Abstract
This paper presents a new biotic index (ESCA, Ecological Status of Coralligenous Assemblages), based on analyses of in situ records of coralligenous macroalgal assemblages in the Mediterranean Sea. The ESCA index was developed on the basis of previous impact evaluation studies carried out by traditional destructive methods. It was validated on an independent dataset collected during a 3-year study carried out at five localities in the north-western Mediterranean, and tested on a gradient of anthropogenic stressors. Assemblage descriptors selected as metrics of the ESCA index were: (a) presence/absence and abundance of sensitive taxa/groups (expressed as sensitivity level of assemblages, SL), (b) diversity of assemblages (expressed as α-diversity) and (c) heterogeneity of assemblages (expressed as β-diversity). The three metrics were combined to give a final value for the multimetric ecological index, Ecological Quality Ratio, calculated as the ratio between the measured values and the value obtained in the reference condition. The Ecological Quality Ratio values ranged from 0 to 1 and five ecological status classes were defined in accordance with the Water Framework Directive. The ESCA index could be used to define the ecological status of the studied sites, providing differences between assemblages similar to those shown by multivariate analyses. Moreover, ecological quality values were quite steady throughout the study period. The ESCA index may represent a useful tool for monitoring programmes in coastal waters, since it has been developed in compliance with the Water Framework Directive; it synthesizes complex information in a simple and reliable way; and its application is simple and cost-effective. However, further studies are necessary to complete index development and to properly evaluate its strengths and weaknesses on a larger scale.
Introduction
Achieving and maintaining good status for marine coastal waters are important goals of current European legislation, which promotes the conservation of aquatic systems and the development of management strategies for sustainable use of water resources through the enforcement of the Water Framework Directive (WFD; EC, Citation2000) and the Marine Strategy Framework Directive (MSFD; EC, Citation2008). Thus, tools to assess the ecological quality status of water bodies (a discrete and significant element of coastal waters such as a stretch of coastline identified according to Annex II of the WFD) and the environmental status of marine regions (a sea region identified under Article 4 of the MSFD) are fundamental for achieving environmental targets and to protect marine waters from further deterioration. In this context, the strategies adopted require studies focused on the identification of bioindicators and biotic indices suitable for assessing the ecological quality of coastal aquatic ecosystems (Borja, Citation2005; Borja et al., Citation2010; Martinez-Crego et al., Citation2010).
Several bio-assessment tools have been developed or adapted for WFD requirements using different biological quality elements, such as phytoplankton, macroalgae, seagrasses and macro-zoobenthos (Birk et al., Citation2012). Macroalgae have been indicated as a biological quality element (BQE) for coastal rocky bottoms (WFD Annex V; EC, Citation2000), since macroalgal assemblages are widely recognized as a good ecological indicator for monitoring surveys and impact evaluation studies (Arevalo et al., Citation2007; Krause-Jensen et al., Citation2007; Pinedo et al., Citation2007; Scanlan et al., Citation2007; Wells et al., Citation2007; Juanes et al., Citation2008; Guinda et al., Citation2008; Díez et al., Citation2012; Neto et al., Citation2012). In the Mediterranean ecoregion, two different biotic indices based on macroalgal assemblages have been adopted to assess the ecological quality status of water bodies under WFD: the CARLIT index (cartography of littoral rocky shore communities: Ballesteros et al., Citation2007; Mangialajo et al., Citation2007) and the EEI (Ecological Evaluation Index: Orfanidis et al., Citation2001, Citation2003, Citation2011; Panayotidis et al., Citation2004). Both indices refer to the photophilic assemblages of the upper subtidal zone (0–3.5 m) and have recently been successfully intercalibrated to classify the generic ‘rocky shore coast’ water body (EC, Citation2013), without any distinction between different types of habitats (e.g. shallow and deep subtidal rocky habitats).
However, this approach could in some cases lead to questionable classification of rocky shore coasts. In fact, assessment based on upper subtidal assemblages could be very useful for detecting some kinds of human pressures, as these assemblages are more sensitive to the effects of pollutants flowing near the sea surface in desalinated water plumes (Soltan et al., Citation2001). Nevertheless, some important pressures like urban and industrial discharges from submarine outfalls, effluent discharges from mariculture cages, trawling, sediment removal, boats and diving act directly on deep subtidal rocky habitats (Aguado-Gimenez & Ruiz-Fernandez, Citation2012). Each water body type may contain several habitats that are exposed to specific physical and chemical factors, are characterized by specific biological assemblages and are often subjected to different kinds of human pressures. Consequently, biological monitoring methods and defined indices of change are generally habitat-specific. An assessment carried out on only one habitat may indicate the whole water body is of one quality status when in fact the majority of the water body, consisting of other habitats, may be of a different quality status (Borja et al., Citation2010). Therefore, to better define the real ecological quality status of the water body, it might be useful to compare the quality status of different habitats in the same rocky shore coastal water body.
Existing literature does not provide for indices based on macroalgal communities of the Mediterranean deep subtidal rocky habitat. In fact, Mediterranean deep subtidal rocky bottoms (from 20 to 120 m; Ballesteros, Citation2006) are characterized by the ‘coralligenous’ habitat, consisting of calcareous structures mainly built by Rhodophyta belonging to the Corallinales (Ballesteros, Citation2006). Coralligenous assemblages show a stratified structure with encrusting Corallinales colonized by prostrate and erect perennial algae, characterized by high biodiversity (Ballesteros, Citation2006). Due to its extent, species diversity, productivity and role played in the carbonate cycle, this ecosystem is recognized as one of the most important habitats of the Mediterranean Sea (Laborel, Citation1961, Citation1987; Ballesteros, Citation2006; UNEP, Citation2007; Piazzi et al., Citation2010). Despite this, coralligenous assemblages are largely neglected in the water body quality assessment of the WFD, since the directive focuses on a single BQE evaluation, not directly requiring an assessment of marine habitats (deconstruction/structural approach). By contrast, the MSFD does not focus on biotic components and requires the assessment of marine habitat types (holistic/functional approach) (Borja et al., Citation2010). In particular, the MSFD requires the assessment of ‘special habitat types’, especially those identified under Community legislation (e.g. Habitats Directive 92/43/CEE) or international conventions (e.g. Barcelona 1995) as being of special scientific or biodiversity interest (Annex III, EC, Citation2008; CSWP, EC, Citation2011; Borja et al., Citation2010; Deter et al., Citation2012a). Thus, coralligenous assemblages should be monitored as descriptors, so development of tools to evaluate the coralligenous habitat status could be important for implementing the European Framework Directives.
Coralligenous assemblages may represent a suitable indicator of anthropogenic impacts as they are sensitive to these pressures (Hong, Citation1983; Cinelli et al., Citation2007; Piazzi et al., Citation2012). Compared with other biological elements like Posidonia oceanica or soft-bottom benthic invertebrates, which have long been used in monitoring programmes, little is known about effects of human impacts on coralligenous assemblages. However, some recent studies have provided useful indications of possible candidate metrics for assessing the ecological status of coralligenous assemblages (Balata et al., Citation2005, Citation2007a, b; Piazzi et al., Citation2007, Citation2011, Citation2012; Gennaro & Piazzi, Citation2011).
Modifications of assemblages subjected to anthropogenic stressors include reduction in species richness (Balata et al., Citation2007a; Piazzi et al., Citation2007) and disappearance or decrease of the most sensitive species with an increase in tolerant and opportunistic species (Piazzi et al., Citation2012). Moreover, in the stressed areas, the spatial heterogeneity (β-diversity) normally characterizing coralligenous assemblages is strongly reduced (Balata et al., Citation2007b; Piazzi et al., Citation2011).
To date, only two methods have been proposed for evaluating the ecological quality of coralligenous assemblages: the Coralligenous Assemblage Index (CAI) (Deter et al., Citation2012a) and the Rapid Visual Assessment technique (RVA) (Gatti et al., Citation2012). The CAI index is based on per cent cover of bryozoa, sludge and builder species obtained through photographic samples (Deter et al., Citation2012a), while the RVA integrates biological, ecological and geomorphological information obtained using visual census methods (Gatti et al., Citation2012). Thus, both methods are based on a holistic approach and do not provide for structural and functional analysis of assemblages considered as a single BQE, as required by WFD. However, in line with the spirit of the recent European Framework Directives, both methods follow a non-destructive approach and were recently tested to assess the ecological quality status of coastal waters.
