Abstract
Species belonging to the dinophyte genus Scrippsiella are frequently reported in marine waters, but information on their distribution in brackish environments is limited. Here we describe a new species, S. plana, through incubation of non-calcified cysts from sediments collected in the South China Sea and Caspian Sea. The vegetative cells consist of a conical epitheca and a rounded hypotheca with the plate formula of Po, X, 4′, 3a, 7′′, 5C+t, 5S, 5′′′, 2′′′′. It differs from other Scrippsiella species by its flattened body in dorsoventral view and a small first anterior intercalary (1a) plate (half the size of plate 3a). Scrippsiella plana strains from the South China Sea and Caspian Sea share identical internal transcribed spacer (ITS) sequences, and show phenotypic plasticity and local adaptation in growth rate at various salinities, consistent with the environments in which they originated. In addition, two strains of S. spinifera were obtained by incubating ellipsoid cysts with calcareous spines from sediments collected along the Turkish and Hawaiian coast. They also share identical ITS sequences and differ from Duboscquodinium collinii (a parasite of tintinnids) only at two base pair positions (in the ITS2 region). Molecular phylogeny based on ITS and large subunit ribosomal DNA (LSU rDNA) sequences revealed that S. plana was nested within the Calciodinellum (CAL) clade and S. spinifera within the S. trochoidea (STR) clade. The phylogenetic position of ‘Peridinium’ wisconsinense is reported for the first time, which supports multiple transitions of the Peridiniales to freshwater.
INTRODUCTION
The dinophyte genus Scrippsiella Balech ex A.R. Loebl. was established with S. sweeneyae (sweeneyi in the original description) Balech as the type species, showing a plate pattern of Po, X, 4′, 3a, 7′′, 5C + t, 4S, 5′′′, 2′′′′ (Balech, Citation1959). To date, approximately 30 extant species were described under this genus (e.g. Indelicato & Loeblich III, 1985; Montresor & Zingone, Citation1988; Lewis, Citation1991; Larsen et al., Citation1995; Montresor, Citation1995; Attaran-Fariman & Bolch, Citation2007; Gu et al., Citation2008, Citation2013; Zinssmeister et al., Citation2012). Scrippsiella species are predominantly marine and free living (Elbrächter et al., Citation2008), although the problematic taxon S. saladensis Balech inhabits exclusively brackish waters (Balech, Citation1963). Another exceptional lifestyle has recently been reported: symbiotic stages of Scrippsiella in other protists (Decelle et al., Citation2012). In this paper one new Scrippsiella species is described, Scrippsiella plana, from the marine South China Sea as well as in the brackish Caspian Sea that produces non-calcareous cysts. We also report the morphology and phylogenetic position of S. spinifera from the Turkish coast and Hawaiian coast.
The cell morphology of Scrippsiella species is rather conserved, showing a symmetric (bipesioid) or less commonly non-symmetric (cinctioid) epitheca (i.e. the second anterior intercalary plate (2a) either large or small, with 1a in contact with 3a). A bipesioid epitheca is common in Scrippsiella, whereas the cinctioid epitheca was only found in S. precaria Montresor & Zingone, S. ramonii Montresor, and S. irregularis Attaran-Fariman & Bolch (Montresor & Zingone Citation1988; Montresor, Citation1995; Attaran-Fariman & Bolch, Citation2007). In the molecular phylogeny they form a well-resolved group together with Naiadinium polonicum (Wołoszyńska) S. Carty, a freshwater species with one or two anterior intercalary plates (Hu et al., Citation2008; Craveiro et al., Citation2015). The bipesioid type is also present in several cyst-based genera, such as Calciodinellum Deflandre and Pernambugia Janofske & Karwath and molecular phylogeny support that these are closely related to Scrippsiella (Montresor et al., Citation2003; Gottschling et al., Citation2005). Whether symmetric or non-symmetric epitheca are systematically important is not clear, and this paper further investigates this by reporting the phylogenetic position of a species with a symmetrical and a species with a non-symmetrical epitheca.
Most Scrippsiella species produce calcareous cysts, which are either process-bearing or relatively smooth (Lewis, Citation1991; Janofske, Citation2000; Meier et al., Citation2002; Montresor et al., Citation2003), but non-calcareous cysts are also reported in several Scrippsiella species, e.g. S. donghaiensis H. Gu, and S. enormis H. Gu (Gu et al., Citation2008, Citation2013). Cyst morphology plays an important role in differentiating Scrippsiella species since many of them share identical thecate morphology (Montresor et al., Citation1997; Gu et al., Citation2008). In contrast, S. spinifera Honsell & Cabrini is unusual in its thecate morphology because it has several antapical spines, which have not been observed in other Scrippsiella species (Honsell & Cabrini, Citation1991). The cyst-theca relationship for this species was established by Kobayashi (Citation1995) from a Japanese sample, however the phylogenetic position of S. spinifera remains to be determined (Gu et al., Citation2013). Here we reestablish the cyst-theca relationship of S. spinifera from the Turkish coast and Hawaiian coast, and report the cyst-theca relationship of a new species, S. plana, from the Caspian Sea and South China Sea. The taxonomy of Scrippsiella became more complicated by the recognition of cryptic species, which can only be differentiated using molecular techniques. The most common species of Scrippsiella, i.e. S. trochoidea (F.Stein) A.R.Loebl., comprises three genetically diverse clades, designated as STR1, STR2 and STR3 (Montresor et al., Citation2003; Gottschling et al., Citation2005). Recently, strains of S. trochoidea from the type locality proved to be in STR2, thus STR1 and STR3 might not be true S. trochoidea at all (Zinssmeister et al., Citation2011; Soehner et al., Citation2012; Kretschmann et al., Citation2014). The true S. trochoidea (STR2) is now considered a heterotypic synonym of S. acuminata (Ehrenb.) Kretschmann, Elbr., Zinssmeister, S. Soehner, Kirsch, Kusber & Gottschling (Kretschmann et al., Citation2015). Molecular phylogeny furthermore suggested that the diversity of Scrippsiella was underestimated (Gu et al., Citation2008). On the other hand, phylogenetic positions of many Scrippsiella species (e.g. S. saladensis, S. regalis (Gaarder) Janofske, S. minima Gao & Dodge, S. tinctoria Indelicato & Loeblich III) remain to be determined.
The molecular phylogenies of Scrippsiella species are mostly based on internal transcribed spacer (ITS) sequences (D’Onofrio et al., Citation1999; Gottschling et al., Citation2005; Zinssmeister et al., Citation2012; Craveiro et al., Citation2013; Gu et al., Citation2013) and occasionally on large subunit ribosomal DNA (LSU rDNA) sequences (Craveiro et al., Citation2011; Zinssmeister et al., Citation2012). There is a clade including species of Calciodinellum, Duboscquodinium Grassé, Naiadinium S. Carty, Pernambugia, Scrippsiella and Theleodinium Craveiro, Pandeirada, Daugbjerg, Moestrup & Calado as well, most of them assigned to Scrippsiella (Gottschling et al., Citation2005; Craveiro et al., Citation2013, Citation2015). Internally, the clade is not finally resolved, neither phylogenetically nor taxonomically, but we refer to this clade as Scrippsiella sensu lato here. In molecular trees, the clade segregates into a number of lineages, comprising CAL (Calciodinellum and related species), LAC (Scrippsiella lachrymosa Lewis and related species), PRE (Scrippsiella precaria and related species), STR (Scrippsiella trochoidea and related species) (Zinssmeister et al., Citation2012; Gu et al., Citation2013). The new sequences (ITS and LSU) reported here contribute further to understanding of the phylogeny and systematics of Scrippsiella species. In addition we report the phylogenetic position of ‘Peridinium’ wisconsinense Eddy, providing further insights into the importance of Scrippsiella species in the transition to freshwater environments.
