Abstract
Many members of the Klebsormidiophyceae typically occur in terrestrial habitats where they are exposed to ultraviolet radiation (UVR). To evaluate the extent of tolerance to this waveband, 32 strains of the genera Entransia, Hormidiella, Interfilum and Klebsormidium were exposed to identical artificial UVR. The respective response patterns were determined in terms of optimum quantum yield of PSII as well as the capability to synthesize and accumulate mycosporine-like amino acids (MAAs) as sunscreens. After exposure to UVR most of the investigated isolates of Hormidiella, Interfilum and Klebsormidium exhibited only a moderate or no decrease in optimum quantum yield. In contrast, both isolates of Entransia showed strong photoinhibition. These photosynthetic activity data are well reflected in the biochemical capability to synthesize MAAs. With the exception of both Entransia strains, where not even traces of MAAs could be detected, all other isolates of Hormidiella, Interfilum and Klebsormidium accumulated UV-absorbing compounds after UVR exposure. While Interfilum and Klebsormidium contained the same putative MAA with an absorption maximum at 324 nm, Hormidiella attenuata showed another putative MAA with an absorption maximum at 325 nm. In addition, both compounds exhibited different retention times during HPLC analysis, suggesting their chemical structure must be different. All data indicated a generally high UVR tolerance in Hormidiella, Interfilum and Klebsormidium which explains well their aeroterrestrial lifestyle.
Introduction
In contrast to aquatic ecosystems, where sublittoral algae are protected against excessive radiation by the overlying water column (Kirk, Citation1994), most terrestrial organisms are affected much more by the exposure to high irradiances including ultraviolet radiation (UVR). UVR at the earth’s surface is typically controlled by numerous factors such as clouds and atmospheric particles, diurnal fluctuations, changes in day length, season, latitude and altitude, which are ultimately responsible for highly variable radiation conditions of terrestrial ecosystems (Karsten & Holzinger, Citation2014, and references therein). For phototrophs, due to their requirements for light, ultraviolet-B (UV-B, 280–315 nm) in particular plays a critical role, because these high-energy wavelengths may lead to alterations and/or damage in the native structure of biomolecules such as proteins and DNA as well as to the induction of metabolically toxic reactive oxygen species (ROS) (for review see Karsten, Citation2008). The consequences of these effects are reflected in reduced or even inhibited physiological and biochemical key processes of phototrophic organisms, such as growth (Mazza et al., Citation1999; Karsten et al., Citation2007), reproduction and survival (Franklin & Forster, Citation1997), pigmentation (Vass, Citation1997), stability of the D1 protein in photosystem II (Vass, Citation1997) as well as the activity of RUBISCO which is the key enzyme for carbon dioxide fixation (Bischof et al., Citation2000). Most algae have to face the dilemma of obtaining sufficient visible light for photosynthesis and avoiding the accompanying harmful UVR, and hence different protective mechanisms have evolved. These include avoidance, repair processes and biosynthesis of antioxidants and/or UV--screening compounds (Karsten, Citation2008). UV-sunscreens are proposed to function as passive shielding solutes by dissipating the absorbed UVR energy in the harmless form of heat without generating photochemical reactions, thereby contributing to the prevention of UV-induced direct and indirect damage to essential biomolecules. In addition, UV-sunscreens may also save metabolic energy by reducing the need for repair processes (Karsten, Citation2008). Several UV-absorbing compounds have been described as potential photoprotectants in algae and cyanobacteria. These include phenolic compounds in brown algae (Roleda et al., Citation2006), scytonemin in cyanobacteria (Garcia-Pichel & Castenholz, Citation1991)and mycosporine-like amino acids (MAA) in cyanobacteria (Garcia-Pichel & Castenholz, Citation1993) and several macro- and microalgae (Karsten et al., Citation2005, Citation2007; Kitzing et al., Citation2014).
MAAs are low-molecular (< 400 kD), colourless, water-soluble compounds, composed of an aminocyclohexenone or -hexenimine ring conjugated with a variety of different substituents, which determine characteristic absorbance maxima ranging from 310 to 360 nm in the UV-A and UV-B region (Shick & Dunlap, Citation2002; Carreto & Carignan, Citation2011). Recent studies on physico-chemical properties and functionality of MAAs underline their important role as photoprotective substances in many marine organisms (Bandaranayake, Citation1998; Shick & Dunlap, Citation2002; Carreto & Carignan, Citation2011). While MAAs are widely studied in numerous marine micro- and macroalgae (Hoyer et al., Citation2001; Karsten et al., Citation2007), much less is known for aeroterrestrial algae (Karsten et al., Citation2005; Kitzing et al., Citation2014).
Although most algae typically inhabit aquatic habitats, many taxa, mainly of the green lineage, also occur on land, where they participate in symbiotic associations with fungi, forming lichens (Friedl & Rybalka, Citation2012), or where they grow on artificial surfaces such as roof tiles or on natural surfaces such as tree bark (Karsten et al., Citation2007). In addition, many green algal taxa can grow under rocks or on or just below the surface of soil (Ettl & Gärtner, Citation1995). Aeroterrestrial algae are also typical components of so-called biological soil crusts, which are found on all continents on Earth, in arid and semi-arid regions as well as from tropical to polar regions, and in the high alpine zone (Karsten & Holzinger, Citation2014). A recent review on these microbiotic crusts clearly indicates the important ecological role of these communities for global carbon fixation (c. 7% of terrestrial vegetation) and nitrogen fixation (c. 50% of terrestrial biological N fixation) (Elbert et al., Citation2012).
