Abstract
The invasiveness of algal species can be facilitated by chemo-ecological traits that allow the establishment of invasive species in a highly competitive environment. Anti-bacterial, anti-quorum sensing, anti-diatom and anti-larval properties of the invasive brown macroalga Sargassum muticum and three native Sargassum species from Oman waters were compared in laboratory and field experiments to assess whether these traits have the potential to facilitate the invasion process. Only the extract of S. muticum inhibited bacterial growth of four marine bacterial strains and quorum sensing in the reporter strain Chromobacterium violaceum CV017. Settlement, growth and survival of the diatom Cylindrotheca closterium and larvae of the bryozoan Bugula neritina were significantly inhibited by all Sargassum extracts in laboratory experiments. However, crude extracts of S. muticum had the strongest antifouling effect. Natural tissue-level concentrations of S. muticum extract reduced diatom density to about 20% compared with the controls. Larval mortality increased by 80–90% compared with controls with S. muticum extract diluted to one-third natural levels. Significant anti-diatom activity of S. muticum was confirmed in the field experiments with Sargassum extracts embedded in a phytagel matrix. Comparison of non-polar compounds by gas chromatography–mass spectrometry demonstrated that S. muticum extracts had overall fewer secondary metabolites but more species-unique compounds than extracts of native Sargassum spp. The greater antifouling defence of invasive vs. native Sargassum species indicates a selective trait that may contribute to the invasion success of S. muticum.
Introduction
All submerged natural and artificial marine substrata are quickly colonized by micro- and macroorganisms in a process known as biofouling (Flemming, Citation2009). Fouling is a successive process that involves bacterial attachment to a substratum as the first step. This is followed by diatoms and other microorganisms colonizing the submerged substratum. After several weeks a well-developed biofilm has established, allowing invertebrate larvae and algal spores to settle and form macrofouling communities (reviewed by Salta et al., Citation2013).
The process of biofouling on the surface of marine organisms is known as epibiosis (Wahl, Citation1989). It can have various adverse effects on marine invertebrates and macroalgae. Surfaces of macroalgae should be clean from epibionts in order to allow photosynthesis, gas exchange and nutrient uptake (Rohde et al., Citation2008; Wahl et al., Citation2012). Epibiotic bacteria, fungi and microalgae can induce disease and tissue necrosis of macroalgae (Bouarab et al., Citation2001; Fernandes et al., Citation2011). Microbial biofilms are also known as important sources of chemical settlement cues for algal spores and invertebrate larvae (Qian et al., Citation2007; Hadfield, Citation2011; Mieszkin et al., Citation2013). This process can promote the subsequent colonization of macrofoulers, which in turn might impair the basibiont’s ability to exchange gases and nutrients, decrease growth rates by lowering photosynthesis (reviewed by Wahl et al., Citation2012), or lead to damage due to grazers preying on epibionts (i.e. ‘shared doom’ effect, Dixon et al., Citation1981).
In order to reduce fouling, macroalgae have evolved a wide variety of chemical defence mechanisms (Henrikson & Pawlik, Citation1995; Wahl, Citation2008; Hellio et al., Citation2009). Macroalgae can control epibiosis on their surfaces directly by excretion of antifouling compounds or indirectly by controlling the composition of microorganisms on their surfaces (Dobretsov & Qian, Citation2002; Dobretsov et al., Citation2006). Numerous active metabolites of different algal species are reported to have antibacterial (Culioli et al., Citation2008; Plouguerné et al., Citation2010b; Pérez et al., Citation2016), anti-algal (Plouguerné et al., Citation2010a; Cen-Pacheco et al., Citation2015), anti-invertebrate (Barbosa et al., Citation2007; da Gama et al. Citation2008; Plouguerné et al., Citation2010a), anti-larval (Culioli et al., Citation2008; Cho, Citation2013) and anti-quorum sensing properties (Dobretsov et al., Citation2009; Goecke et al., Citation2010; Jha et al., Citation2013; Batista et al., Citation2014).
