Abstract
In this study we combine field demographic surveys with controlled culture experiments, to understand if temperature might be the factor leading to differences in life expectancy and seasonality across different latitudinal ranges in the kelp Saccorhiza polyschides. This species overwinters as a macroscopic sporophyte in geographically central populations, like Brittany (northwestern France), while in northern Portugal, the southern range of its distribution, only microscopic forms are present in winter. Our results did not support temperature as the cause of the different life histories observed in central and southern ranges. We found that sporophyte recruitment occurs year-round in Brittany while farther south it is limited to spring. Our results show that this seasonal recruitment is not due to winter temperature, as zoospores were able to develop between 5 and 20°C with an optimum at 10–15°C, placing the Portuguese winter temperatures within the optimum range. In addition, we showed that the synchronized release of zoospores in early autumn in the southern range does not explain the absence of recruits throughout winter, as these took a maximum of 62 days, at 5°C, to develop into a sporophyte visible in the field (5 cm) and only 20 days at 10°C. An alternative hypothesis that could explain the different life histories is the seasonality of high seawater nutrient levels occurring in the southern but not in the central-northern range, where permanently higher nutrient concentrations might allow sporophytes to occur in any season. This hypothesis is supported by the coincidence of macroscopic sporophytes with the seasonal upwelling period in the southern range, but it requires further testing.
INTRODUCTION
Climate change over the last few decades has been impacting the distribution of species across many habitats, both terrestrial (e.g. Brommer, Citation2004; Chen et al., Citation2011) and marine (e.g. Fernández, Citation2011; Nicastro et al., Citation2013; Smale et al., Citation2013). Before lethal effects cause shifts in distributional ranges, changes in vital rates and timing of demographic events within populations are likely to happen (Bates et al., Citation2014). However, changes in these traits have received less attention than changes in production and species distributions (Edwards & Richardson, Citation2004). Besides the major effect of temperature patterns, one of the predicted, and already observable, effects of global warming is a change in wind speed and direction, which greatly affects currents (Bakun, Citation1990; Lemos & Pires, Citation2004; McGregor et al., Citation2007; Gómez-Gesteira et al., Citation2008; Narayan et al., Citation2010). This will in turn affect seawater temperatures by altering upwelling regimes, deregulating their seasonality and intensity. Models predict not only a delay in these events but also a culmination in stronger and later upwelling (Barth et al., Citation2007). In addition to major temperature changes, such changes in timing and volume of colder and nutrient-rich water transported near shore greatly impact local communities and may dissociate nutrient availability from demand (Chen, Citation2000; Barth et al., Citation2007). Moreover, the effect of environmental factors may be coupled, as has been observed for temperature and light exposure (Stæhr & Wernberg, Citation2009) and for nitrogen and temperature (Gerard, Citation1997; Johnson et al., Citation2011). Also, changes in physical conditions may decrease resistance to strong hydrodynamics (Wernberg et al., Citation2011) and have cascading effects on ecosystems by making conditions suitable for introduced species to proliferate or unsuitable for ecologically important ones (Johnson et al., Citation2011).
Kelp forests, which dominate the rocky upper infralittoral zone of most temperate to cold coastal areas, constitute one of the richest marine habitats upon which several species depend. Geographic shifts in kelp distribution have been observed globally, most likely due to global warming, pollution and overexploitation of marine habitats (Müller et al., Citation2009; Wernberg et al., Citation2010; Assis et al., Citation2013). In the Northeastern Atlantic, the annual kelp Saccorhiza polyschides (Lightfoot) Batters is one of the dominant forest-forming species (Smale et al., Citation2013). It can be found from Norway to Morocco, in the Azores and Strait of Messina, and has been reported to survive only in areas with a narrow temperature range (Ardré, Citation1970; Lüning, Citation1990). In Morocco, the Galician coast and the Bay of Biscay its presence is believed to depend on the typical summer upwelling, which lowers water temperature (Fernandez et al., Citation1988; Lüning, Citation1990). In these areas, individuals are only observable in the field from spring to early autumn (Norton, Citation1977; Fernandez et al., Citation1988; Lüning, Citation1990). In contrast, in several areas, from Brittany northwards, they are able to overwinter (Norton & Burrows, Citation1969; Jensen et al., Citation1985). Although S. polyschides sporophytes are known to be able to live at temperatures between 3 and 24°C, at lower latitudes the end of the typical upwelling season (in the beginning of autumn) accompanies the decay of the individuals (Norton, Citation1977; Fernandez et al., Citation1988; Lüning, Citation1990).
The goal of this work is to understand the processes mediating life history variations across different latitudinal ranges in the kelp S. polyschides, by studying reproduction and recruitment in the field and conducting laboratory experiments to test hypotheses related to temperature effects on microscopic life stages. Since a myriad of species depend on these marine forests, it is important to understand what might limit this species’ presence and, thus, how their populations might adapt in the future. Seasonality of reproduction and recruitment were studied in two contrasting populations: in Northern Portugal, where macroscopic S. polyschides sporophytes occur in spring/summer, and in Northwestern France, in Brittany, where they are found all year long. In both regions the sporophytes are annual, but they are only seasonal in the south. To serve as a control for adequate environmental conditions for kelp occurrence, the main sympatric perennial forest-forming kelp species were also studied in both areas. Laminaria ochroleuca Bachelot de la Pylaie, which has a southern distribution limit similar to S. polyschides, was studied in Portugal. Laminaria hyperborea (Gunnerus) Foslie, which has the same northern limit as S. polyschides, and Laminaria digitata (Hudson) Lamouroux were studied in Brittany, where the latter has its southern distribution limit (Lüning, Citation1990; Tuya et al., Citation2012). To understand differences in the seasonality of recruitment of S. polyschides between latitudes, development of the microscopic stages to small sporophytes of these species from both northern Portugal and Brittany were studied in culture under different temperature conditions.
