Abstract
Surface seawater was collected in four different seasons in the coastal East China Sea adjacent to the Yangtze River Estuary and phytoplankton community diversity was analysed using rbcL genetic markers. Phytoplankton diversity (Shannon Index) was found to be highest in autumn and lowest in summer, which was mainly controlled by seawater temperature, river runoff, the Taiwan Warm Current and possibly other environmental factors. For taxa characterized by Form IAB rbcL, the abundance of Chlorophyta was much greater than those of Proteobacteria and Cyanobacteria throughout the year with the most dominant taxa being Bathycoccus prasinos (Chlorophyta) in spring and Micromonas sp. (Chlorophyta) in other seasons. For taxa identified by Form ID rbcL, Coscinodiscophyceae (diatoms) constituted the largest group (most clones) in the phylogenetic tree. Dinophysis fortii (a dinoflagellate) was found to be the most abundant species in winter and spring and Skeletonema spp. (a diatom) dominated the phytoplankton community in summer and autumn. The seasonal dominance of Dinophysis fortii agreed well with the recently increasing proportion of dinoflagellates in the phytoplankton community in the coastal East China Sea. The abundance of Dinophysis fortii was negatively correlated with seawater temperature, suggesting that harmful algal blooms caused by this species may primarily occur in spring.
INTRODUCTION
The East China Sea (ECS) is one of the largest marginal seas in the world, and currently acts as a net sink of atmospheric CO2, exporting a significant amount of organic carbon to the North Pacific (Chen et al., Citation2004). The ECS shelf is strongly influenced by the terrigenous input of the Yangtze River and the Kuroshio Current (KC) and Taiwan Warm Current (TWC) from the south (Zhou et al., Citation2008). Atmospheric deposition may also be substantial during Asian dust events and is associated with heavily anthropogenic emissions across East Asia (Zhu et al., Citation2013; Guo et al., Citation2014). Yangtze River Diluted Water (YDW) is a major source of nitrate and silicate to the coastal ECS, flowing southeastward along the coastline in winter, with a high nutrient area confined to the west of 123°E. In summer, it spreads eastward into the ECS via a thin surface layer as the Yangtze River runoff peaks, and the intrusion of enhanced TWC becomes an important supplemental source of phosphate (Fan & Song, Citation2014). The analysis of 51 surveys between 1981 and 2004 showed a ‘bimodal‘ seasonal distribution of phytoplankton biomass in the coastal ECS with the maximum in August and a sub-peak in May, which was mainly controlled by the seasonal change of nutrient supply of different water masses (Yang et al., Citation2014). Temperature, hydrodynamic conditions, predator–prey relationships and atmospheric deposition could also be important factors influencing the phytoplankton biomass (Gong et al., Citation2003; Chen et al., Citation2004; Tian & An, Citation2012).
The diversity of natural phytoplankton population in the coastal ECS has been studied previously by microscopy (Guo et al., Citation2011; Weng et al., Citation2011; Yang et al., Citation2014), photosynthetic pigments (Furuya et al., Citation2003), flow cytometry (Jiao et al., Citation2005) and polymerase chain reaction (PCR)-based denaturing gradient gel electrophoresis (DGGE) of 18S rRNA (Sun et al., Citation2010). Field surveys using microscopy between 1990 and 2009 discovered a total of 67 dominant taxa of phytoplankton including 53 diatoms, 13 dinoflagellates and two morphotypes of Cyanobacteria in this area. Diatoms were found to be the most important group in the phytoplankton community, in which Skeletonema (mainly S. costatum) was abundant throughout the year and Coscinodiscus and Chaetoceros were dominant taxa in cold (winter and spring) and warm (summer and autumn) seasons, respectively (Yang et al., Citation2014). The abundances of other taxa also varied with the season, e.g. Prorocentrum donghaiense Lu was the major taxon only in spring (Tian et al., Citation2010). The seasonal succession of Coscinodiscus and Chaetoceros was suggested to be correlated with the water stability and associated change of nutrient levels (Yang et al., Citation2014). The proportion of dinoflagellates in the phytoplankton showed a significant increase from less than 5% in the 1980s to approximately 20% in the 2010s. Rapid propagation of some planktonic taxa may cause harmful algal blooms (HABs). In the coastal ECS, HABs occurred over 50 times every year between 2000 and 2009 with the dominant taxa being mostly Skeletonema costatum, Prorocentrum and Karenia mikimotoi (Gao & Shao, Citation2011). Along with HABs, lipophilic toxins can be produced by some planktonic dinoflagellates (Yasumoto et al., Citation1980). Dinophysis and benthic Prorocentrum taxa, the organisms associated with diarrhetic shellfish poisoning (DSP) toxicity outbreaks, were widely distributed throughout Chinese coastal waters (Chen & Ni, Citation1988; Lu & Hodgkiss, Citation2004; Wang et al., Citation2007). Specifically, Dinophysis species appeared and occasionally bloomed with other taxa at Nanji Islands, in the marginal ocean area of the ECS (Wang et al., Citation2007). The detection of dominant taxa that could cause HABs increased significantly after 2007 (Gao & Shao, Citation2011), which was partly due to the application of new techniques. A big advantage of the molecular approach is to identify the phytoplankton taxa which may be overlooked by microscopy, such as many picoplankton. Microscopic identification requires extensive taxonomic experience. Nonetheless, molecular approaches have rarely been used in the study of phytoplankton community structure and diversity in the coastal ECS.
Small subunit rRNA is by far the most popular genetic marker for phylogenetic analysis, because it is very abundant in individual cells and can be used to discriminate organisms at different taxonomic levels from the phylum to the species (Vaulot et al., Citation2008). However, the rbcL gene encoding the large subunit of ribulose-1, 5-bisphosphate carboxylase/oxygenase (RuBisCO) is more effective than rRNA genes for discrimination of phytoplankton, since plastid genes targeting most of the photosynthetic plankton exclude heterotrophic taxa. The RuBisCO can be divided into three forms based on structures of the peptide chain (Andersson & Backlund, Citation2008). Form II exists in some Proteobacteria and symbiotic dinoflagellates. Form III covers Archaea. Form I is found in plants, phytoplankton, some chemoautotrophic and photosynthetic bacteria, and can be divided into four groups based on amino acid sequence homologies. Form IA is found mainly in alpha-, beta- and gamma-Proteobacteria. Form IB is found in Chlorophyta, higher plants and Cyanobacteria with two exceptions, Prochlorococcus marinus and Synechococcus sp. Form IAB is designated according to the two conserved regions of RuBisCO amino acid sequences of Form IA and Form IB. Forms IC and ID can include some chemoautotrophic bacteria and Haptophyceae and Stramenopiles. Overall, Forms IAB and ID are represented in almost all eukaryotic algae and Cyanobacteria (Tabita, Citation1999; Tabita et al., Citation2007). The rbcL gene seems to be more appropriate for phylogenetic analysis of closely related phytoplankton than 18S rRNA because it is less conservative and evolves faster (Tabita et al., Citation2008; Xia et al., Citation2010). Paul et al. (Citation2000) designed two primer pairs and successfully identified the phytoplankton community structure in the southeastern Gulf of Mexico. Using the two primers, Ghosh & Love (Citation2011) analysed the genetic diversity of algae in three wastewater treatment plants.
