Abstract
The green filamentous alga Ulothrix zonata inhabits Lake Baikal and overgrows the underside of hummocky ice during winter and spring. To clarify physiological adaptations of U. zonata to low temperature and the potential contribution of membrane water permeabilities, the expression level of aquaporins from a winter population growing under the lake ice and in interstitial water was compared with that of benthic summer and autumn populations of algae by immuno-detection using an anti-maize PIP1 (plasma membrane intrinsic protein) antiserum. Algal, and in particular Ulvophycean, transcriptome sequences are only partially available and this higher plant-specific PIP1 epitope has not been detected so far in available genomic datasets. PIP1 expression levels were highest in the ice population and the rise in water temperature during summer was correlated with a drop in PIP1 amounts, which slightly increased again in autumn. ITS2 rDNA (internally transcribed spacer region 2) sequences revealed conspecificity of the ice and summer populations. The difference in PIP1 aquaporin expression suggests adaptation of the algae to changing environments by maintaining membrane permeability at low temperatures. Our study shows that the ice population of U. zonata inhabiting Lake Baikal is a unique subject for studying physiological and biochemical adaptation mechanisms of freshwater extremophiles.
Abbreviations:
- bp
- basepairs
- BCIP
- 5-bromo-4-chloro-3-indolylphosphate p-toluidine salt
- DTT
- dithiothreitol
- FA
- fatty acids
- ITS2
- internally transcribed spacer
- LSU rDNA
- ribosomal large subunit gene
- MIP
- membrane intrinsic protein
- nBT
- nitrotetrazolium blue chloride/5-bromo-4-chloro-3-indolyl phosphate
- PIP
- plasma membrane intrinsic protein
- PMSF
- phenylmethylsulphonyl fluoride
- PVP
- polyvinylpyrrolidone
- TIP
- tonoplast intrinsic protein
Introduction
The filamentous green alga Ulothrix zonata (Weber et Mohr) Kütz. (Ulotrichales, Ulvophyceae) is a widely distributed species that typically inhabits the splash zone and shallow waters of temperate lakes. The species appears to be cold-adapted and typically shows seasonal growth patterns (Graham et al., Citation1985b). In Lake Baikal, U. zonata develops intensively in March–April on the lower surface of the ice sheet in Lake Baikal under relatively stable temperatures close to 0°С. It grows in abundance during the ice-free period (May–September) (Izhboldina, Citation1990) and during summer forms a dense cover on stones at the water’s edge, where the filaments are exposed to significant diurnal temperature fluctuations.
Figs 1–4. Changes in habitat and cell dimensions of Ulothrix zonata during different seasons. Figs 1, 2. Ice population in March. (Fig. 1) Ice undersurface overgrown by U. zonata. (Fig. 2) Lightly pigmented filaments of the ice population. Figs 3, 4. Benthic algal population. (Fig. 3) Stones overgrown by U. zonata. (Fig. 4) Short-celled, densely pigmented U. zonata filaments of the summer population. Scale bars: Figs 2 & 4, 100 µm.
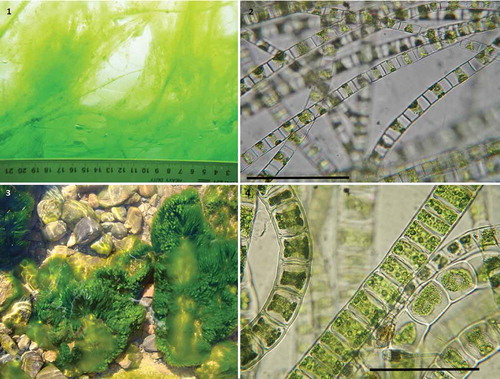
The ability of plants to maintain the transmembrane water flow may be among the key factors linked to cold tolerance. In land plants that are sensitive to cold soils, growth reduction is accompanied by a sharp reduction of water flux which is thought to occur because of higher water viscosity and the inhibition of transmembrane water transport (Lee et al., Citation2012). Plant aquaporins, members of the membrane intrinsic protein (MIP) family, have been shown to be involved in the regulation of the cellular water exchange across membranes (Javot et al., Citation2003; Kaldenhoff et al., Citation2008; Moshelion et al., Citation2009; Postaire et al., Citation2010). Evidence has been found for changes in aquaporin expression in response to abiotic stresses, and different mechanisms regulating aquaporin activity and their abundance in membranes have been identified (Maurel et al., Citation2008; Kaldenhoff et al., Citation2008; Katsuhara et al., Citation2008). Aquaporin-mediated water transport in roots of Arabidopsis thaliana was linked to growth at low temperatures (Lee et al., Citation2012). Aquaporins are a large and evolutionarily conserved superfamily of membrane proteins found in almost all organisms, including eubacteria, archaea, fungi, animals and plants (Agre & Kozono, Citation2003). Seven subfamilies of MIPs are known to date (Danielson & Johanson, Citation2008). Recently, a survey of the known genomes of green algae revealed a high complexity of MIP proteins in these organisms (Anderberg et al., Citation2011).