MATERIALS AND METHODS
Sample collection and cultures
Surface sediment samples were collected from the South China Sea and Caspian Sea, and from each of these samples, one strain of Scrippsiella plana was established (). Surface sediment samples were also collected from the Hawaiian coast and Izmir Bay (Turkey) and from each of these samples, one strain of S. spinifera was established (). Cysts of ‘Peridinium’ wisconsinense were isolated from surface sediment from Plastic Lake, a small (32 ha), 16.3 m deep lake on the Canadian Shield (Ontario, Canada) (). The sediment samples were stored in the dark at 4°C, after which approximately 5 g of wet sediment was mixed with 20 ml of filtered seawater (distilled water for the Canadian sample) and sonicated for 2 min (100 watts) to dislodge detrital particles. The sample was then filtered through a 100 µm sieve and subsequently through a 20 µm sieve. The 20–100 µm fraction was re-suspended in 1 ml filtered seawater, and single cysts were picked using a pipette and each placed in a single well of a 96-well plate each with 300 μl f/2-Si medium (Guillard & Ryther Citation1962) for germination at 20°C and illuminated with 90 μmol photons m−2 s−1 under a 12:12 h L:D cycle (hereafter called ‘standard conditions’). For the S. plana strain LH006 from the Caspian Sea, the salinity was adjusted to 12. Cultures were maintained under standard conditions.
Table 1. Scrippsiella spp. and “Peridinium” wisconsinense, corresponding strains, location name, latitude, longitude, water depth, collection date, sampling device and name of person who did sampling.
Light microscopy (LM)
Vegetative cells were examined using a Zeiss Axio Imager microscope (Carl Zeiss, Göttingen, Germany) that was equipped with differential interference illumination, epifluorescence and a Zeiss Axiocam HRc digital camera. To observe the shape and location of the nucleus, cells were stained with 1:100 000 SYBR Green (Sigma Aldrich, St. Louis, Missouri, USA) for 1 min, and photographed under the Zeiss fluorescence microscope with a Zeiss-38 filter set (excitation BP 470/40, beam splitter FT 495, emission BP 525/50). Cells in the mid-exponential growth phase were fixed with 5% Lugol’s solution and cell size was measured at 400×. Fifty cells were measured for Scrippsiella plana strain SSFC13 and S. spinifera strain SSHWI01 each. Fifteen cysts formed in culture of each of these strains were measured. All cyst and motile cell measurements in the species descriptions cite the minimum, average (in parentheses) and maximum values (µm), in that order. The standard deviation (stdev) is also provided where appropriate.
Scanning electron microscopy (SEM)
For scanning electron microscopy, mid-exponential batch cultures were collected by centrifugation at 4500 g. The supernatant was removed and the cell pellet was re-suspended in 60% ethanol for 1 h at 8ºC to strip off the outer cell membranes. The cells were centrifuged again and the pellet was re-suspended in 40% seawater at room temperature for 30 min. Subsequently, the cell pellet or cysts generated in culture were fixed at room temperature for 3 h with 2.5% glutaraldehyde prepared with f/2-Si medium. The supernatant was removed, and the cell pellet or cysts were applied to a coverslip coated with poly-L-lysine (molecular mass 70 000–150 000). They were allowed to adhere to the coverslip for 30 min, and then washed for 10 min in a 1:1 solution of distilled water and filtered seawater, followed by a second wash for 10 min using only distilled water. The samples were then dehydrated in an ethanol series (10, 30, 50, 70, 90 and 3× in 100%, 10 min at each step), critical point dried (K850 Critical Point Dryer, Quorum/Emitech, West Sussex, UK), sputter-coated with gold, and examined using LEO 1530 Gemini SEM (Zeiss/LEO, Oberkochen, Germany). Plate terminology follows the modified Kofoidian system (Balech, Citation1980).
DNA extraction and PCR amplification
Total algal DNA of S. plana (strains SSFC13 and LH006) and S. spinifera (strain SSHWI01) was extracted from 10 ml of exponentially growing cultures using a plant DNA extraction kit (Sangon, Shanghai, China) according to the manufacturer’s protocol. The total ITS1–5.8S–ITS2 was amplified using ITSA and ITSB primers (Adachi et al., Citation1996). Approximately 1400 bp of the LSU rDNA (D1–D6) was amplified using the primers of D1R (Scholin et al., Citation1994) and 28-1483R (Daugbjerg et al., Citation2000). A 50 µl PCR cocktail containing 0.2 µM primers, PCR buffer, 50 µM dNTP mixture, 10 ng of template genomic DNA, 1U of Ex Taq DNA polymerase (Takara, Dalian, China) was subjected to 35 cycles using a Mastercycler PCR (Eppendorf, Hamburg, Germany). The PCR protocol was as follows: initial denaturation for 3.5 min at 94ºC, followed by 35 cycles of 50 s denaturation at 94ºC, 50 s annealing at 45ºC, and 80 s extension at 72ºC, plus a final extension of 10 min at 72ºC. PCR products were sequenced directly in both directions using theABI Big-Dye dye-terminator technique (Applied Biosystems, Foster City, California, USA), according to the manufacturer’s recommendations.
The cells of S. spinifera strain from the Turkish coast were individually transferred on a glass slide with a frame of vinyl tape, and observed and photographed using an Olympus BX51 microscope equipped with Nomarski differential interference contrast optics (Olympus, Tokyo, Japan) and with an Olympus DP71 digital camera. After taking photographs the cover slip was carefully removed. Under an inverted microscope (Olympus CKX41) the cell was picked up and crushed with a fine glass needle. The whole crushed cell was transferred into a 200 µl PCR tube containing 3 µl of Milli-Q water. This technique and the following PCR protocol are modifications of the methods in Takano & Horiguchi (Citation2006). We determined sequences of ITS regions (internal transcribed spacer 1 – 5.8 rDNA – ITS2) and partial sequences of SSU and LSU rDNA from single-cysts and 5–10 cultured cells. In the first round of PCR, the external primers (SR1 and LSU R2; Takano & Horiguchi Citation2006) were used with PCR mixtures of KOD-Plus-Ver. 2 Kit (Toyobo, Osaka, Japan) and the PCR conditions; one initial cycle of denaturation at 94ºC for 2 min, followed by 35 cycles of denaturation at 94ºC for 30 s, annealing at 50ºC for 30 s, and extension at 72ºC for 2 min and final extension at 72ºC for 5 min. In the second round of PCR, two sets of primers (SR12cF and 25R1, LSU D1R and LSU R2; Takano & Horiguchi, Citation2006) were used with PCR mixtures of KOD-Plus-Ver. 2 Kit (Toyobo, Osaka, Japan), 0.5 µl of the first-round PCR product as DNA template, and the same PCR conditions except for extension at 72ºC for 1 min. In the third round of PCR, three sets of primers (SR12cF and 25F1R, LSU D1R and 25R1, LSU D3A and LSU R2; Takano & Horiguchi, Citation2006) were used with PCR mixtures of the TaKaRa EX taq system (Takara Bio Inc., Shiga, Japan), 0.5 µl of the second-round PCR products as DNA template, and the same PCR conditions except for extension at 72ºC for 30 s. PCR products were sequenced directly using the ABI PRISM BigDye Terminator Cycle Sequencing Kit (Perkin-Elmer, Foster City, California, USA). We sequenced both the forward and reverse strands. Three cysts of ‘Peridinium’ wisconsinense were isolated from surface sediment from Plastic Lake (Canada) and LSU and SSU were sequenced in a similar fashion as the Turkish strains of S. spinifera. Sequences were deposited in GenBank with accession numbers from KT804912 to KT804922.
Alignment and phylogeny
ITS and LSU sequences newly obtained were first aligned with those of related species available in GenBank using ‘BioEdit’ v7.0.0 (Hall, Citation1999), and then using Mafft (Katoh et al., Citation2005) (http://mafft.cbrc.jp/alignment/server/). Ensiculifera cf. imariensis Kobayashi & Matsuoka and Prorocentrum cordatum (Ostenfeld) J.D. Dodge were used as the outgroup of ITS and LSU sequences respectively. For the ITS sequences alignment, we did not find any ambiguous regions. The optimal model was chosen using JmodelTest (Posada, Citation2008). A general time reversible model (GTR +I+G) was selected with Akaike information criterion. Maximum likelihood-based analyses were conducted with ‘RAxML’ v7.2.6 (Stamatakis, Citation2006) using the model GTR+G on the T-REX web server (Boc et al., Citation2012). Node support was assessed with 1000 bootstrap replicates. A Bayesian reconstruction of the data matrix was performed with MrBayes 3.0b4 (Ronquist & Huelsenbeck, Citation2003) using the model GTR+G. Four Markov chain Monte Carlo (MCMC) chains ran for two million generations, sampling every 1000 generations with a burn-in of 10%. A majority rule consensus tree was created in order to examine the posterior probabilities of each clade.
