ABSTRACT
Dictyota cyanoloma has recently been described from the Mediterranean Sea and Macaronesia but doubt had arisen as to whether this species was truly native in Europe. The species is mainly found on non-natural substrata (harbour walls, marinas, boat hulls, etc.), strongly suggesting that it is an introduction. Molecular sequence information from historical herbarium samples proves the presence of D. cyanoloma in the Adriatic Sea as early as 1935. Since approximately the year 2000, however, the number of records as well as the geographic range of the species has expanded significantly. The present-day distribution of D. cyanoloma occupies most of the Mediterranean Sea, Macaronesia, NW Africa and southern Portugal, but recent records from Galicia and SW England (Falmouth, Cornwall) indicate that the species is rapidly expanding northward. Collections from Australia demonstrated that the species is also present from Perth in Western Australia, over much of the southern Australian coastline up to Minnie Water in New South Wales. Phylogenetic analyses resolve D. cyanoloma in a sister clade to a previously unreported Australian Dictyota species. Analysis of genetic diversity of the mitochondrial markers (nad6–nad11 and atp9–orf11) reveals that even though Australian populations contain a much higher haplotype richness, European populations are also fairly diverse. Furthermore, only two out of 25 haplotypes are shared between both regions. These somewhat counterintuitive results could be indicative of a more complicated introduction history.
Introduction
Invasive species are widely recognized as a major threat to marine biodiversity. By changing the relative abundance of native species, invasive species may profoundly affect the structure and functioning of native communities, thereby often negatively impacting local economies (Molnar et al., Citation2008; Simberloff, Citation2011). Even though only a minority of introduced species can be considered invasive, their impact can be devastating. Hence there is the necessity to document introductions of non-native species and to assess the possible risk they impose on the invaded ecosystem (Molnar et al., Citation2008). The Mediterranean Sea and the Atlantic coasts of Europe are considered hotspots of marine species introductions (Galil et al., Citation2014; Mineur et al., Citation2015). A total of 986 alien species have been reported for the Mediterranean Sea, of which 128 are macroalgae (Zenetos et al., Citation2012). This number, however, is likely to be an underestimation of the total number of introduced species owing to the difficulties surrounding morphology-based algal identification (Leliaert et al., Citation2014; Verbruggen, Citation2014). A combination of a limited set of morphological, and potentially diagnostic characters (the ‘low morphology problem’; Van Oppen et al., Citation1996) and considerable morphological plasticity that characterizes many algal species, hampers species identification based on morphological characteristics in algae. As a result, distinguishing native from non-native species becomes difficult (Grosholz, Citation2002). This taxonomic uncertainty also influences an understanding of the biogeography of the species, which is often insufficiently characterized. In a context of introduced species, uncertainty regarding native and introduced distributions led Carlton (Citation1996) to coin the term ‘cryptogenic species’, i.e. species that are not demonstrably native or introduced.
Here we aim to ascertain the origins of D. cyanoloma, which was recently described from the Mediterranean Sea in Tronholm et al. (Citation2010). The species was first reported from the Iberian Peninsula as D. ciliolata by Rull Lluch et al. (Citation2007). Sequence data, however, demonstrated unequivocally that the Iberian specimens were clearly distinct from D. ciliolata (Tronholm et al., Citation2010). Because the species did not correspond to the type material of any Dictyota of Mediterranean or Macaronesian origin and in the absence of evidence for it to be present outside of Europe, D. cyanoloma was described as a new species native to Europe. This study also significantly expanded the known distribution range of the species to include the Adriatic Sea as well as Macaronesia (Tronholm et al., Citation2010). Nevertheless, some aspects relating to the ecology and morphology of the species cast doubt on its status as a native species. First, historical interest in the diversity of Dictyota in Europe (e.g. Hamel, 1931–Citation1939; Weberpeukert, Citation1986; Schnetter et al., Citation1987; Hornig & Schnetter, Citation1988; Hornig et al., Citation1992a, Citation1992b), and the fact that the species is easily discernible by its blue iridescent margins, raises the question why D. cyanoloma had gone unnoticed for so long. Second, the specimens of D. cyanoloma are often collected from non-natural habitats such as marinas (Rull Lluch et al., Citation2007; Aragay Soler et al., Citation2014). Last, a recent sampling campaign recovered what appears to be the same species from the south of Australia (De Clerck and Verbruggen, unpublished). The combination of these factors challenges the status of D. cyanoloma as an indigenous European species. However, to date it remains unknown whether D. cyanoloma is native to Europe and introduced to Australia, or vice versa.
The main objective of this study was to provide molecular support for the origins of this cryptogenic species. Therefore, we first applied a phylogenetic approach to determine the affinities of D. cyanoloma with Australian species of the genus. We then compared the diversity and genetic structure of Australian and European populations based on two variable mitochondrial loci (nad6–nad11 and atp9–orf11). Last, the current and historical geographic range of the species within Europe was assessed employing both historical and recent records.