Understanding why S. polyschides is able to overwinter as a macroscopic sporophyte in some areas but not in others will provide information on the environmental limitations and triggers of its life cycle. It might also shed some light on the conditions affecting its distribution and help in understanding why this species is absent from areas that seem suitable for settlement. This information will also help predict how its distribution may vary in the near future.
MATERIALS AND METHODS
Species models
Although the word kelp originally designates the ashes of brown algae, here we apply the ecological definition of ‘kelp’ to refer to large brown algae capable of forming marine forests. A typical kelp life cycle, as seen in the orders Tilopteridales, Laminariales and Desmarestiales, consists of an alternation between two generations: macroscopic sporophytes and microscopic gametophytes (this does not apply to ‘kelp‘ in the order Fucales, such as the genus Durvillaea). Species are designated as annuals or perennials according to their macroscopic sporophyte phase only. Sporophyte reproduction occurs via haploid zoospores formed in sporangia, in specialized tissue called sori. When mature, the tissue disintegrates releasing the zoospores to settle on the substrate. After settling, zoospores germinate and develop into female and male gametophytes. Eggs on female gametophytes trigger the release of spermatozoids from male gametophytes close enough to detect the signalling pheromone (Maier & Müller, Citation1986; Mohring et al., Citation2013). Since pheromones are rapidly diluted, especially in areas with strong water motion, synchrony in gamete release increases their density, which is fundamental to ensuring fertilization success (Maier & Müller, Citation1986; Norton, Citation1992; Reed et al., Citation1997). This synchrony in life cycle is controlled by environmental cues such as photoperiod and temperature (Norton, Citation1977; Kain, Citation1989). The zoospores and gametophytes have the potential to arrest their development or develop slowly during the most unfavourable period and to accelerate their growth when conditions become conducive (Hoffman & Santelices, Citation1991; Kinlan et al., Citation2003; Carney & Edwards, Citation2010; Carney, Citation2011).
Census setup
In 2005, two 3 m2 areas with S. polyschides, L. digitata and L. hyperborea were chosen for a 2-year survey. Surveys in Brittany were conducted at two sites 2 km apart: Ar Pourven (48º42′43.8′′N, 3º57′30.0′′W) and Duons (48º43′40.8′′N, 3º55′32.4′′W). In Northern Portugal, a 4 m2 area with L. ochroleuca was delimited in 2008 for a 2-year survey in Viana do Castelo (41º41′44.80′′N, 8º51′8.40′′W) and in 2010, another 4 m2 area was chosen in Areosa (41°42′33.56′′N, 8°51′48.81′′W) for a 1-year survey of S. polyschides. All delimited areas were above hydrographic zero, at the shallowest area these species inhabit, being out of water seldom and for short periods during the lowest tides. The surveys were done by tagging all individuals with stipes longer than 2 cm, in a predetermined area, with cable ties. On a monthly basis, new individuals were tagged and stipe and blade length and reproductive status were recorded for all of them.
Culture experiment
Reproductive tissue was collected from 40 S. polyschides individuals from Viana do Castelo, North Portugal (41°42′33.56′′N, 8°51′48.81′′W) and Roscoff, North Brittany, France (48°43′8.76′′N, 3°57′55.08′′W) in early September 2011. Zoospore release and initial culture was performed following the methods of Oppliger et al. (Citation2011). Zoospore release was induced from 1.5 cm2 of reproductive tissue from 5 different random individuals per Petri dish. Tissue from each individual was used in at most 2 replicates, but combined with different random individuals. Sets of 3 replicates from each site were set at different temperatures: 5, 10, 15, 20 and 25°C at a photon irradiance of 40 µmol photons m−2 s−1, in a 12 h day−1 photoperiod and in 1% Provasoli enriched seawater. Three fields of view were defined for each replicate and counting was always done in the same areas, to allow the fate of each stage to be followed. Counts were done at days 0, 1, 3, 5, 8, 11, 15, 20, 25 and 30 and included the following development stages: non-germinated zoospores, germinated zoospores, male gametophyte, female gametophyte and sporophytes. Morphology of the gametophytes and number of sporophytes per female gametophyte were also recorded. As soon as sporophytes appeared in the cultures, photographs of 20 sporophytes per replicate were taken and their length measured with GIMP (GNU image Manipulation Program). When their size reached about 0.02 mm, 20 individuals from each original Petri dish were transferred into a new one, to make sure their density was similar and to follow growth and survival. The experiment ended when sporophytes reached 0.5 cm in length.
Field data analysis
Individuals were divided into recruits and adults according to their total length, which is the best predictor of the individuals’ ability to become reproductive (Chapman, Citation1986).
Since day length and temperature are considered to be the main environmental conditions influencing species life cycle and seasonality, population structure, percentage of reproductive individuals and recruit density were compared with day length and sea surface temperature (SST) data to determine if these can explain the differences in life history. Daily values of SST recorded over the census year in the sampling area (4 km grid) were used, to allow a comparison between them. All SST data were provided by NASA (Aqua MODIS SST, NASA). Recruits were not considered in calculations of either population structure or percentage of reproductive individuals to avoid bias due to recruitment seasonality.
Culture data analysis
Data were analysed with PERMANOVA and PERMDISP, with Primer + Permanova 6 software (Anderson et al., Citation2008). Pair-wise comparisons were used whenever a significant difference between treatments was found. Analyses were performed with 9999 permutations. Germination ratio was analysed considering the factors time and temperature and site of zoospore origin. Sex ratio was calculated as the number of males per female, when 100% of zoospores had developed into gametophytes, and a binomial comparative trial for the null hypothesis of a 1:1 sex ratio was used. Mortality of microscopic stages was calculated for the period zoospores took to produce sporophytes seen with the naked eye (0.02 mm in length). Sporophyte mortality was calculated for the period sporophytes took to grow from 0.02 mm to 0.5 cm in length. Mortality of microscopic stages and sporophytes, sex ratio, and the number of sporophytes obtained from each 100 initial zoospores were analysed considering temperature and zoospore origin. Since no sporophytes were produced at 25°C, this temperature was not considered in the analysis.