Phytoplankton may be classified differently using different phylogenetic methods. Medlin & Kaczmarska (Citation2004) classified diatoms into three major classes, Coscinodiscophyceae, Mediophyceae and the Bacillariophyceae, based on nuclear-encoded small subunit ribosomal RNA (SSU rRNA) analyses (the CMB hypothesis). Thalassiosirales (which includes Thalassiosira and Skeletonema) is classified into Mediophyceae in their dataset. Nonetheless, Theriot et al. (Citation2009) indicated that classification of the evolutionary relationship of the diatoms according to the CMB hypothesis is premature. The CMB hypothesis has been rejected by their analyses of SSU data they collected as well as by an rbcL dataset.
In the present study, Thalassiosira is considered as a member of the Coscinodiscophyceae based on analyses of the rbcL gene and in relation to the results of Prasad et al. (Citation2002) and Wojtal & Kwandrans (Citation2006). The main objective of this study is to determine the dominant taxa of phytoplankton and their seasonal succession in the coastal East China Sea and detect the taxa responsible for HABs adjacent to the Yangtze River Estuary, using molecular methods with rbcL primers designed for RuBisCO Forms ID and IAB.
MATERIALS AND METHODS
Sampling
Surface seawater was collected near Huaniao Island (30.85°N, 122.67°E) in spring (17 April 2012 and 24 March–18 April 2013), summer (31 July 2012 and 18 July–8 August 2013), autumn (6 October 2012 and 23 October–12 November 2013) and winter (29 December 2012 and 1–12 January 2013) periods (). Two or three samples were collected between 10:00 and 14:00 on each sampling date (total 111 discrete samples). One litre of seawater was pre-filtered through 50 μm nylon mesh to remove grazers and then filtered through a 0.22 μm Millipore filter (Synthetic fabric, Shanghai Mosh science equipment Co. Ltd) for collection of phytoplankton. The filter was transferred into a plastic Petri dish (Polyethylene, Experimental Equipment Co., Ltd., Haimen Learning Alliance) sealed with Parafilm (Shanghai Yes Service Biotech, Inc.) and stored at −20°C prior to nucleic acid extraction.
Fig. 1. The phytoplankton community structure in surface seawater sampled at Huaniao Island in the coastal East China Sea in spring (17 April 2012 and 24 March–18 April 2013), summer (31 July 2012 and 18 July–8 August 2013), autumn (6 October 2012 and 23 October–12 November 2013) and winter (29 December 2012 and 1–12 January 2013) periods. ‘Others’ include Zetaproteobacteria, Euglenida, Klebsormidiophyceae, Trebouxiophyceae and Ulvophyceae (Form IAB), and Dictyochophyceae, Fragilariophyceae, Pelagophyceae, Eustigmatophyceae, Chrysophyceae and Raphidophyceae (Form ID).
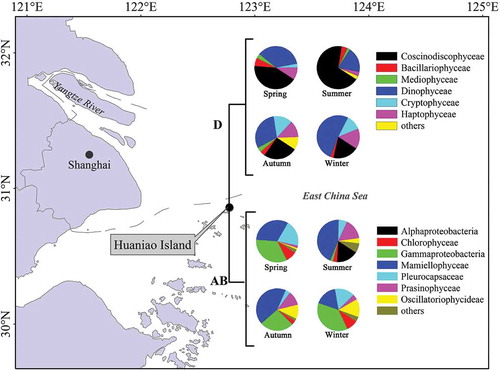
Meteorological data including cloud cover (incident light) and precipitation were obtained from Real-time Data Display from ENVF (Institute for the Environment) Atmospheric & Environmental Database (http://envf.ust.hk/dataview/metplot/current/) for each sampling date. The average salinity and seawater temperature were derived from field observation (YSI EC300 Multi-parameter Water Quality Sonde, Yellow Spring, OH) except for the data for 17 April, 3 July and 6 October 2012, which were obtained from the Ecom-si model (Wu et al., Citation2011) due to instrumental failure.
DNA extraction
DNA extraction followed a protocol with modification (Varela-Alvarez et al., Citation2006). Each filter was cut into pieces and placed into a 2 ml tube. Approximately 0.8 ml of CTAB buffer (2% CTAB, 2% polyvinyl pyrrolidone, 1.4 M NaCl, 20 mM EDTA (pH 8.0) and 100 mM Tris HCl (pH 8.0)) and 0.5 g of glass beads were added to the tube. The mixture was shaken for 5 min and then bathed in a 65°C water bath for about 30 min. The lysate was extracted with 0.8 ml chloroform/isoamyl alcohol (24/1, V/V) and then centrifuged at 12 000 rpm for 15 min. About 500 μl of supernatant was transferred into a new tube, mixed with 0.8 ml phenol/chloroform/isoamyl alcohol (12/12/1, V/V/V) and centrifuged at 12 000 rpm for 15 min. The supernatant was extracted using 0.8 ml chloroform/isoamyl alcohol (24/1, V/V) and centrifuged again. The supernatant was transferred into 0.6 ml of cold isopropyl alcohol and stored at −20°C overnight. Centrifugation was repeated the next day, and the white precipitate was washed with cold 70% ethanol twice, dried at room temperature and finally dissolved in 20–50 μl of double distilled water (ddH2O) for PCR.
Cloning and sequencing
Two sets of rbcL primers targeting RuBisCO Form IAB and Form ID (Majorbio Co., Ltd.; Paul et al., Citation2000) were used to amplify 554 bp and 615 bp fragments of the rbcL gene to capture mainly Cyanobacteria, Chlorophyta and Proteobacteria (Form IAB), and Bacillariophyta, Pyrrophyta, Cryptophyta and Haptophyta (Form ID). PCR amplifications used the Ex Taq™ Version 2.0 PCR kit (Takara, Japan). A total of 50 μl of PCR mixture contained 20 μmol each primer, 10 ng purified template DNA, 25 μl Ex Taq mix and 10 μg of bovine serum albumin. The PCR was performed in a thermal cycler (PCR Thermal Cycler Dice, Takara, Japan) with an initial denaturation at 94°C for 3 min, followed by 40 cycles at 95°C for 1 min, 52°C for 1 min and 72°C for 1.5 min, and the final extension step at 72°C for 15 min. The PCR products were purified using a gel electrophoresis and gel extraction kit (Axygen Inc.), and the replicates derived from the same-day seawater samples were mixed and cloned into the Takara vector (pMD18-T). Plasmids with inserts were transformed into competent cells (DH5α). In total 1232 clones were analysed for nucleotide sequences with 123–180 clones analysed for each season.
Data analysis
pMD18-T plasmid sequences were removed from rDNA sequences. Sequences were aligned with ClustalW (Thompson et al., Citation1997) using Bioedit-7.0 software (Hall, Citation1999). The nucleotide sequences most closely related to rbcL clones were obtained using the NCBI BLAST algorithm (http://blast.ncbi.nlm.nih.gov/Blast.cgi?PROGRAM=blastn&PAGE_TYPE=BlastSearch&LINK_LOC=blasthome) with the database of ‘Nucleotide collection (nr/nt)’ and excluding ‘Models’. Sequences for which the closest match was less than 93% were defined as ‘uncultured microorganism’ (Samanta et al., Citation2014). PHYLIP 3.69 software and the Dnadist program (Felsenstein, Citation1989) were chosen for calculation of the distance matrix as the input file of DOTUR-1.53 (Schloss & Handelsman, Citation2005). DOTUR assigns sequences to Operational Taxonomic Units (OTUs) and constructs rarefaction and collector’s curves, Shannon’s diversity index and Chao1, ACE, Jackknife and Bootstrap richness estimators. The Shannon index was calculated based on the distance matrix at a cutoff of 96% (Ghosh & Love, Citation2011).