Materials and methods
DNA extraction, amplification and sequencing
Dictyota cyanoloma Tronholm, De Clerck, Gómez Garreta & Rull Lluch was sampled from Australia, the North-east Atlantic, the Mediterranean and Macaronesia (). Specimens were taken fresh from the field and apical tips were dried in silica gel for rapid dehydration and storage. In addition, the Ghent and the Zagreb herbaria were screened for D. cyanoloma vouchers and subsampled for DNA extraction. DNA was extracted employing a cetyltrimethyl ammonium bromide extraction method as outlined in Steen et al. (Citation2015). We amplified and sequenced two variable intergenic spacer regions of the mitochondrial genome, the nad6–nad11 and atp9–orf11 regions (). Primers were designed in the flanking coding region of both markers, using PRIMER3 software (Untergasser et al., Citation2012). Primer design was aided by the availability of the mitochondrial genome of D. dichotoma (Secq et al., Citation2006) and a draft mitochondrial genome of D. cyanoloma (De Clerck & Steen, unpublished). Primer sequences are displayed in . Plastid-encoded PSII reaction centre protein D1 (psbA) and RUBISCO large subunit (rbcL), mitochondrial-encoded cytochrome oxidase subunit 1 and 3 (cox1, cox3) and NADH dehydrogenase subunit 1 (nad1), and partial nuclear ribosomal LSU rDNA genes were amplified and sequenced following De Clerck et al. (Citation2006), Hwang et al. (Citation2009) and Silberfeld et al. (Citation2010).
Table 1. Specimens of D. cyanoloma used for the network analysis, with indication of haplotype number (h), habitat, locality with coordinates, year of sampling, and accession number per phylogenetic marker.
Table 2. Primer sequences for the mitochondrial markers nad6–nad11 and atp9–orf11.
Phylogenetic position of Dictyota cyanoloma within the genus Dictyota
A phylogenetic tree was constructed from a multigene alignment, using one representative per species, sequenced for six markers (psbA, rbcL, cox1, cox3, nad1, LSU rDNA) (Supplementary Table 1S). All Dictyota species native to the North-east Atlantic, the Mediterranean and Macaronesia were included (Tronholm et al., Citation2010) as well as species closely related to D. cyanoloma, selected based on a preliminary psbA NJ phylogeny (data not shown). Partitioning strategies were selected with Partitionfinder (Lanfear et al., Citation2012). A partitioning scheme with seven partitions organized by codon position and organelle and a GTR+G model of sequence evolution was adapted in a subsequent Bayesian analysis with MrBayes 3.2 (Ronquist et al., Citation2012). Two parallel runs, consisting of three heated chains and one cold chain each, were run for 9 million generations, using default priors and chain temperature settings. The cold chain was sampled every 1000 generations. Tracer 1.4 was used to assess stationarity of log-likelihoods and parameter values (Drummond & Rambaut, Citation2007). A burn-in sample of 1000 trees was removed before construction of a majority rule consensus tree.
A maximum likelihood ML analysis, using the same partitioning strategy, was conducted employing RAxML v.8.1.11 under a GTRCAT substitution model. The robustness of the resulting phylogenies was tested using 1000 replicates of a rapid bootstrap heuristic (Stamatakis et al., Citation2008).
Geographic distribution of Dictyota cyanoloma
Geographic data from a global Dictyotales dataset (http://www.phycology.ugent.be/Dictyotales/distribution.pdf), summarizing taxonomic and geographic data of more than 4400 specimens, were plotted on a global map, highlighting the occurrences of D. cyanoloma. Additional maps of the presumed introduced and native range were constructed indicating the localities of all samples. A distinction was made between those records published earlier and those either confirmed within this study, or obtained from historical herbarium specimens, or unconfirmed based on photographic evidence. Annual mean minimum and maximum sea surface temperature isotherms obtained from Bio-ORACLE (Tyberghein et al., Citation2012), flanking the inferred species range in both hemispheres were indicated.
Genetic diversity and haplotype network construction
Concatenated nad6–nad11 and atp9–orf11 sequences (1235bp), corrected for indels following Simmons & Ochoterana (Citation2000), were collapsed into haplotypes. Haplotype networks were constructed based on traditional phylogenetic algorithms, an approach which has been demonstrated to outperform widespread network constructing methods (Salzburger et al., Citation2011). Maximum parsimony- and maximum likelihood phylogenies were estimated in MEGA6 (Tamura et al., Citation2013) and RaxML v.8.1.11 (Stamatakis et al., Citation2008), respectively, and converted into haplotype networks using Haploviewer (available at http://www.cibiv.at/~greg/haploviewer).
Levels of genetic variation (haplotype diversity, nucleotide diversity and haplotype richness) in European and Australian populations were estimated in Arlequin 3.5 (Excoffier & Lischer, Citation2010). Estimates of haplotype richness after rarefaction were obtained with a rarefaction calculator based on the program HP-RARE (Kalinowski, Citation2005). Haplotype richness was standardized to the smallest population size (32) to obtain an unbiased estimate of the haplotype richness for inter-population comparisons (Leberg, Citation2002).
Results
Phylogenetic position of Dictyota cyanoloma within the genus Dictyota
Bayesian and maximum likelihood analyses of a multilocus dataset based on six markers (psbA, rbcL, cox1, cox3, nad1, LSU rDNA) resulted in highly congruent and reasonably well-supported phylogenetic trees (). Dictyota cyanoloma was resolved (BS-values = 100; BPP = 1.0) as the sister taxon to an unidentified Dictyota species from Western Australia (Dictyota sp8, HV2563). The larger clade to which D. cyanoloma belongs, contained several other Australian species (D. alternifida, D. dichotoma3, D. diemensis, D. intermedia) next to species of which the actual distribution is hitherto unclear (D. sp7, D. sp21, D. liturata, D. stolonifera) due to restricted taxon sampling.
Geographic distribution of Dictyota cyanoloma
A map displaying the distributions of more than 4400 Dictyotales specimens indicated that D. cyanoloma is present both in Europe and South Australia, but presumably absent elsewhere (). European records stretch from Cornwall, England to the Canary Islands and North-west Africa in the South and the Gulf of Izmir in the eastern Mediterranean Sea. This range is bordered by a 9°C minimum sea surface temperature (SSTmin) and a 27°C maximum sea surface temperature (SSTmax) (). No samples from the southern hemisphere were found outside a thermal range bordered by the 12°C SSTmin and 26°C SSTmax ().