Growth
To adapt the strain to different salinity levels, cultures were transferred successively from the maintenance salinity of 28 to 24, 20, 16, 12, 8, 6, 4, and 2. When an inoculum culture had entered exponential growth phase at a given salinity, subsamples (three replicates) were inoculated at initial concentrations of ~500 cells ml–1 in 200 ml glass bottles, each containing 100 ml of f/2-Si at the next salinity level. The light:dark cycle in all experiments was 12:12 h. For sampling, 0.5 ml were removed from the culture at 3-day intervals and fixed in Lugol’s solution. Each sample was transferred to a Sedgwick–Rafter chamber and a minimum of 200 cells per sample were counted.
Maximum number of divisions per day (k) was determined for the exponential growth phase, using the formula k = [ln(Nt /N0) /△t] /0.6931, where N0 is the population size at the beginning of a time interval, Nt is the population size at the end of a time interval, and △t is the length of the time interval.
RESULTS
Scrippsiella plana Z. Luo, K.N. Mertens, S. Bagheri & H. Gu, sp. nov. (–)
Figs 1–4. Scrippsiella plana, LM of vegetative cells (strain SSFC13). Fig. 1. Ventral view of a cell with chloroplasts (C) near the surface. Fig. 2. Apical view of a cell showing the flattening body. Fig. 3. Ventral view of a cell, showing three putative stalked pyrenoids (P). Fig. 4. A SYBR Green stained cell in ventral view, showing a round nucleus (N) in the central part. Scale bars = 5µm.
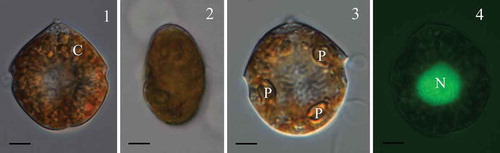
Description: Epitheca conical, hypotheca rounded. Plate tabulation pattern Po, X, 4′, 3a, 7′′, 5C+t, 5S, 5′′′, 2′′′′. Vegetative cells flattened in dorsoventral view, 19.0 (26.6) 34.5 µm long, 15.0 (23.2) 31.0 μm wide and 10.0 (14.4) 17.0 μm deep. Plate 1a small, about half size of plate 3a. Cysts spherical with a diameter of 22.5 (23.8) 25.0 μm and non-calcareous.
Holotype: SEM stub TIO2015PER01 from strain SSFC13 deposited at Third Institute of Oceanography, SOA, Xiamen 361005, China.
Type locality: South China Sea (21°31′24.7″N, 108°5′23.3″E).
Etymology: ‘plana’, Latin, flat; with reference to the flattened body of the vegetative cells.
GenBank accession number: KT804914, the nuclear-encoded LSU rDNA gene sequences of the vegetative stage; KT804921, the internal transcribed spacer region (ITS) sequences of the vegetative stage.
The vegetative cells of Scrippsiella plana (strain SSFC13) were 19.0 (26.6) 34.5 µm long (SD = 3.5 µm, n = 50) and 15.0 (23.2) 31.0 µm wide (SD = 3.2 µm, n =50) and 10.0 (14.4) 17.0 μm (SD =2.0 µm, n = 50) deep. The vegetative cells of S. plana (strains SSFC13 and LH006) were tear-shaped in ventral view () and flattened in dorsoventral view (). There were numerous disc-like chloroplasts and as many as three pyrenoids with starch sheath were visible under LM (, ). The nucleus was subspherical and located in the central part of the cell (). The plate formula was Po, X, 4′, 3a, 7′′, 5C+t, 5S, 5′′′, 2′′′′ (–). The cingulum was displaced over about 2/3 of the girdle width. There were six cingular plates, among them, the first one was relatively narrow and considered as a transitional plate (t). There were two sutures in the dorsal side of the cingulum (). The first apical plate (1′) was relatively narrow and symmetrical (). The apical pore complex (Po) consisted of an apical pore plate and a rectangular canal plate (X) (). The pore plate was raised to form a prominent horn (). There were three anterior intercalary plates (1a, 2a and 3a) on the dorsal part of the epitheca. Plates 1a and 3a were pentagonal, whereas plate 2a was hexagonal (). Plates 2a was asymmetrical and shared similar size with 3a, which were almost twice the size of plate 1a (, ). The sulcus was composed of a hook-shaped anterior sulcal plate (Sa), a right sulcal plate (Sd), a left sulcal plate (Ss), a median sulcal plate (Sm) and a large posterior sulcal plate (Sp) that contacted the cingulum (). The two antapical plates were pentagonal and similar in size ().
Figs 5–10. Scrippsiella plana, SEM of vegetative cells (strain SSFC13). Fig. 5. Ventral view showing the first apical plate (1′) and other plates. Fig. 6. Dorsal view showing the anterior intercalary plates (1a, 2a and 3a). Fig. 7. Lateral view showing the flattening body. Fig. 8. Apical view showing the pore plate (arrow), canal plate (X), the apical plates (1′–4′), anterior intercalary plates (1a, 2a and 3a) and precingular plates (1′′–7′′). Fig. 9. Sulcal plates. Sa = anterior sulcal plate, Sd = right sulcal plate, Ss = left sulcal plate, Sm = median sulcal plate (shown by arrow), Sp = posterior sulcal plate. Fig. 10. Antapical view of a cell, showing five postcingular plates (1′′′–5′′′) and two antapical plates (1′′′′, 2′′′′). Scale bars: Figs 5–8, 10 = 5 µm; Fig. 9 = 2 µm.
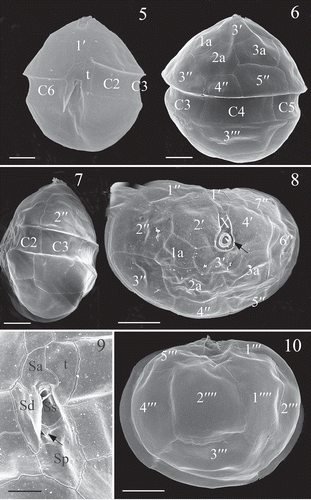
The cysts of Scrippsiella plana formed in culture were spherical with a diameter of 22.5 (23.8) 25.0 μm (SD = 0.8 µm, n = 15) and non-calcareous. They were filled with pale white granules and a bright red accumulation body was always present (). Some cysts were covered with a fine fibrous outer layer () but most of them were smooth (). The archeopyle was theropylic, a split along undetermined sutures. The plate pattern of S. plana is illustrated in –.
Figs 11–13. Scrippsiella plana, cysts produced in culture (strain SSFC13). Fig. 11. LM of living cyst showing a red body (R). Fig. 12. SEM of cyst showing fine filament ornamentation. Fig. 13. SEM of cyst showing smooth surface. Scale bars = 5 µm.
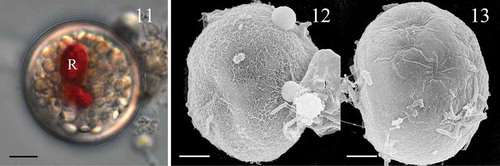
Figs 14–17. Interpreted tabulation of Scrippsiella plana. Fig. 14. Ventral view. Sa = anterior sulcal plate, Sd = right sulcal plate, Ss = left sulcal plate, Sm = median sulcal plate, Sp = posterior sulcal plate. Fig. 15. Dorsal view. Fig. 16. Apical view. Fig. 17. Antapical view.