Si is the number of clones of i OTU, and N is the total number of clones. Phylogenetic trees were built using the neighbour-joining (NJ) method in MEGA 5.0 software (Tamura et al., Citation2007) and maximum parsimony and maximum likelihood analyses were also performed. NJ trees were chosen based on the level of resolution. rbcL clone sequences obtained in this study can be accessed from GenBank using the following accession numbers: KF68362–KF683903; KJ153016–KJ154027. The influences of environmental factors on phytoplankton community structure were analysed by Canonical Correspondence Analysis (CCA). The percentages of OTUs (clones per season) along with corresponding environmental factors were used for CCA.
RESULTS
The diversity of phytoplankton
A total of 587 Form IAB and 645 Form ID rbcL clones were obtained from the seawater samples collected in four different seasons, from which 145 unique clones (OTUs) of Form IAB and 174 unique clones (OTUs) of Form ID were identified based on nucleotide sequence analyses (). The proportions of “uncultured microorganism” ranged between 2% and 7% in different seasons for OTUs identified from both Form IAB and Form ID. The library coverage of Form IAB rbcL clones (> 71%, ) was observed for all samples suggesting that the method could effectively capture Cyanobacteria, Chlorophyta and Proteobacteria. Form ID rbcL clones showed relatively low coverage for autumn samples compared with other seasons but also captured the most targeted phytoplankton taxa. Along with the relatively low library coverage, Shannon index values of autumn samples were the highest, suggesting that the water in autumn may contain the highest diversity of phytoplankton which were thereby difficult to capture completely by the rbcL primers (particularly Form ID rbcL). The same result with the highest phytoplankton diversity in autumn was also observed by Luo et al. (Citation2007) in the ECS using microscopy. The lowest Shannon index values were found in summer. Coscinodiscophyceae (diatoms) and Mamiellophyceae (Chlorophyta) were the most dominant classes in summer with Skeletonema (diatom) and Micromonas (Chlorophyta) accounting for 24% and 44% of all OTUs.
Table 1. Total number of clones, library coverage, number of OTUs, clones of dominant OTUs, diversity index and classification of dominant OTUs derived from Form 1AB and Form 1D rbcL for surface seawater sampled in the coastal East China Sea in spring (17 April 2012 and 24 March–18 April 2013), summer (31 July 2012 and 18 July–8 August 2013), autumn (6 October 2012 and 23 October–12 November 2013) and winter (29 December 2012 and 1–12 January 2013) periods.
The Shannon index is made up of richness and evenness components. Richness can also be measured by the Chao1 index. For taxa identified by Form ID, Chao1 of spring samples was the lowest, but the Shannon index ranked the second highest (), suggesting that the phytoplankton community was highly even and the proportion of OTUs was uniform in spring. In contrast, winter samples showed a low Shannon index and the second highest Chao1 suggesting that the phytoplankton community may be dominated by only a few taxa. For taxa discriminated by Form IAB, spring samples showed the highest Chao1 and a low Shannon index. The highest proportions of Chroococcidiopsis sp. CCMP2733 (97% nucleotide similarity, AEE92832) and Bathycoccus prasinos (98% nucleotide similarity, AAA87204) were observed in spring, accounting for 17% and 18% of total clones, respectively. In winter, 49% of clones were Dinophysis fortii (99% nucleotide similarity, BAD42428), the highest among the four seasons.
The most abundant OTUs discriminated by Form IAB rbcL belonged to Chlorophyceae in all four sampling seasons, of which Micromonas sp. RCC299 (99% nucleotide similarity, YP_002808616) was the most dominant OTU in summer, autumn and winter and Bathycoccus prasinos dominated the spring samples (). At the class level, Mamiellophyceae (Chlorophyta) and Gammaproteobacteria (Proteobacteria) were the most abundant during the warm (summer and autumn) and cold (spring and winter) seasons, respectively (). Mamiellophyceae has been found to be widely distributed in the ECS (Liu et al., Citation2011). Overall, Chlorophyta were more abundant than Proteobacteria and Cyanobacteria identified by Form IAB throughout the year in the coastal ECS.
For phytoplankton taxa discriminated by Form ID rbcL, the succession of dominant OTUs was observed as Skeletonema costatum (96% nucleotide similarity with AEN19678) in summer, Skeletonema subsalsum (99% nucleotide similarity with ABF60378) in autumn to Dinophysis fortii (class Dinophyceae) in winter and spring (). Indeed, the total clones of Dinophyceae were found to be the highest among the classes in winter, and summer was dominated by class Coscinodiscophyceae ().
Phytoplankton community structure
lists the top three dominant OTUs discriminated by rbcL Form IAB and Form ID for the seawater sampled during four different seasons. The similarity of nucleotide sequence between the clones and inferred organism was greater than 96% for most taxa such as Micromonas sp. RCC299, Dinophysis fortii and Skeletonema costatum. The dominant OTUs were distinct in different seasons probably due to the change in environmental factors such as temperature, light intensity and salinity.
Table 2. Closest relatives to clones amplified by Form 1AB and Form 1D rbcL for surface seawater sampled in the coastal East China Sea in spring (17 April 2012 and 24 March–18 April 2013), summer (31 July 2012 and 18 July–8 August 2013), autumn (6 October 2012 and 23 October–12 November 2013) and winter (29 December 2012 and 1–12 January 2013) periods. Relative OTUs (top three dominant OTUs) were obtained using the NCBI BLAST algorithm.
For taxa identified by Form IAB rbcL (), Micromonas sp. RCC299 (Chlorophyta) was the most dominant taxon throughout the year. Phylogenetic trees showed that 8% of spring samples, 44% of summer samples, 42% of autumn samples and 10% of winter samples cluster with Micromonas sp. RCC299 (, ). 18% of spring samples and 1% of autumn samples were related to Bathycoccus prasinos. These two chlorophyte taxa are widely distributed in temperate waters (Massana et al., Citation2004; Romari & Vaulot, Citation2004). The cyanobacterium Chroococcidiopsis sp. was widely represented in the tree, accounting for 14%, 7%, 3% and 7% of total clones in spring, summer, autumn and winter, respectively (, ).
Fig. 2. Phylogenetic tree obtained from a Neighbour joining analysis for the most dominant OTUs (number of clones in parentheses) discriminated by Form IAB rbcL for surface seawater sampled in the coastal East China Sea in four seasons. Sequences existing in GenBank are included with accession numbers in parentheses. The numbers at the nodes represent full heuristic bootstrap values (with 1000 replicates) greater than 50%. The scale bar represents amino acid substitutions/site.
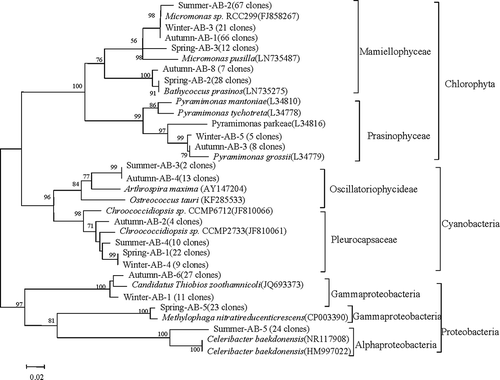
Phytoplankton structure discriminated by Form ID rbcL also varied with the season. Spring samples were dominated by Coscinodiscophyceae, particularly Skeletonema subsalsum (24 clones, 99% nucleotide similarity to ABF60378) and Skeletonema costatum (24 clones, ); Skeletonema was dominant in spring, summer and autumn. Summer samples were mainly composed of Coscinodiscophyceae related to Skeletonema costatum (43 clones) and Thalassiosira minima (31 clones, ). Coscinodiscophyceae therefore was the largest class corresponding to the most abundant clones in the phylogenetic tree (). Previous studies using microscopy have also found that diatoms are the most important group with abundant Skeletonema costatum throughout the year in the studied area. In terms of the most abundant taxa, Dinophysis fortii (Dinophyceae) was identified in spring, autumn and winter (, ). Although some Dinophyceae possess Form II RuBisCO, use of ID rbcL primers can yield clones showing high similarity to some published dinophyte rbcL sequences, as indicated previously (e.g. Bhadury & Ward, Citation2009).
