulgaris, a green unicellular alga (Piotrowska & Czerpak, Citation2009); this concurs with earlier papers stating that CK treatment abolishes the lag phase in chlorophyll synthesis and accelerates its production rate (Buschmann & Lichtenthaler, Citation1982). It is thought that CKs probably reduce chlorophyll destruction and/or increase chlorophyll synthesis (Piotrowska & Czerpak, Citation2009). In our investigation, Euglena gracilis cells exhibited densely packed, dark green chloroplasts in response to exogenously applied CKs (tZ, iP and BAP), thereby supporting earlier reports that CKs enhance chlorophyll content. An increase in chlorophyll content would stimulate photosynthesis, resulting in increased potential for metabolic activity and accumulation of metabolites. It has been previously shown that CKs induced a nine-fold increase in glucose, fructose, galactose and ribose in Beta vulgaris (Ivic et al., Citation2001). CKs led to an increase in metabolically active proteins in the green algae Scenedesmus quadricada, S. aquminatus, Chlorella pyrenoidosa and C. vulgaris (Tatowska & Buczek, Citation1980; Czerpak et al., Citation1999); C. vulgaris treated with CKs and grown in darkness exhibited delayed degradation of protein and/or stimulation of protein synthesis (Piotrowska & Czerpak, Citation2009). As we did not observe a significant increase in the cell size of CK-treated Euglena cultures, it is reasonable to assume that the increased by-product(s) of photosynthesis, namely sugars and proteins, are being utilized for cell division, metabolic activities and the synthesis of subcellular structures. For example, cell membranes appeared thicker in Euglena gracilis treated with tZ and BAP than those of controls.
Auxins and gibberellins are growth-promoting hormones that, in higher plants, are primarily characterized by, but not limited to, their capacity to stimulate cell elongation (Santner et al., Citation2009). Euglena gracilis cells exposed to optimal concentrations of IAA and GA3 had elongated cells with some enlargement. Exogenous IAA and GA3 significantly accelerates the growth rate of cells in numerous algal taxa (Jennings, Citation1968; Yang et al., Citation2000; Li et al., Citation2002; Shi et al., Citation2004; Pan et al., Citation2008). It has been suggested that GA3 increases the growth of Microcystis aeruginosa by enhancing their nitrogen-absorbing ability (Pan et al., Citation2008). At concentrations of this hormone above the optimum, growth rate is inhibited compared with controls (Li et al., Citation2002; Shi et al., Citation2004; Yang et al., 2004; Pan et al., Citation2008).
We are aware that because Euglena gracilis in our experiments was cultured non-axenically, the possibility of microbial contribution to endogenous hormone levels cannot be excluded. However, we never observed bacterial contamination of the cultures, and the detected hormone profiles do not resemble those known for prokaryotes, which generally produce considerably higher levels of methylthiol-type CKs, as well as iP derivatives (Kisiala et al., Citation2013). For this reason, we consider all the endogenous hormone profiles to be of algal origin.
Recently published reports on the ligand preferences of CK receptors indicate that tZ and its conjugates are the most active type of endogenous CKs in vascular plants, while iP-type CKs are considered to have lower biological activity, especially in above-ground organs (Romanov et al., Citation2006; Hirose et al., Citation2008; Stirk et al., Citation2009). However, in non-vascular plants such as mosses or algae, the free bases iP and cZ as well as their riboside and nucleotide conjugates often predominate (Auer, Citation1997; Stirk et al., Citation2003, Citation2009; Stirk & Van Staden, Citation2010). The results of this study identify the main CKs in Euglena gracilis as the cZ derivatives: cZR, cZNT, MeSZ and MeSZR, and to a lesser extent, the free base iP and its derivatives: iPR, iPNT, MeSiP and MeSiPA. These results support the theory that the CK de novo synthesis pathway only evolved within the seed plants, whereas tRNA degradation is the predominant pathway of CK production in lower organisms (reviewed in Spichal, Citation2012). This is also consistent with earlier findings pertaining to endogenous CK profiles of seaweeds and microalgal Chlorophyta and Cyanophyta strains (Stirk et al., Citation1999, Citation2003, Citation2009; Ordog et al., Citation2004).