Quorum sensing (QS) is a chemical bacterial cell-to-cell communication (Fuqua et al., Citation1994; Batista et al., Citation2014). During this process bacteria produce and excrete special signal molecules (autoinducers), which result in the activation or deactivation of target genes responsible for cell division, adhesion, biofilm formation and production of toxins and other chemical compounds (Davies, Citation1998; Dobretsov et al., Citation2007, Citation2009; Jha et al., Citation2013). Inhibition of bacterial QS leads to disruption of biofilm adhesion and formation (Dobretsov et al., Citation2009). Some algal species, like the red alga Delisea pulchra, produce QS inhibitors that prevent microbial fouling on their surface (Manefield et al., Citation1999; de Nys et al., 2006).
The invasion of macroalgae in an ecosystem and the possible replacement of local species might lead to detrimental ecological and economic impacts (Mack et al., Citation2000; Thomsen et al., Citation2009). Invasion success has been correlated in many cases with several abiotic (Moyle & Light, Citation1996; Murphy et al., Citation2016) and biotic (Low et al., Citation2015) conditions of the host habitat. An efficient defence against biofouling might be advantageous for the invader’s success (Cassano et al., Citation2008). Recent studies have highlighted the link between the success of marine bioinvasion and the establishment of chemical defences by introduced organisms (Wikström et al., Citation2006; Cassano et al., Citation2008; Hammann et al., Citation2016). Ships’ ballast water can be an important vector for the transport of macroalgal spores and invertebrate larvae and for the subsequent introduction of exotic species (Flagella et al., Citation2007). The potential for introduction of alien species via ballast water discharge in the Arabian Gulf region is significant since approximately 40% of the world’s total oil transportation passes through these waters. Thus, an equal volume of ballast water is transferred by oil tankers every day in the opposite direction and more than 2.7 × 106 m3 of water (i.e. 17 million barrels) from various areas of the world’s ocean may be transported to the Arabian Gulf, the Sea of Oman and the Arabian Sea every day (Al-Yamani et al., Citation2015). Al-Yamani et al. (Citation2015) suggested that 14 alien species, ranging from algae to fish, have been inadvertently introduced into the Arabian Gulf.
The genus Sargassum is well known to produce a variety of compounds effective against oxidation (Heo & Jeon, Citation2009; Jin et al., Citation2014), diseases (Heo et al., Citation2014), herbivores (Engelen et al., Citation2011) and biofouling (Plouguerné et al., Citation2010a, Citation2010b; Thabard et al., Citation2011; Gerasimenko et al., Citation2013, Citation2014). Secondary metabolites of Sargassum include phenolic compounds, also known as phlorotannins (Kamiya et al., Citation2010). Phlorotannins have been described as UV screens, herbivore and larval deterrents and antimicrobial agents, but are also known to play a role in primary metabolism including serving as brown algal cell wall components or precursors to holdfast adhesive (Shibata et al., Citation2002; Paul & Puglisi, Citation2004; Brock et al., Citation2007).
Sargassum muticum (Yendo) Fensholt 1955 is a highly invasive algal species from the Pacific (Chinese and Japanese Sea) which was unintentionally introduced to Europe with oysters in the early 1970s (Critchley, Citation1983). It spread along the Atlantic coast of western Europe from Portugal in the south to Scandinavia in the north and reached the German part of the North Sea in 1988 (Kornmann & Sahling, Citation1994; Karlsson & Loo, Citation1999). Introduced S. muticum has affected the local algal community in the North Sea by competing with endemic leathery and coarsely branched algae and reduced their community in different regions (Stæhr et al., Citation2000; Buschbaum et al., Citation2012; Engelen et al., Citation2015). Sargassum muticum produces antibacterial, antifungal, anti-algal and anti-invertebrate compounds (Plouguerné et al., Citation2008, Citation2010b; Bazes et al., Citation2009) and is a rich source of phlorotannins (Tanniou et al., Citation2014), suggesting that the invasiveness of S. muticum might be partly explained by its effective chemical defence.