The aim of the present paper is to present and describe a newly proposed methodology for assessing the Ecological Status of Coralligenous Assemblages (ESCA), based on the analysis of this Mediterranean macroalgal community, using non-destructive methodology. Taking an approach similar to that used for macroalgal assemblages in shallow waters (Ballesteros et al., Citation2007; Pinedo et al., Citation2007), development of the ESCA index started from a dataset obtained by making traditional, destructive sample collections, which was used to select and test suitable descriptors for macroalgal coralligenous assemblages. Then, we developed our photographic method for ESCA by adjusting the metrics obtained from traditional destructive methods to the visual census approach.
Thus, the development of the ESCA multimetric index can be synthesized as the following steps: (i) building a development (calibration) dataset based on traditional destructive samples; (ii) selection and testing of a candidate metric, according to a mixed protocol of metric selection criteria providing for an ‘a priori’ selection and multivariate analysis comparing pristine, intermediate and strongly degraded conditions; (iii) adjustment of metrics from the destructive methods to the visual census approach of non-destructive samples; (iv) metric combination and formulation of the multimetric ESCA index; (v) first validation of the method using an independent dataset separate from the index development dataset. These steps are in accordance with general guidelines (Borja & Dauer, Citation2008; Borja et al., Citation2011), although the scarcity of data on macroalgal coralligenous assemblages and the introduction of the visual census approach required some additional and/or modified steps.
Finally, the ESCA index was tested against a gradient of anthropogenic stressors to investigate the nature of the pressure–index relationship.
Materials and methods
Building a dataset for development of the index
The dataset was assembled from data collected within research programmes aiming to evaluate anthropogenic impacts on sublittoral macroalgal coralligenous assemblages in the Tuscany region of the north-western Mediterranean Sea (Balata et al., Citation2005, Citation2007a, b; Piazzi et al., Citation2007, Citation2011, Citation2012; Gennaro & Piazzi, Citation2011).
These data represented the development or calibration dataset (Borja & Dauer Citation2008). Three different pressure indicators (stressors) were selected: biological invasions by Caulerpa racemosa (Forsskål) C. Agardh var. cylindracea (Sonder) Verlaque, Huisman et Boudouresque; increased sedimentation; and nutrient enrichment. For each pressure indicator, three different levels of impact were considered (pristine, intermediate impact and strong impact; ). Sedimentation rate was expressed as g m−2 day−1, nutrient concentration as μmol l−1 and C. racemosa abundance as gdw m−2.
Table 1. Typology and levels of stressors for each environmental condition (Pristine, Intermediate impact and Strong impact). Pristine conditions are considered as Reference Conditions (RC) for the geographical area. Values of nutrient concentration refer to nitrogen + phosphorus.
As part of the above-mentioned studies, different conditions were experimentally obtained in the field by adding nutrients and sediment and by transplanting C. racemosa into experimental quadrats. In order to avoid interactions between different stressors, locations characterized by absence of the stressor or low stress were chosen for the experiments. These manipulative studies were carried out over a one-year period at rocky offshore shoals off south Livorno () where the horizontal bottom is between 25 and 35 m deep. Manipulative treatments and sample collections were carried out by SCUBA divers. For each of the experimental conditions, two sites were chosen hundreds of metres apart and at each site two 25 m2 areas were selected tens of metres apart, taking into account the main scales of spatial variation of the studied system (Balata et al., Citation2006b). In each area, three quadrats of 400 cm2 surface were selected for manipulative treatments. At the end of the study period, all quadrat surfaces were sampled by removing encrusting Corallinales and their epiphytes using a hammer and a chisel, in order to collect all sessile organisms, and material collected from each quadrat was preserved in 4% formalin seawater. In the laboratory, sampling quadrats were reconstructed and species composition and abundance of macroalgae were determined; abundance was evaluated as the orthogonal projection of each species and expressed as percentage coverage of the 400 cm2 quadrat (Boudouresque, Citation1971).
Fig. 1. Map of Tuscany (inset: position in Italy) with stars indicating the study sites. Dashed line represents the −50 m contour. The arrow indicates the location of experimental studies.
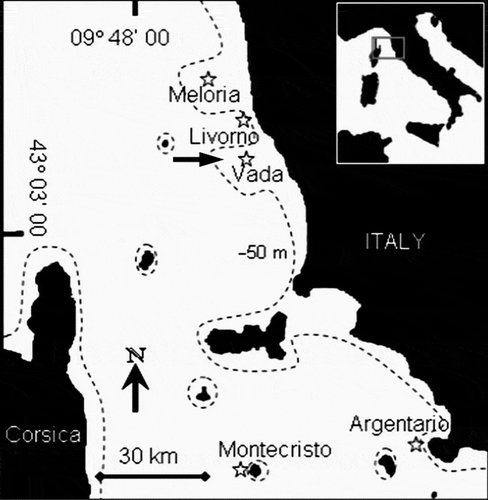
Experimental enhancement of sedimentation consisted of adding 400 g or 150 g of fine grain-size (<200 μm) terrestrial sediment every 45 days to each experimental quadrat, throughout the study period. This corresponded to a mean input of 220 and 83 g m−2 day−1 respectively (Balata et al., Citation2007a, b).
Fragments of C. racemosa 12 cm in length, collected in adjacent patches inside the study site, were anchored in each experimental plot within 1 h of collection using two wire bridges fixed to the substratum by means of 15 cm long nails (Gennaro & Piazzi, Citation2011). Either 15 or 30 fragments were transplanted in different experimental conditions.
In nutrient-enriched quadrats, a nylon-mesh bag containing 40 g or 200 g fertilizing pellets (Osmocote®) was placed along two opposite sides of each 20 × 20 cm quadrat and periodically replaced with new ones in order to maintain a constant concentration of nutrients in the water throughout the study period (Gennaro & Piazzi, Citation2011; Piazzi et al., Citation2012). On three random sampling dates, two replicate water samples were taken by SCUBA divers over the treated quadrats using 60 ml sterile syringes and analysed by a continuous-flow Autoanalyzer (Bran & Luebbe AA3; Germany) to check levels of nutrient enrichment.
Selecting and testing candidate metrics
Based on results of previous investigations (Balata et al., Citation2005, Citation2007a, b; Piazzi et al., Citation2007, Citation2011, Citation2012; Gennaro & Piazzi, Citation2011) three descriptors of changes in coralligenous assemblages were selected according to a priori selection criteria (Borja & Dauer, Citation2008): (a) presence/absence and abundance of sensitive taxa/groups, (b) diversity of assemblages and (c) heterogeneity of assemblages. These descriptors were in accordance with the WFD requirements for BQE Macroalgae (Annex V; EC, Citation2000); they were easy to measure and ecologically relevant, so they were chosen as candidate metrics for the ESCA index.
Presence/absence and abundance of sensitive taxa are ‘autecology-based metrics’ in which taxa are characterized by sensitivity to disturbance. Response of these metrics to the anthropogenic stressors was tested by multivariate analysis in PERMANOVA (PRIMER v6 including the add-on package PERMANOVA plus; Anderson & Gorley, Citation2007). A 2-way model was performed with Condition (reference vs. intermediate impact vs. strong impact) as fixed factor and Site as random factor nested in Condition. A Bray–Curtis dissimilarity matrix was performed prior to analyses and Monte Carlo procedures were used when the number of permutations was low. A canonical analysis of principal coordinates (CAP) conducted on a log(x+1) transformed Bray–Curtis dissimilarity matrix (Anderson & Robinson, Citation2003; Anderson & Willis, Citation2003) was performed in order to discriminate the response of assemblage structure to different levels of stressors, highlighting species or taxa/groups as indicators accounting for such discrimination. Finally, a SIMPER analysis (Clarke, Citation1993) was performed to identify percentage contribution of each species to the Bray–Curtis dissimilarity among conditions.
Diversity of assemblages is a ‘taxonomy-based metric’ (richness metric) that is very common in ecological studies and widely shared among existing assessment methods (Birk et al., Citation2012). It was expressed as ‘α-diversity’, evaluated as number of species per sample (species richness, S), and the response of this metric to the considered environmental stress was tested as differences among samples exposed to minor, intermediate and strong stress by means of PERMANOVA analysis.
