Abstract
The recognition that ultraviolet radiation has harmful effects on the skin has led to the commercial development of inorganic and synthetic organic UV filters that can reduce the negative effects of exposure to sunlight. In addition, moisturizing chemicals are extensively used in personal care products to improve the ability of skin to retain water. Whilst current UV filter and moisturizing chemicals have clear beneficial qualities, they may also have adverse effects such as contact sensitivity, oestrogenicity and even tumorigenic effects on human skin. Furthermore, the accumulation of these chemicals in the aquatic environment could be potentially harmful. Consequently, there is interest in exploiting safer alternatives derived from biological sources, especially from photosynthetic organisms such as cyanobacteria which have developed mechanisms for coping with high UV irradiation and desiccation. In order to overcome the detrimental effects of UV radiation, these microorganisms produce UV screening compounds such as mycosporine-like amino acids and scytonemin, which are good candidates as alternatives to current synthetic UV filters. In addition, extracellular substances produced by some extremophilic species living in hyper-arid habitats have a high water retention capacity and could be used in cosmetic products as moisturizers. In this review, we present an overview of the literature describing the potential of cyanobacterial metabolites as an alternative source for sunscreens and moisturizers.
Introduction
The cosmetic and skin care products industry is a huge and rapidly expanding worldwide business (Kligman, Citation2000). In the USA, where there are more than 2000 companies related to manufacturing and distributing cosmetics, these products are integral to daily grooming. American adults, on average, use at least seven different skin care products each day (Wolf et al., Citation2001). In 2014, the cosmetics market in Germany, France and the UK was 13.01, 10.6 and 10.4 billion Euros, respectively (http://www.statista.com/statistics/382100/european-cosmetics-market-volume-by-country/). Cosmetics, however, may contain products with possible undesirable consequences. A study in the UK revealed that over the course of a year, 23% of women and 13.8% of men experience some kind of adverse reactions to personal care products (Orton & Wilkinson, Citation2004). Cosmetics may have side effects such as contact sensitivity, oestrogenicity and even tumorigenic effects on human skin (Ichihashi et al., Citation2003; Lu et al., Citation2009). In addition, widespread use of cosmetics has led to a ubiquitous presence of some ingredients in the aquatic environment which can cause hormonal effects on aquatic organisms and insects (Christen et al., Citation2011; Ozáez et al., Citation2013).
Given the possible negative consequences of using such ingredients, cosmetic scientists have begun to investigate natural compounds that offer safer alternatives. In addition, the ability of organisms to self-renew and reproduce ensures that supplies are potentially sustainable. This is especially true for photosynthetic organisms using simply light energy, carbon dioxide and basic nutrients (Witcover et al., Citation2013). Indeed, the use of plants as a source of new cosmetic ingredients is an option favoured by many companies (Kole et al., Citation2005). For example, various natural substances extracted from aquatic or land plants can be used in sunscreens owing to both their UV screening properties and antioxidant activities. Carotenoids and phenols in angiosperms (e.g. Mahajan & Wasule, Citation2008), phlorotannins in brown seaweeds and mycosporine-like amino acids in red seaweeds and microalgae are among the well-known antioxidants and UV-absorbing compounds produced by plants (Hupel et al., Citation2011). However, there are economic obstacles to the commercial exploitation of plants, including slow growth rates, seasonal growth, challenges in the cultivation of some species, the need for arable land and hence competition with food production and low levels of the active ingredients, all of which impact on the cost of production.
An alternative approach involves the exploitation of photosynthetic cyanobacteria as a biological source of cosmetic ingredients. Cyanobacteria are genetically tractable prokaryotes which typically have higher photosynthetic and growth rates than higher plants. These microorganisms have basic nutritional requirements and can be grown using closed cultivation systems that do not compete with agriculture (Quintana et al., Citation2011). Importantly, several species of cyanobacteria live in hyper-arid deserts and produce metabolites that give them the ability to cope with both high UV radiation and severe desiccation (Fleming & Castenholz, Citation2007). These extremophilic microorganisms therefore have great potential as a source of cosmetic products to be used in sunscreens and moisturizers.
In this review, we consider sunscreen and moisturizing personal care products (PCPs), highlighting the possible adverse effects related to some chemicals used in these products, and discuss the potential of cyanobacteria as an alternative source of novel metabolites for cosmetics production.
Sunscreens
Ultraviolet (UV: 280–400 nm) radiation induces short-term effects (sunburn) and chronic reactions (premature skin ageing and skin cancer) in human skin. Chronic repeated exposure to sunlight has been shown to be the main cause of benign and malignant skin tumours such as malignant melanoma (Lisby et al., Citation2005). UV-B (280–320 nm), a minor component of the sunlight reaching the earth’s surface, is the most cytotoxic and mutagenic region of the solar spectrum. UV-B is known to suppress immune reactions and upregulate gene expression through intracellular signal transduction pathways. It can also promote the formation of cyclobutane pyrimidine dimers and photoproducts that induce mutation in epidermal cells, and can lead to abnormal cell growth and increased risk of skin cancer (Budden & Bowden, Citation2013). The strong cytotoxic effects of UV-B occur in the upper epidermis as UV-B does not penetrate through to the dermis. In contrast, UV-A (320–400 nm) can reach the dermis where it is responsible for ageing changes such as wrinkling, dryness and pigment abnormalities. In addition, exposure to UV-A generates excess reactive oxygen species that indirectly damage DNA (Daniel et al., Citation2004). The phototoxic effect of UV-B is greater than UV-A, but UV-B exposure is highly dependent on the season, time of day, cloud cover and latitude. In contrast, UV-A is relatively constant with a much higher intensity and is present all year round in harmful doses. Also, unlike UV-B, which cannot penetrate window glass, about 40% of the outdoor UV-A radiation dose can still be received indoors. Therefore, the main requirement of PCPs including sunscreens, facial makeup and lip care products is UV-A protection (Daniel et al., Citation2004).
Although skin cells have various mechanisms and strategies to minimize UV damage (such as antioxidants, DNA repair enzymes and stress signalling), excessive UV exposure can cause mutations and genetic instability. Over the past few decades, sun protection measures have been improved in order to reduce radiation exposure and the harmful effects of UV-A and UV-B. One of the most important protection measures against skin damage is the use of UV filters, which are incorporated in a wide range of PCPs including sunscreens, facial make-up and lip care products (Stiefel & Schwack, Citation2013). In order to better protect against UV radiation, consumers demand PCPs with a high sun protection factor (SPF), and therefore are exposed to significantly larger amounts and also a wider variety of UV filters (Manová et al., Citation2013). The estimated worldwide production of UV filters is about 10 000 metric tonnes per year requiring high production volumes (Manová et al., Citation2015). The most important aspect of the development of a new UV filter is safety: a sunscreen must be heat- and photo-stable, non-irritant, non-sensitizing and non-phototoxic. While the ingredients in many sunscreens can be effective in protecting human skin against UV radiation, they can also have adverse effects such as contact sensitivity and oestrogenicity (Ichihashi et al., Citation2003). In addition, sunscreen ingredients potentially can have an adverse effect on the natural environment, especially when introduced into aquatic systems.
Sunscreen ingredients are generally divided into inorganic (physical blockers) and organic agents (chemical absorbers). Here we explain organic and inorganic UV filters separately and highlight the reported effects related to each group.