The physiological acclimatization and adaptation of algae from freshwater ice communities remain insufficiently studied. Previously, ice and benthic populations of U. zonata from Lake Baikal were shown to differ from marine Ulothrix species by having a high content of polyunsaturated fatty acids (FA) and seasonal fluctuations in water temperature had only a negligible effect on their total amount (Osipova et al., Citation2009). The active control of water flow may be crucial for adaptation to cold temperatures but the details of the mechanisms are unknown (Maurel et al., Citation2008). Water movement across the plasma and tonoplast membranes is mediated by aquaporins, with various members of this gene family specific for development, stress and cellular distribution. In the literature, there are a number of data on the important role of aquaporins belonging to the PIP family in adaptation to low temperatures. Cold treatment down-regulated the expression of most PIP genes in Arabidopsis (Jang et al., Citation2004) and in wheat leaves, a large increase in PIP transcript levels was seen after cold acclimation (from 22ºC to 4ºC) (Herman et al., Citation2006).
In this study, we were interested in the detection of aquaporins related to plasma membrane intrinsic proteins (PIPs) from U. zonata, Lake Baikal, and the possible seasonal variation in their abundance.
Materials and Methods
Chemicals
Ponceau S, Tween-20, DTT, nBT, BCIP and SigmaMarker (Wide Range 6500–200 000 Da) were purchased from Sigma Inc. (St. Louis, Missouri). PMSF were obtained from Calbiochem (USA); PVP from Biomedicals (Germany); nitrocellulose membranes Hybond ECh from Amersham Biosciences (UK); secondary antibodies conjugated to alkaline phosphatase from Promega (USA). The primary antisera used were anti-AtTIP antibodies from Agrisera, Sweden (product AS09 493) recognizing a C-terminal γ-TIP (TIP1) peptide INQNGHEQLPTTDY and rabbit anti-ZmPIP1 antibodies directed against a conserved N-terminal peptide of ZmPIP1 isoforms MEGKEEDVRLGANKFSERQPIGTAAQGTDDKDYKEPPPAPLFEP (Martinez-Ballesta et al., Citation2008). For control of specificity, we used blocking of the antisera with the recombinant GST-ZmPIP1-N-terminus fusion protein, which had been used to raise the antibodies, and the TIP1;1, TIP1;2 (tonoplast intrinsic protein) blocking peptide from Agrisera (product AS09 493P), respectively.
Plant materials
Ulothrix zonata was sampled from the lower surface of the ice cover (0.9–1 m thick) of Lake Baikal in March and April 2006 by scuba diving and from stones at the water’s edge in July 2006, July and October 2007 and on 10 and 19 October 2013. All samples were cleared of visible impurities and transported to the laboratory in a thermostable container within 1 h. The samples were dried on filter paper (Bio-Rad) and then the algae were frozen in liquid N2 and stored at −70ºС until extraction. Approximately 96% of the under-ice samples (March and April 2006), 95% of the samples collected in July 2006 and 2007 and 97–99% of those from October 2007 and 2013 were composed of U. zonata filaments with a negligible amount of epiphytic diatoms. Arabidopsis thaliana leaves were used as a positive control.
Cell size measurements
Measurements were taken of the length and width of 50 cells selected from each population at random under a ‘Peraval’ light microscope (Carl Zeiss Jena, Germany) at 800× magnification. To estimate the cell volume (V) and surface (S), the closest geometric figure (i.e. cylinder) was chosen and calculations were performed using the formulae V= πr2l and S = 2πrl + 2πr2, where r is the radius of cell, and l is the length of cell.
Water temperature monitoring
Temperature was continuously recorded at 1 h intervals at the sampling sites with autonomous programmable sensors (Stow Away TidBit Loggers, OnSet Corp., Cape Cod, Massachusetts), both at the water’s edge and at a depth of 3.6 m. Water temperature at the water’s edge was measured with a mercury thermometer at negative air temperatures (e.g. in October 2007).