Growth rate analysis was done considering the point of appearance of the first sporophytes as time 0. Since the death rate of the Portuguese set at 20°C was very high, they were only studied up to 12 days after their appearance. As such, 20°C sets at day 18 were not included in the growth rate analysis.
Based on field work experience, 5 cm was considered as the minimum size for a sporophyte to be noticed in the field. Linear regression of sporophyte size was used to estimate how long it would take for the sporophytes to reach 5 cm. Data were expressed in days since the zoospore release was induced. Data were analysed with PERMANOVA and PERMDISP. The Portuguese set at 20°C was not included since mortality was too high for them to reach this size.
RESULTS
Census and environmental conditions
Overall the number of individuals surveyed in the field in Roscoff was 273 L. digitata, 51 L. hyperborea and 58 S. polyschides over a surface area of 3 m2; in Northern Portugal it was 82 S. polyschides and 125 L. ochroleuca over 4 m2.
In Northern Portugal, populations of S. polyschides were seasonal, with no overwintering macroscopic sporophytes (). All individuals recruited within 4 months (April to June, ) and sporophytes were observed until the end of September (). They reproduced only once and those present in August 2010 and September 2011 all died at about the same time, with the first storms (). Still, 2010 was a distinctive year for S. polyschides: recruitment occurred late, around May, and the sporophytes did not reach maturity before destruction by the first autumn storms (). In Northern Brittany, on the contrary, S. polyschides individuals were able to overwinter and no seasonality could be identified in the 2-year survey data (). Also, both recruitment and reproduction occurred throughout the year (). Even though some individuals lived longer than 14 months, they were able to reproduce only once through the course of their life.
Fig. 1. Sea surface temperature and number of recruits, reproductive and non reproductive individuals per square metre for S. polyschides populations from (A) North Portugal and (B) Brittany, France. * indicates a lack of data for that month, due to storms precluding fieldwork.
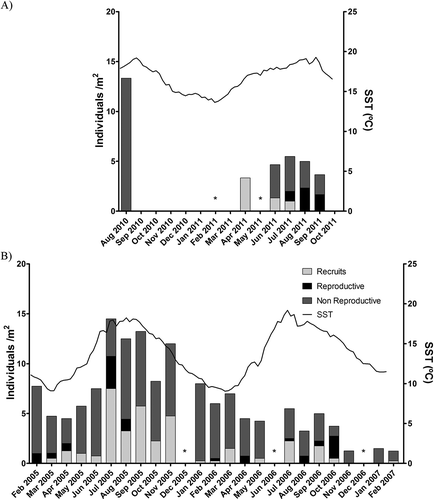
In contrast, L. ochroleuca, the main perennial forest-forming kelp in North Portugal, which shares the habitat with S. polyschides and has the same southern distribution limit, was able to survive, recruit and reproduce all year long, indicating that suitable conditions for kelps can be found throughout the year (Supplementary material, Fig. S1A). The same was observed for the main perennial kelp species from Northern Brittany (L. digitata, Fig. S1B and L. hyperborea, Supplementary Fig. S1C).
Culture
Zoospore germination varied significantly with time (PERMANOVA, time, P < 0.0001, PERMDISP, time, P < 0.0001, Supplementary Fig. S2) apart from site of origin and temperature conditions (PERMANOVA, temperature and site, P = 0.8333). Maximum germination (27.7%) was observed between day 0 and 1. Most germination took place until day 3, after which it decreased below 0.1%. No significant effect of temperature or site of origin on germination was observed (PERMANOVA, temperature, P = 0.8526 and site, P = 0.8796; PERMDISP, temperature, P = 0.8968 and site, P = 0.7597). Mortality of microscopic stages increased significantly with temperature (PERMANOVA, temperature, P = 0.0111; PERMDISP, temperature, P = 0.098, Supplementary Fig. S3). This was maximal for 25°C, where no sporophytes were obtained due to mortality. At day 11, this reached 95% and 90% for the French and Portuguese sets, respectively. Mortality of sporophytes larger than 0.02 mm was significantly different between sites and temperatures (PERMANOVA, site, P = 0.0016 and temperature, P = 0.0022; PERMDISP, site, P = 0.0786 and temperature, P = 0.428, Supplementary Fig. S4). 60.6% of sporophytes from Northern Portugal died while for Northern Brittany mortality of sporophytes was about 32.8%. Sporophyte mortality was significantly higher at 20°C, reaching 75.5%. No significant difference was found for the remaining temperatures. Zoospores from Brittany developed into sporophytes more successfully (21.8%, PERMANOVA, site, P = 0.0224, PERMDISP, site, P = 0.5714, Supplementary Fig. S5) than those from Portugal (9.7%), but no temperature effect was detected (PERMANOVA, temperature, P = 0.1508; PERMDISP, temperature, P = 0.087). Sex ratio was on average 0.5 (males per female), significantly different from 1 (P = 0.0381) and was independent of site of origin (PERMANOVA, site, P = 0.5097; PERMDISP, site, P = 0.985) and temperature (PERMANOVA; temperature, P = 0.4609; PERMDISP, temperature, P = 0.435).