Inorganic UV filters
Inorganic UV filters consist of mineral particles that reflect or scatter sunlight. They are extensively used in sunscreen lotions as broadband sunscreens that reflect both UV-A and UV-B (Hexsel et al., Citation2008). The inorganic UV filters used most frequently in cosmetic products are metal oxides such as titanium dioxide (TiO2) and zinc oxide (ZnO). It has been argued that inorganic UV filters are safer than organic ones. However, there are concerns associated with the penetration and systemic effects of inorganic agents. Notably photoactivation of metal oxides by UV light can lead to the generation of reactive oxygen species such as H2O2 and singlet oxygen which are known to be cytotoxic and genotoxic (Serpone et al., Citation2007). Also, to reduce the opaqueness associated with inorganic filters, TiO2 and ZnO microparticles have been replaced by TiO2 and ZnO nanoparticles. This can have adverse effects as TiO2 nanoparticles can pass through cell membranes and impair function of human dermal fibroblast cultures with decreases in cell area, proliferation, mobility and ability to form collagen (Pan et al., Citation2009). Exposure to TiO2 micro- and nanoparticles can also induce cell death in human neural cells and fibroblasts (Lai et al., Citation2008). Nano-sized zinc oxide particles can also induce neural stem cell apoptosis, although this toxic effect is related to chemistry, rather than particle size (Deng et al., 2008; Nohynek et al., Citation2008). In addition, the impact of nanoparticle release into the natural environment is now a major concern (Baker et al., Citation2014; Cross et al., Citation2015; Schaumann et al., Citation2015).
Synthetic organic UV filters
In contrast to physical blockers, organic UV filters are a group of compounds designed to absorb UV radiation rather than reflect it. The most frequently used organic UV filters in sunscreens and other PCPs are butyl methoxydibenzoylmethane (BM-DBM), ethylhexylmethoxycinnamate (EHMC) and octocrylene (OCR) (). These are photostable chemicals effective in the protection of human skin against UV damage (Manová et al., Citation2013). However, in recent years, concerns have been raised about possible side effects of these synthetic filters.
OCR is a worldwide-approved UV filter which is increasingly used in PCPs offering protection against UV-B wavebands (Karlsson et al., Citation2011). However, this UV filter can be a strong allergen and can lead to contact dermatitis in children and adults (Avenel-Audran et al., Citation2010). Avenel-Audran et al. (Citation2010) indicated that children and adults with a history of photoallergy from sunscreen products had positive patch and photopatch test reactions to OCR. In addition, Karlsson et al. (Citation2011) reported 23 cases of positive patch and photopatch test reactions in patients with a history of reaction to the anti-inflammatory drug ketoprofen. A study in Switzerland by Schlumpf et al. (Citation2010) showed that exposure to UV filters through PCP use was correlated with the presence of chemicals in breast milk and they found that OCR and 4-methylbenzylidene camphor were present in 85.2% of the milk samples.
Methoxydibenzoylmethane (BM-DBM), also known as avobenzone, is another approved organic UV filter with absorption in the UV-A wavelength that has been linked with photoallergic contact dermatitis (Kerr et al., Citation2012). A multicentre photopatch test study on 1031 patients across 12 European countries indicated that this oil-soluble UV filter, along with OCR and benzophenone-3, was the most frequent sunscreen absorber that led to photoallergic contact dermatitis (Kerr et al., Citation2012). The reactive nature of BM-DBM has been reported by several groups, with Paris et al. (Citation2009) finding that UV irradiation of BM-DBM results in an increase in the keto isomer and reactivity with key biomolecules such as nucleosides, tryptophan and tyrosine. Similarly, Stiefel & Schwack (Citation2013) showed that the carbonyl group of BM-DBM and other related UV filters have a high tendency to form adducts with free amino acids or peptides, and suggest that such reactions can occur within the skin layer.
Ethylhexylmethoxycinnamate (EHMC) is a lipophilic UV filter with an absorption spectrum in UV-B that is used extensively in PCPs. EHMC has been the subject of significant debate regarding its accumulation in human tissue, and possible risks associated with potential endocrine-disrupting effects (Manová et al., Citation2015). Investigations on rats into the effects of exposure to high concentrations of EHMC showed a marked decrease in the levels of thyroid hormones, which could affect both reproductive and neurological development (Axelstad et al., Citation2011; Klammer et al., Citation2007). In addition to possible adverse effects of EHMC on human health, its widespread use in cosmetics has led to a ubiquitous presence in the aquatic environment. Although EHMC levels are typically low in treated water (0.01–0.1 µg l−1), they can be higher in lakes, rivers and coastal waters, and can be close to potentially harmful levels in untreated municipal water (up to 19 µg l−1) (Christen et al., Citation2011). An effect on the expression of genes involved in hormonal pathways in fish was observed at EHMC concentrations above 37.5 µg l−1, and histological changes were seen at 394 µg l−1 (Christen et al., Citation2011). Furthermore, EHMC at these higher concentrations may also have a harmful effect on insects, with > 100 µg l−1 affecting the invertebrate hormonal system by upregulating the ecdysone receptor, a key transcription factor (Ozáez et al., Citation2013).
Given the possible adverse effects of the different organic and inorganic UV filters currently used in sunscreen cosmetics, there is a clear need for new UV filters without undesirable effects.
Moisturizers
Water is absolutely critical for the flexibility, normal functioning, maturation and desquamation of the skin, especially its outer layers. Because of the proximity of the outer layers to the environment, this barrier is always under major desiccation stress. Dryness of the skin can lead to undesirable results such as itchiness with visible characteristics such as redness, flaky appearance, cracks and even fissures which reduce the elasticity of the skin (Choi et al., Citation2013). The ability of the skin to hold water is related to the stratum corneum (SC), which acts as a barrier against water loss. The water content of the SC ideally should be 20–35% – at a water content of less than 10% the skin has a rough and flaky appearance (Flynn et al., Citation2001). Given that a critical level of hydration is required to allow the SC to maintain its flexibility and normal functioning, cosmetic scientists have investigated chemical moisturizers that are able to improve the SC’s ability to retain water (Harding et al., Citation2000). Moisturizers are complex mixtures of chemical agents specially designed to make the external layers of skin hydrated and more pliable by attracting water and reducing water evaporation. These cosmetic products are therefore formulated to prevent transepidermal water loss and retain or increase the content of water in the SC (Rawlings et al., Citation2004).
Although the functional mechanism of this active cosmetic category is complicated, there are three basic properties which are consistent across all moisturizers: humectancy, occlusivity and/or emolliency. Humectants are moisturizer ingredients that attract and hold water in the outer layers of skin and act in two directions:
Attracting water from the dermis layer to the epidermis or SC (inside out).
Attracting moisture from the external environment to the skin (outside in).
One major drawback of humectants is that they increase transepidermal water loss by intensification of water absorption into the outer layers of skin where it can then be lost into the environment. To address this problem, humectants are always combined with an occlusive agent – ingredients such as petrolatum, mineral oil and vegetable waxes that form a film on the skin and prevent water loss (Kraft & Lynde, Citation2005).
Moisturizers can be considered as safe products in comparison to most traditional drugs which are used by dermatologists. However, inconvenient and undesirable skin reactions may be encountered through long-term usage of these products. It has been demonstrated that long-term treatment with moisturizers on normal skin increases skin susceptibility to irritants and also influences the barrier function of normal skin (Held et al., Citation1999; Buraczewska et al., Citation2007). In addition, it has been indicated that moisturizers are able to change the mRNA expression of certain epidermal genes (Buraczewska et al., Citation2009). Commonly used humectants in cosmetic moisturizers are glycerin, propylene glycol, sodium pyrrolidone carboxylic acid (sodium PCA) and urea (Rawlings et al., Citation2004). Whilst these are generally regarded as weak contact sensitizers and therefore safe to use in moisturizers, there is evidence that at high concentrations, or when used by people with dermatitis, these compounds can trigger adverse skin reactions (Zesch, Citation1982; Andersen, Citation1999; Lodén et al., Citation2002; Lessmann et al., Citation2005). There is therefore a demand for new moisturizers which use alternative, non-irritant ingredients (Lodén, Citation2003).
Potential of Cyanobacterial Metabolites as Sunscreens
Cyanobacteria represent a diverse group of photosynthetic bacteria – from tiny single cells to large filamentous forms, and have great potential for biotechnological applications in agriculture, mariculture, biomedicals, food and fuel (Singh et al., Citation2016). One of the most interesting properties of cyanobacteria, especially extremophilic species, is their ability to overcome the detrimental effects of UV radiation, and they have a number of avoidance, protection and repair mechanisms (). A key mechanism is the production of UV-absorbing/screening compounds such as mycosporine-like amino acids (MAAs) and scytonemin which serve as natural photo-protectants (Richa et al., Citation2011). In this section we summarize the biotechnological potential of MAAs and scytonemin synthesized by cyanobacteria.