Preparation of protein extracts
Frozen algae were homogenized with high-silica sand and buffer (pH 7.5), containing 50 mM Tris-HCl, 5 mM EDTA, 1 mM PMSF, 2 mM DTT, 0.1% PVP and 0.5 M sucrose in 1:1 ratio (w/v) for 20 min. The homogenate was sonicated (40 kHz for 20 min) and centrifuged at 10 000 g for 20 min, the supernatant fraction was centrifuged at 100 000 g for 1 h in an ultracentrifuge (Sorvall Discovery 90SE, USA). The resulting pellet (microsomal proteins) was re-suspended in 5 mM Tris-НСl buffer (рН 7.5), containing 0.33 M sucrose and 2 mM DTT. A microsomal fraction was taken from leaves of Arabidopsis and used to control the western blot analysis. All procedures were performed at 4°C. Protein contents were determined as described by Bradford (Citation1976), using serum albumin as a calibration standard.
SDS-PAGE and western blot analysis
The microsomal membrane fraction was restored in a water bath at 60°С for 30 min in 0.5 M Tris-HCl buffer (pH 6.8), 1 mM EDTA, 0.001% bromophenol blue, 5% SDS, 20% glycerol and 200 mM DTT. Ten µg of protein extract was separated electrophoretically by 12% SDS-PAGE (Laemmli, Citation1970) and three µg of protein extract of Arabidopsis microsomal fraction was used. Electrophoresis was done using a mini-Protean PAGE cell (Bio-Rad) according to the manufacturer’s instructions. Western blotting and immunodetection were carried out as described by Timmons & Dunbar (Citation1990). Proteins in gels were transferred onto 0.2 μm nitrocellulose membranes in Towin buffer (pH 8.3; 25 mM Tris, 192 mM glycine, 20% (v/v) methanol). Transfer efficiency was determined by 0.5% Ponceau S solution in 0.1% acetic acid. Blots were blocked in a Tris-buffer saline containing non-fat dry milk (5% w/v) and 0.1% Tween-20 overnight at 4°C. Blots were then incubated with anti-ZmPIP1 or anti-AtTIP primary antibodies (dilution 1:1000) for 2 h at 20°C (Martinez-Ballesta et al., Citation2008). After washing for 10 min and another three times for 5 min with TTBS buffer (pH 7.5; 50 mM Tris, 500 mM NaCI, 0,05% Tween-20), blots were incubated with secondary antibodies Anti-Rabbit Ig G(Fe)AP (Promega) (dilution 1:1000) for 2 h at 20°C and immune complexes were detected with nitrotetrazolium. The results were visualized by using the mixture of nBT and BCIP for 5 min. After staining, the membrane was dried, and the results were analysed after scanning. For control of specificity, the diluted anti-ZmPIP1 antiserum was preincubated with 3 µg ml‒1 recombinant GST-ZmPIP1-N-terminus fusion protein for 15 min and then used to probe the Western blot. Similarly, the TIP1 peptide was used (2 µg ml−1) to test the specificity of the TIP1 signals. All experiments were replicated no less than four times.
DNA extraction and sequenсing
To address the question of conspecificity between winter and summer populations of Ulothrix from Lake Baikal, 10 samples representing different years, different seasons and different locations within and around Lake Baikal were selected for DNA sequencing (). Total genomic DNA was isolated using the Chelex method (Goff & Moon, Citation1993) from silica-preserved material. The first approximately 600 nucleotides of the ribosomal large subunit gene (LSU rDNA) were amplified using the universal primers C’1 forward (5’-ACCCGCTGAATTTAAGCATAT-3’; Leliaert, Citation2004) and D2 reverse (5’-TCCGTGTTTCAAGACGG-3’; Rousseau et al., Citation1997) at an annealing temperature of 56ºC; the complete internally transcribed spacer 2 (ITS2) region was amplified using the primer pair ITS3–ITS4 (White et al., Citation1990) at an annealing temperature of 53ºC. PCR amplifications were performed in a Biomed thermocycler with an initial denaturation step of 94°C for 5 min followed by 32 cycles of 1 min at 94°C, 1 min at 53/56°C, and 1 min at 72°C, with a final extension step of 10 min at 72°C. The reaction volume was 25 µl and consisted of approximately 0.1–0.4 µg genomic DNA, 1.25 nmol of each dNTP, 6 pmol of each primer, 1× reaction buffer containing 1.5 mM MgCl2 (New England Biolabs, USA), 5 µl BSA (2.5%), and one unit of Taq polymerase (New England Biolabs). Amplifications were checked by running them on 1% agarose gels and subsequent staining with ethidium bromide. PCR products were purified with ExoSAP-IT® (USB Corporation, USA). Cleaned PCR products were sent to Macrogen, South Korea, for sequencing. The final consensus sequences were constructed with Geneious Pro 5.1.5 (Biomatters Ltd, New Zealand), subsequently checked in Se-Al v2.0a11 (Rambaut, Citation2007) and submitted to EMBL/GenBank (see ).