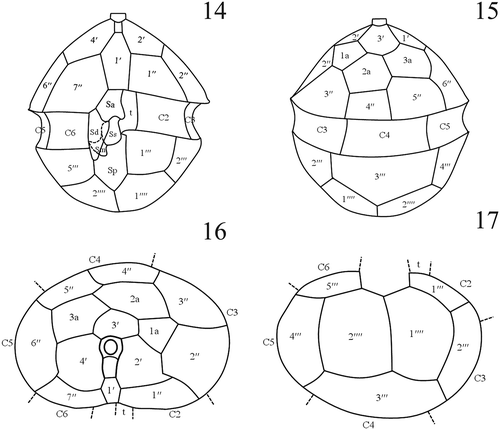
Scrippsiella spinifera (–)
The vegetative cells of Scrippsiella spinifera (strain SSHWI01 from Hawaii) were 34.8 (43.0) 52.7 µm long (SD = 4.3 µm, n = 50), 25.0 (29.2) 33.4 µm wide (SD = 2.3 µm, n = 50) and 24.3 (27.8) 33.2 µm (SD = 2.8 µm, n = 50) deep. Numerous bead-like chloroplasts were located in the periphery of the cell (). There was a large and elongated nucleus (). The epitheca was conical and slightly larger than the trapezoidal hypotheca (, ). A deep incised sulcus divided the antapical region of the hypotheca into two distinct lobes, and each lobe had two spines at its terminal part (). The plate formula was Po, X, 4′, 3a, 7′′, 5C+t, 5S, 5′′′, 2′′′′. The canal plate was about one half the length of plates 2′ and 4′ (). Plate 1′ was very narrow and four-sided (, ). There were three anterior intercalary plates of similar size. Plates la and 3a were pentagonal whereas plate 2a was hexagonal (, ); the cingulum was displaced over about half of the girdle width. The two antapical plates were pentagonal and similar in size (). The sulcus comprised five sulcal plates. The anterior sulcal plate (Sa) was hook shaped and the posterior sulcal plate (Sp) was the largest and contacted the cingulum (). There were numerous pores on the thecal surface surrounded by one or two concentric ridges (). The germinated cells from Izmir Bay, Turkey, were similar to those from Hawaii.
Figs 18–29. Scrippsiella spinifera, vegetative cells and cysts of LM and SEM (all from strain SSHWI01 except Fig. 29 refers to a cyst from Turkish sediments). Fig. 18. LM, ventral view showing cingulum displacement. Fig. 19. LM, A SYBR Green stained cell in ventral view, showing the large and elongated nucleus (N). Fig. 20. SEM, ventral view showing the conical epitheca and the trapezoidal hypotheca. Fig. 21. SEM, lateral view showing the antapical spines (arrow). Fig. 22. SEM, apical-ventral view showing the narrow and symmetrical first apical plate (1′). Fig. 23. SEM, apical view showing the apical plates (1′–4′), anterior intercalary plates (1a, 2a and 3a) and precingular plates (1′′–7′′). Fig. 24. SEM, dorsal view showing the hexagonal 2a plate. Fig. 25. SEM, antapical view, showing five postcingular plates (1′′′–5′′′) and two antapical plates (1′′′′, 2′′′′). Fig. 26. SEM of sulcal plates. Sa = anterior sulcal plate, Sd = right sulcal plate, Ss = left sulcal plate, Sm = median sulcal plate (shown by arrow), Sp = posterior sulcal plate. Figs 27, 28. LM, living cysts generated in culture showing spines with various length. Fig. 29. LM, an empty cyst from Turkish sediments. Scale bars = 5 µm.
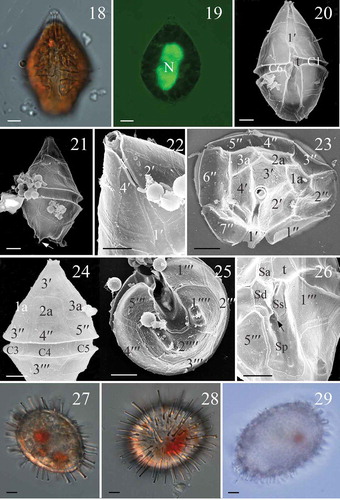
The cysts of Scrippsiella spinifera (strain SSHWI01 from Hawaii) were ellipsoid, light brown, and had one or two prominent red bodies. The cysts were 31.5 (34.9) 40.0 µm long (SD = 3.2 µm, n = 15) and 25.7 (26.7) 31.8 µm (SD = 2.1 µm, n = 15) wide, and they were covered with calcareous spines 3.1–7.1 µm long with capitate ends (, ). There are 5–6 processes per 10 × 10 µm2 (n = 3).
The cyst of Scrippsiella spinifera from Izmir Bay, Turkey was 43.0 µm long and 31.8 µm wide, with calcareous spines 2.7 µm long ().
GenBank accession number: KT804915, the nuclear-encoded LSU rDNA gene sequences of the vegetative stage; KT804919, the internal transcribed spacer region (ITS) sequences of the vegetative stage.
Cyst of ‘Peridinium’ wisconsinense
The cysts of ‘Peridinium’ wisconsinense were large, cavate and proximate with a smooth, slightly ellipsoidal transparent inner layer and a transparent ornamented outer layer appressed to the inner layer in the equatorial region. The outer layer was slightly invaginated in the sulcal area, forming two light shoulders and formed a rounded, sometimes bifurcated apical horn and a single, sharply pointed antapical horn (Fig. S1). Paratabulation was reflected by concentrations of ornamentation and by the archeopyle that was composed of the apical plates 2′, 3′ and 4′ and part of the first apical plate (1′).
Molecular phylogenetic analysis
Scrippsiella plana strains SSFC13 and LH006 from the South China Sea and Caspian Sea shared identical ITS and LSU sequences, and S. spinifera from the Turkish coast and Hawaiian coast (strain SSHWI01) shared identical ITS and LSU sequences too. Scrippsiella spinifera differed from Duboscquodinium collinii Grassé (HM483398, a parasite of Eutintinnus fraknoii Daday) only at 2 and 2 positions (ITS and LSU) and differed from S. cf. spinifera strain SSFC03 at 33 and 18 positions (ITS and LSU, 94.01% and 97.13% similarity). ‘Peridinium’ wisconsinense differed from Chimonodinium lomnickii at 34 positions (LSU, 97.13% similarity).
Maximum likelihood (ML) and Bayesian analyses based on LSU sequences generated two similar trees that differed at only a few topologies. The molecular tree revealed four well resolved clades, i.e. E/Pe (Ensiculifera Balech and Pentapharsodinium Indelicato & A.R.Loebl.), Peridinium Ehrenberg sensu stricto, Scrippsiella sensu lato, and T/Pf (Thoracosphaera Kamptner, Pfiesteria K.A.Steidinger & J.M.Burkholder and related species). The clade of Scrippsiella sensu lato received maximal support (Bayesian posterior probability = 1.0, ML bootstrap support = 100) and comprised mainly Scrippsiella species, but also those of genera Naiadinium, Duboscquodinium, Calciodinellum, Theleodinium, Pernambugia and Tintinnophagus D.W.Coats. Three subclades could be identified including CAL (Calciodinellum and related species), STR (Scrippsiella trochoidea and related species) and PRE (Scrippsiella precaria and related species) with moderate (0.93/100; 0.84/100) to maximal support. Scrippsiella plana was nested within the CAL subclade with moderate support (0.93/100). Scrippsiella spinifera and Duboscquodinium collinii formed a SPI group with strong support (0.96/100) and was nested within the STR subclade. The T/Pf clade also received maximal support and consisted of subclades CHI (Chimonodinium lomnickii (Wołoszyńska) Craveiro, Calado, Daugbjerg, Gert Hansen & Moestrup and related species) and ACI (‘Peridinium’ aciculiferum Lemmermann and related species) with moderate (0.92/100) to maximal support. ‘Peridinium’ wisconsinense was closest to C. lomnickii ().
Fig. 30. Phylogenetic tree inferred from partial LSU rDNA sequences based on maximum likelihood (ML). Prorocentrum cordatum was used as outgroup. Numbers on branches are statistical support values (Bayesian posterior probability/ MLbootstrap support). Bootstrap values >50% and posterior probabilities of 0.9 or above are shown. * indicates maximal support (Bayesian posterior probability = 1.0, MLbootstrap support = 100%). New sequences obtained in this study were indicated in bold format. E/Pe = clade of Ensiculifera and Pentapharsodinium; T/Pf = clade of Thoracosphaera, Pfiesteria and related species; STR = subclade of Scrippsiella trochoidea and its relatives (SPI= group of S. spinifera and related species); CAL = subclade of Calciodinellum and its relatives; PRE = subclade of Scrippsiella precaria and its relatives. Scale bar = number of nucleotide substitutions per site. Those taxa in brackish/freshwater environments are highlighted in grey, those producing non-calcified immotile cells are marked with ☆ and those with a non-symmetric epitheca are marked with △.