Although sporophyte growth rate for Northern Portugal and Brittany varied significantly with temperature for day 6 (considering sporophyte formation as day 0), (PERMANOVA, temperature, P = 0.0002, ), such differences might have been due to dispersion (PERMDISP, temperature, P < 0.0001). From day 6 to 12, sporophyte growth rate varied significantly with site and temperature (PERMANOVA site × temperature, P = 0.0223, ) but differences in dispersion were also significant between sites (PERMDISP, site, P = 0.2234). Significant variation was, however, found between temperatures for this period (PERMANOVA, temperature, P = 0.0015; PERMDISP, temperature, P = 0.0044) and from day 12 to 18 with site and temperature (PERMANOVA, site, P = 0.0135, and temperature, P < 0.0001); significant difference among temperatures may however be due to dispersion (PERMDISP, site, P = 0.6093; temperature, P = 0.0096; ). Optimum temperatures for sporophyte growth were found between 15°C and 10°C. The time needed for a sporophyte to reach 5 cm, big enough to be identified in the field, varied significantly only with temperature (PERMANOVA, Temperature, P = 0.0002). 10°C was the temperature at which they grew fastest, reaching 5 cm in about 20 days. The lowest growth rate was found at 5°C, at which zoospores needed on average 62 days to develop into a 5 cm sporophyte ().
DISCUSSION
In this study, we found that life history differences between southern and central populations were determined by timing of sporophyte recruitment, which took place year-round in Brittany whereas farther south, sporophytes only developed during spring. Our results show that seasonal recruitment in southern populations is not due to temperature limitations for microscopic development of the phases prior to macroscopic sporophyte recruitment. We also showed that the delay of recruitment until spring is not due to synchronized zoospore release in early autumn, as in our experiments zoospores were able to complete all stages of development into gametophytes and new sporophytes in less than a month. These results raised the novel hypothesis that recruitment is delayed until spring due to nutrient limitation in southern areas. This hypothesis is supported by the observation that sporophyte development is coincident with the upwelling season in the southern range, whereas in the central range nutrient levels are higher year-round (Supplementary Fig. S6).
The results from this study on marine forests are in accordance with previous studies relating seasonality of life history parameters to typical upwelling patterns at low latitudes (Fernandez et al., Citation1988; Lüning, Citation1990). Our results show that on the Portuguese coast, populations of S. polyschides have strict seasonality, with most individuals recruiting synchronously in early spring. The population structure surveys revealed that individuals grow rapidly and by the end of the summer most are reproductive. As such, by the beginning of autumn, when decay becomes evident and their stipes break due to the effects of the first storms, most individuals are already able to produce zoospores. On the contrary, although in Northern Brittany the population is semelparous, sporophytes are able to survive, reproduce and recruit over the winter, like the dominant perennial kelp species. The synchrony in life cycle in southern areas is apparently caused by the absence of sporophytes for part of the year. Although monitoring was done in limited areas, observations were consistent both with previous years and the surrounding areas including the whole northern Portuguese coast and between populations 2 km apart in Roscoff.
One of the reasons that could explain the inability of S. polyschides to recruit year-round on the Portuguese coast would be inadequate conditions for kelp recruitment. However, year-round recruitment in L. ochroleuca on this coast suggests that this is not the case. Although these two species have very different vegetative and holdfast structures that secure them to the substrate, in small recruits these are quite similar; both adhere to the substrate via rhizoids, and only later the attachment disc in S. polyschides develops into a bulb, as an additional attachment structure. Therefore, based on the lack of evidence for ecological differences between recruits of both species, we hypothesize that while their ability to support hydrodynamic forces might differ in adults, small recruits are likely to have similar resistance. Temperature is also unlikely to be a cause of the differences in recruitment between these species since they share a similar southern distribution limit and have similar upper temperature limits (Norton, Citation1977; Lüning, Citation1990).
The distribution of S. polyschides sporophytes on the Moroccan, Galician and Bay of Biscay coasts is usually thought to be limited by temperature; it varies between years and is dependent on upwelling (Fernandez et al., Citation1988; Lüning, Citation1990; Fernández, Citation2011). Notwithstanding, sporophytes survive during August, when SSTs may reach 23°C in the Bay of Biscay and 25°C in Casablanca. In these regions, although populations are exposed to higher temperatures for several months, their decay does not occur in midsummer but with the first autumn storms, like in North Portugal (Fernandez et al., Citation1988). Also, the fact that they usually break with the first autumn storms indicates that the end of their life cycle is caused by excessive hydrodynamic force. While this explains why decay of individuals is a synchronized event, it does not offer an explanation for the synchrony in recruitment. Elevated temperature cannot be an explanation for the different life histories between populations from Brittany and Portugal either, since they reach similar maximum values (19°C in summer). Although exposure to maximum temperatures is longer in northern Portugal, these observations do not support the hypothesis that high temperature is the cause of macroscopic sporophyte decay in Portugal by the end of summer. While satellite data have limitations when studying coastal areas, as local extreme conditions are not recorded (Smale & Wernberg, Citation2009; Smit et al., Citation2013), such short-term extreme temperatures are probably not the key limiting factor. Seasonality of S. polyschides is common to the whole northern Portuguese coast and is not a local event. The fact that it occurs on the Moroccan coast supports the theory that decay is unrelated to temperature. Even at the edge of geographic distribution individuals are reported to decay by the end of summer and not during warmer days in mid-summer (Norton & Burrows, Citation1969; Fernandez et al., Citation1988).