Fig. 3. Phylogenetic tree obtained from a Neighbour joining analysis of the most dominant OTUs (number of clones in parentheses) discriminated by Form 1D rbcL for surface seawater sampled in the coastal East China Sea in four seasons. Sequences in GenBank are included with accession numbers in parentheses. The numbers around the branches represent full heuristic bootstrap values (with 1000 replicates) greater than 50%. The scale bar represents substitutions/site.
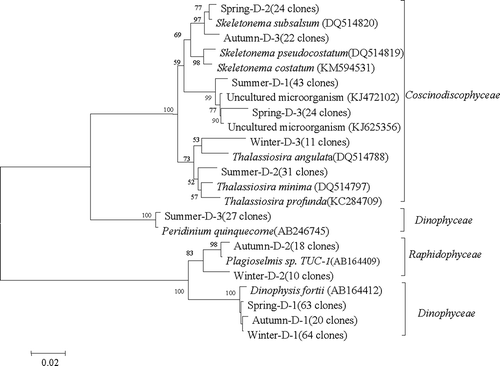
Effects of environmental factors
The community structure of marine phytoplankton is affected by many environmental factors such as water temperature, salinity, light and nutrient concentrations (Table S2). The temperature of sampling sites varied strongly with the season with average values of 13, 26, 21 and 8.8°C in spring, summer, autumn and winter, respectively. Salinity of surface water is negatively correlated with the Yangtze River runoff which is approximately one order of magnitude larger in summer than in winter (Fan & Song, Citation2014). In summer, the YDW is strong enough to flow eastward although its influence is mainly a thin layer on the surface. Also in summer the enhanced TWC may increase the subsurface salinity in the study area. Therefore, water salinity in summer had the lowest average value (24.1) but largest fluctuation (16.9–30.3). Cloud cover affects the light intensity arriving at the sea surface, and it was lowest in summer (3.0) and highest in winter (5.7). Although nutrients are mostly supplied by different water masses, precipitation can be another important source of nutrients for surface seawater in certain times. Precipitation occurred mainly in winter in the study area. Four environmental factors, seawater temperature, salinity, cloud cover and precipitation, were used along with the percentages of OTUs for CCA. Nutrient concentrations were not measured along with the phytoplankton diversity during sampling periods and thus not included in the CCA analysis.
The four environmental factors were firstly tested for covariation. Cloud cover was found to be positively correlated with precipitation (r = 0.50, P < 0.01), and both factors showed significantly negative relationships with seawater temperature (r = –0.49 and −0.61, P < 0.01). No significant relationships were found between salinity and other three factors. Therefore, cloud cover, seawater temperature and precipitation were considered as one independent variable and the CCA results below mainly focused on the influence of seawater temperature and salinity. Seawater temperature and salinity showed correlations with Axis1 with coefficients of 0.9229 and −0.3851, respectively. Winter and spring samples were found to be negatively correlated with seawater temperature (). Conversely, positive relationships were observed between temperature and the phytoplankton taxa detected in summer and autumn. The influence of temperature on winter and summer samples was stronger than that on spring and autumn, respectively.
Fig. 4. The relationships between environmental factors and seawater samples collected in the coastal East China Sea in spring (samples 1–10, ▲), summer (samples 11–20, ◯), autumn (samples 21–30, □) and winter (samples 31–39, ²) analysed by Canonical Correspondence Analysis.
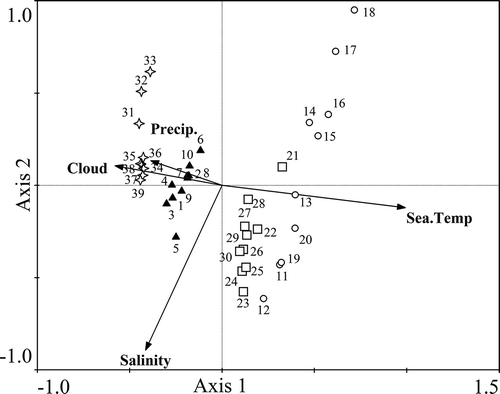
The relationships between dominant OTUs and environmental factors are shown in . Tetraselmis suecica (Chlorophyta) and Peridinium quinquecorne (dinoflagellate) both showed positive relationships with seawater temperature suggesting that high temperature could favour their growth (), and the two taxa were identified to be the dominant taxa only in summer (). Thalassiosira minima (diatom) showed a positive relationship with seawater temperature but a strongly negative relationship with salinity (). The abundance of T. minima may be enhanced as Yangtze River runoff increased and average salinity declined in summer. Proteobacteria Methylophaga nitratireducenticrescens and Candidatus Thiobios zoothamnicoli, Chlorophyte Bathycoccus prasinos and diatoms Thalassiosira profunda and Skeletonema subsalsum were all negatively correlated with seawater temperature. Although Dinophysis fortii (dinoflagellate) showed a negative relationship with seawater temperature, none of the four environmental factors significantly related to its abundance; it was found to be dominant in winter and spring ().
Fig. 5. Canonical correspondence analysis showing the relationships between environmental factors and dominant phytoplankton species including: Chroococcidiopsis sp. belonging to Cyanobacteria (●); Bathycoccus prasinos (B. prasinos), Micromonas sp. and Tetraselmis suecica (T. suecica) belonging to Chlorophyta (▼); Skeletonema costatum (S. costatum), Skeletonema subsalsum (S. subsalsum), Thalassiosira minima (T. minima), Pseudo-nitzschia multistriata (P. multistriata), Pseudo-nitzschia americana (P. americana) and Thalassiosira profunda (T. profunda) belonging to diatoms (△); Peridinium quinquecorne (P. quinquecorne) and Dinophysis fortii (D. fortii) belonging to dinoflagellates (■); Plagioselmis sp., Candidatus Thiobios zoothamnicoli (C. T. zoothamnicoli) and Methylophaga nitratireducenticrescens (M. nitratireducenticrescens) belonging to Proteobacteria (²).