The reason for the invasion success of S. muticum has never been fully explained and this study is the first attempt to compare the chemical defence of this alga with other non-invasive Sargassum species from Oman. This comparison should enable the identification of specific invasive traits promoting the invasion success of S. muticum and might help to assess the invasion probability of S. muticum in Omani waters.
This study investigated the hypothesis that the invasive alga S. muticum is better defended against epibiosis than its non-invasive relatives. The aims of this study were to: (1) investigate antibacterial, anti-QS, anti-algal and anti-larval activity of Sargassum extracts in laboratory experiments; (2) compare antifouling activities of these extracts in a field experiment; and (3) compare and analyse metabolites in Sargassum extracts by GC-MS analysis.
Materials and methods
Sargassum species
Collection of algae and extract preparation
Thalli of the three different Sargassum species from Oman were collected by snorkelling in Khaluf, Oman (20°28′23″N, 58°3′59″E) in October 2012 at a depth of 1–2 m. Sargassum muticum was collected in March 2012 by scuba diving in Oshima, Japan (33°56′26.3″N, 132°24′06.0″E) at a depth of 1–3 m. After collection, all Sargassum specimens were immediately transferred to the laboratory in insulated coolers filled with seawater, where they were gently washed with sterile seawater and cleaned of associated microflora and -fauna. The wet weight (± 0.01 g) was measured with a balance and the volume of each species determined by water displacement in a graduated cylinder. After freeze-drying the algae were weighed again (± 0.01 g) and ground to a fine powder to increase extraction efficiency.
The resulting algal powder was extracted three times with ethyl acetate/methanol (EtOAc:MeOH) (1:1, v/v) (AppliChem GmbH, Darmstadt, Germany). Final extraction was done with 100% methanol. For each gram of alga, 20 ml of solvent was used at every consecutive extraction. Each extract was filtered through Whatman No. 1 filters and remaining solvents were removed by rotary evaporation. The obtained extracts were combined, dried under a stream of nitrogen, weighed, and stored in a freezer at −15°C until used in bioassays ().
Table 1. Yield of crude extracts after extraction of freeze-dried tissue of local Omani Sargassum sp. 1 (S1), Sargassum sp. 2 (S2), Sargassum sp. 3 (S3) and the invasive Sargassum muticum from Japan.
Before the experiments, extracts were re-dissolved to the desired concentration (i.e. above, below or at natural tissue-level concentration) using EtOAc/MeOH (1:1 v/v) (Applichem GmbH) for anti-QS and anti-diatom assays and dimethyl sulfoxide (DMSO) (Prolabo; VWR International, Ltd, Leicestershire, UK) for antibacterial assay. Extracts were left under a hood to completely evaporate the EtOAc/MeOH solvents before bioassays were conducted.
Algal identification
Algal DNA was isolated using the FastDNA® SPIN Kit and the FastPrep® Instrument (Qbiogene, Inc., CA). Mitochondrial cytochrome oxidase 1 and 3 (COI and cox3) gene markers were selected for comparison and identification to take advantage of an abundance of published sequences. The specific primer sets for amplification and sequencing of each marker were: GAZF2 and GAZR2 for COI (Lane et al., Citation2007) and CAF4A and CAR4A for cox3 (Kogame et al., Citation2005). PCR products were sequenced in both directions with the same primer sets by GATC (GATC Biotech AG, Konstanz, Germany). COI and cox3 sequences were deposited in the NCBI GenBank with accession numbers KX661321–KX661324.
Laboratory experiments
Experimental conditions (e.g. assay temperature) were adjusted to provide optimum growth conditions for the fouling organisms in order to detect antifouling effects as quickly as possible, thereby minimizing the risk of extract degradation or loss through diffusion. In all experiments extracts were tested at natural tissue-level concentrations ().