Table 1. List of Ulothrix zonata specimens from Lake Baikal and surroundings for which ITS2 and partial LSU rDNA sequences have been determined.
Results
Water temperatures in the habitat of Ulothrix zonata
Measurements of the water temperature in the shoreline showed marked diurnal fluctuations: in July 2006, the water temperature changed from 5.5ºС at night to 11ºС during the day; in July 2007 from 5ºС at night to 15ºС during the day; in October 2007, the water temperature dropped to 6–8ºС and the night air temperature decreased to −5ºС. In March and April 2006, the under-ice water temperature ranged from 0.1–0.3ºС and did not vary significantly. The water temperature measured on October 10 and 19 2013 was 6–7ºС and 4.3ºС, respectively.
Cell dimensions of Ulothrix zonata
The cells of U. zonata from the ice population were elongated, with cell length exceeding the width by about 4.5 times, and contained large vacuoles ( and ). Cells from the summer populations were more compact and broader ( and ; see also ). The side surface of the cells was 1.5 times larger in the ice population. Fully grown cells from the benthic population (summer) were 1.3 times larger in volume than those of the ice population. This difference in the cell dimensions and vacuolation was correlated with different stages in the life history of U. zonata during different seasons, i.e. vegetative growth was vigorous in spring (still under the ice cover), whilst in summer the populations proliferated intensively by fragmentation and began to produce zoospores and later, gametes. Such extensive phenotypic plasticity, especially in filament diameter, has been documented in U. zonata previously (e.g. Lokhorst & Vroman, Citation1974; Shyam & Saxena, Citation1980). Phenotypic plasticity is pronounced in many green macroalgae, e.g. Ulva (Malta et al., Citation1999), Boodlea (Leliaert et al., Citation2009), Cladophora (van den Hoek, Citation1963), Aegagropila and Pithophora (Boedeker et al., Citation2012), Caulerpa (Ohba et al., Citation1992; De Senerpont Domis et al., Citation2003), and Spirogyra (McCourt & Hoshaw, Citation1990).
Table 2. Cell dimensions of Ulothrix zonata during ice cover period and open water at the ecological test site Berezovy in 2006.
Immunodetection of aquaporins
Aquaporins of the PIP and TIP subfamilies form pores in the cell membranes of plants and are involved in transcellular and intracellular water flow (Maurel et al., Citation2008). Western blots with anti-ZmPIP1 antibodies showed that the microsomal fraction of U. zonata included proteins homologous to the PIP1 subgroup of higher plants (). Signals at approximately 26 kDa and 50 kDa were revealed, which are diagnostic for PIP proteins, where after SDS polyacrylamide electrophoresis monomeric and dimeric forms are typically detected (Kammerloher et al., Citation1994; Daniels et al., Citation1996; Henzler et al., Citation1999) (). Pre-incubation of the antiserum with a recombinant GST-protein containing the PIP1-epitope abolished the Western blot signals (, ), which confirmed that the signals were derived from PIP1-related antigens. PIP1 abundance was highest during spring in algae growing on the underside of the ice sheet at a temperature close to 0°С; it decreased in summer and started to rise again in late October. Similar correlation of PIP1 in the algae and the ambient water temperature was observed in samples collected in late autumn 2013 – abundance of PIP1 increased with decrease of the water temperature from 7 to 4°С ().
Fig. 5. Western blotting of membrane proteins of Ulothrix zonata populations from Lake Baikal using anti-ZmPIP1 antibodies (a, c). Control of antiserum specificity (b, d). The anti-ZmPIP1 antiserum was pre-incubated with a recombinant GST-protein containing the PIP1-epitope and then used to probe the Western blot.