Mycosporine-like amino acids (MAAs)
MAAs are a group of more than 20 water soluble, UV-absorbing metabolites providing screening protection against highly energetic photons in the UV-A wavelengths (Schmid et al., Citation2003). Strong protection is due to MAAs having extremely high molar extinction coefficients (Ɛ) (Ɛ = 28100–50000 l mol−1 cm−1) (Wada et al., Citation2013). MAAs are found in the cytoplasm and outer sheath of cyanobacteria and other algae such as dinoflagellates, where they act as filters to prevent UV-induced damage (Llewellyn & Airs, Citation2010; Carreto & Carignan, Citation2011; Wada et al., Citation2015). MAAs are low molecular weight (< 400 Da) colourless molecules with absorption maxima typically of 310–362 nm. All MAA molecules contain a central cyclohexanone or cyclohexenimine ring that is responsible for UV absorption. Their biosynthesis in cyanobacteria occurs via specific pathways such as the shikimate pathway and pentose phosphate pathway. The structure and absorption spectra of MAAs are due to variation in nitrogen substituents and attached side groups (; Singh, Citation2009).
Table 1. Molecular structure, maximum absorption wavelength and molar extinction coefficient of MAAs from cyanobacteria.
Different kinds of MAAs have been found in cyanobacterial species in a variety of habitats such as the marine environment (Liu et al., Citation2004), hypersaline ponds (Kedar et al., Citation2002), rice fields (Sinha et al., Citation2003) and deserts (Bowker et al., Citation2002), and further investigations in diverse habitats might reveal new MAAs. Recently, glycosylated MAAs have been isolated from the terrestrial cyanobacterium Nostoc commune (Matsui et al., Citation2011; Nazifi et al., Citation2013, Citation2015). One of these MAAs (absorption maximum 334 nm) was identified as a hexose-bound porphyra-334 derivative with a molecular mass of 508 Da, another (absorption maximum 322 nm) as a two hexose-bound palythine-threonine derivative with a molecular mass of 612 Da. It was suggested that these purified glycosylated MAAs could have multifunctional roles as sunscreens and antioxidants. Mycosporines extracted from the red alga Porphyra umbilicalis are employed in the sunscreen products Helioguard 365TM (Mibelle AG Biochemistry, Switzerland) and HelionoriTM (Biosil Technologies, France). In Helioguard 365TM, mycosporines encapsulated into liposomes reduce lipid peroxidation on human skin and improve skin firmness and smoothness, supporting its ability to protect against premature skin ageing (Soule & Garcia-Pichel, Citation2014). Also, MAAs used in HelionoriTM (mycosporine-palythine, porphyra-334 and shinorine) were shown to be photo-stable and heat-stable, with the ability to reduce sunburn and preserve membrane lipids when used at concentrations of 1–4% (Soule & Garcia-Pichel, Citation2014).
MAAs have great biotechnological potential as natural UV sunscreen products. Misonou et al. (Citation2003) studied the protective effects of MAAs against DNA damage and found that they were able to effectively block thymine dimer formation by UV radiation. Oyamada et al. (Citation2008) examined the protective effects of three different kinds of MAAs (shinorine, porphyra-334 and mycosporine-glycine) on human fibroblast cells and found that all three protected the cells from UV-induced cell death, with mycosporine-glycine having the strongest effect. They also found a promotional effect of MAAs on the proliferation of human skin fibroblast cells, and concluded that these secondary metabolites have potential application in cosmetics and toiletries.
In addition to their strong photo-protective capabilities, MAAs are also multifunctional secondary metabolites with various cellular functions. In extremophilic cyanobacteria, MAAs act as compatible solutes, able to boost cellular tolerance to desiccation, salt and heat stress (Singh, Citation2009). Furthermore, these metabolites are effective antioxidant molecules and are able to stabilize free radicals within their ring structure. For example, mycosporine-glycine is able to quench singlet oxygen and hydroxyl radicals before oxidative stress response genes and antioxidant enzymes are induced (Suh et al., Citation2003). Photo-ageing of the skin is often caused by free radicals which are produced upon exposure to UV radiation. Treatment of skin with MAAs counteracts the process of free radical production: application of a cream containing 0.005% Porphyra-334 encapsulated in liposomes significantly improved both firmness and smoothness of the skin after 4 weeks by 10% and 12%, respectively, comparable to creams containing synthetic UV filters (Daniel et al., Citation2004).
Studies have sought to understand the optimal cultivation conditions to maximize the production of MAAs in cyanobacteria. Treatment with blue light, UV-A and UV-B radiation has been shown to increase the induction of MAA synthesis (Riegger & Robinson, Citation1997). Furthermore a pterin UV-B specific photoreceptor has been proposed to mediate the photosensory induction of MAAs (Portwich & Garcia‐Pichel, Citation2000). In addition to UV radiation, other factors such as nutrient availability, salinity and temperature are known to affect accumulation of MAAs in the cell (Oren & Gunde-Cimerman, Citation2007). Surprisingly, Singh (Citation2009) indicated that MAA synthesis under photo-heterotrophic growth is higher than under photo-autotrophic growth because under photo-autotrophic conditions, light and CO2 can act as limiting factors. It was also shown that sulphur is essential for the synthesis of shinorine in Anabaena variabilis, with ammonium enhancing shinorine production and the absence of magnesium decreasing the synthesis of this MAA. The results of these studies show that the optimization of growth conditions can significantly increase the production of these biotechnologically important compounds in cyanobacteria. With such improvements and the development of industrial-scale cultivation technologies, these natural metabolites have the potential to supplement or replace commercially available sunscreens (Llewellyn & Airs, Citation2010; White et al., Citation2011).
The biosynthesis of MAAs has also been investigated and appears to occur via two routes. In the cyanobacterium Anabaena variabilis, five enzymes are involved in the synthesis of the MAA shinorine, through the shikimate pathway: a deoxy-D-arabino-heptulosonate phosphate synthase (DAHP synthase); a dehydroquinase synthase homologue (DHQS); an O-methyl-transferase (O-MT); an ATP grasp ligase and an NRPS-like enzyme (). The shinorine biosynthesis starts with the formation of arabino heptulosonate-7-phosphate, which is then transformed into the intermediate 4-deoxygadusol. In the next step, the combination of 4-deoxygadusol with a glycine molecule gives mycosporine-glycine and finally an NRPS-like enzyme condenses mycosporine-glycine and serine to shinorine (Wada et al., Citation2013; Mandal & Rath, Citation2014). However, Balskus & Walsh (Citation2010) reported a different MAA biosynthetic pathway in cyanobacteria. They found that biosynthesis of shinorine in Anabaena variabilis and Nostoc punctiforme via 4-deoxygadusol proceeds from sedoheptulose 7-phosphate (precursor of the pentose phosphate pathway), rather than deoxy-D-arabino-heptulosonate phosphate (). Biosynthesis of MAA through the pentose phosphate pathway was evidenced by cloning the EVS (2-epi-5-epi-valiolone synthase) biosynthetic gene cluster of A. variabilis into a heterologous host for functional identification of MAA biosynthetic enzymes (Balskus & Walsh, Citation2010). Spence et al. (Citation2012) examined the effect of deleting the EVS gene on MAA biosynthesis in A. variabilis and indicated that the EVS mutant of A. variabilis retained its ability to produce MAA on exposure to UV-A radiation. The authors proposed that functionally duplicate yet distinct convergent pathways exist for the biosynthesis of MAAs in cyanobacteria involving both the pentose phosphate pathway and the shikimate pathway to produce MAA via the convergent intermediate 4-deoxygadusol ().
Fig. 3. Two possible pathways for the biosynthesis of mycosporine-glycine in the cyanobacterium Anabaena variabilis. 4-deoxygadusol can be produced through either the shikimate pathway or the pentose pathway, and is then combined with a glycine molecule to produce mycosporine-glycine. PEP: phosphoenolpyruvate; E4P: erythrose-4-phosphate; DAHP: 2-keto-3-deoxy-D-arabinoheptulosonate-7-phosphate; DHQ: 3-dehydroquinate; 4-dG: 4-deoxygadusol; SP; sedoheptulose-7-phosphate; EV: 2-epi-5-epi-valiolone (re-drawn from Wada et al., Citation2013).