Filamentous green algae of the genus Klebsormidium (Klebsormidiophyceae, Streptophyta) are typical and abundant components of biological soil crusts. Members of the genus represent one of the most widespread groups of aeroterrestrial microchlorophytes in the world, ranging in distribution from polar to tropical habitats with reported occurrence on bare soil, sand dunes, acidic post-mining sites, golf courses, iron railings and urban environments (Rindi et al., Citation2008, Citation2011; Škaloud & Rindi, Citation2013 and references therein). The cryptic and intra-specific genetic diversity within Klebsormidium seems to be high (Rindi et al., Citation2011; Škaloud & Rindi, Citation2013). In addition, more recent data indicate that Klebsormidium in soil crusts is of ecological importance (Karsten & Holzinger, Citation2014). Nevertheless, data on ecophysiological performance under environmental gradients and extremes are sparse. Klebsormidium species isolated from alpine biological soil crusts revealed wide optima and tolerances in growth, photosynthesis and respiration under gradients of light, temperature and desiccation (Karsten et al., Citation2010; Holzinger et al., Citation2011; Karsten & Holzinger, Citation2012). These ecophysiological traits explain the abundance of these species under the extreme environmental conditions of high altitudes. In contrast, the effects of UVR on physiological and biochemical processes have only been investigated for various strains of Klebsormidium fluitans (Kitzing et al., Citation2014). Here, the effect of controlled UVR on growth, photosynthesis and the capability to synthesize MAAs was studied in five ecotypes of K. fluitans collected along an elevational gradient in the Alps. All data indicated a generally high UV tolerance in relation to growth and photosynthesis, and provided evidence of induction and accumulation of a new putative MAA, which seemed to be specific for Klebsormidium. The MAA appears to be a biochemical trait whereby Klebsormidium can tolerate aspects of the aeroterrestrial lifestyle in alpine soil crusts. Using HPLC, Kitzing et al. (Citation2014) detected in their K. fluitans strains a putative MAA with an absorption maximum at 324 nm. In numerous representatives of the Trebouxiophyceae a MAA with an absorption maximum at 324 nm has been described (Hoyer et al., Citation2001; Karsten et al., Citation2005, Citation2007). More recently this has also been reported in the chlorophyceaen Tetraspora sp. (Rastogi & Incharoensakdi, Citation2013). However, the putative K. fluitans MAA was compared with MAA previously described in the Trebouxiophyceae (e.g. Prasiola crispa ssp. antarctica) (Hoyer et al., Citation2001), and although both UV-absorbing compounds exhibited the same absorption spectrum they strongly differed in retention time (Kitzing et al., Citation2014), and hence must be chemically different.
More recently, an increased interest in the common terrestrial Klebsormidiophyceae led to the discovery of a new member of the class, the genus Interfilum (Mikhailyuk et al., Citation2008). In addition, Sluiman et al. (Citation2008) showed that the genera Hormidiella and Entransia are the sister group of Klebsormidium based on nuclear-encoded SSU, group I intron and internal transcribed spacer (ITS) rDNA sequences.
While Klebsormidium and Interfilum species are typically known from various aeroterrestrial, often dry habitats, representatives of Hormidiella occur in moist soil or temporary ditches of rainwater (Iyengar & Kanthamma, Citation1940; Subrahmanyan, Citation1976; Lokhorst et al., Citation2000). Entransia is so far only reported from humid habitats such as the littoral zone of lakes or Sphagnum bogs (Cook, Citation2004, and references therein). In contrast to some Klebsormidium and Interfilum strains, the ecophysiology of Hormidiella and Entransia has never been studied. Therefore, the goal of this investigation was a comprehensive evaluation of the UVR tolerance in numerous members of the Klebsormidiophyceae from different regions of the world with an emphasis on photosynthetic activity and the capability to synthesize and accumulate MAAs.
MaterialS and Methods
Algal material and cultivation conditions
For the present investigation 32 strains of the family Klebsormidiophyceae were used (listed in ) with additional information on origin and habitat. Except for strain K2 (), all other strains have been phylogenetically studied (Mikhailyuk et al., Citation2008; Sluiman et al., Citation2008; Rindi et al., Citation2011; Škaloud & Rindi, Citation2013). Since Klebsormidium and Interfilum were the major focus of the present study we followed the different molecular taxonomic clades according to Rindi et al. (Citation2011). Further strains belonging to the sister genera Hormidiella and Entransia were also included in this study. All investigated samples originated from different biogeographic regions (America, Europe, New Zealand, Africa), different habitats (terrestrial and freshwater) and different climate zones (temperate, arid, continental). Cultures were obtained from commercial culture collections (SAG, CCAP and UTEX) or kindly provided by Professor B. Büdel (University of Kaiserslautern, Germany) through his BIOTA Africa project (www.biota-africa.org).
Table 1. Strain information and clade assignment of Interfilum and Klebsormidium (according to Rindi et al., Citation2011).