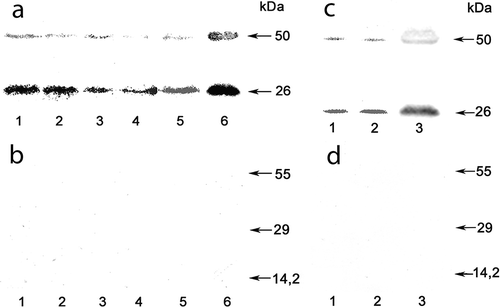
Anti-TIP1 antibodies recognizing a peptide conserved at the C-terminus of γ-TIP proteins detected a signal in the ice population (March and April 2006) with electrophoretic mobility typical for TIP proteins, showing the expected dimeric form at 42 kDa in addition to the monomer at 23 kDa (data not shown). Pre-incubation of the antiserum with a TIP1;1, TIP1;2 blocking peptide abolished the Western blot signals in Arabidopsis, but not U. zonata. Therefore, we cannot conclude unequivocally that proteins identified with anti-AtTIP antibodies are true homologues of aquaporins of the TIP subfamily.
Because the Ulothrix material used originated from natural habitats rather than cultures, the immunodetection was repeated six times and each time strong signals were obtained that cannot be attributed to trace contaminations (from 1 to 5% of epiphytic diatoms).
Conspecificity of ice- and summer populations and ecological differentiation
In order to clarify the relationship of ice- and summer populations, we performed rDNA sequencing of the Ulothrix zonata samples. The LSU rDNA sequences were 578 base pairs (bp) long and identical for all seven samples that were sequenced (). The 10 ITS2 rDNA sequences were 338 bp long and differed by a maximum of two bp (equivalent to less than 1% divergence): the two samples R18 (ice population, Lake Baikal) and Q38 (city of Irkutsk) had two point mutations at positions 203 and 276, while the other eight samples had identical sequences. However, the ITS2 sequence of sample Q42 (Ushkany Islands, Lake Baikal) showed two sites with intra-individual variation (positions 169 and 172), and sample Q32 (small river near Bolshie Koty) showed the same intra-individual variation at position 172. The only ITS2 sequence of U. zonata available on GenBank (origin Czech Republic, accession number Z47999) differed from the samples from the Baikal region by six bp (equivalent to less than 2% divergence).
These low levels of sequence divergences in the typically very variable ITS2 region must be regarded as intraspecific variation. The ITS region has been widely used in Chlorophyta and Ulvophyceae for delineation at the species level and similar or higher levels of sequence divergence have been regarded as representing intraspecific variation. While the ribotype of the ice population differed by two bp from the summer populations, the same ribotype was also found during summer (sample Q38, see ), and the ‘summer ribotype’ was also found in winter in a mountain stream (sample R20). Populations of U. zonata that persist under winter lake ice and give rise to large spring blooms have also been reported from Lake Huron, USA (Graham et al., Citation1985a). Our limited sampling does not reveal any pattern of population differentiation, but suggests low levels of intraspecific variation in U. zonata.
Discussion
First records of aquaporins for the class Ulvophyceae
It has been recently shown that green algae (Chlorophyta) contain proteins of the new subclasses of MIPs: MIP A, MIP B, MIP C, MIP D, MIP E and GIP, PIPs were present in a few cases and TIPs were absent among all investigated taxa (Anderberg et al., Citation2011). However, only members of the green algal classes Trebouxiophyceae, Mamiellophyceae and Chlorophyceae were investigated; Ulvophyceae were not included in that study as there is no genome available for this class at present. Ulothrix zonata is classified in the order Ulotrichales within the Ulvophyceae (Lewis & McCourt, Citation2004; Cocquyt et al., Citation2010; Leliaert et al., Citation2012).
It would be interesting to perform detailed population genetic studies to estimate possible changes in ribotype frequencies over time and geographic scales, also in relation to aquaporin occurrence and expression levels, and to look for genes under selection.
PIP-related genes have been found in the genome of some, but not all, Trebouxiophyceae (Anderberg et al., Citation2011). Thus, the presence of this MIP subfamily in the Chlorophyta has been established, but our detection of PIP1 in Ulothrix represents the first record in the Ulvophyceae. PIP-related genes appear to have been lost in some Trebouxiophyceae, the Chlorophyceae and the Mamiellophyceae (Anderberg et al., Citation2011). Losses of other MIP subfamilies during the evolution of embryophytes has also been documented: the GIP and HIP subfamilies are present in mosses but not embryophytes and the XIP subfamily has been lost in monocots (Danielson & Johanson, Citation2008). However, a puzzling aspect of our immunological finding is that a PIP1-antiserum has been used, which detects a conserved N-terminal epitope so far found only in sequences of higher plants – all known algal MIP sequences (Anderberg et al., Citation2011) would not be detected by this antiserum. Therefore, confirmation of the presence of higher plant-like PIP1s in Ulothrix or other members of the Ulvophyceae by molecular data would be desirable. Limited EST libraries are publicly available for the Ulvophyceae (GenBank taxid 33103 – c. 13 500 ESTs) and blasting conserved aquaporin motifs against these EST libraries yielded no significant hits (tblastn). Our immunological signals were clearly indicating at least an intermediate level of expression, but aquaporin genes might not have been expressed sufficiently or might not have been sequenced when the available EST libraries of other ulvophytes were generated. Thus, there are currently no suitable molecular data available to draw any conclusions regarding the presence or absence of PIP1-like aquaporins in the Ulvophyceae.