Free bases and ribosides are the most physiologically active CK forms (Spichal et al., Citation2004; Sakakibara, Citation2006). Although Sakakibara (Citation2006) suggested that cZ isomers had little or no biological activity, cZ forms were recently proven to have comparable biological activity to tZ forms in an in vitro zygotic pea embryo bioassay (Quesnelle & Emery, Citation2007). Furthermore, the cZ riboside (cZR) was shown to be the most effective cZ derivative and only slightly less active than its trans counterpart (Gajdosova et al., Citation2009). Based on changes in CK distribution observed during Arabidopsis development, Gajdosova et al. (Citation2011) hypothesized that cis-type CKs function as delicate regulators of CK responses in plants under growth-limiting conditions. In the present study, cZR represented the largest proportion of the CKs and total phytohormone complement. Together, this body of evidence suggests that cZ isomers have important functions in the physiology of algal cells.
Euglena profiles of endogenous hormones suggest that methylthiol derivatives are released outside algal cells more quickly than accumulated within. This is contrary to the CK profiles observed for cultured Rhizobium bacteria, where methylthiols were the main CK type detected in the cell pellet (Kisiala et al., Citation2013). Glucoside derivatives were not detected in the present study or in Rhizobium. Stirk et al. (Citation2003, Citation2009) observed only low levels of O-glucosides in microalgae and seaweeds. O-glucosides are considered to be the hormone storage forms, thought to function in higher plants as a slow-release reserve refilling the active aglycons, thereby ensuring a continuous supply of free CKs over a prolonged period of time (Van Staden & Drewes, Citation1991; Strnad, Citation1997). Ordog et al. (Citation2004) and Stirk et al. (Citation2003) suggested that because O-glucosides are resistant to degradation by CK oxidase, they may function to ensure the presence of free active CKs in microalgae where CK oxidase activity is high. However, there is evidence supporting the existence of a CK biosynthetic pathway whereby adenine nucleoside phosphorylase is able to hydrolyse CK nucleotides to free bases (Chen, Citation1997; Sakakibara, Citation2006). As a consequence, glucosylation is not a mechanism used in algae for the regulation of the concentration of active CK forms, unlike in vascular plants (Stirk & Van Staden, Citation2010; Spichal, Citation2012). The existence of such an alternative pathway is consistent with undetectable levels of CK O-glucosides reported in this study. Additionally, a significant ABA presence was observed in the E. gracilis pellet and supernatant samples. Relatively high levels of ABA were detected in the spent media, as compared with most of the tested CK derivatives, with the only exception being methylthiols. The observed specific ABA distribution between Euglena cells and the slightly alkaline growth medium (pH 7.5) is in accordance with the previous report, that ABA transport in vascular plants is regulated by apoplastic pH of the leaf and stem and its flow in the plant intensifies with the increasing pH of the tissue (Jiang & Hartung, Citation2008).
In conclusion, in our work we determined the optimal concentrations for a wide spectrum of exogenous phytohormones which would stimulate cell division and produce maximum cell concentrations of Euglena gracilis in a culture medium. The results of the two hormone assays suggest that plant hormones may act alone and in combinations to promote algal growth. High concentrations of exogenous phytohormones were phytotoxic to Euglena, suppressing cell division and making cells spherical in shape. Additionally, some of the tested phytohormones added to Euglena cultures resulted in dense, dark green chloroplasts, suggesting that exogenous phytohormones increase chlorophyll production. This results in an increased production of energy and cell metabolites that may be used for cell division, and the synthesis and enhancement of cell structures such as the cell membrane. Further evidence for the integral involvement of CKs in algal physiology is provided by the endogenous CKs profiles in Euglena gracilis, which consisted mainly of cZ and to a lesser extent, iP or their derivatives. Moreover, detectable levels of ABA were present in E. gracilis; cultures treated with exogenous ABA formed the highest cell densities, suggesting that ABA plays an important role in alleviating stress of in vitro culture and thus may promote algal cell proliferation. In light of previous CK and ABA studies, the results presented here emphasize the importance of studying hormones in combination.
References
- Auer, C.A. (1997). Cytokinin conjugation: recent advances and patterns in plant evolution. Plant Growth Regulation, 23: 17–32.
- Bensen, R.J., Boyer, J.S. & Mullet, J.E. (1988). Water deficit induced changes in abscisic acid, growth, polysomes and translatable RNA in soybean hypocotyls. Plant Physiology, 88: 289–294.
- Bradley, P.M. (1991). Plant hormones do have a role in controlling growth and development of algae. Journal of Phycology, 27: 317–321.