Fig. 2. Distribution map of a global Dictyota data set (green) highlighting the occurrences of D. cyanoloma (red) and D. sp8 (yellow).
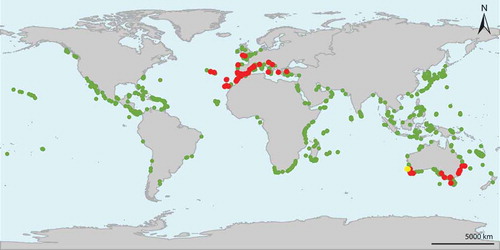
Fig. 3. The sample locations (dots) within the native range (A) and the region of introduction (B) are reported. The reported isotherms on both maps are the annual mean maximum and minimum sea surface temperatures bordering the distribution range of Dictyota cyanoloma within the native region (full lines) contrasted with the annual mean maximum and minimum sea surface temperatures bordering the distribution within the introduced range (dashed lines). A distinction is made between samples from this study (red), earlier published records (green), photographic evidence (blue) and herbarium samples (yellow).
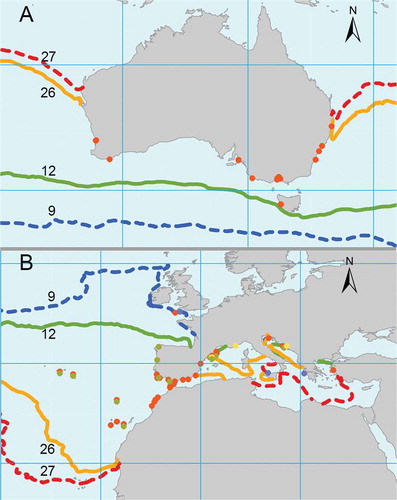
The earliest D. cyanoloma records in Europe were from the Adriatic Sea (Split), collected in 1935. Additional records from 1947, 1958 and 1976 (herbarium), 2009 (Tronholm et al., Citation2010) and 2014 (this study) form circumstantial evidence of its intermittent presence in the Adriatic Sea. The first published record of D. cyanoloma appeared in 2007 by Rull Lluch et al. (Citation2007) and was incorrectly identified as D. ciliolata. Herbarium species sequenced in this study from Cannes (1999) and Barcelona (2005) precede this record. Records within the NE-Atlantic were noted for Aveiro (Barbara et al., Citation2014) and A Coruña (Barbara et al., Citation2014; Peña et al., Citation2014) and SW Britain (2014) (). More southern records were sampled in the Canary Islands (2009) and Morocco (2010) (). A single record was reported for the Aegean Sea, in the Gulf of Izmir in Turkey (Taskin, Citation2013). Next to these published records, evidence based on photographs searchable on the internet indicated the presence of D. cyanoloma in Greece and Sicily (Supplementary Table 2S). All records were confirmed genetically except the specimens from Turkey, Galicia, Greece and Sicily.
Genetic diversity and haplotype networks
A total of 25 haplotypes were found among 93 D. cyanoloma samples. In the haplotype network a 37 bp gap separated three Australian specimens (LT0030, LT0384, HV2123) from the remainder of the specimens. The cox1 gene sequences of the two clusters, however, differed by no more than 1.6%. Compared with typical interspecific distances in Dictyota (~ 20–30%) this is a low value that persuaded us to treat both clusters as the same species. Australian samples contained 16 haplotypes (32 samples), including 12 singletons, while in Europe 11 haplotypes are recorded for 61 samples, including four singletons. Only two haplotypes, however, were shared between Australia and Europe. One of the shared haplotypes was from a Croatian herbarium sample from 1958. Haplotype networks based on ML- () or MP-trees (Supplementary Fig. 1S) were generally consistent in the patterns they reveal, except in the reciprocal positioning of the singleton haplotypes from three out of four of the herbarium specimens and in the position of haplotype 15. These minor differences do not, however, alter the general phylogeographic pattern.
Fig. 4. Haplotype network based on a ML phylogeny indicating Australian (blue), European (orange) and sequenced Split herbarium samples (asterisks). The size of the circles is proportional to the amount of individuals of that haplotype. The number specifies the haplotype. (WA: West Australia, NSW: New South Wales, VIC: Victoria, SA: South Australia, MOR: Morocco, POR: Portugal, AZ: Azores, MAD: Madeira, SP: Spain, CI: Canary Islands, CR: Croatia, UK: United Kingdom, FR: France.)
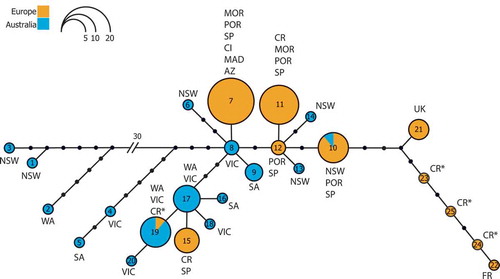
For the calculation of the genetic diversity indices, haplotypes of four European specimens collected in 1935 and 1958 in Split (D3815, D3815b, D3818, D3819) were removed as these were not representative of the current genetic diversity within the introduced range. The corresponding haplotype diversity for Australia and Europe were 0.8931 ± 0.0370 and 0.7713 ± 0.0327 respectively. The nucleotide diversities were 0.008967 ± 0.004653 for Australia compared with 0.002904 ± 0.001660 for Europe. Haplotype richness after rarefaction brought the total number of Australian haplotypes to 16 compared with 7 for Europe.