All algal isolates were cultivated as stock cultures in 300 ml Erlenmeyer flasks filled with Bold’s Basal Medium (MBB; Starr & Zeikus, Citation1993), modified by the addition of triple nitrate concentration, using K2HPO4 instead of (NH4)2HPO4, and vitamin stock solution without nicotinamide (hereinafter referred to as 3 NMBB+V) (Kitzing et al., Citation2014). The cultures were kept at 23 ± 2.5 °C and 25–30 µmol photons m−2 s−1 provided by daylight fluorescent lamps (Osram L15W/950, Lumilux deluxe Daylight, Munich, Germany) under a light:dark cycle of 16:8 h L:D. Radiation measurements were carried out with a Li-Cor LI-190-SZ quantum sensor connected to a Li-Cor LI-250 Light meter (LI-COR Corp., Lincoln, Nebraska, USA). For all physiological experiments only log-phase cultures were used.
UV induction experiments
For the sunscreen induction experiments log-phase pre-cultures were used, which were incubated in 100 mL Erlenmeyer flasks under stock culture conditions (see above) with an enhanced photon flux density of c. 50–55 µmol photons m−2 s−1. From these vital pre-cultures, each time 15–20 ml were transferred in polyacryl Petri dishes (Nümbrecht, Germany) and filled up to 33 ml final volume with 3 NMBB+V medium. The Petri dishes were afterwards exposed to PAR (=P) and PAR+UV-A+UV-B (=PAB), respectively. As a source of radiation, a combination of daylight fluorescent tubes (Osram L 36W/954 Lumilux de lux daylight, Munich, Germany) and Q-Panel UV-A-340 fluorescent lamps (Q-Panel Company, Cleveland, Ohio, USA) was used, which emitted a UV spectrum similar to natural solar radiation. The radiation measurements for UVR were carried out with a PMA broad-band UV radiometer (Solar Light Co., Philadelphia, Pennsylvania, USA) equipped with a PMA 2110 UV-A and a PMA 2106 UV-B detector, respectively. To provide the two different irradiation conditions P (47 µmol photons m−2 s−1 PAR, 0.04–0.07 Wm−2 UV-A radiation, 0 Wm−2 UV-B radiation) and PAB (55 µmol photons m−2 s−1 PAR, 7.25–9.15 Wm−2 UV-A radiation, 0.37–0.45 Wm−2 UV-B radiation) special cut-off filter foils were applied. For the simulation of PAR (400–700 nm) a 400-nm cut-off filter foil (Folex PR, Folex Dreieich, Germany) was used to exclude UV-A and UV-B. For the full treatment with PAR+UV-A+UV-B (here defined as 295–400 nm because the available UV radiometer was calibrated to this particular wavelength) a 295-nm cut-off filter foil (Ultraphan UBT, Digefra, Munich, Germany) was applied. All experiments were conducted at room temperature of approximately 23°C under a 16:8 light-dark cycle. After 4 days’ exposure the algal suspension was gently filtrated under low vacuum on glass fibre filters (GF6 Glasfaserfilter, Whatman GmbH, Dassel, Germany) and subsequently used for measurements of photosynthetic activity and sunscreen analysis. This treatment did not affect optimum quantum yield.
Measurements of photosynthetic activity
The physiological vitality as photosynthetic activity (here as optimum quantum yield Fv/Fm) was measured as in vivo chlorophyll fluorescence using a portable pulse-amplitude-modulated fluorometer (PAM 2000, Walz, Effeltrich, Germany). The determination of the optimum quantum yield (Fv/Fm) was performed according to Hanelt (Citation1998), modified as follows. After 10 min dark incubation and a 5-s far red light, the minimal fluorescence F0 was recorded with a pulse measuring beam (approximately 0.3 µmol photons m−2 s−1, 650 nm), followed by short pulses of saturating white light (0.4–0.8 s, 1000–5000 µmol photons m−2 s−1) to record Fm (Fv=Fm−F0). The distance between fibre-optic and filter surface was always kept constant at 2 mm, filters were kept moist with droplets of medium and the temperature was controlled at about 22°C.
MAA analysis
For the analysis of UV-absorbing mycosporine-like amino acids (MAAs), the biomass after 4 days’ UV-exposure (see above) was filtered on pre-weighed glass fibre filters (GF6 Glasfaserfilter, Whatman GmbH, Dassel, Germany) and immediately frozen at −70°C, freeze-dried overnight and weighed again to define the dried algal biomass. This biomass was extracted and further processed for HPLC analysis as described in detail by Karsten et al. (Citation2009). Samples were analysed with an Agilent HPLC system (Agilent, Waldbronn, Germany) and MAAs were separated on a stainless-steel Phenomenex Synergi Fusion RP-18 column (4 µm, 250 × 3.0 mm I.D.) protected with a RP-18 guard cartridge (20 × 4 mm I.D.) of the same material (Phenomenex, Aschaffenburg, Germany). As mobile phase 2.5% aqueous methanol (v/v) plus 0.1% acetic acid (v/v) in water was used, and run isocratically at a flow rate of 0.5 ml min–1. MAAs were detected online with a photodiode array detector at 330 nm, and absorption spectra (290–400 nm) were recorded each second, directly on the HPLC-separated peaks. Identification was done by spectra, retention time and by co-chromatography with pure MAA standards of palythine, porphyra-334 and shinorine kindly sent by Professor Yabe, Japan, as well as with standards extracted from other marine organisms according to Karsten et al. (Citation1998) and Hoyer et al. (Citation2001).