Heterogeneity of assemblages is a taxonomy-based metric based on a multivariate approach (Birk et al., Citation2012) that has been proposed as a possible descriptor of changes in macroalgal coralligenous assemblages in response to different ecological stresses (Balata et al., Citation2007a; Piazzi & Balata, Citation2008). It was expressed as ‘β-diversity’ evaluated through permutational dispersion multivariate analysis (PERMDISP) (PRIMER v6 including PERMANOVA plus) performed on the available dataset. The PERMDISP analysis evaluates the variability of species composition among sampling units (heterogeneity of assemblages) as multivariate dispersion calculated on the basis of distance from centroids (Anderson, Citation2006). Thus, any changes in compositional variability displayed by PERMDISP may be directly interpretable as changes in β-diversity (Anderson et al., Citation2006).
Conversion from traditional destructive sampling to a photographic approach
The ESCA index is a photographic method, so metrics selected by analysing the destructive samples were reconsidered on the basis of the characteristics of photographic samples (i.e. low species detection power) before combining them in the multimetric index. Some macroalgal species can usually be easily recognized visually in both destructive and photographic samples, by unique thallus features, while other species are morphologically very similar, preventing their identification in photographic samples. Therefore, in order to switch from the traditional destructive approach to the photographic method without losing information, taxa that are not easily recognizable were placed into morphological groups, on the basis of both available literature (Balata et al., Citation2011) and results of multivariate analysis (CAP). In fact, CAP analysis performed on the composition/abundance matrices obtained from destructive samples provides the response of assemblages to different stressors and the species responsible for the response, at the same time. Thus, morphological groups of different species which shared the same thallus form and ecological response to stress were proposed.
The first autecology-based metric selected for the ESCA index is a Sensitivity Level (SL) value, in an approach similar to that reported for the evaluation of shallower subtidal assemblages by the CARLIT index (Ballesteros et al., Citation2007). A SL value was assigned to each of these taxa/groups on the basis of the results provided by CAP analyses, SIMPER test and values of the mean percentage cover of the taxa/groups obtained from destructive samples collected at pristine and impacted sites. Since abundance of each taxon/group changes depending on level of impact (pristine, intermediate or strong), range-values of abundance variation for each taxa/group were defined. These values represent the response of each taxa/group to level of stressor (absent, intermediate and strong) and were expressed as mean per cent cover calculated from replicate samples collected for each condition.
For the calculation of the ESCA index, SL of a study site was obtained by adding all values of SL reported for taxa/groups observed in each photographic sample. α-diversity was defined as the mean number of the main taxa/groups obtained in each photographic sample. β-diversity was evaluated as the mean distance of all photographic samples from centroids calculated in the PERMDISP analysis.
Sampling methods and image analyses
Sampling was conducted on vertical substrata at 30–35 m depth. Both depth and vertical substrata were chosen for several reasons: in the geographical area considered, coralligenous assemblages develop mainly on vertical substrata below 25–30 m, but rocky cliffs normally stop between 35 and 40 m, while on horizontal substrata coralligenous habitat may start to develop at greater depth, depending on water transparency. Depths chosen for sampling were considered suitable for observing shifts in the structure of macroalgal assemblages subject to different environmental conditions (Piazzi & Balata, Citation2011).
In order to take into account the main spatial scales of variability of Tuscan coralligenous assemblages (Piazzi et al., Citation2004; Balata et al., Citation2005), at each studied site, two areas hundreds of metres apart were selected and 30 photographic samples were collected in each area, using an underwater camera fitted with a frame (Acunto et al., Citation2001) suitable for photographing surfaces of 1875 cm2 (50 cm × 37.5 cm). Sampling surface and number of replicates were chosen according to pilot studies (Acunto, Citation2000; Acunto et al., Citation2001). In each area the total investigated surface was larger than that suggested by previous investigations (Kipson et al., Citation2011; Teixido et al., Citation2013). Each photographic sample was analysed with the image processing program ‘Image J’ which allowed evaluation of the percentage cover of the main taxa and/or morphological groups of animals and macroalgae (Balata et al., Citation2011); for the index calculation, only percentage cover of macroalgae was considered.
Combination of metrics and formulation of the ESCA multimetric index
According to the WFD, ecological status must be defined as an Ecological Quality Ratio (EQR) value obtained from the ratio between the value of the metrics/index calculated for the studied site (Ecological Quality Value, EQV) and the same value obtained for the Reference Conditions. Thus, for a given study site the EQV of each assemblage descriptor was calculated (EQVSL, EQVα, EQVβ) and the Ecological Quality Ratio (EQRSL, EQRα, EQRβ) was obtained as the ratio between these values and the value of EQV obtained for the same descriptors in the Reference Conditions.
Choice of Reference Conditions (RC) to calculate the EQR values of the ESCA index was spatially based (Annex II; EC, Citation2000) and referred to ‘true’ RC (Annex III; EC, Citation2010) Montecristo Island (Tuscan Archipelago National Park). In fact, Montecristo Island was selected as Reference site both in the National RC setting and common benchmarking setting for the Mediterranean intercalibration (GIG, Citation2013). Datasets on reference assemblages, obtained in previous long-term monitoring studies carried out at Montecristo Island, were used to calculate values of the three index metrics in the reference sites for each year of study. The Ecological Quality Values of each metric in the reference conditions (EQViRC) was computed as the mean of EQViRC values measured in three different years at the reference site. Therefore, the index EQV reference value takes into account the natural temporal variability of the reference site, according to the WFD requirements.
The final value of EQR for the ESCA index was calculated as the mean of the three EQRi obtained for the assemblage descriptors ((EQRSL + EQRα + EQRβ)/3).
According to the WFD, values of the index should range from 0 to 1; five ecological status classes were defined on the basis of a statistical approach to boundary setting (Birk et al., Citation2012), as follows. We derived the high-good boundary from index variability at the reference site (so all RC variability was above the high-good boundary) then we divided the spectrum of impact below the high-good boundary into four classes. Values of boundaries below the high-good one were fixed on the basis of values of the ESCA index obtained from samples of the previously described experimental impact studies.
Spatio-temporal validation of the index: a case study
In accordance with recommendations by Borja & Dauer (Citation2008), a first validation of the ESCA index was carried out by testing the index using a dataset independent of the index development dataset. The ESCA index was applied to four sites located along the Tuscan coast: Meloria Shoals, Livorno, Vada Shoals and Monte Argentario (). Livorno is an urbanized area, while the other sites are areas marginally influenced by different impacts: an industrial port (Meloria Shoals), chemical plants (Vada Shoals) and fish farms (Monte Argentario). To evaluate ecological differences among the selected sites, values of dissolved nutrient concentrations in the water column (nitrite, nitrate, ammonium, orthophosphate, total nitrogen and phosphorus, expressed in µM) and total phytoplankton abundance (expressed as chlorophyll a values) were compared with values for the reference site. Data obtained in a 3-year monitoring survey (www.arpat.toscana.it) were used to calculate the mean values of each parameter at each site, confirming that the highest ecological quality was at Montecristo Island ().
Table 2. Mean values of nutrient and chlorophyll a (chl a) concentrations in the water column (micromolar, µM) obtained for the five selected sites over 3 years of monitoring survey. TDN = Total dissolved nitrogen, that is the sum of dissolved inorganic nitrogen (DIN = N-NH3+N-NO2−+N-NO3−) and dissolved organic nitrogen in the water column; TDP = Total dissolved phosphorus, that is the sum of the dissolved inorganic phosphorus (P-PO43−) and dissolved organic phosphorus; N-NH3 = ammonia; N-NO2− = nitrite; N-NO3− = nitrate.
The a priori selection of the Reference Conditions based on the MedGIG decision (GIG, Citation2013) was validated by multivariate analysis of variance based on permutations (PERMANOVA; Anderson, Citation2001) to test the following hypotheses: (i) reference assemblages differed from impacted ones in terms of composition and abundance of taxa/groups and in terms of α and β diversity; (ii) differences between assemblages varied between years, according to natural variability at the RC site. The analysis consisted of a 3-way model with Sites (fixed factor with 5 levels) and Year (random factor with 3 levels: 2008, 2009, 2010) as crossed factors and Area (random factor with 2 levels) nested in Site; 30 replicates were considered for each Area. The mean square of factor Site was partitioned into the non-orthogonal contrast reference vs. other sites (Terlizzi et al., Citation2005). PERMANOVA was conducted on a log(x+1) transformed Bray–Curtis dissimilarity matrix. The ESCA index was then calculated for each site and for each year of the study.