Impact of PIP1 aquaporins on the cold adaptation of U. zonata
Our observations suggest that the detected PIP1 antigens are constitutive, yet differentially expressed, cellular membrane proteins with high amounts correlated with low water temperature. Based on the molecular data, the ice- and summer populations appear to be conspecific and the observed differences in the expression of aquaporins represents adaptation to changing environmental conditions. Variations of the PIP1 content in U. zonata were similar to those during adaptation of mesophytes to low temperatures: elevated PIP1 aquaporin levels were found in the leaves of wheat in winter and decreased during spring (Yakovlev & Borovskii, Citation2003). However, the accumulation of PIP1 in spring might also be influenced by the prolific vegetative reproduction of the algae, when plasmalemma aquaporins were synthesized in actively dividing cells. Our current knowledge allows us to suggest that aquaporin subfamilies were lost in some chlorophyte lineages but the higher plant-specific aquaporin PIP1 subfamily was conserved in the course of Ulvophyceae evolution.
The relationships among green algal lineages remain largely unresolved, mainly because multi-gene data are only available for a limited number of taxa. Similarly, phylogenetic relationships among and within the main clades of the core chlorophytes (Ulvophyceae, Trebouxiophyceae and Chlorophyceae) have not been fully resolved (Leliaert et al., Citation2012). Future work should include sequencing the transcriptome of U. zonata and/or sequencing of the amino acid sequence of the isolated aquaporins to assess homology with streptophyte aquaporins. Thus, the ice population of U. zonata inhabiting Lake Baikal is a unique subject for studying physiological and biochemical adaptation mechanisms of fresh-water extremophiles.
Acknowledgements
The authors thank Dr I.V. Khanaev, E.A. Volkova and V.S. Vishnyakov for assistance in sample collection. Professor M. Yuma (Centre for Ecological Research at Kyoto University, Japan) kindly provided loggers for measuring water temperature. E.M. Timoshkina is acknowledged for her assistance in preparing the manuscript and translating it to English.
Disclosure statement
No potential conflict of interest was reported by the author(s).
Additional information
Funding
Notes on contributors
Aleksey Permyakov
A.Permyakov: preparation of protein extracts, SDS-PAGE and western blot analysis, drafting and editing manuscript; S. Osipova: preparation of protein extracts, drafting and editing manuscript; N. Bondarenko: original concept; microscopy, cell size measurements, drafting and editing manuscript; L. Obolkina: samples of plant materials, water temperature monitoring; O. Timoshkin: photographic, water temperature monitoring; C. Boedeker: DNA extraction and sequencing, analysis of molecular data, drafting and editing manuscript; B. Geist: preparation of antibodies; A. Schäffner: consultation process, editing manuscript.
References
- Agre, P. & Kozono, D. (2003). Aquaporin water channels: molecular mechanisms for human diseases. FEBS Letters, 555: 72–78.
- Anderberg, H.I., Danielson, J.A.H. & Johanson, U. (2011). Algal MIPs, high diversity and conserved motifs. BMC Evolutionary Biology, 11: 110–124.
- Boedeker, C., O’Kelly, C.J., Star, W. & Leliaert, F. (2012). Molecular phylogeny and taxonomy of the Aegagropila clade (Cladophorales, Ulvophyceae), including the description of Aegagropilopsis gen. nov. and Pseudocladophora gen. nov. Journal of Phycology, 48: 808–825.
- Bradford, M.M. (1976). A rapid and sensitive method for the quantitation of microgram quantities of protein utilizing the principle of protein-dye binding. Analytical Biochemistry, 72: 248–254.
- Cocquyt, E., Verbruggen, H., Leliaert, F. & De Clerck, O. (2010). Evolution and cytological diversification of the green seaweeds (Ulvophyceae). Molecular Biology and Evolution, 27: 2052–2061.