Since the environmental requirements of macroscopic and microscopic parts of the kelp life cycle may differ, we hypothesized that responses of microscopic stages to temperature might differ between populations from Portugal and Brittany and thus explain why recruitment is synchronous at low latitudes but occurs all year long at higher ones. Germination rate and sex ratio did not vary with site of origin or temperature, thus indicating no differences in adaptation to the different conditions between the two populations. Sex ratio results were however unexpected as twice as many females than males were found. Although it is commonly agreed that sex ratio in kelps is mainly genetically determined (Evans, Citation1963), some dissimilarity in number of males and females may occur due to stressful environmental conditions (Funano, Citation1983; Lee & Brinkhuis, Citation1988; Nelson, Citation2005; Oppliger et al., Citation2011). In a previous report, it was shown that in this species the frequency of females, higher during the first 10 days of germination due to their faster development, decreased to reach a similar number of males and females 1 month after germination (Pereira et al., Citation2011). As culturing conditions (10 and 15ºC) were similar to those in Pereira et al. (Citation2011), we hypothesize that sex ratio might be affected by seasonal or inter-annual variation, which in the present study resulted in rapid fertilization associated with reduced vegetative growth of the gametophytes. Our data on zoospore development into 0.5 cm sporophytes showed no significant differences between the two populations in mortality of microscopic stages. For both populations, mortality of microscopic stages was, however, significantly higher at 25°C, causing the death of all zoospores before any gametophytes were formed. At the remaining temperatures there were no differences in sporophyte production, within or between populations; sporophytes were successfully produced in both, indicating that temperatures between 5 and 20°C are suitable for the development of the gametophytic phases of S. polyschides. However, 20°C was not suitable for development of sporophytes larger than 0.02 mm for the population from Portugal for which mortality was absolute in such conditions. These mortality differences at 20°C may cause lower summer recruitment, but cannot explain the lack of recruitment during winter in Portugal. However, the lack of survival of young sporophytes at 20°C may explain why recruitment takes place only in spring and no new recruits are found throughout summer.
Since, in Portugal, most zoospores are released at about the same time, one possible explanation for the absence of recruitment for most of the year and a synchronous event around April/May would be the time lapse between zoospore release and recruitment. This could potentially be the time needed for zoospores to develop through the gametophyte stages into a sporophyte big enough to be observed in the field. However, the synchronous recruitment peak in spring cannot be explained by the synchronized zoospore release event at the end of summer. Although no significant differences in growth rate of sporophytes could be found between temperatures, growth reached a maximum for 10°C and a minimum at 5°C. This indicates that temperature has a greater impact on the rate of gametophyte development. After sporophytes are formed, growth rate does not vary significantly with temperature. Ten and 15°C represent the average seawater winter temperature in France and Portugal, respectively. Zoospores developing in these conditions needed, on average, 19 and 26 days, respectively, to develop into visible sporophytes (5 cm). Such time lapse is not sufficient to explain why no recruitment is observed between August and April in Portugal. It is important to keep in mind that growth rate is likely to vary with individual size, and our results show that they are likely to increase over time (). Nevertheless, many factors influence the development of the microscopic stages: shading is known to inhibit growth, which, in the field, is expected to be halted with sporophyte decay; natural light and water motion may result in faster development; and lower nutrient concentration, when compared with the enriched culture medium, may be limiting to growth (Edwards, Citation2000; Andersen, Citation2005; Pedersen et al., Citation2012; Carnell & Keough, Citation2014). As such, extrapolation of the results obtained in controlled conditions to the field should be made with caution.
Fig. 3. Number of days needed, from the time of induced zoospore release, for a sporophyte big enough to be observed in the field (5 cm) to develop, for the populations from North Portugal (Pt) and Brittany, France (Fr) at the four temperatures where sporophytes were formed. Significant differences were found between all temperatures. No data for the Portuguese set at 20°C are displayed due to 100% mortality.
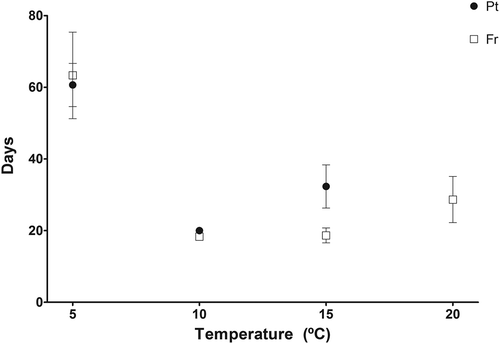
Despite the poor correlation of autumn decay of southern populations with temperature exposure, the life cycle of S. polyschides seems highly correlated with upwelling events, in agreement with previous studies (Fernandez et al., Citation1988; Lüning, Citation1990; Fernández, Citation2011). In northern Portugal, the typical upwelling season begins in April, when the species starts recruiting, and ends abruptly in September, when sporophytes decay and break with the first autumn storms (Vitorino et al., Citation2002; Lemos & Pires, Citation2004). Since temperature does not seem limiting, we hypothesize that the life cycle in these regions might be more closely related to and limited by nutrient concentrations, which increase during the upwelling period. Although the available information is limited, this hypothesis is supported by the comparison of average nutrient concentrations off the coastlines where S. polyschides occurs, showing a trend in relation to the presence or absence of overwintering populations (Supplementary Fig. S6). In the areas off Ireland, Isle of Man and Brittany, where higher concentrations of both phosphates and nitrates are found throughout the year, individuals are able to overwinter. In contrast, in areas with low nutrient concentrations, the occurrence of the macroscopic part of the life cycle coincides with the typical upwelling season, when nutrient levels rise. In these areas, from the Bay of Biscay to Portugal and in the upwelling areas of Morocco, there is typically no upwelling during roughly half of the year, coincident with the absence of sporophytes of S. polyschides. As such, we propose that the differences in life histories between northern and southern ranges are due to seasonal nutrient limitation in the southern but not in the northern range, keeping in mind the possibility of this resulting from the interaction with other environmental factors (Gerard, Citation1997). We hypothesize that in Portugal, the life cycle of S. polyschides is limited by nutrient concentration and, as such, dependent on upwelling events that seasonally bring high nutrient concentrations. Therefore, global warming and associated geographic distribution shifts might not be due to temperature increase alone but also to the associated nutrient limitation due to interruption or weakening of upwelling systems (Johnson et al., Citation2011). This is supported by several studies that indicate that the high rate at which the North Atlantic has been warming during the past 40 years has been especially affecting winter temperatures and weakening upwelling systems (Hurrell & Van Loon, Citation1997; Hurrell et al., Citation2001; Visbeck et al., Citation2001; Lemos & Pires, Citation2004; Johnson et al., Citation2011). These alterations in the upwelling season might be predicted to deregulate seasonality of S. polyschides. A possible consequence that is a serious concern would be that in some areas this kelp might recruit later than usual and be unable to develop to reproductive age before decaying by the end of summer, as was observed in 2010. Such an impact on structural species may cause their local extinction and the subsequent disappearance of several species that rely on them for food and protection.