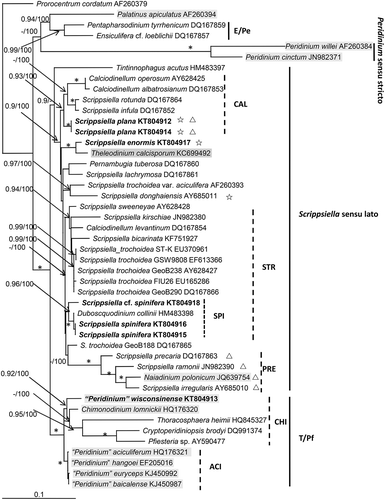
Maximum likelihood (ML) and Bayesian analyses based on ITS sequences generated two similar trees that differed at only a few topologies. Scrippsiella sensu lato received maximal support and consisted of four well resolved clades in the molecular tree, i.e. CAL clade (comprising species of Calciodinellum and some Scrippsiella spp.; receiving moderate support, 0.93/100), PRE clade (comprising Naiadinium polonicum (Wołoszyńska) S. Carty, S. precaria and related species; receiving maximal support), LAC clade (comprising S. lachrymosa and related species; receiving maximal support), STR clade (comprising S. trochoidea and related species; receiving low support, 0.79/100). ‘Peridinium’ aciculiferum and ‘Peridinium’ hangoei diverged earlier than the four clades. Scrippsiella plana was clearly nested within the CAL clade but its closest relatives could not be identified. Scrippsiella spinifera and Duboscquodinium collinii formed a SPI subclade with maximal support and was nested within the STR clade ().
Fig. 31. Phylogenetic tree inferred from ITS and 5.8S rDNA sequences based on maximum likelihood (ML). Ensiculifera cf. imariensis was used as outgroup. Numbers on branches are statistical support values (ML bootstrap support/Bayesian posterior probability). Bootstrap values >50% and posterior probabilities of 0.9 or above are shown. * indicates maximal support (Bayesian posterior probability = 1.0, MLbootstrap support = 100%). New sequences obtained in this study were indicated in bold format. PRE = clade of Scrippsiella precaria and its relatives; STR = clade of Scrippsiella trochoidea and its relatives (SPI = subclade of S. spinifera and related species; subclades STR1, 2, 3); CAL = clade of Calciodinellum and its relatives; LAC = clade of Scrippsiella lachrymosa and its relatives. Scale bar = number of nucleotide substitutions per site. The branch length of those dashed lines was reduced to half size. Those taxa in brackish/freshwater environments are highlighted in grey, those producing non-calcified immotile cells are marked with ☆ and those with a non-symmetric epitheca are marked with △.
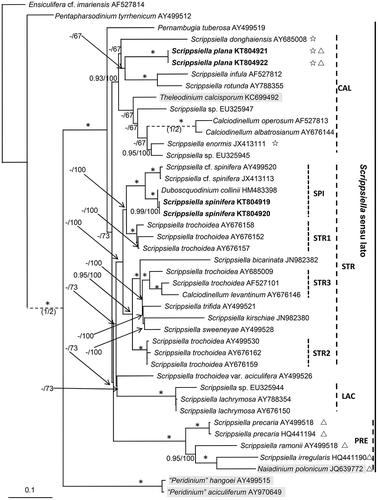
Growth
Scrippsiella plana strains SSFC13 and LH006 exhibited growth at salinities between 2 and 28. For strain SSFC13, exponential division rates were similar at salinities from 6 to 28 (> 0.5 divisions d–1) and much higher than those at salinities of 4 and 2, respectively (). For strain LH006, the highest division rate was 0.74 divisions d–1 at salinity of 12, and then dropped slowly towards higher and lower salinities ().
DISCUSSION
Comparison of Scrippsiella plana and S. spinifera to other Scrippsiella species based on motile stage
Most Scrippsiella species demonstrate a rather conserved thecate morphology, e.g. S. rotunda Lewis and Calciodinellum operosum Deflandre cannot be differentiated using their thecate stage, only using the cyst morphology (Montresor et al., Citation1997). However, here we report two Scrippsiella species with a distinct thecate morphology. Scrippsiella plana is characterized by a dorsoventrally flattened body and a non-symmetric epitheca. To date, only one Scrippsiella species (S. saladensis) was described to possess a dorsoventrally flattened body, but differs from S. plana in that S. saladensis has a symmetric epitheca, a different number of precingular plates (predominantly 6, versus 7 in S. plana) and a Sp plate that does not contact the cingulum (Balech, Citation1963). The vegetative cells of S. saladensis are also much larger than those of S. plana in size (30–47 μm long, 24–41 μm wide versus 19–35 μm long and 15–31 μm wide). Moreover, a dorsoventrally flattened body was recorded only in a few thecate species belonging to the order Peridiniales (e.g. Peridiniopsis niei G. Liu & Z. Hu; Liu et al., Citation2008, Protoperidinium compressum (Abé) Balech, Peridinium quinquecorne Abé; Abé, Citation1981, Naiadinium polonicum Craveiro et al., Citation2015). Based on its unusual thecate morphology, S. plana was described as a new species.
Our Scrippsiella spinifera was characterized by a trapezoidal hypotheca with short spines at the antapical side and numerous trichocyst pores surrounded by concentric ridges, thus fitting the original description (Honsell & Cabrini, Citation1991). Scrippsiella cf. spinifera cultures from the South China Sea also show such concentric ridges surrounding the trichocyst pores (Gu et al., Citation2013), as well as S. spinifera reported from Narragansett Bay, USA (Zhang, Citation1997). Scrippsiella spinifera from western Japan however, does not show concentric ridges surrounding the trichocyst pores (Kobayashi, Citation1995), suggesting that this feature might be variable at intraspecific level.
A non-symmetric epitheca within the genus Scrippsiella is a homoplasy
Apparently Scrippsiella plana shares a non-symmetric epitheca with S. precaria, S. ramonii and S. irregularis, but they are phylogenetically distant, indicating that a non-symmetric epitheca has been acquired multiple times independently. Scrippsiella precaria, S. ramonii and S. irregularis share a small 2a plate with 1a in contact with 3a (Montresor & Zingone, Citation1988; Montresor, Citation1995; Attaran-Fariman & Bolch, Citation2007). These species form the PRE clade in the phylogenetic tree (Attaran-Fariman & Bolch, Citation2007; , in the present study) suggesting that these character states were acquired only once. In fact, the way S. plana achieves non-symmetry differs from that observed in the three species mentioned above, in that S. plana has a small 1a plate (half the size of plate 3a). It is also worth noting that Naiadinium polonicum, a freshwater species with two anterior intercalary plates of unequal size (or only one anterior intercalary plate) also belongs to the PRE clade (Logares et al., Citation2007; Craveiro et al., Citation2015; present study). All species in the clade share an identical plate pattern except the number and arrangement of anterior intercalary plates. The PRE clade might be transferred into Naiadinium later when more phylogenetic information, especially from the type species of Scrippsiella, Scrippsiella sweeneyae, is available.
Molecular phylogeny demonstrates that S. spinifera falls within the STR clade together with the putative S. sweeneyae (strain CCM 280, origin unknown), suggesting that this clade might represent the true Scrippsiella. All species in this clade show a symmetric epitheca and produce calcareous or smooth cysts, if they have a cyst stage.
The phylogenetic importance of the cyst stages of Scrippsiella plana and S. spinifera
Our molecular phylogeny based on ITS and LSU sequences are generally consistent, conform to those previously reported (e.g. Gottschling et al., Citation2005, Citation2012). Scrippsiella plana, which produces non-calcareous cysts, is clearly nested within the CAL clade, which consists of two subclades, each of which includes species producing both smooth calcareous and non-calcareous cysts (). A single Scrippsiella species (S. trochoidea) can produce both calcareous cysts and organic-walled cysts (Wang et al., Citation2007), thus we cannot exclude the possibility that S. plana also can produce calcareous cysts, although no calcareous cysts were observed in our cultures. Moreover, calcareous cysts can be misidentified as other organic cysts after losing calcareous layers (Head et al., Citation2006). The inclusion of the freshwater species Theleodinium calcisporum Craveiro, Pandeirada, Daugbjerg, Moestrup & Calado within the CAL clade is consistent with the fact that it also produces smooth calcareous cysts (Craveiro et al., Citation2013), however, it has three apical plates and two anterior intercalary plates (Craveiro et al., Citation2013), unlike other species in the CAL clade. The discovery of these non-calcareous cysts in S. plana emphasizes the variability of cyst wall composition within the genus Scrippsiella.