Antibacterial assay
Inhibition of bacterial growth by Sargassum spp. extracts was assessed via a spectrophotometric method using a microplate photometer (Devienne & Raddi, Citation2002). Test bacteria included Escherichia coli K12 (SQUH-156), Chromobacterium violaceum CV026 (SQUMSF-026), Shewanella sp. (SQUMSF-018) and Pseudomonas putida (SQUMSF-007). All bacteria were obtained from the culture collection of Sultan Qaboos University and were chosen in order to cover a range of antibacterial activities against both marine and terrestrial bacterial strains, which are commonly used in antibacterial assays (e.g. Nylund et al., Citation2005; Plouguerné et al., Citation2010b). The marine bacteria Shewanella sp. and P. putida were isolated from 21-day-old biofilms on submerged wooden substrata at Marina Bandar Al Rowdah, Muscat, Oman (23°34′55″N, 58°36′27″E). All bacterial cultures were grown overnight in Luria Bertani broth (LB, BD, USA) at 31°C. For cultivation of marine bacteria, filtered, autoclaved seawater from the Marina Bandar Al-Rowdah was used to prepare LB broth. Before each bioassay bacteria were diluted with fresh LB broth to a final density of about 2 × 106 cells ml−1 to assure similar initial conditions for all bacterial strains and replicates (Devienne & Raddi, Citation2002). Before the experiment, algal extracts were added at the respective tissue concentrations to 96-well plates (Nunc 96 MicroWell™ polystyrene plates – MaxiSorp™). 200 µl of bacteria-containing LB medium were added to each well. The antibiotics chloramphenicol (Sigma, USA) and, in the case of E. coli, streptomycin (Sigma, USA), were added at concentrations of 100 mg ml−1 as positive controls, whereas 25 µl ml−1 of the solvent DMSO was used as a negative control. Wells without extracts were used as additional controls. Each treatment and control was replicated three times. Optical densities (ODs) of the different treatments were measured in a microplate photometer reader at 620 nm (Thermo Scientific Multiscan FC, Type: 357). A turbidimetric growth curve was generated by measuring the OD after 0, 1, 2, 4, 6, 12 and 24 h from the start of the experiment. After the measurements the specific slope of each growth curve was calculated. All measurements were done in triplicate.
Anti-Quorum Sensing (QS) assay
The QS inhibitory activity of Sargassum spp. extracts was tested using the reporter strain Chromobacterium violaceum CV017 (Chernin et al., Citation1998). This reporter strain produces short side-chain N-Acyl homoserine lactones (AHL), which induce the production of the purple pigment violacein. Changes in violacein concentrations represent changes in QS activity (Dobretsov et al., Citation2009; Jha et al., Citation2013). Prior to the experiment C. violaceum CV017 was grown in Luria-Bertani (LB) medium (BD, USA) at 30°C overnight. To obtain differences in QS activity between Sargassum spp., 2-, 3-, 4- and 6-fold higher concentrations were tested in addition to the natural tissue-level concentration. Then 15 µl of each extract at each concentration were applied onto sterile cellulose paper discs (diameter = 6 mm; Carl Roth GmbH & Co. KG, Germany). 15 µl of ethyl acetate/methanol (1:1) were applied to a sterile disc and used as a control. The solvents were evaporated prior to the experiments. Three replicates were tested for each extract concentration. 200 μl of a liquid culture of the reporter strain (density of 2 × 106 cell ml−1) was pipetted on the LB agar plates (diameter = 9 cm) and spread with a Drigalski spatula. Afterwards dried discs with either extract (treatment) or solvent (control) were placed onto the agar. The agar plates were incubated for 24 h at 30°C until the bacteria developed a visible purple colour. The observed transparent zones around the disc indicated presence of QS inhibition. The zones were measured to the nearest 0.2 mm by a ruler. The minimum inhibitory concentration (MIC) was determined for each extract as the minimal concentration of extract causing an inhibition zone of at least 1 mm.
In order to determine that inhibition zones are indeed the result of a QS inhibition and not caused by toxicity of the extracts, antibacterial experiments with C. violaceum CV026 were conducted (see antibacterial assay). Chromobacterium violaceum CV026 is a mutant strain incapable of producing AHL and violacein (Jha et al., Citation2013) and only the growth inhibition due to extracts can be observed.