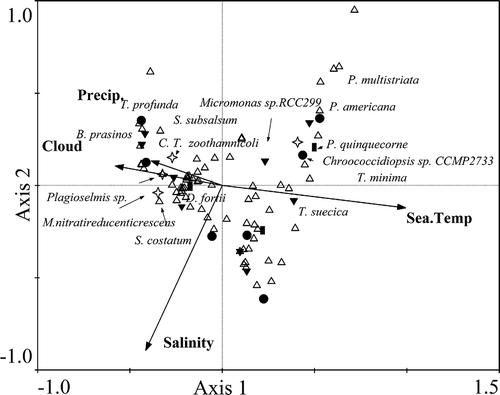
DISCUSSION
The rbcL genetic markers used to analyse the seasonal genetic diversity of phytoplankton in the coastal East China Sea adjacent to the Yangtze River Estuary may not indicate the proportion of a given taxon equivalent to that in the natural environment due to the biases introduced by PCR, as well as the low number of clones recovered relative to the number of organisms in natural seawater. Specifically, the efficiency and accuracy of PCR can be affected by many factors including primer-template mismatches, reactant concentrations, the number of PCR cycles, annealing temperature, the complexity of the DNA template and others (Von Wintzingerode et al., Citation1997; Smith et al., Citation2002; Forney et al., Citation2004; Mao et al., Citation2012). Additionally, Form I rbcL primers chosen in this study may have omitted a significant portion of dinoflagellates such as Prorocentrum (dominant in HABs in the coastal ECS). Form II RuBisCO actually exists in the cells of symbiotic dinoflagellates but was not amplified in this study (Bhadury & Ward, Citation2009).
The diversity of phytoplankton was found to be higher in autumn than in other seasons, and the dominant class and taxa also varied with the season. Our results for the dominant taxa and seasonal variation of diatoms and dinoflagellates are generally consistent with microscopic observations previously performed in this area. In addition, many Chlorophyta, Proteobacteria and Cyanobacteria taxa which may be overlooked by microscopy have been identified using the Form IAB rbcL genetic marker, and their diversity and seasonal variation are revealed. Overall, based on Form IAB rbcL, Chlorophyta were much more abundant than Cyanobacteria and Proteobacteria in coastal ECS throughout the year (). Micromonas sp. and Chroococcidiopsis sp. were the most dominant taxa of Chlorophyta and Cyanobacteria, respectively. Worden et al. (Citation2009) reported that Micromonas sp. RCC299 appeared in all temperature regimes of the globe, indicating its eurythermal characteristics and subsequently wide distribution. Zingone et al. (Citation1999) found that Micromonas pusilla was abundant from autumn to spring with peak values up to 6.6×103 cells ml−1, but was undetectable in summer in the Gulf of Naples in the Mediterranean Sea (Zingone et al., Citation1999). Micromonas can dominate taxa in the cold Arctic Ocean (Zhaxybayeva et al., Citation2009) and it was also observed widely in the Southern ocean (Díez et al., Citation2001), Blanes Gulf (Massana et al., Citation2004), and the English Channel (Romari & Vaulot, Citation2004). Chroococcidiopsis sp., the most dominant taxon, has good tolerance to extreme environmental conditions such as unfavourable temperature (Burkhard & Wessels, Citation1991) and salinity (Dor et al., Citation1991; Dillon et al., Citation2002). Both Micromonas sp. and Chroococcidiopsis sp. may cause HABs in coastal oceans (Shamsudin, Citation1999; Zingone et al., Citation1999). Chroococcidiopsis sp. showed negative and positive relationships with salinity and seawater temperature, respectively (). In contrast, Micromonas sp. was negatively correlated with seawater temperature.
Micromonas sp. was the dominant member of the Chlorophyta in summer, autumn and winter, but in spring Bathycoccus prasinos was found to be the most abundant (). A similar succession was reported in the western English Channel, where Bathycoccus prasinos became the most abundant species during spring (March 2001) and Micromonas pusilla peaked in both late spring and late summer (Not et al., Citation2005). Micromonas and Bathycoccus (Mamiellophyceae) are common dominant taxa of phytoplankton found in coastal oceans (Not et al., Citation2004; Treusch et al., Citation2011). Nonetheless, the seasonal variation of Chlorophyta has rarely been studied in the ECS.
Gammaproteobacteria were found to be the most dominant Proteobacteria with a total of 61 clones identified in autumn, winter and spring, and Alphaproteobacteria dominated the Proteobacteria group in summer in this study (, ). Our results are consistent with previous observations that Alphaproteobacteria is a common dominant group in both coastal and open oceans (Pinhassi & Hagstrom, Citation2000; Alonso-Saez et al., Citation2007) and Gammaproteobacteria is a common bacterium in sediments (Akob et al., Citation2007). The proportion of Alphaproteobacteria was found to be higher in summer but lower in other seasons compared with Gammaproteobacteria in this study. A similar succession between Proteobacteria taxa has been reported in the South China Sea with the highest proportions of Alphaproteobacteria and Gammaproteobacteria in April and October, respectively (Dong et al., Citation2014).
A seasonal succession was also found between diatom and dinoflagellate taxa. Skeletonema costatum, S. subsalsum and Dinophysis fortii are the most dominant taxa in summer, autumn and winter and spring in the coastal ECS, respectively (). The dominance of Skeletonema in the phytoplankton community of the ECS has been observed previously (Yang et al., Citation2014). Although no study directly reports the seasonal succession from Skeletonema to Dinophysis in the ECS, Prorocentrum donghaiense Lu (a dinoflagellate which was not identified in this study) has been found to be the major taxon in spring (Tian et al., Citation2010). It is very likely that a seasonal succession from diatom to dinoflagellate taxa occurs in the ECS since the proportion of dinoflagellates has increased substantially (fourfold) within the past 30 years (Yang et al., Citation2014).
Chlorophyta include picoeukaryotes, and along with Cyanobacteria both are important groups of primary producers in the ECS (Li & Luan, Citation1998). Synechococcus spp. (Cyanobacteria) could contribute approximately 10% and 3% of total primary productivity in the ECS in winter and summer, respectively (Xiao et al., Citation2003). Picoplankton could contribute approximately 68% of primary productivity in the coastal ECS in autumn (Liu et al., Citation2001). The biomass of picoplankton is strongly influenced by microzooplankton grazing but not by mesozooplankton (Zhang & Wang, Citation2001; Wang et al., Citation2005). Wilhelm & Avan (Citation2001) found that the grazing rate of microzooplankton would decrease as the cell abundance of phytoplankton was diluted significantly in the Northeast Atlantic. In these situations, microzooplankton chose to feed on Cyanobacteria instead of diatoms and Chlorophyta, although a preference for Chlorophyta by copepods was observed in the Norwegian Sea (Meyer et al., Citation1998). In the coastal ECS with abundant picoplankton, microzooplankton should have a high grazing rate and probably choose to feed on Chlorophyta which overwhelm Cyanobacteria throughout the year. The abundance of Chlorophyta is likely to be more affected by this predator–prey relationship than Cyanobacteria.
Dinophysis fortii was found to be the most dominant taxon, outnumbering the diatom taxa in spring and winter in the coastal ECS. Dinophysis fortii could adapt to temperatures between 7.2°C and 22.5°C, and it was observed in February at an average temperature of 7.5°C in Okkirai Bay (Koike et al., Citation2001). Similarly, Aubry et al. (Citation2000) observed that D. fortii was more abundant in October than in July in coastal waters of the northwest Adriatic Sea. Sidari et al. (Citation1995) found that D. fortii was more abundant in deeper and saltier waters in the Gulf of Trieste (Northern Adriatic Sea). In this study, Dinophysis fortii showed a positive relationship with salinity and a negative relationship with seawater temperature (), which may partly explain its dominance in winter and spring ().