Test of the ESCA-stressors relationship
In order to evaluate the response to anthropogenic stressors, the ESCA index was applied to macroalgal assemblages subjected to different levels of pressure (biological invasions, increased sediments and nutrient enrichment). A regression was performed to test the relationship between each considered pressure and the ESCA index; the degree of correlation between the EQR of each studied area and the level of stressors was calculated and reported as the squared correlation coefficient (determination coefficient, R2). Significance was tested with Statistica 10 software.
Results
Selecting and testing candidate metrics
PERMANOVA analyses of species composition and abundance of macroalgal coralligenous assemblages showed significant differences between the reference and the stressed conditions ().
Table 3. Results of PERMANOVA analysis of species composition and abundance of macroalgal coralligenous assemblages. Significant effects are in bold. RC = reference conditions, I = intermediate impact, S = strong impact. C = fixed factor Condition; Site (C) = random factor Site nested in C; df = degrees of freedom; MS = Mean Squares; Pseudo-F = PERMANOVA F ratio associated with the general multivariate null hypothesis of no differences among groups; P(MC): P value of the pseudo-F statistic obtained with the Monte Carlo procedure.
CAP analyses showed a significant disjunction among different levels of stressors (P(perm)= 0.0001) for all the three pressure indicators considered, and the macroalgal species responsible for separation between stressed and pristine conditions seemed to be independent of the type of stressor (). The pristine condition was usually characterized by the green algae Halimeda tuna, Flabellia petiolata and Palmophyllum crassum, the brown alga Zanardinia typus and the red algae Meredithia microphylla, Peyssonnelia spp., Osmundea pelagosae, Laurencia chondrioides and Acrodiscus vidovichii. Nevertheless, the most sensitive species are H. tuna, P. crassum, Z. typus and O. pelagosae, which always decreased or disappeared in impacted areas. Flabellia petiolata, M. microphylla, L. chondrioides, A. vidovichii and Peyssonnelia spp. are also sensitive taxa decreasing in sites invaded or subjected to a high sedimentation rate (, ), while they were more tolerant to nutrient enrichment (). Stressed sites are mostly characterized by turfs, although some of the turf species can differ in relation to the type of stressor: the invasive alien red alga Womersleyella setacea and the native Antithamnion piliferum and the green alga Cladophora prolifera characterized all three stressed assemblages; the green alga Pseudochlodesmis furcellata was more abundant in areas with high nutrient concentration and sedimentation rate; and the Rhodophyta Heterosiphonia crispella, Polysiphonia furcellata and Polysiphonia subulifera were more abundant in high sedimentation rate areas (, ). Mesophyllum alternans, Lithothamnion philippii, Mesophyllum macroblastum, Lithophyllum pustulatum and Lithophyllum stictaeforme were the most common encrusting Corallinales in the study area: these species did not appear to be affected by the stressors tested.
Fig. 2. Canonical analysis of principal coordinates (CAP) showing the discriminant-type ordination of macroalgal samples subject to minor, intermediate and strong impact under three different stressors: biological invasion (a), sedimentation (b) and nutrient enrichment (c). Data are from destructive samples collected in the artificially manipulated habitats () after one year of exposure to the stressors. CAP1 and CAP2 are the first two principal axes that maximize the group differences among samples exposed to minor, intermediate and strong stress; superimposed vectors indicate macroalgal species that are more or less responsible for observed differences among groups of samples. Vectors correspond to Pearson correlations of individual species with the resulting CAP axes; direction and length of vectors indicate respectively the sign (increasing/decreasing) and strength (correlation value) of the relationship with the CAP axes. ○ reference conditions, ▼ intermediate impact, ● strong impact. A. vidovichii = Acrodiscus vidovichii, A. piliferum = Antithamnion piliferum, C. prolifera = Cladophora prolifera, F. petiolata = Flabellia petiolata, H. tuna = Halimeda tuna, H. crispella = Heterosiphonia crispella, L. chondrioides = Laurencia chondrioides, M. microphylla = Meredithia microphylla, O. pelagosae = Osmundea pelagosae, P. crassum = Palmophyllum crassum, Poly. furcellata = Polysiphonia furcellata, P. subulifera = Polysiphonia subulifera, P. furcellata = Pseudochlorodesmis furcellata, W. setacea = Womersleyella setacea, Z. typus = Zanardinia typus.
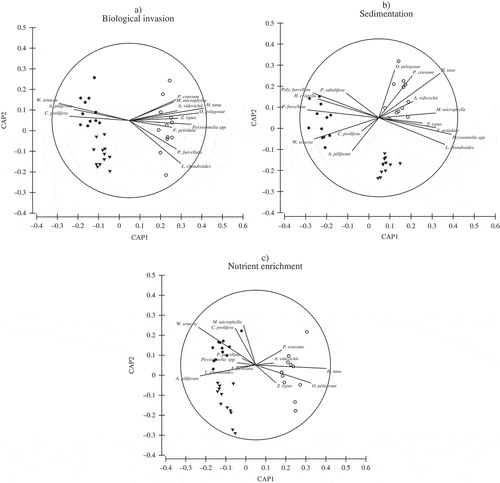
SIMPER tests confirmed the results of CAP analyses: Halimeda tuna, Flabellia petiolata, Zanardinia typus, Meredithia microphylla, Peyssonnelia spp., Osmundea pelagosae, Laurencia chondrioides, Womersleyella setacea, Acrothamnion preissii, Cladophora prolifera and Pseudochlorodesmis furcellata were the taxa contributing most to dissimilarity between reference and stressed conditions ().
Table 4. Results of SIMPER test showing dissimilarity between reference and stressed conditions and the percentage contribution of the main taxa that determine this dissimilarity. BioInv = Biological Invasion, SR = Sedimentation rate, NC = Nutrient concentration. diss = percentage dissimilarity.
Diversity of assemblages, expressed as the mean number of species per sample, was 36.75 in pristine conditions and decreased in all the stressed areas; the lowest values were observed in the invaded assemblages. PERMANOVA analyses showed significant differences between conditions for all the considered levels of stress (). Heterogeneity of assemblages tested by PERMDISP analyses showed significant differences between pristine and stressed conditions. Average distance from centroids decreased following the gradient of stress ().
Table 5. Diversity (α-diversity) and heterogeneity (β-diversity) of assemblages subjected to the three different stressors. Diversity was expressed as the mean value of species number calculated for all samples; heterogeneity was expressed as the mean distance from centroids of all samples calculated by PERMDISP analysis.
Conversion from traditional destructive sampling to a photographic approach
Macroalgal indicators detected by CAP analysis that are easy to identify in both destructive and photographic samples (the Chlorophyta Halimeda tuna, Flabellia petiolata, Palmophyllum crassum and Pseudochlodesmis furcellata, the red algae Peyssonnelia spp. and the brown alga Zanardinia typus) were considered as ‘taxa’. Species that are hardly recognizable visually were grouped in four morphological groups: ‘Algal turf’ (the Rhodophyta Womersleyella setacea, Heterosiphonia crispella, Anthithamnion piliferum, Polysiphonia furcellata and P. subulifera and the green alga Cladophora prolifera), ‘Erect corticated terete algae’ (the Rhodophyta Osmundea pelagosae and Laurencia chondrioides), ‘Flattened Rhodophyta with cortication’ (Meredithia microphylla and Acrodiscus vidovichi) and encrusting Corallinales (Mesophyllum alternans, Lithothamnion philippii, Mesophyllum macroblastum, Lithophyllum pustulatum and L. stictaeforme). To each of the taxa/groups and for each abundance range, a sensitivity level value was assigned according to a numerical scale ranging from +6 to −4, with the maximum values corresponding to the most sensitive species and the minimum values to the most tolerant ones. In the final list, each of the taxa/group indicators was listed multiple times, in order of its decreasing range of cover from the pristine to the strongly impacted samples and with the corresponding values of SL. Thus, the list showed macroalgal indicators in order of their ecological sensitivities, according to a decreasing gradient from high to low sensitivity to environmental disturbance ().
Table 6. Sensitivity levels (SL) of the main taxa/groups distinguished in the photographic samples in relation to their presence and abundance. Each of the taxa/group indicators is reported together with the range of variation of the percentage cover observed for a specific environmental condition (pristine, intermediate and strong impact) and with the corresponding sensitivity level value. The same taxon/group is listed multiple times from the top down, according to an increasing gradient of disturbance from the pristine group of samples to the strongly impacted ones.