Statistical analysis
Mean values and standard deviations were calculated from three independent replicates per treatment. Statistical significance of all means was analysed using one-way ANOVAs. In addition, the Tukey’s post hoc test was applied to detect possible a posteriori homogeneous sub-groups of means that differed significantly.
Results
Optimum quantum yield under UVR
According to the potential maximum quantum yield of PS II (Fv/Fm) already under control conditions some strain-specific variability in the quantum yield values were noted ranging from 0.6 to 0.75 (data not shown). This range in the optimum quantum yield values points to isolate-specific properties, since all strains were treated with identical conditions. For a better comparison of the relative changes in the optimum quantum yield after UVR treatment, all data were standardized to 100% of the respective Fv/Fm control values (), which allowed a comprehensive evaluation of all isolate-specific responses and their proportionality (control versus treatment).
Fig. 1. Changes in photosynthetic optimum quantum yield (Fv/Fm) expressed as percentage of the respective control (Fv/Fm values between 0.6 and 0.75) after 4 days’ exposure to artificial PAR+UV-A+UV-B radiation in the investigated isolates of Interfilum, Klebsormidium, Hormidiella and Entransia (n=3, mean value ± SD). Asteriks indicate significant differences to the respective control for each investigated isolate as revealed by Tukey’s post hoc test. I1 to I7: Interfilum strains; K1 to K22: Klebsormidium strains; H: Hormidiella attenuata; E1 and E2: Entransia fimbriata.
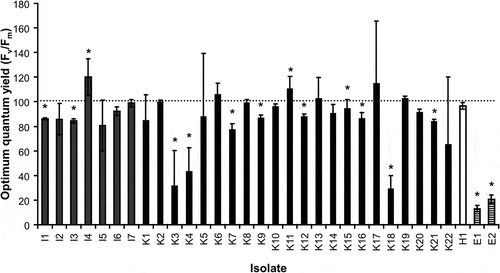
With the exception of strains I4 and K11 which exhibited a slight increase in Fv/Fm of 10–20%, most of the investigated isolates of Interfilum, Klebsormidium and Hormidiella showed after UVR no or only a moderate decrease in optimum quantum yield between 10 and 20%, respectively () (P < 0.05). I2, I5, I6, I7, K6, K8, K10, K11, K13, K14, K17, K19, K20 and H1 were not affected by UVR. In contrast, Fv/Fm in the Klebsormidium strains K3, K4 and K18 was strongly affected after UVR treatment as reflected in 60–70% inhibition (). The same was observed for both isolates of Entransia, which showed about 80–85% decrease in Fv/Fm compared with control conditions () (P < 0.05).
MAA accumulation under UVR
With the exception of both Entransia strains, where not even traces of UV-absorbing MAAs could be detected, all other isolates of Interfilum, Klebsormidium and Hormidiella accumulated UV-absorbing compounds at least after UVR treatment as measured and verified via HPLC. In Interfilum and Klebsormidium a putative MAA with a retention time of 4.5 min and an absorption maximum at 324 nm was detected (), while Hormidiella attenuata contained another unique putative MAA with a retention time of 5.6 min and an absorption maximum at 325 nm, which was distinct from that in Interfilum and Klebsormidium (). In addition, comparison with other known MAAs such as palythine, asterina-330, mycosporine-glycine, shinorine and porphyra-334 did not match the Interfilum–Klebsormidium and the Hormidiella MAA (data not shown).
Fig. 2. HPLC chromatograms recorded at 330 nm and absorption spectra of extracts of Klebsormidium isolate K14 and Hormidiella attenuata (H1) after 4 days’ exposure to artificial PAR+UV-A+UV-B radiation. Above: overlay of two chromatograms of both extracts indicating a mis-match in retention time of the Klebsormidium MAA (4.5 min) with that of H. attenuata (5.6 min). Below: overlay of the absorbance spectra (nm) of both MAAs indicating different absorbance maxima for isolate K14 (324 nm) and H. attenuata (325 nm).
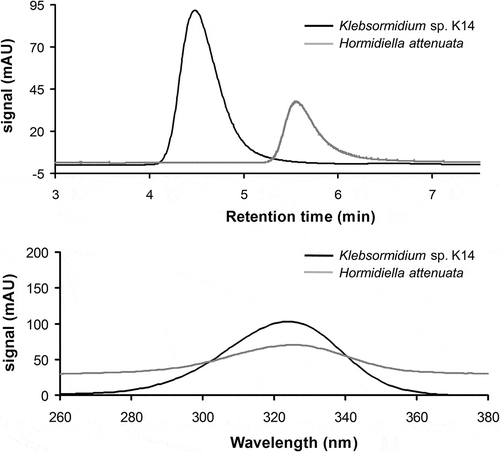
The steady-state contents of the detected MAAs under control conditions without UVR in Interfilum, Klebsormidium and Hormidiella attenuata showed broad ranges from 0 (I6) to 0.92 mg g−1 DW (I1), from 0.06 (K4) to 3.18 mg g−1 DW (K12) and of c. 0.07 mg g−1 DW (H1), respectively (). After UVR exposure a strong increase in MAA contents were detected in all Interfilum and most Klebsormidium isolates ranging from 0.4 (I6) to 2.33 mg g−1 DW (I1) and from 0.21 (K3) to 6.21 mg g−1 DW (K20), respectively (P < 0.05). In Hormidiella attenuata the MAA value significantly rose up to 1.26 mg g−1 DW () (P < 0.05). Consequently, MAA enrichment factors between 1 and 38 were recorded ().