Discussion
Using a combination of phylogenetic and phylogeographic analyses, we have demonstrated that Dictyota cyanoloma, recently described from Europe (Tronholm et al., Citation2010) is also present in large parts of Australia. Below we discuss the plausibility of a number of alternative hypotheses that could potentially account for this pattern.
Alternative hypotheses for the disjunct distribution of Dictyota cyanoloma
An initial hypothesis, that Australian and European specimens belong to different species, is not supported by the genetic data. Genetic divergence between Australian and European populations of typical barcoding markers such as the cox1 gene is very close to 0%. As a comparison, interspecific p-distances among Dictyota species vary from 7–8% to about 25%. In addition, a haplotype network based on mitochondrial nad6–nad11 and atp9–orf11 regions fails to illustrate two clusters corresponding to European and Australian samples. Haplotypes between both regions are interspersed.
It is also unlikely that D. cyanoloma is characterized by a continuous biogeographic range extending from Europe to Australia. First, seaweeds are predominantly characterized by relatively narrow thermal tolerances (Santelices, Citation1980; Van den Hoek, Citation1982; Eggert, Citation2012), making exceptionally wide latitudinal ranges spanning tropical as well as temperate regions uncommon. Second, no specimens were sampled in tropical latitudes or in other temperate areas (e.g. southern Africa) as shown by our global Dictyotales dataset (). It is therefore most likely that D. cyanoloma has a truly disjunct distribution, with established populations in Europe and Australia. In the light of our present knowledge of natural seaweed distributions and cases of anthropogenic introductions of non-native species through maritime traffic and aquaculture, a discontinuous distribution pattern as demonstrated by Dictyota cyanoloma is highly indicative for an introduction history.
Evidence supporting an Australian native range of Dictyota cyanoloma
Under the hypothesis that the current biogeographic range of Dictyota cyanoloma involves an introduction, the question remains as to which area should be considered the native range. Several lines of evidence support the hypothesis that D. cyanoloma has been introduced in Europe from Australia. First, a phylogenetic analysis shows that D. cyanoloma belongs to a clade consisting of species with a mainly South Pacific distribution, many of them restricted to Australia. Furthermore, the fact that D. sp8, the sister species of D. cyanoloma, is recorded only from Australia is additional evidence for an Australian native range. Australasia is known as one of the main three regions from which species are introduced into the Mediterranean, next to the Red Sea and the North-western Pacific (Mineur et al., Citation2015). While Lessepsian migration and the transport of live shellfish, especially oysters, are the main introduction pathways and vectors from the latter two regions respectively, it has not been demonstrated what caused the introductions of Australasian species into the Mediterranean (Mineur et al., Citation2015), except for aquarium trade in the case of Caulerpa taxifolia (Jousson et al., Citation1998).
Even though reduced genetic diversity of invasive populations may not be as commonplace as once hypothesized (Roman & Darling, Citation2007; Dlugosch & Parker, Citation2008), for the marine environment the opposite pattern seems to be the rule rather than the exception. At meta-analysis by Rius et al. (Citation2015) revealed that no less than 74% of the marine introduced species for which genetic data are available, display similar genetic diversity values in both native and introduced ranges. These results strongly favour the notion that genetic diversity is not necessarily impaired when species are introduced. A reduction of genetic diversity, as documented for the invasive seaweed species Codium fragile ssp. fragile (Provan et al., Citation2005) and for Sargassum muticum (Bae et al., Citation2013; Cheang et al., Citation2010), could therefore represent an exception rather than the rule.
For Dictyota cyanoloma, allelic richness is higher in Australia than in Europe, but haplotype and nucleotide diversities indicate that Australian as well as European populations harbour a high level of genetic diversity. Australian haplotype diversities are only slightly higher than in Europe, but the nucleotide diversities differ significantly. This discrepancy can be indicative of a bias in calculated diversity measures (Goodall-Copestake et al., Citation2012). Diverging haplotypes within a sample suggest undersampling and inflate estimates of nucleotide diversity, as is the case for the Australian population. The high proportion of singleton haplotypes (12 out of 16) together with the large genetic gap for Australian specimens suggest that this region is undersampled, and thus might inflate nucleotide diversities. Allelic richness is higher for Australia (16 versus seven haplotypes).
Recent insights, however, claim that genetic diversity is not necessarily depauperate after an aquatic invasion. In many cases, large inocula and multiple introductions boost the genetic diversity in the introduced range (Roman & Darling, Citation2007). Introduced populations of Undaria pinnatifida clearly illustrate the importance of the above factors. While Australasian introduced populations show a high genetic diversity, European introduced populations have a much lower diversity in comparison to the Asian source populations. This disparity stems from the fact that the European introduction was the consequence of an intentional introduction for aquaculture, while the Australasian populations were the consequence of recurrent introductions by accidental translocations associated with maritime traffic (Voisin et al., Citation2005). Multiple introductions or great genetic diversity within the inocula together with beneficial life history characteristics as clonal propagation could have enabled D. cyanoloma to by-pass successfully a bottleneck associated with the founder event and to establish a genetically diverse introduced population.
Within Europe, D. cyanoloma is thriving in non-natural environments such as ports, marinas and yachting clubs (, ), and artificial substrata such as pontoons, jetties and neglected ship hulls are often fouled by the species (). Along the Iberian Peninsula and the NW Moroccan coast, some natural habitats harbour populations of the species. In comparison sampling localities within Australia are almost strictly natural environments. This observation is concurrent with the idea of Europe being the introduced range, as marine invaders are often growing on artificial substrata.
Figs 5 and 6. Dictyota cyanoloma. Fig. 5. Dictyota cyanoloma clearly showing its characteristic blue iridescent margin, photograph taken by Joana Aragay, port of Almeria, 2013. Fig. 6. Boat hull fouled by Dictyota cyanoloma, photograph taken by Olivier De Clerck at Garachico Bay, Tenerife, 2014.