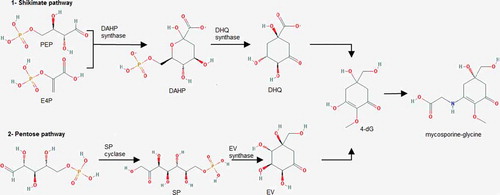
Scytonemin
Scytonemin has a maximum absorption at 380 nm, and is found in the extracellular polysaccharide sheath of cyanobacterial mats, crusts or colonies where it serves as a protectant against short wavelength solar UV radiation. This lipid-soluble pigment is a small dimeric molecule (544 Da) that is unique among natural products and is composed of indolic and phenolic subunits linked by an olefinic carbon atom (). Due to its large extinction coefficient (Ɛ = 250 l g−1 cm−1 at 384 nm), scytonemin is considered as an efficient photo-protective compound (Wada et al., Citation2013). There are two forms of scytonemin based on the redox and acid-base conditions during the extraction process: an oxidized form (green) named fuscochlorin and a reduced form (red) known as fuscorhodin ().
Scytonemin is synthesized from metabolites of aromatic amino acid (tryptophan and tyrosine) biosynthesis (). UV-A treatment efficiently induces synthesis of scytonemin, whereas blue, green or red light has no significant effect (Garcia‐Pichel & Castenholz, Citation1991). Other stress factors can also affect the synthesis of this alkaloid pigment in cyanobacteria. Dillon et al. (Citation2002) examined the effect of heat, osmotic and oxidative stress on the synthesis of scytonemin in Chroococcidiopsis sp. and found that an increase in both temperature and photo-oxidative conditions in conjunction with UV-A causes a synergic increase in the rate of production, whereas increased salt concentration inhibited synthesis. In contrast, Rath et al. (Citation2012) showed that salinity induced the production of scytonemin in Lyngbya aestuarii. Fleming & Castenholz (Citation2008) studied the effects of nitrogen source (N2, NO3− or NH4+) on scytonemin synthesis in Nostoc punctiforme, showing that this cyanobacterial species synthesized three to seven times more scytonemin while fixing nitrogen than when utilizing nitrate or ammonium. The exact mechanisms of scytonemin induction are unclear but multiple environmental signals may act to determine the level of this pigment in different species of cyanobacteria (Rastogi et al., Citation2015).
Fig. 5. Proposed biosynthesis pathway of scytonemin (Wada et al., Citation2013).
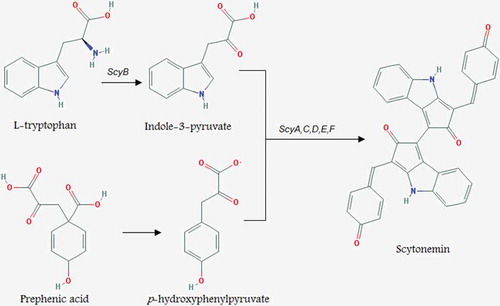
A study on Nostoc punctiforme by Soule et al. (Citation2007) revealed that an 18-gene cluster (ORFs: NpR1276–NpR1259) is associated with scytonemin production and NpR1273 was directly involved in the biosynthesis process. Also four putative genes, NpR1271 to NpR1274, were found to be responsible for the assembly of the scytonemin molecule. The transcription levels of all 18 genes were increased after 48 hours of UV-A exposure and all were co-transcribed as part of a single transcriptional unit (Soule et al., Citation2009). Recently, Malla & Sommer (Citation2014) generated the monomer moiety of scytonemin from tryptophan and tyrosine in Escherichia coli and discovered that only three enzymes are required for its in vivo production. The precise mechanism of scytonemin biosynthesis is still ambiguous, however, recent studies indicated that biosynthesis of this pigment in cyanobacteria is a highly conserved process (Rastogi et al., Citation2015). Wada et al. (Citation2013) proposed a pathway for scytonemin synthesis in cyanobacteria in which enzymes ScyA, B and C are assumed to catalyse the synthesis of scytonemin monomers, while ScyC, D and F are assumed to catalyse the dimerization of the subunits ().
Scytonemin is highly stable under different abiotic stressors and performs UV screening activity without any additional metabolic investment (Singh et al., Citation2010). A photo-protective role of scytonemin has been shown (Garcia‐Pichel et al., Citation1992; Dillon & Castenholz, Citation1999; Gao & Garcia-Pichel, Citation2011) and Garcia‐Pichel & Castenholz (Citation1991) reported that scytonemin prevents up to 90% of solar UV radiation from entering the cell. In addition, scytonemin has antioxidant activity and functions as a radical scavenger to prevent cellular damage resulting from reactive oxygen species produced upon UV-radiation exposure (Matsui et al., Citation2012; Rastogi et al., Citation2015). Scytonemin also represses proliferation of T-cell leukaemia Jurkat cells (IC50 = 7.8 µM) in humans (Singh et al., Citation2010). Given the potential of scytonemin in UV screening and free radical scavenging, together with its non-toxic properties, this highly stable pigment offers biotechnological opportunities for exploitation by the cosmetics industry (Stevenson et al., Citation2002; Rastogi & Sinha, Citation2009).
Potential of Cyanobacterial Metabolites as Moisturizers
Extremophilic species of cyanobacteria have protective mechanisms which enable them to survive inhospitable habitats such as hyper-arid deserts and salt environments. One of these mechanisms is the production of copious external polysaccharide layers in the form of protective sheaths, slimes or capsules. Cyanobacteria such as Chroococcidiopsis and Nostoc species are able to survive with minimal water by synthesizing both internal and external polysaccharides (Warren-Rhodes et al., Citation2013; Smith et al., Citation2014). Tamaru et al. (Citation2005) indicated that the desiccation tolerance of Nostoc commune is strongly associated with extracellular polymeric substances (EPS). They removed the EPS from N. commune cells by homogenizing colonies with a blender and filtering through a coarse filter paper, and showed that cells depleted in EPS were highly sensitive to desiccation. The hydrophilic/hydrophobic characteristics of EPS enable it to trap and accumulate water, and retard water loss during periods of desiccation (Tamaru et al., Citation2005). Li et al. (Citation2011) showed that Nostoc commune EPS has strong moisture-absorption and retention capacities compared with chitosan (a hygroscopic agent) and urea (a humectant). They found that after exposure to 43% relative humidity for 24 hours, the moisture absorption rate of EPS (10.1%) was much higher than that of chitosan (6.3%) and urea (5.8%).
Conventional humectant materials used in moisturizing creams, such as urea, glycerin and propylene glycol, have reasonable moisture absorption abilities but their moisture retention capacity is poor (Zhao et al., Citation2013). This leads to an increase in transepidermal water loss mitigated by occlusive agents that can have limitations such as undesirable odour, a greasy texture and potential allergenicity (Kraft & Lynde, Citation2005). A study by Li et al. (Citation2011) found that the moisture retention value of cyanobacterial EPS (28%) was much greater than that of urea (15.9%) and chitosan (7.3%) indicating that cyanobacterial EPS have great potential to be used as humectants in cosmetic moisturizers without the need to be combined with an occlusive agent. The moisture retention capacity of sacran, a giant polysaccharide extracted from the cyanobacterium Aphanothece sacrum, is 10-fold higher than hyaluronic acid. This jelly-like anionic polysaccharide consists of 11 types of monosaccharides with about 11% sulphate groups and 12% carboxyl groups per sugar chain (Okajima et al., Citation2008, Citation2009). It has been suggested that the strong moisture retention capacity of cyanobacterial EPS is due to strong interactions between water molecules and the hydrophilic –OH groups of the polysaccharides (Zhao et al., Citation2013).