Fig. 3. The effect of different radiation conditions (P: PAR, PAB: PAR+UV-A+UV-B) on the formation and accumulation of mycosporine-like amino acids (MAAs) (mg g−1 dry weight) in the investigated isolates of genera Interfilum, Klebsormidium, Hormidiella and Entransia (n = 3, mean value ± SD). Asterisks point to significant differences between both radiation treatments for each isolate as revealed by Tukey’s post hoc test.
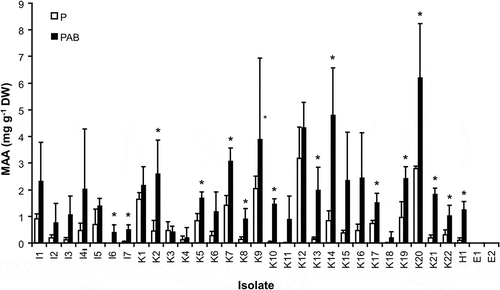
The optimum quantum yield of each strain after UVR treatment was plotted against the respective MAA content (), in order to test the relative effectiveness of the presence of MAAs to reduce UV-induced inhibition of photosynthetic activity. The increase in total MAA content was significantly positively correlated with Fv/Fm (r = 0.51) (P < 0.01), and a linear function could be calculated (y = 4.35x + 0.25). Increasing MAA contents were accompanied with higher values of the optimum quantum yield under UVR (). This correlation points to two groups of strains (labelled as small and big circles in ) which differed in their capacity to accumulate MAAs under UVR. The MAA accumulation response was reflected in a UVR sensitive or insensitive optimum quantum yield ().
Discussion
The PAM measurements typically reveal information about the potential optimum quantum yield of PS II (Fv/Fm) which represents an important marker to evaluate the physiological state of the respective investigated alga (Hanelt, Citation1998). The data clearly indicate that the optimum quantum yield of most Interfilum and Klebsormidium strains as well as in Hormidiella was not or only slightly affected by UVR. The only exceptions were the Klebsormidium isolates K3 (K. flaccidium), K4 (K. elegans) and K18 (Klebsormidium sp.), which exhibited strong inhibition of the Fv/Fm values (). These isolates originated from Germany (soil in a beet field), the Netherlands (bark of an oak tree) and South Africa (biological soil crust), respectively. Both European habitats can be described as shaded, and Gray et al. (Citation2007) reported that green algae from biological soil crusts, in most cases, do not grow fully exposed at the top of the community. The authors showed that for biotic crusts of North American deserts the abundant green microalgae vertically occupy microenvironments within the soil crust which contributed to protection from damaging solar radiation. Therefore, it is reasonable to assume that K18 (Klebsormidium sp.) might occur under shaded conditions inside the South African soil crust, which would explain why this isolate along with K3 (K. flaccidium) and K4 (K. elegans) exhibited a low photosynthetic tolerance against UVR.
In contrast to all other Klebsormidiophyceae studied, both Entransia isolates exhibited the strongest decrease in the optimum quantum yield of PS II after UVR exposure, pointing to pronounced photoinhibition or even photodamage. Members of this genus typically occur in freshwater or moist conditions such as the sheltered littoral zone of lakes or in association with Sphagnum bogs, both of which can be characterized as rather shaded habitats, which would explain the rather high sensitivity to UVR.
The generally high UVR tolerance of most Interfilum and Klebsormidium strains, however, is well supported by a study on five ecotypes of K. elegans from alpine regions of the Alps which indicated only minor effects of UV-A and UV-B treatment on the optimum quantum yield too (Kitzing et al., Citation2014). These data are in agreement with those on other unicellular aeroterrestrial green algae, for example, Apatococcus sp. isolated from building facades (Karsten et al., Citation2007) or members of the aeroterrestrial green macroalgal genus Prasiola (Holzinger et al., Citation2006). Prasiola species typically grow subaerially on various hard substrata such as rock cliffs underneath bird colonies, often several metres above sea level, where they experience the greatest fluctuations in all physico-chemical factors including UVR (Holzinger et al., Citation2006). Prasiola crispa from polar regions also showed a UVR-insensitive photosynthesis (Holzinger et al., Citation2006).
Besides physiological traits, morphological or ecological protection may also contribute to UVR tolerance. Terrestrial algae often develop thick cell walls (Karsten & Holzinger, Citation2014), in many cases with mucilage layers or with incorporated macromolecules such as sporopollenins or sporopollenin-like substances (algenans) that might absorb the UVR (VanWinkle-Swift & Rickoll, Citation1997; Pescheck et al., Citation2010). In addition, under natural conditions, terrestrial green algae are most often incorporated into biofilms, while Klebsormidium species can form multi-layered structures on top of or interwoven with the upper millimetres of soil particles (Häubner et al., Citation2006). Both microstructures most probably contribute to a high degree of self-shading and hence photoprotection of individual cells inside such a population.