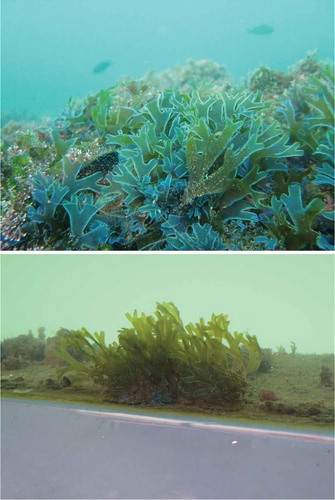
The combination of these findings offers support for the hypothesis of an introduction of D. cyanoloma from the southern hemisphere into the Mediterranean and Europe.
Introduction and potential spread within Europe
Molecular data demonstrated that the species was at least present in the Adriatic Sea as early as 1935. Its presence in the Split area is confirmed by molecular data obtained from herbarium samples collected in 1947, 1958 and 1976. Other herbarium records from Cannes (1999) and Barcelona (2005) were confirmed to be genuine D. cyanoloma and all pre-date the finding of Rull Lluch et al. (Citation2007). Since the publication of Tronholm et al. (Citation2010), species observations (UK, Greece, Sicily) and published records (Taskin, Citation2013; Barbara et al., Citation2014; Peña et al., Citation2014) increased steadily, with reports for the North-east Atlantic until the eastern Mediterranean and Macaronesia. The question remains as to whether D. cyanoloma was unreported until about a decade ago despite being widespread in southern Europe or whether the species only spread recently despite being locally present in the Mediterranean Sea for several decades. Accepting the historical records in the Adriatic as evidence for a species introduction within this area would be premature, as no other collections than the Ghent and Zagreb herbarium were screened for this species. In this respect and based on our data, we cannot exclude the hypothesis that D. cyanoloma was present along most European coastlines throughout most of the 20th century. The same was the case for Codium fragile, which had been colonizing habitats across the world in some cases nearly 100 years before it was noticed and remaining cryptically present (Provan et al., Citation2005).
The occurrence of populations of D. cyanoloma in marinas and yachting clubs suggest an important role of recreational boating as a secondary dispersal vector. This could explain the presence of D. cyanoloma in the Falmouth marina in Cornwall. This marina is one of the most famous and well-visited yachting marinas in the south of the UK, often used as a stop-over point for yachts returning from southern Europe.
Comparison of the thermal boundaries of the native and introduced ranges shows that these are similar, except that D. cyanoloma has established sustainable populations in significantly lower temperatures in its introduced range than in its native range. The most southern sample from Tasmania does not go below a mean minimum temperature of 12°C, while in Europe, populations are established in the south-west of Britain (UK), where mean minimum temperatures are as low as 9°C. This could indicate that the climatic tolerance for the species is wider than the conditions to which it is subjected in its native range. The same has been noted for several invasive tropical algae in the Mediterranean, which thrive in temperatures lower than the limit of tropical temperatures (Boudouresque & Verlaque, Citation2002). Extreme mean maximum temperatures isotherms for the species are comparable in both the native and introduced range (26 and 27° C, respectively). Due to this northward extension of the minimum thermal boundary in Europe, D. cyanoloma has been able to establish itself in a geographic range exceeding the size of its presumed potential range.
Conclusions and future prospects
Using gene sequence data we provide ample evidence for the introduced nature of D. cyanoloma in Europe. However, several questions remain unanswered to date. The main question is whether D. cyanoloma was ubiquitous but unrecognized within the Mediterranean throughout the 20th century, or whether it was an introduction that remained contained during a lag phase of several decades before expanding its range (Crooks et al., Citation1999; Mack et al., Citation2000; Sakai et al., Citation2001). An initial step to resolve this matter would be to screen other European herbaria for historical records. Additionally, a population genetic study including thorough sampling of both the introduced and native range, together with the use of more variable multilocus molecular markers such as microsatellites, could shed a light on different ecological processes as colonization and dispersal. Contrasting the multilocus genetic diversity of both native and introduced populations could pinpoint source populations and allow us to infer whether there was a single or multiple successive introductions within Europe. This approach might answer important questions regarding pathways and dynamics of secondary spread within the introduced range. There are indications that recreational boating acts as a secondary vector since D. cyanoloma is growing abundantly in marinas and harbours, where polluted conditions hamper the growth of native species. Recent reports of populations in natural environments raise suspicions that they might spread from these marina populations. These patterns should be monitored, as well as the ecological impact of the species on the native communities. Last, it seems evident that D. cyanoloma is still spreading north. Predicting the eventual range in Europe therefore is important. Regarding the extent to which the species has spread, it is important to assess what exactly the potential range within Europe could be.
Supplementary information
The following supplementary material is accessible via the Supplementary Content tab on the article’s online page at http://dx.doi.org/10.1080/09670262.2016.1212998
Supplementary fig. 1S. Haplotype networks based on both best scoring MP phylogenies
Supplementary table 1S. Specimen table employed for phylogenetic inference
Supplementary table 2S. Links to photographic evidence of D. cyanoloma occurrences
Supplementary material
Download MS Word (178.6 KB)Acknowledgements
Lisa Rennocks, Marta Sanson, Judy Sutherland and Howard Steele are gratefully acknowledged for the collection of samples and sharing of information.
Disclosure statement
No potential conflict of interest was reported by the authors.