- Buczek, J., Kubik-Dovosz, G. & Tatkowska, E. (1975). The influence of gibberellic acid and kinetin on the growth of Scenedesmus quadriqauda (Turp.). Breb. Acta Societatis Botanicorum Poloniae, 44: 415–421.
- Burkiewicz, K. (1987). The influence of gibberellins and cytokinins on the growth of some unicellular Baltic algae. Botanica Marina, 30: 63–69.
- Burkle, L., Cedzich, A., Dopke, C., Stransky, H., Okumoto, S., Gillissen, B., Kuhn, C. & Frommer, W.B. (2003). Transport of cytokinins mediated by purine transporters of the PUP family expressed in phloem, hydathodes, and pollen of Arabidopsis. The Plant Journal, 34: 13–26.
- Buschmann, C. & Lichtenthaler, H.K. (1982). The effect of cytokinins on growth and pigment accumulation of radish seedlings (Raphanus sativus L.) grown in the dark and at different light quanta fluence rates. Photochemistry and Photobiology, 35: 217–221.
- Carimi, F., Terzi, M., Michele, R.D., Zottini, M. & Schiavo, F.L. (2004). High levels of the cytokinin BAP induce PCD by accelerating senescence. Plant Science, 166: 963–969.
- Chen, C.M. (1997). Cytokinin biosynthesis and interconversion. Physiologia Plantarum, 101: 665–673.
- Cohen, A.C., Bottini, R. & Piccoli, P.N. (2008). Azospirillum brasilense Sp 245 produces ABA in chemically-defined culture medium and increases ABA content in arabidopsis plants. Plant Growth Regulation, 54: 97–103.
- Creelman, R.A. & Zeevaart, J. (1985). Abscisic acid accumulation in spinach leaf slices in the presence of penetrating and nonpenetrating solutes. Plant Physiology, 77: 25–28.
- Czerpak, R., Krotke, A. & Mical, A.H. (1999). Comparison of stimulatory effect of auxins and cytokinins on protein, saccharides and chlorophylls content in Chlorella pyrenoidosa Chick. Polskie Archiwum Hydrobiologii, 46: 71–82.
- De Nys, R., Jameson, P.E., Chin, N., Brown, M.T. & Sanderson, K.J. (1990). The cytokinins as endogenous growth regulators in Macrocystis pyrifera (L.) C. Ag. (Phaeophyceae). Botanica Marina, 33: 467–475.
- Efetova, M., Zeier, J., Riederer M., Lee, C.-W., Stingl, N., Mueller, M., Hartung, W., Hedrich, R. & Deeken, R. (2007). A central role of abscisic acid in drought stress protection of Agrobacterium-induced tumors on Arabidopsis. Plant Physiology, 145: 853–862.
- Emery, R.J.N., Leport, L., Barton, J. E., Turner, N.C. & Atkins, C.A. (1998). Cis-isomers of cytokinins predominate in chickpea seeds throughout their development. Plant Physiology, 117: 1515–1523.
- Farrow, S.C. & Emery, R.J.N. (2012). Concurrent profiling of indole-3-acetic acid, abscisic acid, and cytokinins and structurally related purines by high-performance-liquid-chromatography tandem electrospray mass spectrometry. Plant Methods, 8: 42–59.
- Gajdosova, S., Kaminek, M., Hoyerova, K., Dobrev, P.I., Gaudinova, A., Klima, P., Zizkova, E. & Motyka, V. (2009). Contribution to metabolic studies of cis-zeatin-type cytokinin with so far unknown function. FEBS Journal, 276: 196–197.
- Gajdosova, S., Spichal, L., Kaminek, M., Hoyerova K., Novak, O., Dobrev, P.I., Galuszka, P., Klima, P., Gaudinova, A., Zizkova, E., Hanus, J., Dancak, M., Travnicek, B., Pesek, B., Krupicka M., Vankova, R., Strnad, M. & Motyka, V. (2011). Distribution, biological activities, metabolism, and the conceivable function of cis-zeatin-type cytokinins in plants. Journal of Experimental Botany, 62: 2827–2840.
- Gupta, V., Kumar, M., Brahmbhatt, H., Reddy, C.R.K., Seth, A. & Jha, B. (2011). Simultaneous determination of different endogenetic plant growth regulators in common green seaweeds using dispersive liquid-liquid microextraction method. Plant Physiology and Biochemistry, 49: 1259–1263.