In contrast to terrestrial algae, there are many publications on the effect of UVR on photosynthesis of aquatic micro- and macroalgae (for review see Karsten, Citation2008, and references therein). The emerging picture is that no general physiological response exists in algae, since some species exhibit strong inhibition of photosynthesis while others are quite tolerant or even resistant. Many factors contribute to species-specific UVR response patterns, including biogeographic region (e.g. low versus high latitude habitats), seasons, as well as the physiological state and biochemical capabilities of the organism. The effects of UVR on photosynthetic mechanisms are also manifold (Vass, Citation1997). The main molecular target seems to be centred directly at PS II or at adjacent structures. This includes damage of the light-harvesting complex (Lorenz et al., Citation1997), D1 protein or oxygen-evolving complex (Renger et al., Citation1986) or photo destruction of pigments (Strid et al., Citation1990). All these damages may result in an impairment of energy transfer to the PS II reaction centre, thereby blocking electron flow between both photosystems (Bischof et al., Citation2006).
The underlying mechanisms which guarantee unchanged photosynthesis or other insensitive physiological traits under UVR exposure may be related to the presence of sunscreen compounds. These molecules are represented in many terrestrial algal and cyanobacterial taxa by mycosporine-like amino acids (MAAs) (Garcia-Pichel & Castenholz, Citation1993; Karsten et al., Citation2005; Kitzing et al., Citation2014). MAAs are presumed to function as passive shielding solutes by dissipating the absorbed UVR energy in the form of harmless heat without generating photochemical reactions. In addition, they exhibit high molar absorptivity for UV-A and UV-B, and have been reported as photochemically stable molecules, both of which are prerequisites for their sunscreen function (Bandaranayake, Citation1998). There is also experimental evidence on the role of MAAs as UVR sunscreen compounds, for example, in dinoflagellates (Neale et al., Citation1998).
All the strains of Interfilum and Klebsormidium studied synthesized and accumulated a unique putative MAA which was exclusively found in both genera, and which matched those recently described in various K. fluitans isolates (Kitzing et al., Citation2014). This putative Interfilum–Klebsormidium MAA was compared with that formerly described in the aeroterrestrial green alga Prasiola crispa ssp. antarctica (Hoyer et al., Citation2001) and in various other taxa of the green algal class Trebouxiophyceae (Karsten et al., Citation2005). Although both UV-absorbing compounds exhibited the same absorption spectrum (Kitzing et al., Citation2014), they strongly differed in retention time (data not shown), and hence the chemical structure must be different. In Hormidiella attenuata another unique putative MAA with an absorption maximum at 325 nm was detected for the first time, which is clearly different from that in Interfilum and Klebsormidium. This compound was also strongly accumulated during UVR exposure. Although an elucidation of the chemical structure of both MAAs is missing, the chromatographic behaviour (specific column and specific eluent for MAAs) and the shape and maximum of the respective absorption spectrum point to a MAA chromophore. Therefore, it is reasonable to assume that the UV-absorbing compounds in Interfilum, Klebsormidium and Hormidiella represent new, so far chemically not identified MAAs. In contrast, both Entransia isolates lacked any trace of UV-absorbing compounds. The quantitative MAA distribution patterns among all Klebsormidiophyceae studied clearly indicate that the higher the content the more insensitive was the optimum quantum yield of PSII under UVR treatment. The strong inhibition of photosynthetic activity under UVR in Entransia can thus be explained by the missing biochemical capability to synthesize and enrich MAAs.
In conclusion, many members of the Klebsormidiophyceae occur in aeroterrestrial habitats where they might be exposed to high UVR. To counteract potential radiation damage by this waveband Klebsormidium, Interfilum and Hormidiella seem to be well protected because of their ability to accumulate group-specific sunscreen compounds.
Acknowledgements
We thank Thomas Pröschold for selecting the different Klebsormidiophyceae strains.
Additional information
Funding
Notes on contributors
Christine Kitzing
This work was done in the frame of the diploma thesis of CK under the supervision of UK. UK developed the concept, introduced MAA analysis, helped drafting, did editing. CK did all practical and statistical work, wrote the first drafts of all chapters.
REFERENCES
- Bandaranayake, W.M. (1998). Mycosporines: are they nature’s sunscreens? Natural Product Reports, 15: 159–172.
- Bischof, K., Hanelt, D. & Wiencke, C. (2000). UV-effects on photosynthesis and related enzyme reactions of marine macroalgae. Planta, 211: 388–395.
- Bischof, K., Rautenberger, R., Brey, L. & Perez-Llorens, J.L. (2006) Physiological acclimation along gradients of solar irradiance within mats of the filamentous green macroalga Chaetomorpha linum from southern Spain. Marine Ecology Progress Series, 306: 165–175.
- Carreto, J.I. & Carignan, M.O. (2011). Mycosporine-like amino acids: relevant secondary metabolites. Chemical and ecological aspects. Marine Drugs, 9: 387–446.