Another characteristic which makes cyanobacterial EPS suitable for cosmetic application is its antioxidant activity. EPS is capable of scavenging both superoxide anions and hydroxyl radicals in vitro (Li et al., Citation2011). These compounds also can increase antioxidant enzyme activity, decrease lipid peroxidation and reduce oxidative damage induced by paraquat (Li et al., Citation2011). In addition, several reports have indicated that cyanobacterial polysaccharides may have antiviral activity. For instance, nostoflan, an acidic polysaccharide isolated from Nostoc flagelliforme, has been indicated to have antiviral activity against pathogenic viruses such as HSV-1, HSV-2, human cytomegalovirus and influenza A virus (Kanekiyo et al., Citation2005) through preventing the virus binding to the host cells (Kanekiyo et al., Citation2007, Citation2008).
Cyanobacterial species synthesize and secrete EPS that consist of hydrated molecules of high molecular mass. These are composed of an array of uronic acid (glucuronic and galacturonic acid) and neutral sugar (glucose, galactose, mannose, fructose, ribose, xylose, arabinose, fucose and rhamnose) residues (De Philippis & Vincenzini, Citation1998), as well as non-carbohydrate constituents such as phosphate, lactate, acetate and glycerol (Chakraborty & Pal, Citation2014). Furthermore, cyanobacterial EPS contain sulphate groups, a feature unique among bacteria (Whitton, Citation2012). Surprisingly, many cyanobacterial EPS are characterized by a significant level of hydrophobicity because of the presence of some hydrophobic groups such as ester-linked acetyl groups, peptidic moieties and deoxyhexoses (Chakraborty & Pal, Citation2014). The composition of EPS in some species is labile and can be affected by environmental changes. For instance, Caiola et al. (Citation1996) reported that Chroococcidiopsis cells have the ability to modify their envelope composition in response to water stress. They showed that desiccated cultures of these species have a complex envelope formed of sporopollenin-like compounds, acid sulphated and beta-linked polysaccharides, lipids and proteins which all are part of a very elaborate structure that allows the cyanobacterium to lose water slowly and in a controlled way.
The genetics of EPS biosynthesis in cyanobacteria have not been thoroughly examined but Pereira et al. (Citation2009) proposed that genes related to EPS synthesis can be divided into three classes: (1) those encoding the enzymes involved in the biosynthetic pathways of nucleotide sugars or other components (rfb ABCD genes); (2) those coding for the glycosyl transferases which have not been thoroughly characterized; and (3) those required for the oligosaccharide or polysaccharide processing (wz genes).
Several environmental factors influence the expression of genes related to EPS production. In many species of cyanobacteria, nutrient deficiency (nitrogen and phosphorus) enhances EPS production rates as a consequence of an excess of photo-assimilated carbon, and excretion acts as an overflow mechanism to avoid damage to the photosynthetic apparatus (Chakraborty & Pal, Citation2014). EPS production rates in cyanobacterial species can be affected by other factors such as salt stress, irradiance, light cycle, temperature and culture age (De Philippis & Vincenzini, Citation1998). Culture age can also affect sugar composition, both qualitatively and quantitatively (Gloaguen et al., Citation1995). Therefore, the production rate of EPS in cyanobacterial species could be increased either through genetic manipulation, or through the selective use of physiological conditions.
Conclusions and Future Perspectives
The biotechnological exploitation of cyanobacteria as a source of bioactive compounds for the cosmetic industry represents a promising area of research. This group of microorganisms produce UV absorbing/screening compounds such as MAAs and scytonemin that serve as natural photoprotectants and can be suitable alternatives to currently used synthetic UV filters. Also, some extremophilic species of cyanobacteria living in hyper-arid environments produce copious amount of external polysaccharide layers that act as water/moisture absorbers and are potent candidates to be used as moisturizers in skin products. However, whilst cyanobacterial compounds have attractive properties for the cosmetic industry, the key bottleneck is the economic and sustainable production of these bio-compounds at the large scales required by the industry. On-going research into the intensive cultivation of photosynthetic microorganisms in photobioreactors such as the tubular system shown in , is bringing new understanding in terms of design, operation and scale-up (Fernandes et al., Citation2015), and will steadily improve both the economics and feasibility of industrial production of cyanobacteria. Furthermore, the discovery and characterization of superior cyanobacterial strains from the environment, together with their domestication and metabolic improvement using modern genetic engineering approaches (Lai & Lan, Citation2015), have the potential to bring significant improvements in productivity. Such technical improvements coupled to market demand should see the increasing application of cyanobacterial metabolites in the cosmetics sector.
Acknowledgements
Research on cyanobacteria in SP’s lab is funded by grant BB/F016948/1 from the UK’s Biotechnology and Biological Sciences Research Council (BBSRC). CAL’s research on UV sunscreens was supported by BBSRC grant BB/E01898X/1.
Disclosure Statement
No potential conflict of interest was reported by the author(s).
Additional information
Funding
References
- Andersen, F.A. (1999). Final safety assessment for PCA and sodium PCA. International Journal of Toxicology, 18: 25–34.
- Avenel-Audran, M., Dutartre, H., Goossens, A., Jeanmougin, M., Comte, C., Bernier, C., Benkalfate, L., Michel, M., Ferrier-Lebouedec, M.C., Vigan, M., Bourrain, J.L., Outtas, O., Peyron, J.L. & Martin, L. (2010). Octocrylene, an emerging photoallergen. Archives of Dermatology, 146: 753–757.
- Axelstad, M., Boberg, J., Hougaard, K.S., Christiansen, S., Jacobsen, P.R., Mandrup, K.R., Nellemann, C., Lund, S.P. & Hass, U. (2011). Effects of pre-and postnatal exposure to the UV-filter octylmethoxycinnamate (OMC) on the reproductive, auditory and neurological development of rat offspring. Toxicology and Applied Pharmacology, 250: 278–290.
- Baker, T.J., Tyler, C.R. & Galloway, T.S. (2014). Impacts of metal and metal oxide nanoparticles on marine organisms. Environmental Pollution, 186: 257–271. doi: 10.1016/j.envpol.2013.11.014.
- Balskus, E.P. & Walsh, C.T. (2010). The genetic and molecular basis for sunscreen biosynthesis in cyanobacteria. Science, 329: 1653–1656.
- Bowker, M.A., Reed, S.C., Belnap, J. & Phillips, S.L. (2002).Temporal variation in community composition, pigmentation, and Fv/Fm of desert cyanobacterial soil crusts. Microbial Ecology, 43: 13–25.
- Budden, T. & Bowden, N.A. (2013). The role of altered nucleotide excision repair and UVB-induced DNA damage in melanomagenesis. International Journal of Molecular Sciences, 14: 1132–1151.
- Buraczewska, I., Berne, B., Lindberg, M., Törmä, H. & Lodén, M. (2007). Changes in skin barrier function following long‐term treatment with moisturizers, a randomized controlled trial. British Journal of Dermatology, 156: 492–498.
- Buraczewska, I., Berne, B., Lindberg, M., Lodén, M. & Törmä, H. (2009). Long-term treatment with moisturizers affects the mRNA levels of genes involved in keratinocyte differentiation and desquamation. Archives of Dermatological Research, 301: 175–181.
- Caiola, M.G., Billi, D. & Friedmann, E.I. (1996). Effect of desiccation on envelopes of the cyanobacterium Chroococcidiopsis sp. (Chroococcales). European Journal of Phycology, 31: 97–105.
- Carreto, J.I. & Carignan, M.O. (2011). Mycosporine-like amino acids: relevant secondary metabolites. Chemical and ecological aspects. Marine Drugs, 9: 387–446.
- Chakraborty, T. & Pal, R. (2014). An overview of cyanobacterial exopolysaccharides: features, composition and effects of stress exposure. International Journal of Life Sciences, 8: 1–9.
- Choi, J.W., Kwon, S.H., Huh, C.H., Park, K.C. & Youn, S.W. (2013). The influences of skin visco‐elasticity, hydration level and aging on the formation of wrinkles: a comprehensive and objective approach. Skin Research and Technology, 19: e349–e355.