SUPPLEMENTARY INFORMATION
The following supplementary material is accessible via the Supplementary Content tab on the article’s online page at
Supplementary Fig. S1: Number of recruits and reproductive and non-reproductive individuals per square metre for L. ochroleuca from North Portugal (A) and L. digitata (B) and L. hyperborea (C) from Brittany.
Supplementary Fig. S2: Germination rate in relation to time.
Supplementary Fig. S3: Mortality of microscopic stages (spores, gametophytes and sporophytes smaller than 0.02 mm) in relation to temperature.
Supplementary Fig. S4: (A) Macroscopic sporophyte (longer than 0.02 mm) mortality in relation to temperature.
Supplementary Fig. S5: Number of sporophytes obtained from 100 initial zoospores varied between sites of origin only (PERMANOVA, site, P = 0.023)
Supplementary Fig. S6: (A) Average yearly concentration of nitrates (B) and phosphates, in µmol l–1, for areas where the presence/absence of overwintering individuals of S. polyschides is described (NOAA/OAR/ESRL PSD, Boulder, Colorado, USA, from their website at http://www.esrl.noaa.gov/psd/).
ACKNOWLEDGEMENTS
We thank the Ecokelp partners from the Station Biologique de Roscoff, Carolyn Engel, Laurent Lévèque and the Service Mer and Observation of the Station Biologique de Roscoff, for collecting the field data on the northern kelp populations.
Biological material from Brittany, used to establish the zoospore culture, was sent to the Algarve Centre of Marine Sciences (CCMAR) from Station Biologique de Roscoff with ASSEMBLE remote access, grant agreement no. 227799.
Additional information
Funding
Notes on contributors
Tânia R. Pereira
Tânia R. Pereira: original concept, field monitoring, culture experiments, data analysis, drafting and editing manuscript; Aschwin H. Engelen: Concept, field monitoring methodology, editing manuscript; Gareth A. Pearson: Concept, editing manuscript; Myriam Valero: Original concept, editing manuscript; Ester A. Serrão: Original concept, editing manuscript.
Aschwin H. Engelen
Tânia R. Pereira: original concept, field monitoring, culture experiments, data analysis, drafting and editing manuscript; Aschwin H. Engelen: Concept, field monitoring methodology, editing manuscript; Gareth A. Pearson: Concept, editing manuscript; Myriam Valero: Original concept, editing manuscript; Ester A. Serrão: Original concept, editing manuscript.
Gareth A. Pearson
Tânia R. Pereira: original concept, field monitoring, culture experiments, data analysis, drafting and editing manuscript; Aschwin H. Engelen: Concept, field monitoring methodology, editing manuscript; Gareth A. Pearson: Concept, editing manuscript; Myriam Valero: Original concept, editing manuscript; Ester A. Serrão: Original concept, editing manuscript.
Myriam Valero
Tânia R. Pereira: original concept, field monitoring, culture experiments, data analysis, drafting and editing manuscript; Aschwin H. Engelen: Concept, field monitoring methodology, editing manuscript; Gareth A. Pearson: Concept, editing manuscript; Myriam Valero: Original concept, editing manuscript; Ester A. Serrão: Original concept, editing manuscript.
Ester A. Serrão
Tânia R. Pereira: original concept, field monitoring, culture experiments, data analysis, drafting and editing manuscript; Aschwin H. Engelen: Concept, field monitoring methodology, editing manuscript; Gareth A. Pearson: Concept, editing manuscript; Myriam Valero: Original concept, editing manuscript; Ester A. Serrão: Original concept, editing manuscript.
REFERENCES
- Andersen, R.A. (2005). Algal Culturing Techniques. Amsterdam: Elsevier Academic Press.
- Anderson, M.J., Gorley, R.N. & Clarke, K.R. (2008). PERMANOVA+ for PRIMER. Guide to Software and Statistical Methods. PRIMER-E Ltd, Plymouth.
- Ardré, F. (1970). Contribution a l’étude des algues marines du Portugal. I: La Flore. Portugalia Acta Biologica, 10: 137–555.
- Assis, J., Coelho, N., Alberto, F., Valero, M., Raimondi, P., Reed, D. & Serrão, E.A. (2013). High and distinct range edge genetic diversity despite local bottlenecks. PLoS ONE, 8: e68646. doi:10.1371/journal.pone.0068646.
- Bakun, A. (1990). Global climate change and intensification of coastal ocean upwelling. Science, 247: 198–201.
- Barth, J.A., Menge, B.A., Lubchenco, J., Chan, F., Bane, J.M., Kirincich, A.R., McManus, M.A., Nielsen, K.J., Pierce, S.D. & Washburn, L. (2007). Delayed upwelling alters nearshore coastal ocean ecosystems in the Northern California current. Proceedings of the National Academy of Sciences USA, 104: 3719–3724.