The contamination of shellfish with lipophilic toxins produced by planktonic dinoflagellates in the genus Dinophysis is a worldwide problem for public health and aquaculture quality assurance (Yasumoto et al., Citation1980). Dinophysis taxa are ubiquitous in cool-temperate coastal waters. High levels of diarrhetic shellfish poisoning (DSP)-toxin contamination as a result of blooms of D. acuta have been documented in some coastal regions of New Zealand (MacKenzie et al., Citation2002). The occurrence and distribution of DSP toxins in nine taxa of shellfish (e.g. species of Venerupis, Mactra and Mytilus) along the coast of China were first reported by Zhou et al. (Citation1999). Although no DSP toxicity incidents were reported in the coastal seas of China until 2011, Dinophysis and benthic Prorocentrum, the organisms associated with DSP toxicity outbreaks are widely distributed throughout Chinese coastal waters (Chen & Ni, Citation1988; Lu & Hodgkiss, Citation2004; Wang et al., Citation2007). Dinophysis blooms, along with blooms of other species, have been observed occasionally at Nanji Islands in the ECS (Wang et al., Citation2007). These microalgae probably acquire plastids from cryptophytes in the environment prior to their blooms (Takahashi et al., Citation2005). Other potentially toxic taxa such as Pseudo-nitzschia spp. (including P. multistriata, P. americana and P. pungens) were also identified by the Form ID rbcL genetic marker. Nonetheless, they were not the dominant OTUs in this study with the highest proportion of approximately 14% of total OTUs being observed in summer. Sommer (Citation1994) indicated that stronger light and higher temperatures are conducive to Pseudo-nitzschia spp., and in our analysis, they showed weak positive relationships with seawater temperature ().
The dominance of Dinophysis fortii observed in this study suggests that dinoflagellates may play an important role in the phytoplankton community and may contribute to algal blooms in the coastal ECS. This also coincides with the changing of the blooming taxa from diatoms to dinoflagellates over the last decade in this area (Li et al., Citation2009). The abundance of D. fortii showed a significant negative correlation with seawater temperature (r = −0.576, P = 0.0001), consistent with the result of CCA. No significant relationships were found between Skeletonema costatum and Pseudo-nitzschia and environmental factors. Nicklisch et al. (Citation2008) suggested that temperature, light and other physical factors may be the main limiting factor of phytoplankton growth due to the current high level of nutrients in coastal waters. Generally, a temperature range between 20 and 30°C is suitable for red tide formation, and a sharp rise of more than 2°C within a week may cause an algal bloom. A high proportion of D. fortii coupled with relatively low temperatures may lead to spring blooms of this species in the coastal ECS.
DISCLOSURE STATEMENT
No potential conflict of interest was reported by the author(s).
SUPPLEMENTARY INFORMATION
The following supplementary material is accessible via the Supplementary Content tab on the article’s online page at http://dx.doi.org/10.1080/09670262.2015.1107138
Supplementary table S1. Dominant species of phytoplankton and their abundances. Identified at various locations and times in the East China Sea by previous studies published in Chinese.
Supplementary table S2. Cloud cover, precipitation, salinity and seawater temperature in the coastal East China Sea on sampling dates in this study.
Supplementary table S3. The degree of OTU similarity to reference sequence identified by Form IAB rbcL genetic marker.
Supplementary table S4. The degree of OTU similarity to reference sequence identified by Form ID rbcL genetic marker
Supplemental_tables S1-S4
Download PDF (389.4 KB)ACKNOWLEDGEMENTS
This work is sponsored by the National Natural Science Foundation of China (41375141), National Basic Research Program of China (2014CB953700), SRF for ROCS, SEM, Shanghai Science and Technology Committee (12DJ1400102), Hangzhou Science and Technology Development Project (20131813A03 and 20130533B04), and Hangzhou research project for environmental protection (2012001).
Additional information
Notes on contributors
Ying Chen
Z. Xu, analysis of molecular data, drafting manuscript; Y. Chen, original concept, editing manuscript; X. Meng, analysis of samples; F. Wang, collecting samples; Z. Zheng, assisting with sample collection and analysis.
REFERENCES
- Akob, D.M., Mills, H.J. & Kostka, J.E. (2007). Metabolically active microbial communities in uranium-contaminated subsurface sediments. FEMS Microbiology Ecology, 59: 95–107.
- Alonso-Saez, L., Balague, V. & Sa, E.L. (2007). Seasonality in bacterial diversity in north-west Mediterranean coastal waters: assessment through clone libraries, fingerprinting and fish. FEMS Microbiology Ecology, 60: 98–112.
- Andersson, I. & Backlund, A. (2008). Structure and function of Rubisco. Plant Physiology and Biochemistry, 46: 275–291.
- Aubry, F.B., Bastianini, M., Bertaggia, R., Baroni, A. & Socal, G. (2000). Seasonal dynamics of Dinophysis in coastal waters of the NW Adriatic Sea, 1990–1996. Botanica Marina, 43: 423–430.
- Bhadury, P. & Ward, B.B. (2009). Molecular diversity of marine phytoplankton communities based on key functional genes. Journal of Phycology, 45: 1335–1347.
- Burkhard, B. & Wessels, D.C.J. (1991). Rock inhabiting blue-green algae/cyanobacteria from hot arid regions. Archiv für Hydrobiologie, 64: 385–398.
- Chen, G.W. & Ni, S.D. (1988). Taxonomic studies on three genera of Dinophyceae in South China Sea. Oceanologia et Limnologia Sinica, 19: 238–248. [in Chinese]
- Chen, Y. L., Chen, H., Gong, G., Lin, Y., Jan, S. & Takahashi, M. (2004). Phytoplankton production during a summer coastal upwelling in the East China Sea. Continental Shelf Research, 24: 1321–1338.
- Díez, B., Pedrós-Alió, C.C., Marsh, T. L. & Massana, R. (2001). Application of denaturing gradient gel electrophoresis (DGGE) to study the diversity of marine picoeukaryotic assemblages and comparison of DGGE with other molecular techniques. Applied and Environmental Microbiology, 67: 2942–2951.
- Dillon, J.G., Tatsumi, C.M., Tandingan, P.G. & Castenholz, R.W. (2002). Effect of environmental factors on the synthesis of scytonemin, a UV-screening pigment, in a cyanobacterium, Chroococcidiopsis sp. Archives of Microbiology, 177: 322–331.
- Dong Y., Zhao Y., Zhang W.Y., Li Y., Zhou F., Zhang W.C. & Xiao T. (2014). Bacterial diversity and community structure in the East China Sea by 454 sequencing of the 16S rRNA gene. Chinese Journal of Oceanology and Limnology, 32: 527–541.
- Dor, I., Carl, N. & Baldinger, I. (1991). Polymorphism and salinity tolerance as a criterion for differentiation of three new species of Chroococcidiopsis, Chroococcales. Archiv für Hydrobiologie, 64: 411–421.
- Fan, W. & Song, J. (2014). A numerical study of the seasonal variation of nutrients in the Changjiang River estuary and its adjacent area. Ecological Modelling, 291: 69–81.
- Felsenstein, J. (1989). PHYLIP – Phylogeny Inference Package (Version 3.2). Cladistics, 5: 164–166.
- Forney, L.J., Zhou, X. & Brown, C.J. (2004). Molecular microbial ecology: land of the one-eyed king. Current Opinion in Microbiology, 7: 210–220.
- Furuya, K., Hayashi, M., Yabushita, Y. & Ishikawa, A. (2003). Phytoplankton dynamics in the East China Sea in spring and summer as revealed by HPLC-derived pigment signatures. Deep-sea Research Part II, 50: 367–387.
- Gao, B. & Shao, A. (2011). Study on characteristics, mechanisms and strategies on Harmful Algal Blooms in China coastal waters. Marine Forecasts, 28: 68–77. [in Chinese]
- Ghosh, S. & Love, N.G. (2011). Application of rbcL based molecular diversity analysis to algae in wastewater treatment plants. Bioresource Technology, 102: 3619–3622.