- Christen, V., Zucchi, S. & Fent, K. (2011). Effects of the UV-filter 2-ethyl-hexyl-4-trimethoxycinnamate (EHMC) on expression of genes involved in hormonal pathways in fathead minnows (Pimephales promelas) and link to vitellogenin induction and histology. Aquatic Toxicology, 102: 167–176.
- Cross, R.K., Tyler, C. & Galloway, T.S. (2015). Transformations that affect fate, form and bioavailability of inorganic nanoparticles in aquatic sediments. Environmental Chemistry, 12: 627–642.
- Daniel, S., Cornelia, S. & Fred, Z. (2004). UV-A sunscreen from red algae for protection against premature skin aging. Cosmetics and Toiletries Manufacture Worldwide, 204: 139–143.
- De Philippis, R. & Vincenzini, M. (1998). Exocellular polysaccharides from cyanobacteria and their possible applications. FEMS Microbiology Reviews, 22: 151–175.
- Deng, X., Luan, Q., Chen, W., Wang, Y., Wu, M., Zhang, H. & Jiao, Z. (2009). Nanosized zinc oxide particles induce neural stem cell apoptosis. Nanotechnology, 20: 101–115.
- Dillon, J.G. & Castenholz, R.W. (1999). Scytonemin, a cyanobacterial sheath pigment, protects against UVC radiation: implications for early photosynthetic life. Journal of Phycology, 35: 673–681.
- Dillon, J.G., Tatsumi, C.M., Tandingan, P.G. & Castenholz, R.W. (2002). Effect of environmental factors on the synthesis of scytonemin, a UV-screening pigment, in a cyanobacterium (Chroococcidiopsis sp.). Archives of Microbiology, 177: 322–331.
- Fernandes, B.D., Mota, A., Teixeira, J.A. & Vicente, A.A. (2015). Continuous cultivation of photosynthetic microorganisms: approaches, applications and future trends. Biotechnological Advances, 33: 1228–1245.
- Fleming, E.D. & Castenholz, R.W. (2007). Effects of periodic desiccation on the synthesis of the UV‐screening compound, scytonemin, in cyanobacteria. Environmental Microbiology, 9: 1448–1455.
- Fleming, E.D. & Castenholz, R.W. (2008). Effects of nitrogen source on the synthesis of the UV-screening compound, scytonemin, in the cyanobacterium Nostoc punctiforme PCC 73102. FEMS Microbiology Ecology, 63: 301–308.
- Flynn, T.C., Petros, J., Clark, R.E. & Viehman, G.E. (2001). Dry skin and moisturizers. Clinics in Dermatology, 19: 387–392.
- Gao, Q. & Garcia-Pichel, F. (2011). Microbial ultraviolet sunscreens. Nature Reviews Microbiology, 9: 791–802.
- Garcia‐Pichel, F. & Castenholz, R.W. (1991). Characterization and biological implications of scytonemin, a cyanobacterial sheath pigment. Journal of Phycology, 27: 395–409.
- Garcia‐Pichel, F., Sherry, N.D. & Castenholz, R.W. (1992). Evidence for an ultraviolet sunscreen role of the extracellular pigment scytonemin in the terrestrial cyanobacterium Chlorogloeopsis sp. Photochemistry and Photobiology, 56: 17–23.
- Gloaguen, V., Morvan, H. & Hoffmann, L. (1995). Released and capsular polysaccharides of Oscillatoriaceae (Cyanophyceae, Cyanobacteria). Algological Studies, 78: 53–69.
- Harding, C.R., Watkinson, A., Rawlings, A.V. & Scott, I.R. (2000). Dry skin, moisturization and corneodesmolysis. International Journal of Cosmetic Science, 22: 21–52.
- Held, E., Sveinsdóttir, S. & Agner, T. (1999). Effect of long-term use of moisturizer on skin hydration, barrier function and susceptibility to irritants. Acta Dermato-Venereologica, 79: 49–51.
- Hexsel, C.L., Bangert, S.D., Hebert, A.A. & Lim, H.W. (2008). Current sunscreen issues: 2007 Food and Drug Administration sunscreen labelling recommendations and combination sunscreen/insect repellent products. Journal of the American Academy of Dermatology, 59: 316–323.
- Hupel, M., Poupart, N. & Gall, E.A. (2011). Development of a new in vitro method to evaluate the photoprotective sunscreen activity of plant extracts against high UV-B radiation. Talanta, 86: 362–371.
- Ichihashi, M., Ueda, M., Budiyanto, A., Bito, T., Oka, M., Fukunaga, M., Tsuru, K. & Horikawa, T. (2003). UV-induced skin damage. Toxicology, 189: 21–39.
- Kanekiyo, K., Lee, J. B., Hayashi, K., Takenaka, H., Hayakawa, Y., Endo, S. & Hayashi, T. (2005). Isolation of an antiviral polysaccharide, Nostoflan, from a terrestrial cyanobacterium, Nostoc flagelliforme. Journal of Natural Products, 68: 1037–1041.
- Kanekiyo, K., Hayashi, K., Takenaka, H., Lee, J. B. & Hayashi, T. (2007). Anti-herpes simplex virus target of an acidic polysaccharide, Nostoflan, from the edible blue-green alga Nostoc flagelliforme. Biological and Pharmaceutical Bulletin, 30: 1573–1575.
- Kanekiyo, K., Hayashi, K., Lee, J.B., Takenaka, H. & Hayashi, T. (2008). Structure and antiviral activity of an acidic polysaccharide from an edible blue-green alga, Nostoc flagelliforme. Yakugaku zasshi: Journal of the Pharmaceutical Society of Japan, 128: 725–731.
- Karlsson, I., VandenBroecke, K., Mårtensson, J., Goossens, A. & Börje, A. (2011). Clinical and experimental studies of octocrylene’s allergenic potency. Contact Dermatitis, 64: 343–352.
- Kedar, L., Kashman, Y. & Oren, A. (2002). Mycosporine-2-glycine is the major mycosporine-like amino acid in a unicellular cyanobacterium (Euhalothece sp.) isolated from a gypsum crust in a hypersaline saltern pond. FEMS Microbiology Letters, 208: 233–237.
- Kerr, A.C., Ferguson, J., Haylett, A.K., Rhodes, L.E., Adamski, H., Alomar, A., Serra, E., Antoniou, C., Aubin, F., Vigan, M., Biedermann, T., Fischer, J., Bourrain, J.L., Bruynzeel, D., Rustemeyer, T., Carrascosa, J.M., Chowdhury, M.M., de la Cuadra, J., Foti, C., Gardeazabal, J., Gawkrodger, D.J., Gimenez-Arnau, A., Goncalo, M., Goossens, A., Lecha, M., Marguery, M.C., Neumann, N.J., Niklasson, B., Peyron, J.L., Pigatto, P., Spiewak, R., Stone, N., Tanew, A., Thomas, P., Bonnevalle, A., Wilkinson, S.M., Wolf, P., Gruber-Wackernagel, A. & Wulf, H.C. (2012). A European multi-centre photopatch test study (EMCPPTS). British Journal of Dermatology, 166: 1002–1009.
- Klammer, H., Schlecht, C., Wuttke, W., Schmutzler, C., Gotthardt, I., Köhrle, J. & Jarry, H. (2007). Effects of a 5-day treatment with the UV-filter octyl-methoxycinnamate (OMC) on the function of the hypothalamo-pituitary–thyroid function in rats. Toxicology, 238: 192–199.
- Kligman, A.M. (2000). Cosmetics: a dermatologist looks to the future: promises and problems. Dermatologic Clinics, 18: 699–709.
- Kole, P.L., Jadhav, H.R., Thakurdesai, P. & Nagappa, A.N. (2005). Cosmetic potential of herbal extracts. Natural Product Radiance, 4: 315–321.
- Kraft, J.N. & Lynde, C.W. (2005). Moisturizers: what they are and a practical approach to product selection. Skin Therapy Letters, 10: 1–8.
- Lai, J.C., Lai, M.B., Jandhyam, S., Dukhande, V.V., Bhushan, A., Daniels, C.K. & Leung, S.W. (2008). Exposure to titanium dioxide and other metallic oxide nanoparticles induces cytotoxicity on human neural cells and fibroblasts. International Journal of Nanomedicine, 3: 533–545.