Additional information
Funding
Notes on contributors
Frederique Steen
F. Steen, O. De Clerck: original concept, generating data and drafting manuscript; J. Aragay, D. Vitales, A. Gomez Garreta sampling and generating molecular data; F. Bunker, H. Verbruggen and F.P. Mancuso sampling invaded and native range; A. Zuljevic screening herbarium collections and sampling. All authors contributed to the writing of the manuscript.
Joana Aragay
F. Steen, O. De Clerck: original concept, generating data and drafting manuscript; J. Aragay, D. Vitales, A. Gomez Garreta sampling and generating molecular data; F. Bunker, H. Verbruggen and F.P. Mancuso sampling invaded and native range; A. Zuljevic screening herbarium collections and sampling. All authors contributed to the writing of the manuscript.
Ante Zuljevic
F. Steen, O. De Clerck: original concept, generating data and drafting manuscript; J. Aragay, D. Vitales, A. Gomez Garreta sampling and generating molecular data; F. Bunker, H. Verbruggen and F.P. Mancuso sampling invaded and native range; A. Zuljevic screening herbarium collections and sampling. All authors contributed to the writing of the manuscript.
Heroen Verbruggen
F. Steen, O. De Clerck: original concept, generating data and drafting manuscript; J. Aragay, D. Vitales, A. Gomez Garreta sampling and generating molecular data; F. Bunker, H. Verbruggen and F.P. Mancuso sampling invaded and native range; A. Zuljevic screening herbarium collections and sampling. All authors contributed to the writing of the manuscript.
Francesco Paolo Mancuso
F. Steen, O. De Clerck: original concept, generating data and drafting manuscript; J. Aragay, D. Vitales, A. Gomez Garreta sampling and generating molecular data; F. Bunker, H. Verbruggen and F.P. Mancuso sampling invaded and native range; A. Zuljevic screening herbarium collections and sampling. All authors contributed to the writing of the manuscript.
Francis Bunker
F. Steen, O. De Clerck: original concept, generating data and drafting manuscript; J. Aragay, D. Vitales, A. Gomez Garreta sampling and generating molecular data; F. Bunker, H. Verbruggen and F.P. Mancuso sampling invaded and native range; A. Zuljevic screening herbarium collections and sampling. All authors contributed to the writing of the manuscript.
Daniel Vitales
F. Steen, O. De Clerck: original concept, generating data and drafting manuscript; J. Aragay, D. Vitales, A. Gomez Garreta sampling and generating molecular data; F. Bunker, H. Verbruggen and F.P. Mancuso sampling invaded and native range; A. Zuljevic screening herbarium collections and sampling. All authors contributed to the writing of the manuscript.
Amelia Gómez Garreta
F. Steen, O. De Clerck: original concept, generating data and drafting manuscript; J. Aragay, D. Vitales, A. Gomez Garreta sampling and generating molecular data; F. Bunker, H. Verbruggen and F.P. Mancuso sampling invaded and native range; A. Zuljevic screening herbarium collections and sampling. All authors contributed to the writing of the manuscript.
Olivier De Clerck
F. Steen, O. De Clerck: original concept, generating data and drafting manuscript; J. Aragay, D. Vitales, A. Gomez Garreta sampling and generating molecular data; F. Bunker, H. Verbruggen and F.P. Mancuso sampling invaded and native range; A. Zuljevic screening herbarium collections and sampling. All authors contributed to the writing of the manuscript.
References
- Aragay Soler, J., Gomez Garreta, A., Ribera Siguan, M.A. & Rull Lluch, J. (2014). New data about the phenology and the distribution of Dictyota cyanoloma Tronholm et al. in the Mediterranean Iberian coasts. In Proceedings of the 5th Mediterranean Symposium on Marine vegetation. Portoroz, Slovenia, 194–195.
- Bae, D.Y., Ang Jr, P.O. & Boo, S.M. (2013). Mitochondrial cox3 and trnW-I sequence diversity of Sargassum muticum. Aquatic Botany, 104: 220–223.
- Barbara, I., Peteiro, C., Peña, V., Altamirano, M., Piñeiro-Corbeira, C., Sanchez, N., Diaz-Tapia, P., Garcia-Redondo, V., Garcia-Fernandez, A. & Zanolla, M. (2014). Fragmentos taxonomicos, corologicos, nomenclaturales y fitocenologicos. Acta Botanica Malacitana, 39: 207–239.
- Boudouresque, C.F. & Verlaque, M. (2002). Biological pollution in the Mediterranean Sea: invasive versus introduced macrophytes. Marine Pollution Bulletin, 44: 32–38.
- Carlton, J.T. (1996). Biological invasions and cryptogenic species. Ecology, 77: 1653–1655.
- Cheang, C.C., Chu, K.H., Fujita, D., Yoshida, G., Hiraoka, M., Critchley, A., Choi, H.G., Duan, D., Serisawa, Y. & Ang, P.O. (2010). Low genetic variability of Sargassum muticum (Phaeophyceae) revealed by a global analysis of native and introduced populations. Journal of Phycology, 46: 1063–1074.
- Crooks, J.A., Soulé, M.E. & Sandlund, O. (1999). Lag times in population explosions of invasive species: causes and implications. Invasive Species and Biodiversity Management: 103–125.
- De Clerck, O., Leliaert, F., Verbruggen, H., Lane, C.E., De Paula, J.C., Payo, D.A. & Coppejans, E. (2006). A revised classification of the Dictyoteae (Dictyotales, Phaeophyceae) based on rbcL and 26S ribosomal DNA sequence analyses. Journal of Phycology, 42: 1271–1288.
- Dlugosch, K. & Parker, I. (2008). Founding events in species invasions: genetic variation, adaptive evolution, and the role of multiple introductions. Molecular Ecology, 17: 431–449.