Anti-diatom assay
The algal extracts were tested for anti-diatom activity against the benthic diatom, Cylindrotheca closterium (Reimann & Lewin, Citation1964; CCAP 1017/8), obtained from the CCAP (Culture Collection of Algae and Protozoa, SAMS Research Services Ltd, Scottish Marine Institute, Dunbeg, Argyll, PA37 1QA, UK). The diatom cultures were grown under controlled conditions at 17.8°C with 16 ± 0.5 µmol photons m−2 s−1 cool-white fluorescent light, with a 12:12 light-dark cycle in F/2 medium (Guillard & Ryther, Citation1962). Prior to the experiments, crude extracts of all Sargassum spp. were pipetted into 24 well plates (TPP, polystyrene, Sigma-Aldrich) at species-specific natural tissue-level concentrations. Untreated wells and those containing EtOAc/MeOH served as controls. After complete evaporation of the solvent, 1 ml of diatom culture at 20.31 × 104 cells ml−1 was added and allowed to grow and attach for 6 d (Silkina et al., Citation2012). The assay was conducted with six replicates. After 6 d the diatom densities were determined under a microscope (Leica microsystems CMS GmbH; magnification 40 × 10). Diatoms were counted in 10 randomly selected fields of view (0.25 mm2) and the mean diatom densities (cells cm−2) in treatments were compared with controls to assess possible growth inhibition by the extracts (Cho, Citation2013).
Anti-larval settlement bioassay
Anti-larval activity was assessed using larvae of the bryozoan Bugula neritina (Schmitt et al., Citation1995). Prior to the experiment, adults of B. neritina were collected from submerged ship hulls and ropes hanging from the floating docks of the Marina Bander Al Rowdah. The bryozoans were directly transported to the lab and stored in dark, aerated buckets (4 l) filled with natural filtered (pore size = 0.45 µm) seawater for the next 24 h. Bryozoan larvae were collected with a pipette (for details see Dahms et al., Citation2004) and immediately used in the experiments. In the first experiment the effect of natural tissue-level concentrations of Sargassum extract on larval settlement was tested. In the second experiment extracts were diluted to one-third concentration and used in the anti-larval bioassay. In both experiments the extracts were added into 24 multi-well polystyrene dishes (Corning® Costar®, USA) and kept under the fume hood until complete evaporation of solvents. The solvent and wells without extracts served as controls. Subsequently 1 ml of filtered (pore size = 0.45 µm) seawater containing 10–20 bryozoan larvae was added to each well. Numbers of settled, swimming and dead larvae were counted using a dissecting microscope (Axiostar Plus) after 1 h, 8 h and 20 h. Larvae were scored as settled after metamorphosis, which is marked by eversion of the metasomal internal sac (Zimmer & Woollacott, Citation1977). Mean percentages of settled, swimming and dead larvae in each treatment were compared with the controls.
Field experiment
Preparation of gels and field experiments
The antifouling activities of the Sargassum extracts were tested in a field experiment in February 2013 with a modified method of Henrikson & Pawlik (Citation1995). The gels were prepared by adding 1.63 g of Phytagel® (Sigma-Aldrich, Germany) to 50 ml distilled water with vigorous mixing for 10 s using a hand-held blender. The mixture was heated to boiling point in a microwave oven and then cooled to 70°C. Extracts were added to the liquid gel solution at natural tissue concentrations and vigorously mixed for even distribution. Control gels were prepared by adding the same amount of solvent to the liquid gel. 1 ml of the liquid gel mixtures was poured into silicone moulds (5 × 12 × 4 mm, BioSciences, USA). Six replicates were prepared for each Sargassum extract and the controls. Moulds were stored at 4°C for 12 h before field deployment to ensure the homogeneous diffusion of the extract compounds throughout the gel. The moulds were exposed to micro-fouling for 7 days at a depth of 30 cm and water temperatures of 28°C in the Marina Bandar Al Rowdah. In previous assays with Phytagel substrata, a 7-day period was found to produce a mature microfouling community in the Marina waters (data not shown). The moulds were attached to individual ropes and hung randomly on a pontoon at a minimum distance of 3 m from each other. After 7 d the moulds were retrieved and individual gels were immediately fixed with formalin (4% solution in artificial filtered seawater). Fixed gels were brought directly to the laboratory for later determination of bacterial and diatom densities.