- Cook, M.E. (2004). Structure and asexual reproduction of the enigmatic Charophycean green alga Entransia fimbriata (Klebsormidiales, Charophyceae). Journal of Phycology, 40: 424–431.
- Elbert, W., Weber, B., Burrows, S., Steinkamp, J., Büdel, B., Andreae, M.O. & Pöschl, U. (2012). Contribution of cryptogamic covers to the global cycles of carbon and nitrogen. Nature Geoscience, 5: 459–462.
- Ettl, H. & Gärtner, G. (1995). Syllabus der Boden-, Luft- und Flechtenalgen. Gustav Fischer, Stuttgart, p. 721.
- Friedl, T. & Rybalka, N. (2012). Systematics of the green algae: a brief introduction to the current status. Progress in Botany, 73: 259–280.
- Franklin, L.A. & Forster, F.M. (1997). The changing irradiance environment: consequences for marine macrophyte physiology, productivity and ecology. European Journal of Phycology, 32: 207–232.
- Garcia-Pichel, F. & Castenholz, R.W. (1991) Characterization and biological implications of scytonemin, a cyanobacterial sheath pigment. Journal of Phycology, 27: 395–409.
- Garcia-Pichel, F. & Castenholz, R.W. (1993). Occurrence of UV-absorbing, mycosporine-like compounds among cyanobacterial isolates and an estimate of their screening capacity. Applied and Environmental Microbiology, 59: 163–169.
- Gray, D.W., Lewis, L.A. & Cardon, Z.G. (2007). Photosynthetic recovery following desiccation of desert green algae (Chlorophyta) and their aquatic relatives. Plant Cell Environment, 30: 1240–1255.
- Hanelt, D. (1998). Capability of dynamic photoinhibition in Arctic macroalgae is related to their depth distribution. Marine Biology, 131: 361–369.
- Häubner, N., Schumann, R. & Karsten, U. (2006). Aeroterrestrial algae growing in biofilms on facades – response to temperature and water stress. Microbial Ecology, 51: 285–293.
- Holzinger, A., Karsten, U., Lütz, C. & Wiencke, C. (2006). Ultrastructure and photosynthesis in the supralittoral green macroalga Prasiola crispa from Spitsbergen (Norway) under UV exposure. Phycologia, 45: 168–177.
- Holzinger, A., Lütz, C. & Karsten, U. (2011). Desiccation stress causes structural and ultrastructural alterations in the aeroterrestrial green alga Klebsormidium crenulatum (Klebsormidiophyceae, Streptophyta) isolated from an alpine soil crust. Journal of Phycology, 47: 591–602.
- Hoyer, K., Karsten, U., Sawall, T. & Wiencke, C. (2001). Photoprotective substances in Antarctic macroalgae and their variation with respect to depth distribution, different tissues and developmental stages. Marine Ecology Progress Series, 211: 117–129.
- Iyengar, M.O.P. & Kanthamma, S. (1940). Hormidiella, a new member of the Ulothrichaceae. Journal of the Indian Botanical Society, 19: 157–166.
- Karsten, U., Sawall, T., Hanelt, D., Bischof, K., Figueroa, F.L., Flores-Moya, A. & Wiencke, C. (1998) An inventory of UV-absorbing mycosporine-like amino acids in macroalgae from polar to warm-temperate regions. Botanica Marina, 41: 443–453.
- Karsten, U. (2008). Defense strategies of algae and cyanobacteria against solar UVR. In Algal Chemical Ecology (C.D. Amsler, editor), 273–296. Springer, Berlin.
- Karsten, U. & Holzinger, A. (2012). Light, temperature, and desiccation effects on photosynthetic activity, and drought-induced ultrastructural changes in the green alga Klebsormidium dissectum (Streptophyta) from a high alpine soil crust. Microbial Ecology, 63: 51–63.
- Karsten, U. & Holzinger, A. (2014). Green algae in alpine biological soil crust communities – acclimation strategies against ultraviolet radiation and dehydration. Biodiversity and Conservation, 23: 1845–1858.
- Karsten, U., Friedl, T., Schumann, R., Hoyer, K. & Lembcke, S. (2005). Mycosporin-like amino acids and phylogenies in green algae: Prasiola and its relatives from the Trebouxiophyceae (Chlorophyta). Journal of Phycology, 41: 557–566.
- Karsten, U., Lembcke, S. & Schumann, R. (2007). The effects of ultraviolet radiation on photosynthetic performance, growth and sunscreen compounds in aeroterrestrial biofilm algae isolated from building facades. Planta, 225: 991–1000.
- Karsten, U., Escoubeyrou, K. & Charles, F. (2009). The effect of re-dissolution solvents and HPLC columns on the analysis of mycosporine-like amino acids in the eulittoral macroalgae Prasiola crispa and Porphyra umbilicalis. Helgoland Marine Research, 63: 231–238.
- Karsten, U., Lütz, C. & Holzinger, A. (2010). Ecophysiological performance of the aeroterrestrial green alga Klebsormidium crenulatum (Charophyceae, Streptophyta) isolated from an alpine soil crust with an emphasis on desiccation stress. Journal of Phycology, 46: 1187–1197.
- Kirk, J.T.O. (1994). Optics of UV-B radiation in natural waters. Archives of Hydrobiology, 45: 1–16.