Cysts of S. spinifera were first described from western Japan as oval, however, the concentric ridges surrounding the trichocyst pores were not observed on the thecate stage of these Japanese specimens (Kobayashi, Citation1995). S. cf. spinifera cultures from the South China Sea formed oval cysts as well, and they show concentric ridges surrounding the trichocyst pores (Gu et al., Citation2013). Oval cysts of S. spinifera were also reported from Narragansett Bay, USA and the corresponding cells have trichocyst pores surrounded by concentric ridges (Zhang, Citation1997). Here we report ellipsoid cysts of S. spinifera for the first time, whose spine length can vary greatly, as also reported in oval cysts assigned respectively to S. spinifera and S. cf. spinifera (Zhang, Citation1997; Gu et al., Citation2013). Cyst morphology is a key character to differentiate Scrippsiella species with identical theca morphology (Montresor et al., Citation1997; Elbrächter et al., Citation2008; Gu et al., Citation2008), and our results revealed two clades within the S. spinifera species complex corresponding to oval cysts (S. cf. spinifera) and ellipsoid cysts (S. spinifera) for the first time. We suspect the strains studied here are true S. spinifera since the specimens from Izmir Bay on the Turkish coast are closer to the type locality (Gulf of Trieste, Northern Adriatic Sea, Italy; Honsell & Cabrini, Citation1991), and the strains here designated as Scrippsiella cf. spinifera, producing an oval cyst from the South China Sea (Gu et al., Citation2013) might represent a new species.
Distribution of Scrippsiella plana, S. spinifera and the importance of phenotypic plasticity in S. plana
Both Scrippsiella species were recovered from geographically distant localities, suggesting that each are not endemic and are widely distributed, although probably restricted to warmer waters. In addition, S. plana displays a wide tolerance to salinity. Scrippsiella plana from the South China Sea showed phenotypic plasticity in growth rate, which can be explained by the salinity fluctuation (8–26) at the mouth of Beilun River (Lan, Citation2012), where the strain was isolated. This is consistent with the idea that plasticity can evolve in fine-grained conditions, characterized by habitats with varying environmental factors (Levins, Citation1968). Scrippsiella plana from the Caspian Sea showed phenotypic plasticity too, but it also displayed local adaptation, i.e. the maximum growth rate at the salinity of 12. Similar adaptation was also found in strains of the cold water species Polarella glacialis (Rengefors et al., Citation2015).
A possible relation of Scrippsiella spinifera to the parasite Duboscquodinium collinii?
The high similarity of ITS sequence between Scrippsiella spinifera and Duboscquodinium collinii support a close relationship between them. ITS region was used as a genetic marker to differentiate various dinoflagellate species (Litaker et al., Citation2007; Stern et al., Citation2012), but our results emphasize that caution is needed. The life cycle transitions of D. collinii, especially the plate pattern in the dinospore (vegetative) stage is still not clear (Coats et al., Citation2010) and will be helpful to understand its systematic position. A symbiont of Acanthochiasma sp. (Radiolaria) was identified as Scrippsiella sp. based on molecular phylogeny, which is closely related with true S. acuminata (Decelle et al., Citation2012). The genus Duboscquodinium currently incorporates only D. collinii and D. kofoidii Grassé and was classified within the family Duboscquellaceae together with Duboscquella Chatton (Fensome et al., Citation1993), but was not supported by molecular phylogeny based on SSU rDNA sequences (Coats et al., Citation2010). Coats et al. (Citation2010) showed the close relationship between Duboscquodinium and species of Scrippsiella (i.e. a member of the Thoracosphaeraceae). Since Duboscquodinium has priority over Scrippsiella, whether the latter is a junior synonym of the former, is an interesting topic for future study.
Molecular phylogeny of ‘Peridinium’ wisconsinense and implications for multiple transitions to freshwater
In the molecular phylogeny based on LSU sequences, ‘Peridinium’ wisconsinense, ‘P’. aciculiferum, ‘P’. euryceps and ‘P’. baicalense were classified within the T/Pf clade, separated from the Peridinium sensu stricto clade. ‘Peridinium’ aciculiferum and ‘P’. euryceps differ from the type species of Peridinium (Peridinium cinctum (O.F.Müller) Ehrenberg) in the number of cingular plates (six versus five) (Rengefors & Meyer, Citation1998; Logares et al., Citation2007), suggesting that they are not true Peridinium. ‘Peridinium’ wisconsinense will possibly have to be reassigned to Chimonodinium, pending further detailed investigation of the cingular and sulcal plates and ultrastructural features, which have not been described yet.
The scattering of freshwater species in various clades suggested by the LSU sequences implies multiple transitions of the Peridiniales to freshwater. The report of cysts from the non-marine Tertiary of Alaska as Geiselodinium tyonekensis sp. nov. by Engelhardt (Citation1976) that are nearly identical to those shown to form by the large, distinctive dinoflagellate ‘Peridinium’ wisconsinense in culture (Wall & Dale, Citation1968; McCarthy et al., Citation2011) may help to constrain the timing of this divergence to some time prior to the Miocene. Adaptation to freshwater environments in the lineage of T/Pf clade appear to have occurred independently of the lineage that includes most other species of the genus Peridinium, including the type species P. cinctum and other common species (e.g. P. willei Huitfeldt-Kaas). At least two separate marine–freshwater transitions thus appear to be recorded in the dinoflagellate cyst record.
Supplementary information
The following supplementary material is accessible via the Supplementary Content tab on the article’s online page at http://dx.doi.org/10.1080/09670262.2015.1120348
Supplementary Fig. S1. A living cyst of “Peridinium” wisconsinense showing the rounded apical horn and sharply pointed antapical horn.
Acknowledgements
We thank two anonymous reviewers for constuctive suggestions that improved the ms greatly. We thank Serdar Uzar for his help in collecting samples from İzmir Bay. Anne de Vernal and Geneviève Vautour are thanked for obtaining samples from Hawaii. Anne de Vernal and Maryse Henry are thanked for use of the facilities at GEOTOP.
Disclosure statement
No potential conflict of interest was reported by the author(s).
Additional information
Funding
Notes on contributors
Zhaohe Luo
Z. Luo: cyst-theca experiment, microscopy, drafting and editing manuscript; K. N. Mertens: cyst-theca experiment, microscopy, drafting and editing manuscript; S. Bagheri: sampling, drafting and editing manuscript; H. Aydin: sampling, editing manuscript; Y. Takano: sequencing, analysis of molecular data, editing manuscript; K. Matsuoka: editing manuscript; F. M.G. McCarthy: sampling, editing manuscript; H. Gu: original concept, drafting and editing manuscript.
Kenneth Neil Mertens
Z. Luo: cyst-theca experiment, microscopy, drafting and editing manuscript; K. N. Mertens: cyst-theca experiment, microscopy, drafting and editing manuscript; S. Bagheri: sampling, drafting and editing manuscript; H. Aydin: sampling, editing manuscript; Y. Takano: sequencing, analysis of molecular data, editing manuscript; K. Matsuoka: editing manuscript; F. M.G. McCarthy: sampling, editing manuscript; H. Gu: original concept, drafting and editing manuscript.
Siamak Bagheri
Z. Luo: cyst-theca experiment, microscopy, drafting and editing manuscript; K. N. Mertens: cyst-theca experiment, microscopy, drafting and editing manuscript; S. Bagheri: sampling, drafting and editing manuscript; H. Aydin: sampling, editing manuscript; Y. Takano: sequencing, analysis of molecular data, editing manuscript; K. Matsuoka: editing manuscript; F. M.G. McCarthy: sampling, editing manuscript; H. Gu: original concept, drafting and editing manuscript.
Hilal Aydin
Z. Luo: cyst-theca experiment, microscopy, drafting and editing manuscript; K. N. Mertens: cyst-theca experiment, microscopy, drafting and editing manuscript; S. Bagheri: sampling, drafting and editing manuscript; H. Aydin: sampling, editing manuscript; Y. Takano: sequencing, analysis of molecular data, editing manuscript; K. Matsuoka: editing manuscript; F. M.G. McCarthy: sampling, editing manuscript; H. Gu: original concept, drafting and editing manuscript.