- Hirose, N., Takei, K., Kuroha, T., Kamada-Nobusada T., Hayashi, H. & Sakakibara H. (2008). Regulation of cytokinin biosynthesis, compartmentalization and translocation. Journal of Experimental Botany, 59: 75–83.
- Hirsch, R., Hartung, W. & Glimmler, H. (1989). Abscisic acid content of algae under stress. Botanica Acta, 102: 326–334.
- Huang, C.Y. (1991). Regulation of ionic fluxes and protein-release from Anabaena HA101 by exogenous abscisic acid. Botanical Bulletin of Academia Sinica, 32: 265–270.
- Ivic, S.D., Sicher, R.C. & Smigocki, A.C. (2001). Growth habit and sugar accumulation in sugarbeet (Beta vulgaris L.) transformed with cytokinin biosynthesis gene. Plant Cell Reports, 20: 770–773.
- Jennings, R.C. (1968). Gibberellins as endogenous growth regulators in green and brown algae. Planta (Berlin), 80: 34–42.
- Jennings, R.C., Broughton, W.J. & McComb, A.J. (1972). Effect of kinetin on the phycoerythrin and chlorophyll content of red algae. Phytochemistry, 11: 1937–1943.
- Jiang, F. & Hartung, W. (2008). Long-distance signalling of abscisic acid (ABA): the factors regulating the intensity of the ABA signal. Journal of Experimental Botany, 59: 37–43.
- Kisiala, A., Laffont, C., Emery, R.J.N. & Frugier, F. (2013). Bioactive cytokinins are selectively secreted by Sinorhizobium meliloti nodulating and nonnodulating strains. Molecular Plant Microbe Interactions, 26: 1225–1231.
- LaRosa, P.C., Handa, A.K., Hasegawa, P.M. & Bressan, R.A. (1985). Abscisic acid accelerates adaptation of cultured tobacco cells to salts. Plant Physiology, 79: 138–142.
- Li, Y.J., Liu, S.F. & Li, M. (2002). Influences of three plant growth hormones on growth of Amphora coffeaeformis and Navichla corymbosa. Journal of Fishery Sciences of China, 9: 18–22.
- Martin, R.C., Mok, M.C., Habben, J.E. & Mok, D.W.S. (2001). A maize cytokinin gene encoding an O-glucosyltransferase specific to cis-zeatin. Proceedings of the National Academy of Sciences USA, 98: 5922–5926.
- Mauch-Mani, B. & Mauch, F. (2005). The role of abscisic acid in plant-pathogen interactions. Current Opinion in Plant Biology, 8: 409–414.
- Mekhalfi, M., Avilan, L., Leburn, R., Botebol, H. & Gontero, B. (2012). Consequences of presence of 24-epibrassinolide, on cultures of a diatom, Asterionella formosa. Biochimie, 94: 1213–1220.
- Mok, M.C. (1994). Cytokinins and plant development – an overview. In Cytokinins: Chemistry, Activity and Function (Mok, D.W.S. & Mok, M.C., editors), 155–161. CRC Press, Boca Raton, FL.
- Nakashima, K. & Yamaguchi-Shinozaki, K. (2013). ABA signaling in stress-response and seed development. Plant Cell Reports, 32: 959–970.
- Ordog, V., Stirk, W.A., Van Staden, J., Novak, O. & Strnad, M. (2004). Endogenous cytokinins in three genera of microalgae from the Chlorophyta. Journal of Phycology, 40: 88–95.
- Pan, X., Chang, F., Kang, L., Liu, Y., Li, G. & Li, D. (2008). Effects of gibberellin GA3 on growth and microcystin production in Microcystis aeruginosa (Cyanophyta). Journal of Plant Physiology, 165: 1691–1697.
- Pedersen, M. (1973). Identification of a cytokinin 6-(3-methyl-2-butenylamino)purine, in seawater and the effects of cytokinins on brown algae. Physiologia Plantarum, 28: 101–105.
- Piotrowska, A. & Czerpak, R. (2009). Cellular response of light/dark-grown green alga Chlorella vulgaris Beijerinck (Chlorophyceae) to exogenous adenine- and phenylurea-type cytokinins. Acta Physiologiae Plantarum, 31: 573–585.
- Quesnelle, P.E. & Emery, R.J.N. (2007). Cis-cytokinins that predominate in Pisum sativum during early embryogenesis will accelerate embryo growth in vitro. Canadian Journal of Botany, 85: 91–103.