- Drummond, A.J. & Rambaut, A. (2007). BEAST: Bayesian evolutionary analysis by sampling trees. BMC Evolutionary Biology 7.
- Eggert, A. (2012). Seaweed responses to temperature. In Seaweed Biology (Wiencke, C. and Bischof, K., editors), 47–66. Springer, the Netherlands.
- Excoffier, L. & Lischer, H.E.L. (2010). Arlequin suite ver 3.5: a new series of programs to perform population genetics analyses under Linux and Windows. Molecular Ecology Resources, 10: 564–567.
- Galil, B.S., Marchini, A., Occhipinti-Ambrogi, A., Minchin, D., Narscius, A., Ojaveer, H. & Olenin, S. (2014). International arrivals: widespread bioinvasions in European Seas. Ethology, Ecology and Evolution, 26: 152–171.
- Goodall-Copestake, W.P., Tarling, G.A. & Murphy, E.J. (2012). On the comparison of population-level estimates of haplotype and nucleotide diversity: a case study using the gene cox1 in animals. Heredity, 109: 50–56.
- Grosholz, E. (2002). Ecological and evolutionary consequences of coastal invasions. Trends in Ecology and Evolution, 17: 22–27.
- Hamel, G. (1939). Phéophycées de France. Fascicules V: 337–432.
- Hörnig, I. & Schnetter, R. (1988). Notes on Dictyota dichotoma, Dictyota menstrualis, Dictyota indica and Dictyota pulchella Spec-Nova (Phaeophyta). Phyton-Annales Rei Botanicae, 28: 277–291.
- Hörnig, I., Schnetter, R. & Vanreine, W.F.P. (1992a). The Genus Dictyota (Phaeophyceae) in the North-Atlantic. 2. Key to the Species. Nova Hedwigia, 54: 397–402.
- Hörnig, I., Schnetter, R., Vanreine, W.F.P., Coppejans, E., Achenbachwege, K. & Over, J.M. (1992b). The genus Dictyota (Phaeophyceae) in the North-Atlantic. 1. A new generic concept and new species. Nova Hedwigia, 54: 45–62.
- Hwang, I.-K., Lee, W.J., Kim, H.-S. & De Clerck, O. (2009). Taxonomic reappraisal of Dilophus okamurae (Dictyotales, Phaeophyta) from the western Pacific Ocean. Phycologia, 48: 1–12.
- Jousson, O., Pawlowski, J., Zaninetti, L., Meinesz, A. & Boudouresque, C.-F. (1998). Molecular evidence for the aquarium origin of the green alga Caulerpa taxifolia introduced to the Mediterranean Sea. Marine Ecology Progress Series, 172: 275–280.
- Kalinowski, S.T. (2005). HP-RARE 1.0: a computer program for performing rarefaction on measures of allelic richness. Molecular Ecology Notes, 5: 187–189.
- Lanfear, R., Calcott, B., Ho, S.Y.W. & Guindon, S. (2012). PartitionFinder: combined selection of partitioning schemes and substitution models for phylogenetic analyses. Molecular Biology and Evolution, 29: 1695–1701.
- Leberg, P.L. (2002). Estimating allelic richness: effects of sample size and bottlenecks. Molecular Ecology, 11: 2445–2449.
- Leliaert, F., Verbruggen, H., Vanormelingen, P., Steen, F., López-Bautista, J.M., Zuccarello, G.C. & De Clerck, O. (2014). DNA-based species delimitation in algae. European Journal of Phycology, 49: 179–196.
- Mack, R.N., Simberloff, D., Mark Lonsdale, W., Evans, H., Clout, M. & Bazzaz, F.A. (2000). Biotic invasions: causes, epidemiology, global consequences, and control. Ecological Applications, 10: 689–710.
- Mineur, F., Arenas, F., Assis, J., Davies, A.J., Engelen, A.H., Fernandes, F., Malta, E.-J., Thibaut, T., Van Nguyen, T., Vaz-Pinto, F., Vranken, S., Serrão, E.A. & De Clerck, O. (2015). European seaweeds under pressure: consequences for communities and ecosystem functioning. Journal of Sea Research, 98: 91–108.
- Molnar, J.L., Gamboa, R.L., Revenga, C. & Spalding, M.D. (2008). Assessing the global threat of invasive species to marine biodiversity. Frontiers in Ecology and the Environment, 6: 485–492.
- Peña, V., Bárbara, I., Grall, J., Maggs, C.A. & Hall-Spencer, J. (2014). The diversity of seaweeds on maerl in the NE Atlantic. Marine Biodiversity, 44: 533–551.
- Provan, J., Murphy, S. & Maggs, C.A. (2005). Tracking the invasive history of the green alga Codium fragile ssp. tomentosoides. Molecular Ecology, 14: 189–194.
- Rius, M., Turon, X., Bernardi, G., Volckaert, F. A. & Viard, F. (2015). Marine invasion genetics: from spatio-temporal patterns to evolutionary outcomes. Biological Invasions, 17: 869–885.
- Roman, J. & Darling, J.A. (2007). Paradox lost: genetic diversity and the success of aquatic invasions. Trends in Ecology and Evolution, 22: 454–464.
- Ronquist, F., Teslenko, M., Van Der Mark, P., Ayres, D.L., Darling, A., Hohna, S., Larget, B., Liu, L., Suchard, M.A. & Huelsenbeck, J.P. (2012). MrBayes 3.2: efficient bayesian phylogenetic inference and model choice across a large model space. Systematic Biology, 61: 539–542.