Yoshihito Takano
Z. Luo: cyst-theca experiment, microscopy, drafting and editing manuscript; K. N. Mertens: cyst-theca experiment, microscopy, drafting and editing manuscript; S. Bagheri: sampling, drafting and editing manuscript; H. Aydin: sampling, editing manuscript; Y. Takano: sequencing, analysis of molecular data, editing manuscript; K. Matsuoka: editing manuscript; F. M.G. McCarthy: sampling, editing manuscript; H. Gu: original concept, drafting and editing manuscript.
Kazumi Matsuoka
Z. Luo: cyst-theca experiment, microscopy, drafting and editing manuscript; K. N. Mertens: cyst-theca experiment, microscopy, drafting and editing manuscript; S. Bagheri: sampling, drafting and editing manuscript; H. Aydin: sampling, editing manuscript; Y. Takano: sequencing, analysis of molecular data, editing manuscript; K. Matsuoka: editing manuscript; F. M.G. McCarthy: sampling, editing manuscript; H. Gu: original concept, drafting and editing manuscript.
Francine M.G. McCarthy
Z. Luo: cyst-theca experiment, microscopy, drafting and editing manuscript; K. N. Mertens: cyst-theca experiment, microscopy, drafting and editing manuscript; S. Bagheri: sampling, drafting and editing manuscript; H. Aydin: sampling, editing manuscript; Y. Takano: sequencing, analysis of molecular data, editing manuscript; K. Matsuoka: editing manuscript; F. M.G. McCarthy: sampling, editing manuscript; H. Gu: original concept, drafting and editing manuscript.
Haifeng Gu
Z. Luo: cyst-theca experiment, microscopy, drafting and editing manuscript; K. N. Mertens: cyst-theca experiment, microscopy, drafting and editing manuscript; S. Bagheri: sampling, drafting and editing manuscript; H. Aydin: sampling, editing manuscript; Y. Takano: sequencing, analysis of molecular data, editing manuscript; K. Matsuoka: editing manuscript; F. M.G. McCarthy: sampling, editing manuscript; H. Gu: original concept, drafting and editing manuscript.
References
- Abé, T. (1981). Studies on the family Peridiniales. Seto Marine Biological Laboratory, Special publication Series, 6: 1–409.
- Adachi, M., Sako, Y. & Ishida, Y. (1996). Analysis of Alexandrium (Dinophyceae) species using sequences of the 5.8S ribosomal DNA and internal transcribed spacer regions. Journal of Phycology, 32: 424–432.
- Attaran-Fariman, G. & Bolch, C.J.S. (2007). Scrippsiella irregularis sp. nov. (Dinophyceae), a new dinoflagellate from the southeast coast of Iran. Phycologia, 46: 572–582.
- Balech, E. (1959). Two new genera of dinoflagellates from California. Biological Bulletin, 116: 195–203.
- Balech, E. (1963). Dos dinoflagelados de una laguna salobre de la Argentina. Notas del Museo, Universidad Nacional de la Plata, 20: 111–123.
- Balech, E. (1980). On the thecal morphology of dinoflagellates with special emphasis on circular and sulcal plates. Anales del Centro de Ciencias del Mar y Limnologia, Universidad Nacional Autonomia de Mexico, 7: 57–68.
- Boc, A., Diallo, A.B. & Makarenkov, V. (2012). T-REX: a web server for inferring, validating and visualizing phylogenetic trees and networks. Nucleic Acids Research, 40: W573–W579.
- Coats, D.W., Kim, S., Bachvaroff, T.R., Handy, S.M. & Delwiche, C.F. (2010). Tintinnophagus acutus n.g., n. sp. (Phylum Dinoflagellata), an ectoparasite of the ciliate Tintinnopsis cylindrica Daday 1887, and its relationship to Duboscquodinium collini Grassé 1952. Journal of Eukaryotic Microbiology, 57: 468–482.
- Craveiro, S.C., Calado, A.J., Daugbjerg, N., Hansen, G. & Moestrup, Ø. (2011). Ultrastructure and LSU rDNA-based phylogeny of Peridinium lomnickii and description of Chimonodinium gen. nov. (Dinophyceae). Protist 162: 590–615.
- Craveiro, S.C., Pandeirada, M.S., Daugbjerg, N., Moestrup, Ø. & Calado, A.J. (2013). Ultrastructure and phylogeny of Theleodinium calcisporum gen. et sp. nov., a freshwater dinoflagellate that produces calcareous cysts. Phycologia, 52: 488–507.
- Craveiro, S.C., Daugbjerg, N., Moestrup, Ø. & Calado, A.J. (2015). Fine-structural characterization and phylogeny of Peridinium polonicum, type species of the recently described genus Naiadinium (Dinophyceae). European Journal of Protistology, 51: 259–279.
- Daugbjerg, N., Hansen, G., Larsen, J. & Moestrup, Ø. (2000). Phylogeny of some of the major genera of dinoflagellates based on ultrastructure and partial LSU rDNA sequence data, including the erection of three new genera of unarmoured dinoflagellates. Phycologia, 39: 302–317.
- D’Onofrio, G., Marino, D., Bianco, L., Busico, E. & Montresor, M. (1999). Toward an assessment on the taxonomy of dinoflagellates that produce calcareous cysts (Calciodinelloideae, Dinophyceae): a morphological and molecular approach. Journal of Phycology, 35: 1063–1078.
- Decelle, J., Siano, R., Probert, I., Poirier, C. & Not, F. (2012). Multiple microalgal partners in symbiosis with the acantharian Acanthochiasma sp. (Radiolaria). Symbiosis, 58: 233–244.
- Elbrächter, M., Gottschling, M., Hildebrand-Habel, T., Keupp, H., Kohring, R., Lewis, J., Meier, S.K.J., Montresor, M., Streng, M. & Versteegh, G.J.M. (2008). Establishing an agenda for calcareous dinoflagellate research (Thoracosphaeraceae, Dinophyceae) including a nomenclatural synopsis of generic names. Taxon, 57: 1289–1303.
- Engelhardt, D.W. (1976). Geiselodinium tyonekensis sp. nov., a dinoflagellate cyst from the nonmarine Tertiary (Miocene) of Alaska. Geoscience and Man, XV: 121–124.
- Fensome, R.A., Taylor, F.J.R., Norris, G., Sarjeant, W.A.S., Wharton, D.I. & Williams, G.L. (1993). A classification of fossil and living dinoflagellates. Micropaleontology Special Publication, 7: 1–245.
- Gottschling, M., Knop, R., Plötner, J., Kirsch, M., Willems, H. & Keupp, H. (2005). A molecular phylogeny of Scrippsiella sensu lato (Calciodinellaceae, Dinophyta) with interpretations on morphology and distribution. European Journal of Phycology, 40: 207–220.
- Gottschling, M., Soehner, S., Zinssmeister, C., John, U., Plötner, J., Schweikert, M., Aligizaki, K. & Elbrächter, M. (2012). Delimitation of the Thoracosphaeraceae (Dinophyceae), including the calcareous dinoflagellates, based on large amounts of ribosomal RNA sequence data. Protist 163: 15–24.
- Gu, H., Sun, J., Kooistra, W.H.C.F. & Zeng, R. (2008). Phylogenetic position and morphology of thecae and cysts of Scrippsiella (Dinophyceae) species in the East China Sea. Journal of Phycology, 44: 478–494.
- Gu, H., Luo, Z., Liu, T. & Lan, D. (2013). Morphology and phylogeny of Scrippsiella enormis sp. nov. and S. cf. spinifera (Peridiniales, Dinophyceae) from the China Sea. Phycologia, 52: 182–190.
- Guillard, R.R.L. & Ryther, J.H. (1962). Studies of marine planktonic diatoms. I. Cyclotella nana Hustedt and Detonula confervacea Cleve. Canadian Journal of Microbiology, 8: 229–239.
- Hall, T.A. (1999). BioEdit: a user-friendly biological sequence alignment editor and analysis program for Windows 95/98/NT. pp. 95–98.
- Head, M.J., Lewis, J. & De Vernal, A. (2006). The cyst of the calcareous dinoflagellate Scrippsiella trifida: resolving the fossil record of its organic wall with that of Alexandrium tamarense. Journal of Paleontology, 80: 1–18.
- Honsell, G. & Cabrini, M. (1991). Scrippsiella spinifera sp. nov. (Pyrrhophyta): a new dinoflagellate from the northern Adriatic Sea. Botanica Marina, 34: 167–175.