- Lai, M.C. & Lan, E.I. (2015). Advances in metabolic engineering of cyanobacteria for photosynthetic biochemical production. Metabolites, 5: 636–658.
- Lessmann, H., Schnuch, A., Geier, J. & Uter, W. (2005). Skin‐sensitizing and irritant properties of propylene glycol. Contact Dermatitis, 53: 247–259.
- Li, H., Xu, J., Liu, Y., Ai, S., Qin, F., Li, Z., Zhang, H. & Huang, Z. (2011). Antioxidant and moisture-retention activities of the polysaccharide from Nostoc commune. Carbohydrate Polymers, 83: 1821–1827.
- Lisby, S., Gniadecki, R. & Wulf, H.C. (2005). UV‐induced DNA damage in human keratinocytes: quantitation and correlation with long‐term survival. Experimental Dermatology, 14: 349–355.
- Liu, Z., Häder, D.P. & Sommaruga, R. (2004). Occurrence of mycosporine-like amino acids (MAAs) in the bloom-forming cyanobacterium Microcystis aeruginosa. Journal of Plankton Research, 26: 963–966.
- Llewellyn, C.A. & Airs, R.L. (2010). Distribution and abundance of MAAs in 33 species of microalgae across 13 classes. Marine Drugs, 8: 1273–1291.
- Lodén, M. (2003). Do moisturizers work? Journal of Cosmetic Dermatology, 2: 141–149.
- Lodén, M., Andersson, A.C., Anderson, C., Bergbrant, I.M., Frödin, T., Ohman, H., Sandström, M.H., Särnhult, T., Voog, E., Stenberg, B., Pawlik, E., Preisler-Häggqvist, A., Svensson, A. & Lindberg, M. (2002). A double-blind study comparing the effect of glycerin and urea. Acta Dermato-Venereologica, 82: 45–47.
- Lu, Y.P., Lou, Y.R., Xie, J.G., Peng, Q., Shih, W.J., Lin, Y. & Conney, A.H. (2009). Tumorigenic effect of some commonly used moisturizing creams when applied topically to UVB-pretreated high-risk mice. Journal of Investigative Dermatology, 129: 468–475.
- Mahajan, U. & Wasule, D. (2008). Sunscreen and anti-oxidant activities of herbal gel formulations. Pharmacognosy Magazine, 4: 99–101.
- Malla, S. & Sommer, M.O. (2014). A sustainable route to produce the scytonemin precursor using Escherichia coli. Green Chemistry, 16: 3255–3265.
- Mandal, S. & Rath, J. (2014). Extremophilic Cyanobacteria for Novel Drug Development. Springer, Dordrecht.
- Manová, E., von Goetz, N., Hauri, U., Bogdal, C. & Hungerbühler, K. (2013). Organic UV filters in personal care products in Switzerland: a survey of occurrence and concentrations. International Journal of Hygiene and Environmental Health, 216: 508–514.
- Manová, E., von Goetz, N. & Hungerbuehler, K. (2015). Aggregate consumer exposure to UV filter ethylhexyl methoxycinnamate via personal care products. Environment International, 74: 249–257.
- Matsui, K., Nazifi, E., Kunita, S., Wada, N., Matsugo, S. & Sakamoto, T. (2011). Novel glycosylated mycosporine-like amino acids with radical scavenging activity from the cyanobacterium Nostoc commune. Journal of Photochemistry and Photobiology B: Biology, 105: 81–89.
- Matsui, K., Nazifi, E., Hirai, Y., Wada, N., Matsugo, S. & Sakamoto, T. (2012). The cyanobacterial UV-absorbing pigment scytonemin displays radical-scavenging activity. Journal of General and Applied Microbiology, 58: 137–144.
- Misonou, T., Saitoh, J., Oshiba, S., Tokitomo, Y., Maegawa, M., Inoue, Y., Hori, H. & Sakurai, T. (2003). UV-absorbing substance in the red alga Porphyra yezoensis (Bangiales, Rhodophyta) block thymine photodimer production. Marine Biotechnology, 5: 194–200.
- Nazifi, E., Wada, N., Yamaba, M., Asano, T., Nishiuchi, T., Matsugo, S. & Sakamoto, T. (2013). Glycosylated porphyra-334 and palythine-threonine from the terrestrial cyanobacterium Nostoc commune. Marine Drugs, 11: 3124–3154.
- Nazifi, E., Wada, N., Asano, T., Nishiuchi, T., Iwamuro, Y., Chinaka, S., Matsugo, S. & Sakamoto, T. (2015). Characterization of the chemical diversity of glycosylated mycosporine-like amino acids in the terrestrial cyanobacterium Nostoc commune. Journal of Photochemistry and Photobiology B: Biology, 142: 154–168.
- Nohynek, G.J., Dufour, E.K. & Roberts, M.S. (2008). Nanotechnology, cosmetics and the skin: is there a health risk? Skin Pharmacology and Physiology, 21: 136–149.
- Okajima, M.K., Bamba, T., Kaneso, Y., Hirata, K., Fukusaki, E., Kajiyama, S.I. & Kaneko, T. (2008). Supergiant ampholytic sugar chains with imbalanced charge ratio form saline ultra-absorbent hydrogels. Macromolecules, 41: 4061–4064.
- Okajima, M.K., Miyazato, S. & Kaneko, T. (2009). Cyanobacterial megamolecule sacran efficiently forms LC gels with very heavy metal ions. Langmuir, 25: 8526–8531.
- Oren, A. & Gunde-Cimerman, N. (2007). Mycosporines and mycosporine-like amino acids: UV protectants or multipurpose secondary metabolites? FEMS Microbiology Letters, 269: 1–10.
- Orton, D.I. & Wilkinson, J.D. (2004). Cosmetic allergy. American Journal of Clinical Dermatology, 5: 327–337.
- Oyamada, C., Kaneniwa, M., Ebitani, K., Murata, M. & Ishihara, K. (2008). Mycosporine-like amino acids extracted from scallop (Patinopecten yessoensis) ovaries: UV protection and growth stimulation activities on human cells. Marine Biotechnology, 10: 141–150.
- Ozáez, I., Martínez-Guitarte, J.L. & Morcillo, G. (2013). Effects of in vivo exposure to UV filters (4-MBC, OMC, BP-3, 4-HB, OC, OD-PABA) on endocrine signaling genes in the insect Chironomus riparius. Science of the Total Environment, 456: 120–126.
- Pan, Z., Lee, W., Slutsky, L., Clark, R.A., Pernodet, N. & Rafailovich, M.H. (2009). Adverse effects of titanium dioxide nanoparticles on human dermal fibroblasts and how to protect cells. Small, 5: 511–520.
- Paris, C., Lhiaubet‐Vallet, V., Jiménez, O., Trullas, C. & Miranda, M.A. (2009). A blocked diketo form of avobenzone: photostability, photosensitizing properties and triplet quenching by a triazine‐derived UVB‐filter. Photochemistry and Photobiology, 85: 178–184.
- Pereira, S., Zille, A., Micheletti, E., Moradas-Ferreira, P., De Philippis, R. & Tamagnini, P. (2009). Complexity of cyanobacterial exopolysaccharides: composition, structures, inducing factors and putative genes involved in their biosynthesis and assembly. FEMS Microbiology Reviews, 33: 917–941.
- Portwich, A. & Garcia‐Pichel, F. (2000). A novel prokaryotic UVB photoreceptor in the cyanobacterium Chlorogloeopsis PCC 6912. Photochemistry and Photobiology, 71: 493–498.
- Quintana, N., Van der Kooy, F., Van de Rhee, M.D., Voshol, G.P. & Verpoorte, R. (2011). Renewable energy from Cyanobacteria: energy production optimization by metabolic pathway engineering. Applied Microbiology and Biotechnology, 91: 471–490.
- Rastogi, R.P. & Sinha, R.P. (2009). Biotechnological and industrial significance of cyanobacterial secondary metabolites. Biotechnology Advances, 27: 521–539.