- Bates, A.E., Pecl, G.T., Frusher, S., Hobday, A.J., Wernberg, T., Smale, D.A., Sunday, J.M., Hill, N.A., Dulvy, N.K., Colwell, R.K., Holbrook, N.J., Fulton, E.A., Slawinski, D., Feng, M., Edgar, G.J., Radford, B.T., Thompson, P.A. & Watson, R.A. (2014). Defining and observing stages of climate-mediated range shifts in marine systems. Global Environmental Change, 26: 27–38.
- Brommer, J.E. (2004). The range margins of northern birds shift polewards. Annales Zoologici Fennici, 41: 391–397.
- Carnell, P.E. & Keough, M.J. (2014) Spatial variable synergistic effects of disturbance and additional nutrients on kelp recruitment and recovery. Oecologia, 175: 409–416.
- Carney, L.T. (2011). A multispecies laboratory assessment of rapid sporophyte recruitment from delayed kelp gametophytes. Journal of Phycology, 47: 244–251.
- Carney, L.T. & Edwards, M.S. (2010). Role of nutrient fluctuations and delayed development in gametophyte reproduction by Macrocystis pyrifera (Phaeophyceae) in southern California. Journal of Phycology, 46: 987–996.
- Chapman, A.R.O. (1986) Age vs. stage an analysis of age-specific and size-specific mortality and reproduction in a population of Laminaria longicruris. Journal of Experimental Marine Biology and Ecology, 97: 113–122.
- Chen, C.T.A. (2000). The Three Gorges Dam: reducing the upwelling and thus productivity in the East China Sea. Geophysical Research Letters, 27: 381–383.
- Chen, I.C., Hill, J.K., Ohlemüller, R., Roy, D.B. & Thomas, C.D. (2011). Rapid range shifts of species associated with high levels of climate warming. Science, 333: 1024–1026.
- Edwards, M.S. (2000). The role of alternate life-history stages of a marine macroalga: a seed bank analogue? Ecology, 81: 2404–2415.
- Edwards, M. & Richardson, A. (2004). Impact of climate change on marine pelagic phenology and trophic mismatch. Nature, 430: 881–884.
- Evans, L. (1963). A large chromosome in the laminarian nucleus. Nature, 198: 215.
- Fernández, C. (2011). The retreat of large brown seaweeds on the north coast of Spain: the case of Saccorhiza polyschides. European Journal of Phycology, 46: 352–360.
- Fernandez, J.A., Perez-Celorrio, B. & Ibañez, M. (1988). Sobre la presencia de Saccorhiza polyschides (Light) Batt. En la costa guipuzcoana. ¿Especie indicadora de cambios climáticos? Lurralde, 11: 201–216.
- Funano, T. (1983). The ecology of Laminaria religiosa Miyabe. I. The life history and alternation of nuclear phases of Laminaria religiosa, and the physiological ecology of the gametophytes and the embryonal sporophytes. Hokusui-Shiho, 25: 61–109.
- Gerard, V. (1997). The role of nitrogen nutrition in high-temperature tolerance of the kelp, Laminaria saccharina (Chromophyta). Journal of Phycology, 33: 800–810.
- Gómez-Gesteira, M., de Castro, M., Álvarez, I., Lorenzo, M.N., Gesteira, J.L.G. & Crespo, A.J.C. (2008). Spatio-temporal upwelling trends along the Canary Upwelling System (1967–2006). Annals of the New York Academy of Sciences, 1146: 320–337.
- Hoffman, A.J. & Santelices, B. (1991). Bank of algal microscopic forms: hypothesis on their functioning and comparison with seed banks. Marine Ecology Progress Series, 79: 185–194.
- Hurrell, J.W. & Van Loon, H. (1997). Decadal variations in climate associated with the North Atlantic Oscillation. Climatic Change, 36: 301–326.
- Hurrell, J.W., Kushnir, Y. & Visbeck, M. (2001). The north Atlantic oscillation. Science, 291: 603–605.
- Jensen, A., Indergaards, M. & Holt, T.J. (1985). Seasonal variation in the chemical composition of Saccorhiza polyschides (Laminariales, Phaeophyceae). Botanica Marina, XXVIII: 375–281.
- Johnson, C.R., Banks, S.C., Barrett, N.S., Cazassus, F., Dunstan, P.K., Edgar, G.J., Frusher, S.D., Gardner, C., Haddon, M., Helidoniotis, F., Hill, K.L., Holbrook, N.J., Hosie, G.W., Last, P.R., Ling, S.D., Melbourne-Thomas, J., Miller, K., Pecl, G.T., Richardson, A.J., Ridgway, K.R., Rintoul, S.R., Ritz, D.A., Ross, D.J., Sanderson, J.C., Shepherd, S.A., Slotwinski, A., Swadling, K.M. & Taw, N. (2011). Climate change cascades: shifts in oceanography, species’ ranges and subtidal marine community dynamics in eastern Tasmania. Journal of Experimental Marine Biology and Ecology, 400: 17–32.
- Kain, J.M. (1989). The seasons in the subtidal. British Phycological Journal, 24: 203–215.
- Kinlan, B.P., Graham, M.H., Sala, E. & Dayton, P.K. (2003). Arrested development of giant kelp (Macrocystis pyrifera, Phaeophyceae) embryonic sporophytes: a mechanism for delayed recruitment in perennial kelps? Journal of Phycology, 39: 47–57.
- Lee, J.A. & Brinkhuis, B.H. (1988). Seasonal light and temperature interaction effects on development of Laminaria saccharina (Phaeophyta) gametophytes and juvenile sporophytes. Journal of Phycology, 24: 181–191.
- Lemos, R.T. & Pires, H.O. (2004). The upwelling regime off the West Portuguese Coast, 1941–2000. International Journal of Climatology, 24, 511–524.