- Gong, G., Wen, Y., Wang, B. & Liu, G. (2003). Seasonal variation of chlorophyll a concentration, primary production and environmental conditions in the subtropical East China Sea. Deep-sea Research Part II, 50: 1219–1236.
- Guo, L., Chen, Y., Wang, F., Meng, X., Xu, Z. & Zhuang, G. (2014). Effects of Asian dust on the atmospheric input of trace elements to the East China Sea. Marine Chemistry, 163: 19–27.
- Guo, S.J., Tian, W., Dai, M.H., Liu, Z. & Sun, J. (2011). Phytoplankton assemblages in the East China Sea in summer 2009. Advances in Marine Science, 29: 474–486. [in Chinese]
- Hall, T.A. (1999). BioEdit: a user-friendly biological sequence alignment editor and analysis program for Windows 95/98/nt. Nucleic Acids Symposium Series, 41: 95–98.
- Jiao, N.Z., Yang, Y.H., Hong, N., Ma, Y., Harada, S., Koshikawa, H. & Watanabe, M. (2005). Dynamics of autotrophic picoplankton and heterotrophic bacteria in the East China Sea. Continental Shelf Research, 25: 1265–1279.
- Koike, K., Otobe, H., Takagi, M., Yoshida, T., Ogata, T. & Ishimaru, T. (2001). Recent occurrence of Dinophysis fortii, Dinophyceae in the Okkirai Bay, Sanriku, Northern Japan, and related environmental factors. Journal of Oceanography, 57: 165–175.
- Li, C. & Luan, F. (1998). A preliminary study on the distribution of size-fractionated chlorophyl-l a in the euphotic zone of the East China Sea in spring. Marine Sciences, 4: 59–62. [in Chinese]
- Li, J., Glibert, P.M., Zhou, M.J., Lu, S.H. & Lu, D.D. (2009). Relationships between nitrogen and phosphorus forms and ratios and the development of dinoflagellate blooms in the East China Sea. Marine Ecology Progress Series, 383: 11–26.
- Liu, L.S., Li, Z.C, Zhou, J., Zheng, B.H. & Tang, J.L. (2011). Temporal and spatial distribution of red tide in Yangtze River Estuary and adjacent waters. Environmental Science, 32: 2497–2504. [in Chinese]
- Liu, Z.L., Ning, X. R. & Cai, Y. M. (2001). Primary productivity and standing stock of the phytoplankton in the Hangzhou Bay to the Zhoushan Fishing Ground during autumn. Acta Oceanologica Sinica, 23: 93–99. [in Chinese]
- Lu, S.H. & Hodgkiss, I.J. (2004). Harmful algal bloom causative collected from Hong Kong waters. Hydrobiologia, 512: 231–238.
- Luo, M.B., Lu, J.J., Wang, Y.L., Shen, X.Q. & Chao, M. (2007). Horizontal distribution and dominant species of phytoplankton in the East China Sea. Acta Ecologica Sinica, 27: 5076–5085. [in Chinese]
- MacKenzie, L., McNabb, P., Holland, P., Beuzenberg, V., Selwood, A. & Suzuki, T. (2002). Complex toxin profiles in phytoplankton and Greenshell mussels (Perna canaliculus), revealed by LC–MS/MS analysis. Toxicon, 40: 1321–1330.
- Mao, D.P., Zhou, Q., Chen, C.Y. & Quan, Z.X. (2012). Coverage evaluation of universal bacterial primers using the metagenomic datasets. BMC Microbiology, 12: 66.
- Massana, R., Balague, V. & Guillou, L. (2004). Picoeukaryotic diversity in an oligotrophic coastal site studied by molecular and culturing approaches. FEMS Microbiology Ecology, 50: 231–243.
- Medlin, L.K. & Kaczmarska, I. (2004). Evolution of the diatoms V: morphological and cytological support for the major clades and a taxonomic revision. Phycologia, 43: 245–270.
- Meyer, H.B., Irigoien, X. & Head, R.N. (1998). Selective feeding on natural phytoplankton by Calanus finmarchicus before, during and after the 1997 spring bloom in the Norwegian Sea. Limnology and Oceanography, 44: 154–165.
- Nicklisch, A., Shatwell, T. & Koehler, J. (2008). Analysis and modelling of the interactive effects of temperature and light on phytoplankton growth and relevance for the spring bloom. Journal of Plankton Research, 30: 75–91.
- Not, F., Latasa, M. & Marie, D. (2004). A single species, Micromonas pusilla (Prasinophyceae), dominates the eukaryotic picoplankton in the Western English Channel. Applied and Environmental Microbiology, 70: 4064–4072.
- Not, F., Latasa, M., Massana, R., Colson, C., Marie, D. & Eikrem, W. (2005). Late summer community composition and abundance of photosynthetic picoeukaryotes in Norwegian and Barents Seas. Limnology and Oceanography, 50: 1677–1686.
- Paul, J.H., Alfreider, A. & Wawrik, B. (2000). Micro- and macrodiversity in rbcL sequences in ambient phytoplankton populations from the southeastern Gulf of Mexico. Marine Ecology Progress Series, 198: 9–18.
- Pinhassi, J. & Hagstrom, A. (2000). Seasonal succession in marine bacterioplankton. Aquatic Microbial Ecology, 21: 245–256.
- Prasad, A., Nienow, J.A. & Livingston R.J. (2002). The marine diatom genus Tryblioptychus hendey (Thalassiosiraceae, Coscinodiscophyceae): fine structure, taxonomy, systematics and distribution. Diatom Research, 17: 291–308.
- Romari, K. & Vaulot, D. (2004). Composition and temporal variability of picoeukaryote communities at a coastal site of the English Channel from 18S rDNA sequences. Limnology and Oceanography, 49: 784–798.
- Samanta, B., Bhadury, P. & Mock, T. (2014). Analysis of diversity of chromophytic phytoplankton in a mangrove ecosystem using rbcL gene sequencing. Journal of Phycology, 50: 328–340.
- Schloss, P.D. & Handelsman, J. (2005). Introducing DOTUR, a computer program for defining operational taxonomic units and estimating species richness. Applied and Environmental Microbiology, 71: 1501–1506.
- Shamsudin, L. (1999). The blue green algal bloom in the nearshore waters of Cukai Bay facing the South China Sea. Environmental Monitoring and Assessment, 59: 123–134.
- Sidari, L., Cok, S., Cabrini, M., Tubaro, A. & Honsell, G. (1995). Temporal distribution of toxic phytoplankton in the Gulf of Trieste (Northern Adriatic Sea) in 1991 and 1992. In Proceedings of Sixth International Conference on Toxic Marine Phytoplankton (Lassus, P., Arzul, G., Erard, E., Gentien, P. & Marcaillou, C., editors), 231–236. Lavoisier, Intercept Ltd, Paris.
- Smith, S., Vigilant, L. & Morin, P.A. (2002). The effects of sequence length and oligonucleotide mismatches on 5’ exonuclease assay efficiency. Nucleic Acids Research, 30: 1–11.
- Sommer, U. (1994). Are marine diatoms favored by high Si: N ratio? Marine Ecology Progress Series, 115: 309–315.
- Sun, J., Yu, Z.G., Gao, Y.H., Zhou, Q.Q., Zhen, Y. & Chen, H.T. (2010). Phytoplankton diversity in the East China Sea and Yellow Sea measured by PCR-DGGE and its relationships with environmental factors. Chinese Journal of Oceanology and Limnology, 28: 315–322.