- Daniels, M.J., Chaumont, F., Mirkov, T.E. & Chrispeels, M.J. (1996). Characterization of a new vacuolar membrane aquaporin sensitive to mercury at a unique site. Plant Cell, 8: 587–599.
- Danielson, J.Å.H. & Johanson, U. (2008). Unexpected complexity of the Aquaporin gene family in the moss Physcomitrella patens. BMC Plant Biology, 8: 45. doi:10.1186/1471-2229-8-45.
- De Senerpont Domis, L.N., Fama, P., Bartlett, A.J., Van Reine, W.F.P., Espinosa, C.A. & Trono, G.C. (2003). Defining taxon boundaries in members of the morphologically and genetically plastic genus Caulerpa (Caulerpales, Chlorophyta). Journal of Phycology, 39: 1019–1037.
- Goff, L. & Moon, D. (1993). PCR amplification of nuclear and plastid genes from algal herbarium specimens and algal spores. Journal of Phycology, 29: 381–384.
- Graham, M.J., Graham, L.E. & Kranzfelder, J.A. (1985a). Light, temperature and photoperiod as factors controlling reproduction in Ulothrix zonata (Ulvophyceae). Journal of Phycology, 21:235–239.
- Graham, M. J., Kranzfelder, J. A. & Auer, M. T. (1985b). Light and temperature as factors regulating seasonal growth and distribution of Ulothrix zonata (Ulvophyceae). Journal of Phycology, 21: 228–234.
- Henzler, T., Waterhouse, R.N., Smyth, A.J., Carvajal, M., Cooke, D.T., Schäffner, A.R., Steudle, E. & Clarkson, D.T. (1999). Diurnal variations in hydraulic conductivity and root pressure can be correlated with the expression of putative aquaporins in the roots of Lotus japonicas. Planta, 210: 50–60.
- Herman, E.M., Rotter, K., Premakumar, R., Elwinger, G., Bae, R., Ehler-King, L., Chen, S.X. & Livingston, D.P. (2006). Additional freeze hardiness in wheat acquired by exposure to –3°C is associated with extensive physiological, morphological, and molecular changes. Journal of Experimental Botany, 57: 3601–3618.
- Izhboldina, L. (1990). Meio- and macrophytobenthos of Lake Baikal (algae). Irkutsk State University Publ., Irkutsk. [In Russian]
- Jang, J.Y., Kim, D.G., Kim, Y.O., Jin Sun Kim, J.S. & Kang, H. (2004). An expression analysis of a gene family encoding plasma membrane aquaporins in response to abiotic stresses in Arabidopsis thaliana. Plant Molecular Biology, 54: 713–725.
- Javot, H., Lauvergeat, V., Martin, F., Santoni, V., Güclü, J., Vinh, J., Heyes, J., Franck, K.I., Schäffner, A.R., Bouchez, D. & Maurel, C. (2003). Role of a single aquaporin isoform in root water uptake. Plant Cell, 15: 509–522.
- Kaldenhoff, R., Ribas-Carbo, M., Sans, J.F., Lovisolo, C., Heckwolf, M. & Uehlein, N. (2008). Aquaporins and plant water balance. Plant Cell and Environment, 31: 658–666.
- Kammerloher, W., Fischer, U., Piechottka, G. P. & Schäffner, A. R. (1994). Water channels in the plant plasma membrane cloned by immunoselection from a mammalian expression system. Plant Journal, 6: 187–199.
- Katsuhara, M., Hanba, Y., Shiratake, K. & Maeshima, M. (2008). Expanding roles of plant aquaporins in plasma membranes and cell organelles. Functional Plant Biology, 35: 1–14.
- Laemmli, U. (1970). Cleavage of structural proteins during the assembly of the bacteriophage T4. Nature, 277: 680–685.
- Lee, S.H., Chung, G.C., Jang, J.Y., Ahn, S.J. & Zwiazek, J.J. (2012). Overexpression of PIP2;5 aquaporin alleviates effects of low root temperature on cell hydraulic conductivity and growth in Arabidopsis. Plant Physiology, 159: 479–488.
- Leliaert, F. (2004). Taxonomic and phylogenetic studies in the Cladophorophyceae (Chlorophyta). Ph.D. Dissertation, Ghent University, Belgium. 294 pp. Available at: http://www.lib.ugent.be/ (last accessed 24 Oct 2012).
- Leliaert, F., Verbruggen, H., Wysor, B. & De Clerck, O. (2009). DNA taxonomy in morphologically plastic taxa: algorithmic species delimitation in the Boodlea complex (Chlorophyta: Cladophorales). Molecular Phylogenetics and Evolution, 53: 122–133.