- Rastogi, R.P., Sonani, R.R. & Madamwar, D. (2015). Cyanobacterial sunscreen scytonemin: role in photoprotection and biomedical research. Applied Biochemistry and Biotechnology, 176: 1551–1563.
- Rath, J., Mandal, S. & Adhikary, S.P. (2012). Salinity induced synthesis of UV-screening compound scytonemin in the cyanobacterium Lyngbya aestuarii. Journal of Photochemistry and Photobiology B: Biology, 115: 5–8.
- Rawlings, A.V., Canestrari, D.A. & Dobkowski, B. (2004). Moisturizer technology versus clinical performance. Dermatologic Therapy, 17: 49–56.
- Richa, R.P., Rastogi, S., Kumari, K.L., Singh, V.K., Kannaujiya, G., Singh, M. & Kesheri, R.P. (2011). Biotechnological potential of mycosporine-like amino acids and phycobiliproteins of cyanobacterial origin. Biotechnology and Bioengineering, 1: 159–171.
- Riegger, L. & Robinson, D. (1997). Photoinduction of UV-absorbing compounds in Antarctic diatoms and Phaeocystis antarctica. Marine Ecology Progress Series, 160: 13–25.
- Schaumann, G.E., Philippe, A, Bundschuh, M., Metreveli, G., Klitzke, S., Rakcheev, D., Grün, A., Kumahor, S.K., Kühn, M., Baumann, T., Lang, F., Manz, W., Schulz, R. & Vogel, H.J. (2015). Understanding the fate and biological effects of Ag- and TiO2-nanoparticles in the environment: the quest for advanced analytics and interdisciplinary concepts. Science of the Total Environment, 535: 3–19 (SI).
- Schlumpf, M., Kypke, K., Wittassek, M., Angerer, J., Mascher, H., Mascher, D., Vokt, C., Birchler, M. & Lichtensteiger, W. (2010). Exposure patterns of UV filters, fragrances, parabens, phthalates, organochlor pesticides, PBDEs, and PCBs in human milk: correlation of UV filters with use of cosmetics. Chemosphere, 81: 1171–1183.
- Schmid, D., Schürch, C., Zülli, F., Nissen, H.P. & Prieur, H. (2003). Mycosporine-like amino acids: natural UV-screening compounds from red algae to protect the skin against photoaging. SöFW Journal, 129: 38–42.
- Serpone, N., Dondi, D. & Albini, A. (2007). Inorganic and organic UV filters: their role and efficacy in sunscreens and suncare products. Inorganica Chimica Acta, 360: 794–802.
- Singh, J.S., Kumar, A., Rai, A.N. & Singh, D.P. (2016). Cyanobacteria: a precious bio-resource in agriculture, ecosystem, and environmental sustainability. Frontiers in Microbiology, 7: 529.
- Singh, S.P. (2009). Study on mycosporine-like amino acids (MAAs) in cyanobacteria: a biochemical, bioinformatics and molecular biology approach. Doctoral Thesis, Friedrich-Alexander-Universität Erlangen-Nürnberg, Germany.
- Singh, S.P., Kumari, S., Rastogi, R.P., Sinha, R. & Sinha, R.P. (2010). Photoprotective and biotechnological potentials of cyanobacterial sheath pigment, scytonemin. African Journal of Biotechnology, 9: 580–588.
- Sinha, R.P., Ambasht, N.K., Sinha, J.P. & Häder, D.P. (2003). Wavelength-dependent induction of a mycosporine-like amino acid in a rice-field cyanobacterium, Nostoc commune: role of inhibitors and salt stress. Photochemical and Photobiological Sciences, 2: 171–176.
- Smith, H.D., Baqué, M., Duncan, A.G., Lloyd, C.R., McKay, C.P. & Billi, D. (2014). Comparative analysis of cyanobacteria inhabiting rocks with different light transmittance in the Mojave Desert: a Mars terrestrial analogue. International Journal of Astrobiology, 13: 271–277.
- Soule, T. & Garcia‐Pichel, F. (2014). Ultraviolet photoprotective compounds from cyanobacteria in biomedical applications. In Cyanobacteria: An Economic Perspective (Sharma, N.K., Rai, A.K. & Stal, L.J.), 119–143. John Wiley & Sons, Chichester.
- Soule, T., Stout, V., Swingley, W.D., Meeks, J.C. & Garcia-Pichel, F. (2007). Molecular genetics and genomic analysis of scytonemin biosynthesis in Nostoc punctiforme ATCC 29133. Journal of Bacteriology, 189: 4465–4472.
- Soule, T., Garcia-Pichel, F. & Stout, V. (2009). Gene expression patterns associated with the biosynthesis of the sunscreen scytonemin in Nostoc punctiforme ATCC 29133 in response to UVA radiation. Journal of Bacteriology, 191: 4639–4646.
- Spence, E., Dunlap, W.C., Shick, J.M. & Long, P.F. (2012). Redundant pathways of sunscreen biosynthesis in a cyanobacterium. ChemBioChem, 13: 531–533.
- Stevenson, C.S., Capper, E.A., Roshak, A.K., Marquez, B., Eichman, C., Jackson, J.R., Mattern, M., Gerwick, W.H., Jacobs, R.S. & Marshall, L.A. (2002). The identification and characterization of the marine natural product scytonemin as a novel antiproliferative pharmacophore. Journal of Pharmacology and Experimental Therapeutics, 303: 858–866.
- Stiefel, C. & Schwack, W. (2013). Rapid screening method to study the reactivity of UV filter substances towards skin proteins by high‐performance thin‐layer chromatography. International Journal of Cosmetic Science, 35: 588–599.
- Suh, H.J., Lee, H.W. & Jung, J. (2003). Mycosporine glycine protects biological systems against photodynamic damage by quenching singlet oxygen with a high efficiency. Photochemistry and Photobiology, 78: 109–113.
- Tamaru, Y., Takani, Y., Yoshida, T. & Sakamoto, T. (2005). Crucial role of extracellular polysaccharides in desiccation and freezing tolerance in the terrestrial cyanobacterium Nostoc commune. Applied and Environmental Microbiology, 71: 7327–7333.
- Wada, N., Sakamoto, T. & Matsugo, S. (2013). Multiple roles of photosynthetic and sunscreen pigments in cyanobacteria focusing on the oxidative stress. Metabolites, 3: 463–483.
- Wada, N., Sakamoto, T. & Matsugo, S. (2015). Mycosporine-like amino acids and their derivatives as natural antioxidants. Antioxidants, 4: 603–646.
- Warren‐Rhodes, K.A., McKay, C.P., Boyle, L.N., Wing, M.R., Kiekebusch, E.M., Cowan, D.A., Stomeo, F., Pointing, S.B., Kaseke, K.F., Eckardt, F., Henschel, J.R., Anisfeld, A., Seely, M. & Rhodes, K.L. (2013). Physical ecology of hypolithic communities in the central Namib Desert: the role of fog, rain, rock habitat, and light. Journal of Geophysical Research: Biogeosciences, 118: 1451–1460.
- White, D.A., Polimene, L. & Llewellyn, C.A. (2011). Effects of ultraviolet‐A radiation and nutrient availability on the cellular composition of photoprotective compounds in Glenodinium foliaceum (Dinophyceae). Journal of Phycology, 47: 1078–1088.
- Whitton, B.A. (2012). Ecology of Cyanobacteria II. Their Diversity in Space and Time. Springer, Dordrecht.
- Witcover, J., Yeh, S. & Sperling, D. (2013). Policy options to address global land use change from biofuels. Energy Policy, 56: 63–74.
- Wolf, R., Wolf, D., Tüzün, B. & Tüzün, Y. (2001). Cosmetics and contact dermatitis. Dermatologic Therapy, 14:181–187.
- Zhao, L., Fan, F., Wang, P. & Jiang, X. (2013). Culture medium optimization of a new bacterial extracellular polysaccharide with excellent moisture retention activity. Applied Microbiology and Biotechnology, 97: 2841–2850.
- Zesch, A. (1982). Skin irritation by topical drugs. Dermatosen in Beruf und Umwelt. Occupation and Environment, 31: 74–78.