- Rull Lluch, J.R., Ballesteros, E., Barcelo, M.C., Garreta, A.G. & Siguan, M.A.R. (2007). Dictyota ciliolata sonder ex Kützing (Phaeophyceae, Dictyotales) in the Mediterranean Sea. Cryptogamie, Algologie, 28: 89–97.
- Sakai, A.K., Allendorf, F.W., Holt, J.S., Lodge, D.M., Molofsky, J., With, K.A., Baughman, S., Cabin, R.J., Cohen, J.E. & Ellstrand, N.C. (2001). The population biology of invasive species. Annual Review of Ecology and Systematics, 32: 305–332.
- Salzburger, W., Ewing, G.B. & Von Haeseler, A. (2011). The performance of phylogenetic algorithms in estimating haplotype genealogies with migration. Molecular Ecology, 20: 1952–1963.
- Santelices, B. (1980). Phytogeographic characterization of the temperate coast of Pacific South America. Phycologia, 19: 1–12.
- Schnetter, R., Hornig, I. & Weberpeukert, G. (1987). Taxonomy of some North-Atlantic Dictyota species (Phaeophyta). Hydrobiologia, 151: 193–197.
- Secq, M.P.O., Goer, S.L., Stam, W.T. & Olsen, J.L. (2006). Complete mitochondrial genomes of the three brown algae (Heterokonta: Phaeophyceae) Dictyota dichotoma, Fucus vesiculosus and Desmarestia viridis. Current Genetics, 49: 47–58.
- Silberfeld, T., Leigh, J.W., Verbruggen, H., Cruaud, C., De Reviers, B. & Rousseau, F. (2010). A multi-locus time-calibrated phylogeny of the brown algae (Heterokonta, Ochrophyta, Phaeophyceae): investigating the evolutionary nature of the “brown algal crown radiation”. Molecular Phylogenetics and Evolution, 56: 659–674.
- Simberloff, D. (2011). How common are invasion-induced ecosystem impacts? Biological Invasions, 13: 1255–1268.
- Simmons, M.P. & Ochoterena, H. (2000). Gaps as characters in sequence-based phylogenetic analyses. Systematic Biology, 49: 369–381.
- Stamatakis, A., Hoover, P. & Rougemont, J. (2008). A rapid bootstrap algorithm for the RAxML web servers. Systematic Biology, 57: 758–771.
- Steen, F., Vieira, C., Leliaert, F., Payri, E.C. & Clerck, O.D. (2015). Biogeographic affinities of Dictyotales from Madagascar: a phylogenetic approach. Cryptogamie, Algologie, 36: 129–141.
- Tamura, K., Stecher, G., Peterson, D., Filipski, A. & Kumar, S. (2013). MEGA6: molecular evolutionary genetics analysis version 6.0. Molecular Biology and Evolution, 30: 2725–2729.
- Taskin, E. (2013). New records of three dictyotalean brown algae for Turkey. Botanica Marina, 56: 299–302.
- Tronholm, A., Steen, F., Tyberghein, L., Leliaert, F., Verbruggen, H., Siguan, M.A.R. & De Clerck, O. (2010). Species delimitation, taxonomy, and biogeography of Dictyota in Europe (Dictyotales, Phaeophyceae). Journal of Phycology, 46: 1301–1321.
- Tyberghein, L., Verbruggen, H., Pauly, K., Troupin, C., Mineur, F. & De Clerck, O. (2012). Bio-ORACLE: a global environmental dataset for marine species distribution modelling. Global Ecology and Biogeography, 21: 272–281.
- Untergasser, A., Cutcutache, I., Koressaar, T., Ye, J., Faircloth, B.C., Remm, M. & Rozen, S.G. (2012). Primer3 – new capabilities and interfaces. Nucleic Acids Research, 40: e115.
- Van Den Hoek, C. (1982). The distribution of benthic marine algae in relation to the temperature regulation of their life histories. Biological Journal of the Linnean Society, 18: 81–144.
- Van Oppen, M.J., Klerk, H., Olsen, J.L. & Stam, W.T. (1996). Hidden diversity in marine algae: some examples of genetic variation below the species level. Journal of the Marine Biological Association of the United Kingdom, 76: 239–242.
- Verbruggen, H. (2014). Morphological complexity, plasticity, and species diagnosability in the application of old species names in DNA-based taxonomies. Journal of Phycology, 50: 26–31.
- Voisin, M., Engel, C.R. & Viard, F. (2005). Differential shuffling of native genetic diversity across introduced regions in a brown alga: aquaculture vs. maritime traffic effects. Proceedings of the National Academy of Sciences USA, 102: 5432–5437.
- Weberpeukert, G. (1986). Ontogenic, autoecological and taxonomic investigations on selected species of the genus of Dictyota (Dictyotales, Phaeophyceae) .1. Cytological data as differentiating characteristics. Nova Hedwigia, 42: 123–149.
- Zenetos, Α., Gofas, S., Morri, C., Rosso, A., Violanti, D., Garcia Raso, J.E., Cinar, M.E., Almogi-Labin, A., Ates, A.S., Azzuro, E., Ballesteros, E., Bianchi, C.N., Bilecenoglu, M., Gambi, M.C., Giangrande, A., Gravili, C., Hyams-Kaphzan, O., Karachle, P.K., Katsanevakis, S., Lipej, L., Mastrototaro, F., Mineur, F., Pancucci-Papadopoulou, M.A., Ramos Espla, A., Salas, C., San Martin, G., Sfriso, A., Streftaris, N. & Verlaque, M. (2012). Alien species in the Mediterranean Sea by 2012. A contribution to the application of European Union’s Marine Strategy Framework Directive (MSFD). Part 2. Introduction trends and pathways. Mediterranean Marine Science, 13: 328–352.