- Lüning, K. (1990). Seaweeds. Their Environment, Biogeography and Ecophysiology. Wiley, New York.
- Maier, I. & Müller, D.G. (1986). Sexual pheromones in algae. Biological Bulletin, 170: 145–175.
- McGregor, H.V., Dima, M., Fischer, H.W. & Mulitza, S. (2007). Rapid 20th century increase in coastal upwelling of Northwest Africa. Science, 315: 637–639.
- Mohring, M.B., Kendrick, G.A., Wernberg, T., Rule, M.J. & Vanderklift, M.A. (2013). Environmental influence on kelp performance across reproductive period: an ecological trade-off between gametophyte survival and growth? PLOS ONE, 8(6): e65310.
- Müller, R., Laepple, T., Bartsch, I. & Wiencke, C. (2009). Impact of oceanic warming on the distribution of seaweeds in polar and cold-temperate waters. Botanica Marina, 52: 617–638.
- Narayan, N., Paul, A., Mulitza, S. & Schulz, M. (2010). Trends in coastal upwelling intensity during the late 20th century. Ocean Science Discussions, 7: 335–360.
- Nicastro, K.R., Zardi, G.I., Teixeira, S., Neiva, J., Serrão, E.A. & Pearson, G.A. (2013). Shift happens: trailing edge contraction associated with recent warming trends threatens a distinct genetic lineage in the marine macroalga Fucus vesiculosus. BMC Biology, 11: 1–13.
- Nelson, W.A. (2005). Life history and growth in culture of the endemic New Zealand kelp Lessonia variegata J. Agardh in response to different regimes of temperature, photoperiod and light. Journal of Applied Phycology, 17: 23–28.
- Norton, T.A. (1977). Experiments on the factors influencing the geographical distribution of Saccorhiza polyschides and Saccorhiza dematodea. New Phytologist, 78: 625–635.
- Norton, T.A. (1992). Dispersal by macroalgae. British Phycological Journal, 27: 293–301.
- Norton, T.A. & Burrows, E.M. (1969). Studies on the marine algae of the British Isles. 7. Saccorhiza polyschides(Lightf.) Batt. British Phycological Journal, 4: 19–53.
- Oppliger, V.L., Correa, J.A., Faugeron, S., Beltrán, J., Tellier, F., Valero, M. & Destombe, C. (2011). Sex ratio variation in the Lessonia nigrescens complex (Laminariales, Phaeophyceae): effect of latitude, temperature and marginality. Journal of Phycology, 47: 5–12.
- Pedersen, M.F., Nejrup, L.B., Fredriksen, S., Christie, H. & Norderhaug, K.M. (2012). Effects of wave exposure on population structure, demography, biomass and productivity of the kelp Laminaria hyperborea. Marine Ecology Progress Series, 451: 45–60.
- Pereira, T.R., Engelen, A.H., Pearson, G.A., Serrão, E.A., Destombe, C. & Valero, M. (2011). Temperature effects on the microscopic haploid stage development of Laminaria ochroleuca and Saccorhiza polyschides, kelps with contrasting life histories. Cahiers de Biologie Marine, 52: 395–403.
- Reed, D.C., Anderson, T.W., Ebeling, A.W. & Anghera, M. (1997).The role of reproductive synchrony in the colonization potential of kelp. Ecology, 78: 2443–2457.
- Smale, D.A. & Wernberg, T. (2009). Satellite-derived SST data as a proxy for water temperature in nearshore benthic ecology. Marine Ecology Progress Series, 387: 27–37.
- Smale, D.A., Burrows, M.T., Moore, P., O’Connor, N. & Hawkins, S.J. (2013). Threats and knowledge gaps for ecosystem services provided by kelp forests: a northeast Atlantic perspective. Ecology and Evolution, 3: 4016–4038.
- Smit, A.J., Roberts, M., Anderson, R.J., Dufois, F., Dudley, S.F.J., Bornman, T.G., Olbers, J. & Bolton, J.J. (2013). A coastal seawater temperature dataset for biogeographical studies: large biases between in situ and remotely-sensed data sets around the coast of South Africa. PloS ONE 8: e81944.
- Stæhr, P.A. & Wernberg, T. (2009). Physiological responses of Eklonia radiata (Laminariales) to a latitudinal gradient in ocean temperature. Journal of Phycology, 45: 91–99.
- Tuya, F., Cacabelos, E., Duarte, P., Jacinto, D., Castro, J.J., Silva, T., Bertocci, I., Franco, J.N., Arenas, F., Coca, J. & Wernberg, T. (2012). Patterns of landscape and assemblage structure along a latitudinal gradient in ocean climate. Marine Ecology Progress Series, 466: 9–19.
- Visbeck, M.H., Hurell, J.W., Polvani, L. & Cullen, H.M. (2001). The North Atlantic Oscillation: past, present and future. Proceedings of the National Academy of Sciences USA, 98: 12876–12877.
- Vitorino, J., Oliveira, A., Jouanneau, J.M. & Drago, T. (2002). Winter dynamics in the northern Portuguese shelf. Part 1: physical processes. Progress in Oceanography, 52: 129–153.
- Wernberg, T., Thomsen, M.S., Tuya, F., Kendrick, G.A., Stæhr, P.A. & Toohey, B.D. (2010). Decreasing resilience of kelp beds along a latitudinal temperature gradient: potential implications for a warmer future. Ecology Letters, 13: 685–694.
- Wernberg, T., Russell, B.D., Moore, P.J., Ling, S.D., Smale, D.A., Campbell, A., Coleman, M.A., Steinberg, P.D., Kendrick, G.A. & Connell, S.D. (2011). Impacts of climate change in a global hotspot for temperate marine biodiversity and ocean warming. Journal of Experimental Marine Biology and Ecology, 400: 7–16.