Combination of metrics and formulation of the ESCA multimetric index
The application of the index to the reference site gave a mean value of 1.0±0.07 (mean±SD), which indicates a natural temporal variability of ESCA lower than 0.1.
The biological response of macroalgal assemblages to the different levels of the considered stressors (biological invasions, sediments increasing and nutrient enrichment in the Intermediate and Strongly impacted conditions) resulted in values of the ESCA index ranging between 0.76 and 0.62 in the areas subjected to the intermediate level of stressors and between 0.55 and 0.42 in the strongly stressed areas (). According to the natural variability of assemblages and taking account of their response to the pressures considered, the final boundaries of classes for the ESCA index were defined as: 1.0–0.81, High; 0.80–0.66, Good; 0.65–0.51, Moderate; 0.50–0.36, Poor; 0.35–0, Bad.
Table 7. Values of Ecological Quality Ratio of assemblage descriptors, calculated for different levels of the three stressors considered. EQRα = values of the diversity descriptor; EQRβ = values of the heterogeneity descriptor; EQRSL = values of the sensitivity level descriptor. RC = reference conditions; BioInv = Biological Invasion; SR = Sedimentation rate; NC = Nutrient concentration.
Spatial-temporal validation of the index: a case study
The studied assemblages were dominated by Peyssonnelia spp., encrusting Corallinales and turf; Pseudochlorodesmis furcellata, Palmophyllum crassum, Flabellia petiolata, Halimeda tuna, erect terete algae, flattened Rhodophyta, Zanardina typus and Dictyotales were also common at lower abundance. Erect corticate terete algae, flattened Rhodophyta, Palmophyllum crassum and Halimeda tuna showed higher percentage cover at Montecristo Island than at the other sites. On the contrary, turf was less abundant at Montecristo Island than at the other sites, except for Livorno (). PERMANOVA analysis showed significant differences in the structure of assemblages between the reference and the other four sites; differences among areas were also significant, whereas no significant differences were detected among the years (). The ESCA index varied between 0.39 and 0.71 among the four sites. Meloria was accorded good status during the 3 years of study, while Livorno shifted from a poor status in 2008 to a moderate status in 2009–2010, and Monte Argentario and Vada shifted from moderate status to good status during the study period ().
Table 8. Mean percentage cover per sample of the main taxa/groups at the five study sites.
Table 9. Results of PERMANOVA analysis on taxa/group composition and abundance of macroalgal coralligenous assemblages. Significant effects are in bold. RC = Montecristo Island, reference conditions.
Table 10. Values of the ESCA index (EQR) and corresponding classification of the ecological status of the sites, calculated for each year of the study. EQRSL = values of the sensitivity level descriptor; EQRα = values of the diversity descriptor; EQRβ = values of the heterogeneity descriptor.
Test of the ESCA-stressors relationship
Regression lines always showed a significant negative correlation between the EQR values and values of different level of pressure indicators (; F < 0.001, F < 0.001 and F < 0.01 respectively for (a) biological invasion, (b) increasing sediment and (c) nutrient enrichment). These results confirm a clear tendency for a positive response of the ESCA index to the considered stressors.
Fig. 3. Relationship between EQR determined by the ESCA index and values obtained for each of the stressors considered: (top) ESCA-Biological invasion, (middle) ESCA-Sediment increasing and (bottom) ESCA-Nutrient enrichment. Data are from destructive samples collected in the artificially manipulated habitats () after one year of exposure to the stressors.
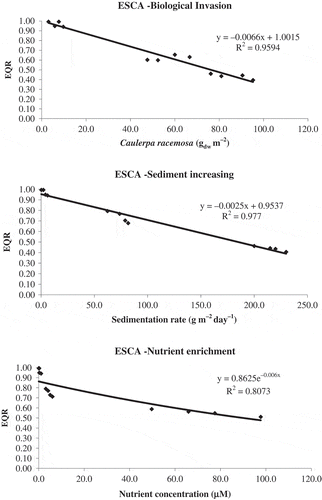
Values of the squared correlation coefficients highlighted a strong linear association between the two variables for biological invasion (R2 = 0.9594) and sedimentation rate (R2 = 0.977), while the relationship between EQR and nutrient concentrations is better described by a non-linear, exponential model (R2 = 0.8073).
Discussion
Results of the study confirmed the potential for using macroalgal coralligenous assemblages as an ecological indicator. Moreover, they allowed us to define the ESCA index and to test it along Tuscan coastlines.
Coralligenous assemblages showed a significant response to all stressors considered, even if changes observed in areas subjected to nutrient enrichment were less evident than those detected for the other stressors. This could be due to the duration of the experimental study (one year) which was probably enough to cause a severe shift in macroalgal assemblages subjected to direct cover by Caulerpa racemosa or sediments, while a longer period may be required for nutrients to cause a replacement of sensitive species by opportunistic ones.
Although there were differences among the considered impacts, such as the responses of Flabellia petiolata, Peyssonnelia spp. and flattened Rhodophyta to different stressors, a general trend in the shift of macroalgal assemblages was observed.
Halimeda tuna, Palmophyllum crassum, Zanardinia typus and erect corticated terete algae were the most sensitive taxa/groups; they always decreased in abundance regardless of the type of stressor, so may be considered the best biological indicators of human pressures on coralligenous assemblages. Under heavy loads of sediment and overgrowth by C. racemosa, decrease of these algae was probably due to the combined effects of low light levels, inhibition of recruitment and mechanical disturbance (Airoldi, Citation2003). Moreover, under stress conditions turf-forming species can outcompete erect macroalgae through pre-emption of substratum (Airoldi, Citation1998), since they can reproduce by vegetative propagation and regenerate populations after disturbance more quickly than species reproducing sexually (Airoldi, Citation2000, Citation2003).
Both native and non-native turf-forming algae, such as Womersleyella setacea (Verlaque, Citation1989), increased in abundance under all the impacted conditions, although the species composition changed, as has been described in previous studies (Balata et al., Citation2011; Piazzi et al., Citation2011). The increase of turf in macroalgal assemblages under stressed conditions is a well-known pattern in various coastal marine systems (Airoldi, Citation1998, Citation2003; Irving & Connell, Citation2002; Gorgula & Connell, Citation2004), and represents a valuable indicator of impact. Turf-forming algal species have a high surface-area/volume ratio so tend to grow faster, require more nutrients and have higher nutrient uptake rates than thicker algae with a lower surface-area/volume ratio (Taylor et al., Citation1998; Teichberg et al., Citation2008). These attributes of turf-forming algae could explain their well-known success under stress conditions and nutrient enrichment regimes (Díez et al., Citation1999; Gorgula & Connel, Citation2004). So, the results of our study clearly indicated that anthropogenic stressors acting on coralligenous assemblages drive the ecosystem from a pristine state, where the slow-growing, shade-adapted species are dominant, to a degraded state, where opportunistic species with relatively high growth rates dominate.
Both the diversity and heterogeneity metrics responded significantly to stress, with reduced values in the impacted sites compared with reference conditions; however, α-diversity showed a different response depending on the type of stressor, making it more sensitive to biological invasion. Heterogeneity decreased significantly, regardless of the type of disturbance, suggesting that it may be a more valid descriptor of ecological quality of coralligenous assemblages. In fact, due to the influence of biological interactions, coralligenous assemblages usually show a patchy distribution, maintaining habitat heterogeneity at very high levels of β-diversity. The loss of structuring perennial species and the spread of ephemeral algae under stressed conditions leads to a widespread biotic homogenization (Balata et al., Citation2007a; Piazzi & Balata, Citation2008; Piazzi et al., Citation2012), and the consequent loss of β-diversity may not depend on the increase or decrease of species richness but rather on the identity of species and their ecological function (Piazzi & Balata, Citation2008). Therefore, β-diversity represents a fundamental aspect of the ecosystems which should be taken into consideration to a greater extent when developing ecological indices.
Results of the present study showed that the ESCA index has allowed correct definition of the ecological status of the studied sites, since the resulting ecological classification agreed with environmental data and expert judgement about the same sites. Moreover, application of ESCA highlighted differences between assemblages similar to those shown by multivariate patterns. Another important finding of the investigation is the steady pattern observed through the study period. In fact, the global structure of macroalgal assemblages remained stable through the years, despite the seasonal variability of some species (Piazzi et al., Citation2004). This could represent an important element of the strength of an ecological index based on this kind of bioindicator, because of the reduction of natural temporal variability that usually characterizes the shallower macroalgal assemblages (Piazzi et al., Citation2002).