- Romanov, G.A., Lomin, S.N. & Schmulling, T. (2006). Biochemical characteristics and ligand-binding properties of Arabidopsis cytokinin receptor AHK3 compared to CRE1/AHK4 as revealed by a direct binding assay. Journal of Experimental Botany, 57: 4051–4058.
- Sakakibara, H. (2006). Cytokinins: activity, biosynthesis, translocation. Annual Review of Plant Biology, 57: 431–449.
- Santner, A., Calderon-Villalobos, L.I.A. & Estelle, M. (2009). Plant hormones are versatile chemical regulators of plant growth. Nature Chemical Biology, 5: 301–307.
- Shi, C.Y., Cai, W.R., Gan, X.H., Wang, B.F., Zhao, L.X. & Tang, X.Y. (2004). Effects of six plant growth regulators on the growth of Spirulina platensis. Journal of Anhui Agricultural University, 31: 26–29.
- Spichal, L. (2012). Cytokinins – recent news and views of evolutionally old molecules. Functional Plant Biology, 39: 267–284.
- Spichal, L., Rakova, N.Y., Riefler, M., Mizuno, T., Romanov, G.A., Strnad, M. & Schmulling, T. (2004). Two cytokinin receptors of Arabidopsis thaliana, CRE/AHK4 and AHK3 differ in their ligand specificity in a bacterial assay. Plant Cell Physiology, 45: 1299–1305.
- Stewart, C.R., Voetberg, G. & Rayapati, P.J. (1986). The effects of benzyladenine, cycloheximide, and cordycepin, on wilting-induced abscisic acid and proline accumulation and abscisic acid- and salt-induced proline accumulation in barley leaves. Plant Physiology, 82: 703–707.
- Stirk, W.A. & Van Staden, J. (2010). Flow of cytokinins through the environment. Plant Growth Regulation, 62: 101–116.
- Stirk, W.A., Ordog, V. & Van Staden, J. (1999). Identification of the cytokinin isopentenyladenine in a strain of Arthronema africanum (Cyanobacteria). Journal of Phycology, 35: 89–92.
- Stirk, W.A., Novak, O., Strnad, M. & Van Staden, J. (2003). Cytokinins in macroalgae. Plant Growth Regulation, 41: 13–24.
- Stirk, W.A, Novak, O., Hradecka, V., Pencik, A., Rolcik, J., Strnad, M. & Van Staden, J. (2009). Endogenous cytokinins, auxins and abscisic acid in Ulva fasciata (Chlorophyta) and Dictyota humifusa (Phaeophyta): towards understanding their biosynthesis and homoeostasis. European Journal of Phycology, 44: 231–240.
- Stirk, W.A., Van Staden, J., Novak, O., Dolezal, K., Strnad, M., Dobrev, P.I., Sipos, G., Ordog, V. & Balint, P. (2011). Changes in endogenous cytokinin concentrations in Chlorella (Chlorophyceae) in relation to light and the cell cycle. Journal of Phycology, 47: 291–301.
- Strnad, M. (1997). The aromatic cytokinins. Physiologia Plantarum, 101: 674–688.
- Synkova, H., Semoradova, S. & Bjurketova, L. (2004). High content of endogenous cytokinins stimulates activity of enzymes and proteins involved in stress response in Nicotiana tabacum. Plant Cell Tissue and Organ Culture, 79: 169–179.
- Tatkowska, E. & Buczek, J. (1980). Effect of phytohormones on the growth of Scenedesmus quadricauda (Turp.) Breb. Acta Societatis Botanicorum Poloniae, 49: 211–220.
- Tarakhovskaya, E.R., Maslov, Y.I. & Shishova, M.F. (2007). Phytohormones in algae. Russian Journal of Plant Physiology, 54: 163–170.
- Tuteja, N. (2007). Abscisic acid and abiotic stress signaling. Plant Signaling and Behavior, 2: 163–170.
- Van Staden, J. & Drewes, F.E. (1991). The biological activity of cytokinin derivatives in the soybean callus bioassay. Plant Growth Regulation, 10: 109–115.
- Yang, Q.Y., Shi, Q.Q., Chen, B.L. & Wu, S.G. (2000). Study on advancing the growth rate of Pavlova virdis Tseng with plant hormone. Journal of Fujian Teachers University (Natural Science), 16: 80–83.
- Zeevaart, J.A.D. & Creelman, R. (1988). Metabolism and physiology of abscisic acid. Annual Review of Plant Physiology and Plant Molecular Biology, 39: 439–473.