- Leliaert, F., Smith, D.R., Moreau, H., Herron, M.D., Verbruggen, H., Delwiche, C.F. & De Clerck, O. (2012). Phylogeny and molecular evolution of the green algae. Critical Reviews in Plant Sciences, 31: 1–46.
- Lewis, L.A. & McCourt, R.M. (2004). Green algae and the origin of land plants. American Journal of Botany, 91: 1535–1556.
- Lokhorst, G.M. & Vroman, M. (1974). Taxonomic studies on the genus Ulothrix (Ulothrichales, Chlorophyceae) III. Acta Botanica Neerlandica, 23: 561–602.
- Malta, E.-J., Draisma, S.G.A. & Kamermans, P. (1999). Free-floating Ulva in the southwest Netherlands: species or morphotypes? A morphological, molecular and ecological comparison. European Journal of Phycology, 34: 443–454.
- Martinez-Ballesta, M., Bastías, E., Zhu, C., Schäffner, A.R., González-Moro, B., González-Murua, C. & Carvajal, M. (2008). Boric acid and salinity effects on maize roots. Response of aquaporins ZmPIP1 and ZmPIP2, and plasma membrane H+-ATPase, in relation to water and nutrient uptake. Physiologia Plantarum, 132: 479–490.
- Maurel, C., Verdoucq, L., Luu, D.T. & Santoni, V. (2008). Plant aquaporins: membrane channels with multiple integrated functions. Annual Review of Plant Biology, 59: 595–624.
- McCourt, R.M. & Hoshaw, R.W. (1990). Noncorrespondence of breeding groups, morphology, and monophyletic groups in Spirogyra (Zygnemataceae: Chlorophyta) and the application of species concepts. Systematic Botany, 15: 69–78.
- Moshelion, M., Hachez, C., Ye, Q., Cavez, D., Bajji, M., Jung, R. & Chaumont, F. (2009). Membrane water permeability and aquaporin expression increase during growth of maize suspension cultured cells. Plant Cell and Environment, 32: 1334–1345.
- Ohba, H., Nashima, H. & Enomoto, S. (1992). Culture studies on Caulerpa (Caulerpales, Chlorophyceae) III. Reproduction, development and morphological variation of laboratory-cultured C. racemosa var. peltata. Botanical Magazine. Tokyo, 105: 589–600.
- Osipova, S., Dudareva, L., Bondarenko, N., Nasarova, A., Sokolova, N., Obolkina, L. & Timoshkin, O. (2009). Temporal variation in fatty acid composition of Ulothrix zonata (Chlorophyta) from ice benthic communities of Lake Baikal. Phycologia, 48: 130–135.
- Postaire, O., Tournaire-Roux, C., Grondin, A., Boursiac, Y., Morillon, R., Schäffner, A.R. & Maurel, C. (2010). A PIP1 aquaporin contributes to hydrostatic pressure-induced water transport in both the root and rosette of Arabidopsis. Plant Physiology, 152: 1418–1430.
- Rambaut, A. (2007). Se-Al (multiple sequence alignment program), v2.0a11. Available at: http://tree.bio.ed.ac.uk/software/seal (last accessed 8 May 2012).
- Rousseau, F., Leclerc, M.C. & de Reviers, B. (1997). Molecular phylogeny of European Fucales (Phaeophyceae) based on partial large-subunit rDNA sequence comparisons. Phycologia, 36: 438–446.
- Shyam, R. & Saxena, P.N. (1980). Morphological and cytological investigations of Ulothrix zonata and taxonomy of related species. Plant Systematics and Evolution, 135: 151–158.
- Timmons, T. & Dunbar, B. (1990). Protein blotting and immunodetection. Methods Enzymology, 182: 679–688.
- van den Hoek, C. (1963). Revision of the European species of Cladophora. 248 pp., Brill E.J., Leiden.
- White, T.J., Bruns, T., Lee, S. & Taylor, J. (1990). Amplification and direct sequencing of fungal ribosomal genes for phylogenetics PCR protocols. In A Guide to Molecular Methods and Applications (Innis, M.A., Gelfand, D.H., Sninsky, J.J. & White, T.J., editors), 315–322. Academic Press, New York.
- Yakovlev, A. & Borovskii, G. (2003). Low temperature adaptation/disadaptation effect on the aquaporins content in the winter wheat membranes. European Journal of Biochemistry. Abstract no.: P 4.9:19.