Determination of bacterial and diatom density
Attached bacteria on the gel surface (n=6) of each treatment and control were visualized with 0.5 mg ml−1 DNA-binding fluorochrome 4, 6-diamidino-2-phenylindole (VECTASHIELD® with DAPI, Biologo). The gels were stained with the dye for 15 min in the dark. Bacterial numbers were counted in 10 randomly selected fields (area = 0.0146 cm2) using an epifluorescence microscope (Axiophot, Zeiss; magnification 1000×; λex = 359 nm, λem = 441 nm; Coleman, Citation1980).
Diatom numbers were counted in 10 randomly selected fields (area= 0.07 mm2) using a microscope (Axiostar Plus, Zeiss; magnification 400×). The main genera of diatoms were determined by screening the whole gel surface. Gels with damaged surfaces were excluded from the analysis.
Extraction and quantification of phlorotannins
Phlorotannins were extracted from Sargassum spp. following the procedure described in Zhang et al. (Citation2006). Briefly, 20 ml MeOH/water (1:1) was added to 0.5 g of freeze-dried algal powder, adjusted to pH 2 and shaken for 1 h (150 rpm) at room temperature. After centrifugation, the pellet was extracted with acetone/water (7:3) under the same conditions and centrifuged. The two liquid extracts were combined and mixed. 100 µl of this solution was diluted 1:10 with water and used as the sample solution. The total phenolic content of all extracts was determined photometrically (Thermo Fisher Scientific Inc., Waltham, Massachusetts, USA) with a microplate-adapted Folin–Cioalteu assay (Zhang et al., Citation2006). The polyphenol quantification is based on a colour reaction between easily oxidized polyphenols or hydroxylated aromatic compounds and phosphotungsten-polymolybdic acid (Zhang et al., Citation2006). Phloroglucinol (1,3,5-trihydroxybenzene, Sigma-Aldrich, Germany) was used as a standard and a calibration curve was generated with a range of concentrations from 6.25 to 100 µg ml−1. Total phenolic contents (TPCs) were expressed as percentages of phenolic compounds per DW of the algae.
Analysis and comparison of chemical composition of Sargassum extracts
Composition of crude extracts of Sargassum spp. was compared using gas chromatography–mass spectrometry (GC–MS). The analysis was performed on a Perkin Elmer Clarus 600 Series GC-MS analyser with electron impact ionization. Crude extracts were dissolved in hexane at a concentration of 1 µg ml−1 and 1 µl of the solution was injected into the GC–MS. The total GC run time was 53 minutes with helium as carrier gas and a flow rate of 1 ml min−1. The initial oven temperature was 80°C for 2 min, after which the temperature was increased by 10°C min−1 until it reached 290°C. The final temperature was held for 30 min. The injector temperature was 290°C and the split ratio was 1:30. GC–MS separations were achieved in a HP Ultra 2 capillary column (5% Phenyl, 95% methylpolysiloxane, length = 25 m, diameter = 200 µm, film thickness = 0.33 µm, Hewlett Packard). The NIST GC–MS library (NIST 2011 version 2.3 and Wiley, 9th edition) was used to identify compounds in the extracts. Hexane was used as negative control. Identity of peaks and the number of peaks in each extract (chemical diversity) were recorded. The closest match with the highest probability in the library was recorded, while compounds with probability below 75% were considered unknown.
Statistical analysis
All statistical calculations were performed using SPSS IBM Statistics version 22, Illinois, USA.
The slopes of the bacterial growth assay were compared with the negative and positive controls by a Mann–Whitney U test for two independent variables. To measure the intensity of growth inhibition, the log response ratio (LogRR) was calculated as LogRR = log (T/C), where T is the mean slope of bacterial growth on either algal extracts or antibiotics, and C is the mean slope of bacterial growth on the solvent control. A positive value of LogRR indicates facilitation and a negative value indicates inhibition of bacterial growth (Borenstein et al., Citation2009).