- Hu, S., Liu, G.X., Zhou, G.J., Mei, H. & Hu, Z.Y. (2008). Peridinium polonicum, a new record of freshwater toxic dinoflagellate from China. Journal of Wuhan Botanical Research, 26: 454–457 ( in Chinese with English Abstract).
- Indelicato, S. & Loeblich III, A. (1985). A description of the marine dinoflagellate, Scrippsiella tinctoria sp. nov. Japan Journal of Phycology, 33: 127–134.
- Janofske, D. (2000). Scrippsiella trochoidea and Scrippsiella regalis, nov. comb. (Peridiniales, Dinophyceae): a comparison. Journal of Phycology, 36: 178–189.
- Katoh, K., Kuma, K., Toh, H. & Miyata, T. (2005). MAFFT version 5: improvement in accuracy of multiple sequence alignment. Nucleic Acids Research, 33: 511–518.
- Kobayashi, S. (1995). First record of cysts of Scrippsiella spinifera Honsell & Cabrini (Dinophyceae). Bulletin of Plankton Society of Japan, 42: 75–79.
- Kretschmann, J., Zinssmeister, C. & Gottschling, M. (2014). Taxonomic clarification of the dinophyte Rhabdosphaera erinaceus Kamptner, ≡ Scrippsiella erinaceus comb. nov. (Thoracosphaeraceae, Peridiniales). Systematics and Biodiversity, 12: 393–404.
- Kretschmann, J., Elbrächter, M., Zinssmeister, C., Soehner, S., Kirsch, M., Kusber, W.-H. & Gottschling, M. (2015). Taxonomic clarification of the dinophyte Peridinium acuminatum Ehrenb.,≡ Scrippsiella acuminata, comb. nov. (Thoracosphaeraceae, Peridiniales). Phytotaxa, 220: 239–256.
- Lan, D. (2012). Current status of marine resources and environment at the mouth of Beilun River. Xiamen University Press, Xiamen, China, 249 pp.
- Levins, R. (1968). Evolution in changing environments: some theoretical explorations. Princeton University Press, Princeton, NJ. 132 pp.
- Larsen, J., Kuosa, H., Ikävalko, J., Kivi, K. & Hällfors, S. (1995). A redescription of Scrippsiella hangoei (Schiller) comb. nov. – a ‘red tide’ dinoflagellate from the northern Baltic. Phycologia, 34: 135–144.
- Lewis, J. (1991). Cyst-theca relationships in Scrippsiella (Dinophyceae) and related orthoperidinioid genera. Botanica Marina, 34: 91–106.
- Litaker, W.R., Vandersea, M.W., Kibler, S.R., Reece, K.S., Stokes, N.A., Lutzoni, F.M., Yonish, B.A., West, M.A., Black, M.N.D. & Tester, P.A. (2007). Recognizing dinoflagellate species using ITS rDNA sequences. Journal of Phycology, 43: 344–355.
- Liu, G.X., Pei, G.F. & Hu, Z.Y. (2008). Peridiniopsis niei sp. nov. (Dinophyceae), a new species of freshwater red tide dinoflagellates from China. Nova Hedwigia, 87: 487–499.
- Logares, R., Rengefors, K., Kremp, A., Shalchian-Tabrizi, K., Boltovskoy, A., Tengs, T., Shurtleff, A. & Klaveness, D. (2007). Phenotypically different microalgal morphospecies with identical ribosomal RNA: a case of rapid adaptive evolution? Microbial Ecology, 53: 549–561.
- McCarthy, F.M.G., Mertens, K.N., Ellegaard, M., Sherman, K., Pospelova, V., Ribeiro, S., Blasco, S. & Vercauteren, D. (2011). Resting cysts of freshwater dinoflagellates in southeastern Georgian Bay (Lake Huron) as proxies of cultural eutrophication. Review of Palaeobotany and Palynology, 166: 46–62.
- Meier, K., Janofske, D. & Willems, H. (2002). New calcareous dinoflagellates (Calciodinelloideae) from the Mediterranean Sea. Journal of Phycology, 38: 602–615.
- Montresor, M. (1995). Scrippsiella ramonii sp. nov. (Peridiniales, Dinophyceae), a marine dinoflagellate producing a calcareous resting cyst. Phycologia, 34: 87–91.
- Montresor, M. & Zingone, A. (1988). Scrippsiella precaria sp. nov. (Dinophyceae), a marine dinoflagellate from the Gulf of Naples. Phycologia, 27: 387–394.
- Montresor, M., Janofske, D. & Willems, H. (1997). The cyst-theca relationship in Calciodinellum operosum emend. (Peridiniales, Dinophyceae) and new approach for the study of calcareous cysts. Journal of Phycology, 33: 122–131.
- Montresor, M., Sgrosso, S., Procaccini, G. & Kooistra, W.H.C.F. (2003). Intraspecific diversity in Scrippsiella trochoidea (Dinopbyceae): evidence for cryptic species. Phycologia, 42: 56–70.
- Posada, D. (2008). jModelTest: phylogenetic model averaging. Molecular Biology and Evolution, 25: 1253–1256.
- Rengefors, K. & Meyer, B. (1998). Peridinium euryceps sp. nov. (Peridiniales, Dinophyceae), a cryophilic dinoflagellate from Lake Erken, Sweden. Phycologia, 37: 284–291.
- Rengefors, K., Logares, R., Laybourn-Parry, J. & Gast, R.J. (2015). Evidence of concurrent local adaptation and high phenotypic plasticity in a polar microeukaryote. Environmental Microbiology, 17: 1510–1519.
- Ronquist, F. & Huelsenbeck, J.P. (2003). MrBayes 3: Bayesian phylogenetic inference under mixed models. Bioinformatics, 19: 1572–1574.
- Scholin, C.A., Herzog, M., Sogin, M. & Anderson, D.M. (1994). Identification of group- and strain-specific genetic markers for globally distributed Alexandrium (Dinophyceae). II. sequence analysis of a fragment of the LSU rRNA gene. Journal of Phycology, 30: 999–1011.
- Soehner, S., Zinssmeister, C., Kirsch, M. & Gottschling, M. (2012). Who am I – and if so, how many? Species diversity of calcareous dinophytes (Thoracosphaeraceae, Peridiniales) in the Mediterranean Sea. Organisms Diversity and Evolution, 12: 339–348.
- Stamatakis, A. (2006). RAxML-VI-HPC: maximum likelihood-based phylogenetic analyses with thousands of taxa and mixed models. Bioinformatics, 22: 2688–2690.
- Stern, R.F., Andersen, R.A., Jameson, I., Küpper, F.C., Coffroth, M.-A., Vaulot, D., Le Gall, F., Véron, B., Brand, J.J. & Skelton, H. (2012). Evaluating the ribosomal internal transcribed spacer (ITS) as a candidate dinoflagellate barcode marker. PLoS ONE, 7: e42780.
- Takano, Y. & Horiguchi, T. (2006). Acquiring scanning electron microscopical, light microscopical and multiple gene sequence data from a single dinoflagellate cell. Journal of Phycology, 42: 251–256.
- Wall, D. & Dale, B. (1968). Modern dinoflagellate cysts and evolution of the Peridiniales. Micropaleontology, 14: 265–304.
- Wang, Z., Qi, Y. & Yang, Y. (2007). Cyst formation: an important mechanism for the termination of Scrippsiella trochoidea (Dinophyceae) bloom. Journal of Plankton Research, 29: 209–218.
- Zhang, J. (1997). Study of dinoflagellate life cycle and its role in population dynamics. University of Rhode Island, Ph.D thesis. 245 pp.
- Zinssmeister, C., Soehner, S., Facher, E., Kirsch, M., Meier, K.J.S. & Gottschling, M. (2011). Catch me if you can: the taxonomic identity of Scrippsiella trochoidea (F. Stein) A.R. Loebl. (Thoracosphaeraceae, Dinophyceae). Systematics and Biodiversity, 9: 145–157.
- Zinssmeister, C., Soehner, S., Kirsch, M., Facher, E., Sebastian Meier, K. & Keupp, H. (2012). Same but different: Two novel bicarinate species of extant calcareous dinophytes (Thoracosphaeraceae, Peridiniales) from the Mediterranean Sea. Journal of Phycology, 48: 1107–1118.