- Tabita, F. R. (1999). Microbial ribulose 1, 5-bisphosphate carboxylase/oxygenase: a different perspective. Photosynthesis Research, 60: 1–28.
- Tabita, F.R., Hanson, T.E., Li, H.Y., Satagopan, S., Singh, J. & Chan, S. (2007). Function, structure, and evolution of the RubisCO-like proteins and their RubisCO homologs. Microbiology and Molecular Biology Reviews, 71: 576–599.
- Tabita, F.R., Hanson, T.E., Satagopan, S., Witte, B.H. & Kreel, N.E. (2008). Phylogenetic and evolutionary relationships of RubisCO and the RubisCO-like proteins and the functional lessons provided by diverse molecular forms. Philosophical Transactions of the Royal Society B, 363: 2629–2640.
- Takahashi, Y., Takishita, K., Koike, K., Maruyama, T., Nakayama, T. & Kobiyama, A. (2005). Development of molecular probes for Dinophysis, Dinophyceae plastid: a tool to predict blooming and explore plastid origin. Marine Biotechnology, 7: 95–103.
- Tamura, K., Dudley, J. & Nei, M. (2007). MEGA4: Molecular Evolutionary Genetics Analysis (MEGA) software version 4.0. Molecular Biology and Evolution, 224: 1596–1599.
- Theriot, E.C., Cannone, J.J., Gutell, R. R. & Alverson, A. J. (2009). The limits of nuclear-encoded SSU rDNA for resolving the diatom phylogeny. European Journal of Phycology, 44: 277–290.
- Thompson, J.D., Gibson, T.J. & Plewniak, F. (1997). The Clustal X windows interface: flexible strategies for multiple sequence alignment aided by quality analysis tools. Nucleic Acids Research, 25: 4876–4882.
- Tian, R. & An, J. (2012). Relationship between aerosol transport routes and red tide occurrences in the East China Sea. Environmental Earth Sciences, 9: 32–38.
- Tian, W., Sun, J., Fan, X.P. & Wang, M. (2010). Phytoplankton community in coastal waters of the East China Sea in spring 2008. Advances in Marine Science, 28: 170–178. [in Chinese]
- Treusch, A. H., Demir-Hilton, E. & Vergin, K. L. (2011). Phytoplankton distribution patterns in the northwestern Sargasso Sea revealed by small subunit rRNA genes from plastids. SME Journal, 6: 481–492.
- Varela-Alvarez, E., Andreakis, N., Lago-Leston, A., Pearson, G.A., Serrao, E.A., Procaccini, G., Duarte, C. M. & Marba, N. (2006). Genomic DNA isolation from green and brown algae (Caulerpales and Fucales) for microsatellite library construction. Journal of Phycology, 42: 741–745.
- Vaulot, D., Eikrem, W., Viprey, M. & Moreau, H. (2008). The diversity of small eukaryotic phytoplankton(≤ 3µm) in marine ecosystems. FEMS Microbiology Ecology, 32: 795–820.
- Von Wintzingerode, F., Gobel, U.B. & Stackebrandt, E. (1997). Determination of microbial diversity in environmental samples: pitfalls of PCR-based rRNA analysis. FEMS Microbiology Ecology, 21: 213–229.
- Wang, J.H., Qin, Y.T., Liu, C.C., Sun, Y., Cheng, X. & Xu, R. (2007). A background investigation of toxic algae and biotoxin in the Yangtze estuary. Transactions of Oceanology and Limnology, 53: 52–61. [in Chinese]
- Wang, X.D., Sun, J., Liu, D.Y. & Wang, M. (2005). Control of phytoplankton community by marine mesozooplankton selective grazing. Advances in Marine Science, 23: 525–533. [in Chinese]
- Weng, H.X., Tian, R.X., Ji, Z.Q. & Yu, X.A. (2011). Potential relationships between atmospheric particulate matter transported by winter monsoons and red tides in the East China Sea. Chinese Science Bulletin, 56: 297–305.
- Wilhelm, G. & Avan, N. A. (2001). Taxon-specific growth and selective microzooplankton grazing of phytoplankton in the Northeast Atlantic. Journal of Marine Systems, 30: 241–261.
- Wojtal, A.Z. & Kwandrans, J. (2006). Diatoms of the Wyżyna Krakowsko-Częstochowska upland (Spoland) – Coscinodiscophyceae (Thalassiosirophycidae). Polish Botanical Journal, 51: 177–207.
- Worden, A.Z., Lee, J-H. & Mock, T. (2009). Green evolution and dynamic adaptations revealed by genomes of the marine picoeukaryote Micromonas. Science, 10: 267–272.
- Wu, H., Zhu, J.R., Shen, J. & Wang, H. (2011). Tidal modulation on the Changjiang River plume in summer. Journal of Geophysical Research, 116: doi 10.1029/2011JC007209.
- Xia, J.L., Liu, P., Wan, M.X., Wang, R.M., Li, L. & Qiu, G.Z. (2010). Cloning and sequence analysis of rbcL gene from Chlorella sorokiniana and comparison with 18S rDNA gene in taxonomy. Progress in Modern Biomedicine, 10: 4601–4605. [in Chinese]
- Xiao, T., Yue, H. D. & Zhang, W. C. & Wang, R. (2003). Distribution of Synechococcus spp. in the East China Sea and its role in microbial food web. Oceanologia et Limnologia Sinica, 34: 33–43. [in Chinese]
- Yang, S., Han, X., Zhang, G., Sun, B., Wang, X. & Shi, X. (2014). Seasonal changes in phytoplankton biomass and dominant species in the Changjiang River estuary and adjacent seas: General trends based on field survey data 1959–2009. Journal of Ocean University of China, 13: 926–934.
- Yasumoto, T., Oshima, Y., Sugaware, W., Fukuyo, Y., Oguri, H., Igarashi, T. & Fujita, N. (1980). Identification of Dinophysis fortii as the causative organism of diarrhetic shellfish poisoning. Bulletin of the Japanese Society for the Science of Fish, 46: 1405–1411.
- Zhang, W. & Wang, R. (2001). Grazing pressure of microzooplankton on phytoplankton and primary production in marine ecosystem. Acta Oceanologica Sinica, 21: 1360–1366. [in Chinese]
- Zhaxybayeva, O., Doolittle, W.F., Papke, R.T. & Gogarten. J.P. (2009). Intertwined evolutionary histories of marine Synechococus and Prochlorococcus marinus. Genome Biology and Evolution, l: 325–339.
- Zhou, M., Li, J., Luckas, B., Yu, R. C., Yan, T. & Hummert, C. (1999). A recent shellfish toxin investigation in China. Marine Pollution Bulletin, 39: 331–334.
- Zhou, M., Shen, Z. & Yu, R. (2008). Responses of a coastal phytoplankton community to increased nutrient input from the Changjiang (Yangtze) River. Continental Shelf Research, 28: 1483–1489.
- Zhu, L., Chen, Y., Guo, L. & Wang, F. (2013). Estimate of dry deposition fluxes of nutrients over the East China Sea: the implication of aerosol ammonium to non-sea-salt sulfate ratio in nutrient deposition of coastal oceans. Atmospheric Environment, 69: 131–138.
- Zingone, A., Sarno, D. & Forlani, G. (1999). Seasonal dynamics in the abundance of Micromonas pusilla (Prasinophyceae) and its viruses in the Gulf of Naples (Mediterranean Sea). Journal of Plankton Research, 21: 2143–2159.