Relevance to policy and management requirements are the final criteria of the WFD for selecting an ecological index. The ESCA index has been developed according to the main compliance criteria of the WFD as (i) selected index metrics are consistent with assemblage descriptors indicated by normative definitions (Annex V; EC, Citation2000), (ii) the ecological status is assessed in one of the five required classes and (iii) the EQR scale ranges from 1 (best conditions) to 0 (worst conditions). Moreover, the multimetric nature of the index allows complex information to be summarized in a simple and reliable way, as it includes several ecological aspects of coralligenous assemblages. The concurrent use of multiple descriptors with complementary strengths represents a useful approach in order to minimize the effect of the variability of ecosystem responses to different stressors (Simboura et al., Citation2005; Martinez-Crego et al., Citation2008; Borja et al., Citation2009a, b). The selected metrics were effective in detecting changes of assemblage structure under human-induced stressed conditions, allowing the index to distinguish clearly amongst sites subjected to different levels of impact.
In comparison with indices based on traditional destructive methods, the application of ESCA is simpler and more cost-effective. Selected assemblage descriptors require simple, straightforward and widely used measures, while photographic techniques allow rapid collection of the large number of samples required in a habitat with high small-scale spatial variability (Balata et al., Citation2006a) and reduce times and costs for sorting procedures and taxonomy. Previous studies have shown that analysis of macroalgal assemblages based on morphological groups represents a valid tool for detecting response of coralligenous macroalgae to human-induced stress (Balata et al., Citation2011) and the photographic method has been recognized to be a suitable technique for monitoring quality status of coralligenous habitats (Acunto et al., Citation2001; Balata et al., Citation2005; Kipson et al., Citation2011; Deter et al., Citation2012b; Teixidó et al., Citation2013).
Finally, a non-destructive sampling approach is in line with the spirit of the European Directives, allowing the index to be employed in protected areas and in areas colonized by sensitive species.
The ESCA index is the first ecological index using rocky deep macroalgal assemblages to classify coastal waters. Moreover, it focuses on a single BQE to evaluate the status of a ‘special habitat type’ through a mixed structural/functional analysis of assemblages, and represents, in this sense, the first attempt to harmonize the opposite approaches of the WFD and MSFD. However, use of the ESCA index together with other non-destructive methods can provide more complete information on the ecological quality of coralligenous assemblages, avoiding potential conflicts in the spatial overlap of the two directives (Borja et al., Citation2010).
From a European general policy laws perspective, the ESCA index approach may offer further advantages if it can also classify coastal waters through analysis of sessile macrozoobenthic assemblages (Cecchi & Piazzi, Citation2010), even if this aspect was not investigated in the present paper. This could represent an important contribution to the implementation of the WFD 2000/60, since most of the efforts were applied to assessment with soft-bottom benthic invertebrates (Borja et al., Citation2000; Simboura & Zenetos, Citation2002; Rosenberg et al., Citation2004; Muxika et al., Citation2007) (GIG, Citation2009, Citation2013) or shallow rocky invertebrates (Orlando-Bonaca et al., Citation2012).
Despite the interesting potential of ESCA as highlighted by our study, the Mediterranean-wide datasets available on macroalgal coralligenous assemblages are still small and the method should be tested on a larger set of data, in order to evaluate the effectiveness of the index on both a wider gradient of disturbance and geographical scale. In this context, the definition of appropriate reference conditions represents a fundamental step for the application of European general policy laws (Borja et al., Citation2012; Gaspar et al., Citation2012). Montecristo Island was listed among the Reference Sites of the intercalibration network (GIG, Citation2013) so the reference conditions chosen for this study are in compliance with the WFD and correctly represent the pristine condition for the considered biogeographical area. However, the application of ESCA in other parts of the Mediterranean basin will require evaluation of the geographical range in which the reference values established in this study can be considered valid and to eventually determine other area-specific reference conditions.
Finally, a more complete pressure analysis must be developed in order to test the response of the ESCA index to the human pressures acting along the Italian coasts. In fact, establishing a robust relationship between ecological indices and human pressures represents an indispensable step in defining how the quality element behaves under disturbance in terms of type and extent of its response.
Acknowledgements
We are particularly grateful to Carlo Nike Bianchi, Monica Montefalcone and Carla Morri and two anonymous referees whose comments and suggestions have greatly improved the manuscript. We also thank Christine Maggs, Editor-in-Chief, for her support and suggestions in improving the manuscript.
References
- Acunto, S. (2000). Tecniche di campionamento fotografico e studio della variabilità spazio-temporale in popolamenti coralligeni. PhD Thesis, University of Pisa.
- Acunto, S., Balata, D. & Cinelli, F. (2001). Spatial variability in the coralligenous assemblage and evaluations of the sampling method. Biologia Marina Mediterranea, 8: 191–200.
- Aguado-Gimenez, F. & Ruiz-Fernandez, J.M. (2012). Influence of an experimental fish farm on the spatio-temporal dynamic of a Mediterranean maërl algae community. Marine Environmental Research, 74: 47–55.
- Airoldi, L. (1998). Roles of disturbance, sediment stress and substratum retention on spatial dominance in algal turf. Ecology, 79: 2759–2770.
- Airoldi, L. (2000). Effects of disturbance, life-history and overgrowth on coexistence of algal crusts and turfs. Ecology, 8: 798–814.
- Airoldi, L. (2003). The effects of sedimentation on rocky coastal assemblages. Oceanography and Marine Biology: An Annual Review, 41: 161–203.
- Anderson, M.J. (2001). A new method for a non-parametric multivariate analysis of variance. Austral Ecology, 26: 32–46.
- Anderson, M.J. (2006). Distance-based test for homogeneity of multivariate dispersions. Biometrics, 62: 245–253.
- Anderson, M.J. & Robinson, J. (2003). Generalised discriminant analysis based on distances. Australian and New Zealand Journal of Statistics, 45: 301–318.
- Anderson, M.J. & Willis, T.J. (2003). Canonical analysis of principal coordinates: a useful method of constrained ordination for ecology. Ecology, 84: 511–524.
- Anderson, M.J., Ellingsen, K.E. & McArdle, B.H. (2006). Multivariate dispersion as a measure of beta diversity. Ecology Letters, 9: 683–693.
- Anderson, M.J. & Gorley, R.N. (2007). PERMANOVA+ for PRIMER: Guide to Software and Statistical Methods. PRIMER – E, Plymouth.
- Arevalo, R., Pinedo, S. & Ballesteros, E. (2007). Changes in the composition and structure of Mediterranean rocky-shore communities following a gradient of nutrient enrichment: descriptive study and test proposed methods to assess water quality regarding macroalgae. Marine Pollution Bulletin, 55: 104–113.
- Balata, D., Piazzi, L., Cecchi, E. & Cinelli, F. (2005). Variability of Mediterranean coralligenous assemblages subject to local variation in sediment deposits. Marine Environmental Research, 60: 403–421.
- Balata, D., Acunto, S. & Cinelli, F. (2006a). Spatio-temporal variability and vertical distribution of a low rocky subtidal assemblage in the north-west Mediterranean. Estuarine Coastal and Shelf Science, 67: 553–561.
- Balata, D., Acunto, S., Piazzi, L. & Cinelli F. (2006b). Spatial variability of coralligenous assemblages. Biologia Marina Mediterranea, 13: 108–109.
- Balata, D., Piazzi, L. & Benedetti-Cecchi, L. (2007a). Sediment disturbance and loss of beta diversity on subtidal rocky reefs. Ecology, 8: 2455–2461.
- Balata, D., Piazzi, L. & Cinelli, F. (2007b). Increase of sedimentation in a subtidal system: effects on the structure and diversity of macroalgal assemblages. Journal of Experimental Marine Biology and Ecology, 351: 73–82.
- Balata, D., Piazzi, L. & Rindi, F. (2011). Testing a new classification of morphological functional groups of marine macroalgae for the detection or responses to disturbance. Marine Biology, 158: 2459–2469.
- Ballesteros, E. (2006). Mediterranean coralligenous assemblages: a synthesis of present knowledge. Oceanography and Marine Biology: An Annual Review, 44: 123–195.