- Kitzing, C., Pröschold, T. & Karsten, U. (2014). UV-induced effects on growth, photosynthetic performance and sunscreen contents in different populations of the green alga Klebsormidium fluitans (Streptophyta) from alpine soil crusts. Microbial Ecology, 67: 327–340.
- Lokhorst, G.M., Star, W. & Lukesova, A. (2000). The new species Hormidiella attenuate (Klebsormidiales), notes on morphology and reproduction. Algological Studies, 100: 11–27.
- Lorenz, M., Schubert, H. & Forster, R.M. (1997) In vitro- and in vivo effects of ultraviolet-B radiation on the energy transfer in phycobilisomes. Photosynthetica, 33: 517–527.
- Mazza, C.A., Battista, D., Zima, A.M., Szwarcberg-Bracchitta, M., Giordano, C.V., Acevedo, A., Scopel, A.L. & Ballare, C.L. (1999). The effects of solar ultraviolet-B radiation on the growth and yield of barley are accompanied by increased DNA damage and antioxidant responses. Plant, Cell and Environment, 22: 61–70.
- Mikhailyuk, T.I., Sluiman, H., Massalski, A., Mudimu, O., Demchenko, E., Kondratyuk, S. & Friedl, T. (2008). New streptophyte green algae from terrestrial habitats and an assessment of the genus Interfilum (Klebsormidiophyceae, Streptophyta). Journal of Phycology, 44: 1586–1603.
- Neale, P.J., Banaszak, A.T. & Jarriel, C.R. (1998) Ultraviolet sunscreens in Gymnodinium sanguineum (Dinophyceae): mycosporine- like amino acids protect against inhibition of photosynthesis. Journal of Phycology, 34: 928–938.
- Pescheck, F., Bischof, K. & Bilger, W. (2010) Screening of ultraviolet-A and ultraviolet-B radiation in marine green macroalgae (Chlorophyta). Journal of Phycology, 46: 444–455.
- Rastogi, R.P. & Incharoensakdi, A. (2013). UV radiation-induced accumulation of photoprotective compounds in the green alga Tetraspora sp. CU2551. Plant Physiology and Biochemistry, 70: 7–13.
- Renger, G., Voss, M., Grabber, P. & Schulze, A. (1986) Effect of UV irradiation on different partial reactions of the primary processes of photosynthesis. In Stratospheric ozone reduction, solar ultraviolet radiation and plant life (R.C. Worrest, M.M. Caldwell, editors) NATO ASI Series, vol. G8. Springer, Berlin, pp 171–184.
- Rindi, F., Guiry, M.D. & López-Bautista, J.M. (2008). Distribution, morphology and phylogeny of Klebsormidium (Klebsormidiales, Charophyceae) in urban environments in Europe. Journal of Phycology, 44: 1529–1540.
- Rindi, F., Mikhailyuk, T.I., Sluiman, H.J., Friedl, T. & López-Bautista, J.M. (2011). Phylogenetic relationships in Interfilum and Klebsormidium (Klebsormidiophyceae, Streptophyta). Molecular Phylogenetics and Evolution, 58: 218–231.
- Roleda, M.Y., Clayton, M.N. & Wiencke, C. (2006). Screening capacity of UV-absorbing compounds in spores of Arctic Laminariales. Journal of Experimental Marine Biology and Ecology, 338: 123–133.
- Shick, J.M. & Dunlap, W.C. (2002). Mycosporine-like amino acids and related gadusol: biosynthesis, accumulation and UV-protective functions in aquatic organisms. Annual Review of Physiology, 64: 223–262.
- Strid, A., Chow, W.S. & Anderson, J.M. (1990) Effects of supplementary ultraviolet-B radiation on photosynthesis in Pisum sativum. Biochimica Biophysica Acta, 1020: 260–268.
- Škaloud, P. & Rindi, F (2013). Ecological differentiation of cryptic species within an asexual protist morphospecies: a case study of filamentous green alga Klebsormidium (Streptophyta). Journal of Eukaryotic Microbiology, 60: 350–362.
- Sluiman, H.J., Guihal, C. & Mudimu, O. (2008). Assessing phylogenetic affinities and species delimitation in Klebsormidiales (Streptophyta): nuclear-encoded rDNA phylogenies and its secondary structure models in Klebsormidium, Hormidiella and Entransia. Journal of Phycology, 44: 183–195.
- Subrahmanyan, A. (1976). Structure and reproduction of Hormidiella bharatiensis sp. nov. Hydrobiologia, 48: 33–36.
- Starr, R.C. & Zeikus, J.A. (1993). UTEX – the culture collection of algae at the University of Texas at Austin 1993 list of cultures. Journal of Phycology, 29: 1–106.
- VanWinkle-Swift, K.P. & Rickoll, W.L. (1997). The zygospore wall of Chlamydomonas monoica (Chlorophyceae): morphogenesis and evidence for the presence of sporopollenin. Journal of Phycology, 33: 655–665.
- Vass, I. (1997). Adverse effects of UV-B light on the structure and function of the photosynthetic apparatus. In Handbook of Photosynthesis (M. Pessarakli, editor), 931–949. Marcel Dekker Inc., New York.