- Ballesteros, E., Torras, X., Pinedo, S., Garcia, M., Mangialajo, M. & De Torres, M. (2007). A new methodology based on littoral community cartography dominated by macroalgae for the implementation of European Water Framework Directive. Marine Pollution Bulletin, 55: 172–180.
- Birk, S., Bonne, W., Borja, A., Brucet, S., Courrat, A., Poikane, S., Solimini, A., van de Bund, W., Zampoukas, N. & Hering, D. (2012). Three hundred ways to assess Europe’s surface waters: an almost complete overview of biological methods to implement the Water Framework Directive. Ecological Indicators, 18: 31–41.
- Borja, A. (2005). The European Water Framework Directive: a challenge for nearshore, coastal and continental shelf research. Continental Shelf Research, 25: 1768–1783.
- Borja, A. & Dauer, D.M. (2008). Assessing the environmental quality status in estuarine and coastal systems: comparing methodologies and indices. Ecological Indicators, 8: 331–337.
- Borja, A., Franco, J. & Perez, V. (2000). A marine biotic index to establish the ecological quality of soft bottom benthos within European estuarine and coastal environments. Marine Pollution Bulletin, 40: 1100–1114.
- Borja, A., Franco, J., Valencia, V., Bald, J., Muxika, I., Belzunce, M.J. & Solaun, O. (2004). Implementation of the European Water Framework Directive from the Basque country (northern Spain): a methodological approach. Marine Pollution Bulletin, 48: 209–218.
- Borja, A., Bald, J., Franco, J., Larreta, J., Muxika, I., Revilla, M., German Rodriguez, J., Solaun, O., Uriarte, A. & Valencia, V. (2009a). Using multiple ecosystem components, in assessing ecological status in Spanish (Basque country) Atlantic marine waters. Marine Pollution Bulletin, 59: 54–64.
- Borja, A., Ranasinghe, A. & Weiseberg, S.B. (2009b). Assessing ecological integrity in marine waters, using multiple indices and ecosystem components: challenges for the future. Marine Pollution Bulletin, 59: 1–4.
- Borja, A., Elliott, M., Carstensen, J., Heiskanen, A.S. & van de Bund, W. (2010). Marine management – towards an integrated implementation of the European Marine Strategy Framework and the Water Framework Directives. Marine Pollution Bulletin, 60: 2175–2186.
- Borja, A., Barbone, E., Basset, A., Borgensen, G., Brkljacic, M., Elliot, M., Garmendia, J.M., Marques, J.C., Mazik, K., Mukik, A.I., Neto, J.M., Norling, K., Rodriguez, J.G., Rosati, I., Rygg, B., Teixeira, H. & Trayanova, A. (2011). Response of single benthic metrics and multi-metric methods to anthropogenic pressure gradients, in five distinct European coastal and transitional ecosystems. Marine Pollution Bulletin, 62: 499–513.
- Borja, A., Dauer, D.M. & Grémare A. (2012). The importance of setting targets and reference conditions in assessing marine ecosystem quality. Ecological Indicators, 12: 1–7.
- Boudouresque, C.F. (1971). Méthodes d’étude qualitative et quantitative du benthos (en particulier du phytobenthos). Tethys, 3: 79–104.
- Cecchi, E. & Piazzi, L. (2010). A new method for the assessment of the ecological status of coralligenous assemblages. Biologia Marina Mediterranea, 17: 170–171.
- Cinelli, F., Balata, D. & Piazzi, L. (2007). Threats to coralligenous assemblages: sedimentation and biological invasions. Proceedings of the Third Mediterranean Symposium on Marine Vegetation (Marseille, 27–29 March 2007), pp. 42–47.
- Clarke, K.R. (1993). Non-parametric multivariate analyses of changes in community structure. Austral Ecology, 18: 117–143.
- Deter, J., Descamp, P., Ballesta, L., Boissery, P. & Holon F. (2012a). A preliminary study toward an index based on coralligenous assemblages for the ecological status assessment of Mediterranean French coastal waters. Ecological Indicators, 20: 345–352.
- Deter, J., Descamp, P., Boissery, P., Ballesta, L. & Holon, F. (2012b). A rapid photographic method detects depth gradient in coralligenous assemblages. Journal of Experimental Marine Biology and Ecology, 418: 75–82.
- Diez, I., Scilla, A., Santolaria, A. & Gorostiaga, J.M. (1999). Phytobenthic intertidal community structure along an environmental pollution gradient. Marine Pollution Bulletin, 38: 463–472.
- Díez, I., Bustamante, M., Santolaria, A., Tajadura, J., Muguerza, N., Borja, A., Muxika, I., Saiz-Salinas, J.I. & Gorostiaga, J.M. (2012). Development of a tool for assessing the ecological quality status of intertidal coastal rocky assemblages, within Atlantic Iberian coasts. Ecological Indicators, 12: 58–71.
- EC (2000). DIRECTIVE 2000/60/EC of the European Parliament and of the Council, of 23 October 2000, establishing a framework for Community action in the field of water policy. Official Journal of the European Communities, G.U.C.E. 22/12/2000, L 327.
- EC (2008). DIRECTIVE 2008/56/EC of the European Parliament and of the Council, of 17 June 2008, establishing a framework for Community action in the field of marine environmental policy (Marine Strategy Framework Directive). Official Journal of the European Commission, G.U.C.E. 25/6/2008, L 164/19.
- EC (2010). Guidance Document on the Intercalibration Process 2008–2011. 102 pp. Common Implementation Strategy for the Water Framework Directive. Annex III: Guidelines for deriving reference conditions and defining alternative benchmarks (18 March 2010).
- EC (2011). Commission Staff Working Paper, Brussels 2011. Relation between the initial assessment of marine waters and the criteria for good environmental status. SEC (2011) 1255 final, Brussels 14.10.2011.
- EC (2013). Commission Decision II of 20 September 2013 establishing, pursuant to Directive 2000/60EC of the European Parliament and of the Council, the values of the Member State monitoring system classifications as a result of the intercalibration exercise and repealing Decision 2008/915/EC. Brussels, 2013. Official Journal of the European Union.
- Gaspar, R., Pereira, L. & Neto, J.M. (2012). Ecological reference conditions and quality states of marine macroalgae sensu Water Framework Directive: an example from the intertidal rocky shores of Portuguese coastal waters. Ecological Indicators, 19: 24–38.
- Gatti, G., Montefalcone, M., Rovere, A., Parravicini, V., Morri, C., Albertelli, G. & Nike Bianchi, C. (2012). Seafloor integrity down the harbor waterfront: the coralligenous shoals off Vado Ligure (NW Mediterranean). Advances in Oceanography and Limnology, 3: 51–67.
- Gennaro, P. & Piazzi, L. (2011). Synergism between the invasion of macroalga Caulerpa racemosa var. cylindracea and seawater nutrient enrichment. Marine Ecology Progress Series, 427: 59–70.
- GIG (2009). JRC Scientific and Technical Reports. Water Framework Directive intercalibration technical report. Part 3: Coastal and Transitional Waters. Sect. 2 – Benthic invertebrates. Four parts: Mediterranean GIG; Black Sea GIG; North East Atlantic GIG; and Baltic GIG. WFD Intercalibration Technical Report.
- GIG (2013). JRC Scientific and Technical Reports. Water Framework Directive intercalibration technical report, Second phase (2008–2011) in draft. JRC European Commission, IES Institute for Environmental and Sustainability.
- Gorgula, S.K. & Connell, S.D. (2004). Expansive covers of turf-forming algae on human-dominated coast: the relative effects of increasing nutrient and sediment loads. Marine Biology, 145: 613–619.
- Guinda, X., Juanes, J.A., Puente, A. & Revilla, J.A. (2008). Comparison of two methods for quality assessment of macroalgal assemblages, under different pollution types. Ecological Indicators, 8: 743–753.
- Hong, J.S. (1983). Impact of pollution on the benthic community. Environmental impact of the pollution on the benthic coralligenous community in the Gulf of Fos, northwestern Mediterranean. Bulletin of Korean Fisheries Society, 16: 273–290.
- Irving, A.D. & Connell, S.D. (2002). Interactive effects of sedimentation and microtopography on the abundance of subtidal turf-forming algae. Phycologia, 41: 517–522.
- Juanes, J.A., Guinda, X., Puente, A. & Revilla, J.A. (2008). Macroalgae, a suitable indicator of the ecological status of coastal rocky communities in the NE Atlantic. Ecological Indicators, 83: 351–359.
- Kipson, S., Fourt, M., Teixidó, N., Cebrián, E., Casas, E., Ballesteros, E., Zabala, M. & Garrabou, J. (2011). Rapid biodiversity assessment and monitoring method for highly diverse benthic communities: a case study of Mediterranean coralligenous outcrops. PLoS One 6(11): e27103. doi:10.1371/journal.pone.0027103.
- Krause-Jensen, D., Carstensen, J. & Dahl, K. (2007). Total and opportunistic algal cover in relation to environmental variables. Marine Pollution Bulletin, 55: 114–125.
- Laborel, J. (1961). Le concrétionnement algal “coralligene” et son importance geomorphologique en Méditerranée. Recueil des Travaux Scientifiques de la Station Marine d’Endoume, 23: 37–60.
- Laborel, J. (1987). Marine biogenic constructions in the Mediterranean, a review. Scientific Report of Port-Cros National Park, 13: 97–127.
- Mangialajo, L., Ruggieri, N., Asnaghi, V., Chiantore, M., Povero, P. & Cattaneo-Vietti, R. (2007). Ecological status in the Ligurian Sea: the effect of coastline urbanisation and the importance of proper reference sites. Marine Pollution Bulletin, 55: 30–41.
- Martinez-Crego, B., Verges, A., Alcoverro, T. & Romero, J. (2008). Selection of multiple seagrass indicators for environmental biomonitoring. Marine Ecology Progress Series, 361: 93–109.
- Martinez-Crego, B., Alcoverro, T. & Romero, J. (2010). Monitoring the quality of coastal waters at a large scale: bioindicators’ strengths and weakness. Journal of Environmental Monitoring, 12: 1013–1028.
- Muxika, I., Borja, A. & Bald, J. (2007). Using historical data, expert judgement and multivariate analysis in assessing reference conditions and benthic ecological status, according to the European Water Framework Directive. Marine Pollution Bulletin, 55: 16–29.
- Neto, J.M., Gaspar, R., Pereira, L. & Marques, J.C. (2012). Marine Macroalgae Assessment Tool (MarMat) for intertidal rocky shores. Quality assessment under the scope of the European Water Framework Directive. Ecological Indicators, 19: 39–47.
- Orfanidis, S., Panayotidis, P. & Stamatis, N. (2001). Ecological evaluation of transitional and coastal waters: a marine benthic macrophytes-based model. Mediterranean Marine Sciences, 2: 45–65.
- Orfanidis, S., Panayotidis, P. & Stamatis, N. (2003). An insight to the ecological evaluation index (EEI). Ecological Indicators, 3: 27–33.
- Orfanidis, S., Panayotidis, P. & Ugland, K.I. (2011). Ecological Evaluation Index (EEI) application: a step forward for functional group, the formula and reference condition value. Mediterranean Marine Sciences, 12: 199–231.
- Orlando-Bonaca, M., Mavric, B. & Urbanic, G. (2012). Development of a new index for the assessment of hydromorphological alterations of the Mediterranean rocky shore. Ecological Indicators, 12: 26–36.
- Panayotidis, P., Montesanto, B. & Orfanidis, S. (2004). Use of low budget monitoring of macroalgae to implement the European Water Framework Directive. Journal of Applied Phycology, 16: 49–59.
- Piazzi, L. & Balata, D. (2008). The spread of Caulerpa racemosa var. cylindracea in the Mediterranean Sea: an example of how biological invasions can influence beta diversity. Marine Environmental Research, 65: 50–61.
- Piazzi, L. & Balata, D. (2011). Coralligenous habitat: patterns of vertical distribution of macroalgal assemblages. Scientia Marina, 75: 399–406.
- Piazzi, L., Pardi, G., Balata, D., Cecchi, E. & Cinelli, F. (2002). Seasonal dynamics of a subtidal north-western Mediterranean macroalgal community in relation to depth and substrate inclination. Botanica Marina, 45: 243–252.
- Piazzi, L., Balata, D., Pertusati, M. & Cinelli, F. (2004). Spatial and temporal variability of Mediterranean macroalgal coralligenous assemblages in relation to habitat and substrate inclination. Botanica Marina, 47: 105–115.
- Piazzi, L., Balata, D. & Cinelli, F. (2007). Invasions of alien macroalgae in Mediterranean coralligenous assemblages. Cryptogamie Algologie, 28: 289–301.
- Piazzi, L., Balata, D., Cecchi, E., Cinelli, F. & Sartoni, G. (2010). Species composition and patterns of diversity of macroalgal coralligenous assemblages of northwestern Mediterranean Sea. Journal of Natural History, 44: 1–22.
- Piazzi, L., Gennaro, P. & Balata, D. (2011). Effects of nutrient enrichment on macroalgal coralligenous assemblages. Marine Pollution Bulletin, 62: 1830–1835.
- Piazzi, L., Gennaro, P. & Balata, D. (2012). Threats to macroalgal coralligenous assemblages in the Mediterranean Sea. Marine Pollution Bulletin, 64: 2623–2629.
- Pinedo, S., Garcia, M., Satta, M.P., de Torres, M. & Ballesteros, E. (2007). Rocky-shore communities as indicators of water quality: a case study in the northwestern Mediterranean. Marine Pollution Bulletin, 55: 126–135.
- Rosenberg, R., Blomqvist, M., Nilsson, H.C., Cederwall, H. & Dimming, A. (2004). Marine quality assessment by use of benthic species abundance distributions: a proposed new protocol within the European Union Water Framework Directive. Marine Pollution Bulletin, 49: 728–739.
- Scanlan, C.M., Foden, J., Wells, E. & Best, M.A. (2007). The monitoring of opportunistic macroalgal blooms for the Water Framework Directive. Marine Pollution Bulletin, 55: 162–171.
- Simboura, N. & Zenetos, A. (2002). Benthic indicators to use in ecological quality classification of Mediterranean soft bottom marine ecosystems, including a new Biotic index. Mediterranean Marine Sciences, 3: 77–111.
- Simboura, N., Panayotidis, P. & Papathanassiou, E. (2005). A synthesis of the biological quality elements for the implementation of the European Water Framework Directive in the Mediterranean ecoregion: the case of Saronikos Gulf. Ecological Indicators, 5: 253–266.
- Soltan, D., Verlaque, M., Boudouresque, C.F. & Francour, P. (2001). Changes in macroalgal communities in the vicinity of a Mediterranean sewage outfall after the setting up of a treatment plant. Marine Pollution Bulletin, 42: 59–70.
- Taylor, R.B., Peek, J.T.A. & Rees, T.A.V. (1998). Scaling of ammonium uptake by seaweeds to surface area: volume ratio: geographical variation and the role of uptake by passive diffusion. Marine Ecology Progress Series, 169: 143–148.
- Teichberg, M., Fox, S.E., Aguila, C., Olsen, Y.S. & Valiela, I. (2008). Macroalgal responses to experimental nutrient enrichment in shallow coastal waters: growth, internal nutrient pools, and isotopic signatures. Marine Ecology Progress Series, 368: 117–126.
- Teixidó, N., Casas, E., Cebrián, E., Linares, C. & Garrabou, J. (2013). Impacts on coralligenous outcrop biodiversity of a dramatic coastal storm. PLoS One 8(1): e53742. doi:10.1371/journal.pone.0053742.
- Terlizzi, A., Benedetti-Cecchi, L., Bevilacqua, S., Fraschetti, S., Guidetti, P. & Anderson, M.J. (2005). Multivariate and univariate asymmetrical analyses in environmental impact assessment: a case study of Mediterranean subtidal sessile assemblages. Marine Ecology Progress Series, 289: 27–42.
- UNEP (2007). Draft decision on the “Action plan for the protection of the coralligenous and other calcareous bio-concretions in the Mediterranean”. UNEP (DEPI)/MED WG.320/20, 2 pp + Annex.
- Verlaque, M. (1989). Contribution à la flore des algues marines de la Méditerranée: espèces rares ou nouvelles pour les côtes françaises. Botanica Marina, 32: 101–113.
- Wells, E., Wilkinson, M., Wood, P. & Scanlan, C. (2007). The use of macroalgal species richness and composition in intertidal rocky seashores in the assessment of ecological quality under the European Water Framework Directive. Marine Pollution Bulletin, 55: 151–161.