After assuring normality and homogeneity of the data a one-way ANOVA was used to assess the effects of Sargassum extracts on the diatom Cylindrotheca closterium. Differences between the extracts and the control were determined with subsequent Tukey post-hoc tests.
The numbers of settled, swimming and dead B. neritina larvae were expressed as percentages and arcsine-transformed before analysis. In the case of zero dead or settled larvae, a value of 1/4n (n = number of larvae in one single replicate) was given to improve the arcsine transformation (Zar, Citation1999). Due to non-homogeneous variances, differences between larval settlement rates were evaluated with the Mann–Whitney U test (Zar, Citation1999).
The diatom and bacterial densities on the gels in the field experiment were square-root-transformed in order to ensure normality of variance and analysed with a one-way ANOVA followed by LSD post-hoc test (Zar, Citation1999).
Results
Extraction yield
The extraction of Sargassum spp. yielded a species-specific amount of crude extract from which the specific natural tissue concentration was calculated and used for all bioassays ().
Algal identification
The COI and cox3 sequences did not allow identification to species level but clearly indicated that the algae are three different species of the genus Sargassum. Sargassum sp. 1 and sp. 3 probably belong to the Sargassum section Ilicifoliae clade and Sargassum sp. 2 could be identified as Sargassum cf. latifolium. The invasive Sargassum species from Japan was clearly identified as S. muticum. The three native Sargassum species from Omani waters are named henceforth as S1, S2 and S3.
Antibacterial assay
The more negative the values of the log response ratio (logRR), the greater the inhibitory effect on bacterial growth. Among the Sargassum spp. extracts only invasive Sargassum muticum inhibited the growth of all tested bacterial strains significantly and acted similarly to the positive control (). S1, 2 and 3 showed similar activity towards most bacterial strains. S1 inhibited Shewanella sp. and P. putica significantly, whereas S3 had a rather significant stimulatory effect on Shewanella sp. and C. violaceum.
Fig. 1. Log response ratios of growth of the bacteria Shewanella sp., Escherichia coli, Chromobacterium violaceum and Pseudomonas putida in response to natural concentration extracts of local Omani (dotted bars) Sargassum sp. 1 (S1), Sargassum sp. 2 (S2), Sargassum sp. 3 (S3), the invasive S. muticum (S.m., striped bars), and the antibiotic positive control (AB, open bars) chloramphenicol (or streptomycin in the case of E. coli). A positive value of LogRR indicates facilitation and a negative value indicates inhibition of bacterial growth (see text for details). Asterisks indicate significant differences between treatments and negative control (DMSO) according to the Mann–Whitney U test (P ≤ 0.05).
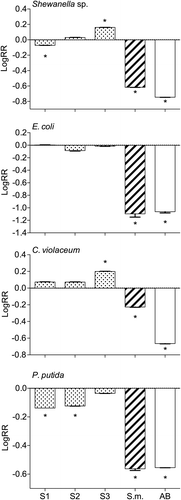
Fig. 2. Densities of the diatom Cylindrotheca closterium after growing for 7 d in the presence of natural concentration extracts of Sargassum muticum (A) and local Sargassum sp. 1 (S1) (B; representative of all three local Sargassum species), and the solvent control (C). Arrows indicate C. closterium cells.
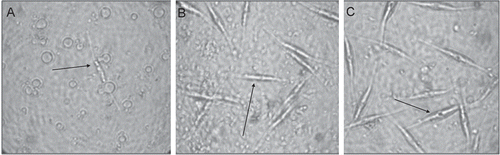
Fig. 3. The effect of crude, natural tissue concentration extracts of local (dotted bars) Sargassum sp. 1 (S1), Sargassum sp. 2 (S2), Sargassum sp. 3 (S3), and the invasive (striped bar) S. muticum (S.m.) on cell densities (mean ± SE, n = 6) of the diatom Cylindrotheca closterium. Control contained an ethyl acetate/methanol mixture (1:1). Significant differences according to the Mann–Whitney U test (P < 0.05) are indicated by different letters above the bars.
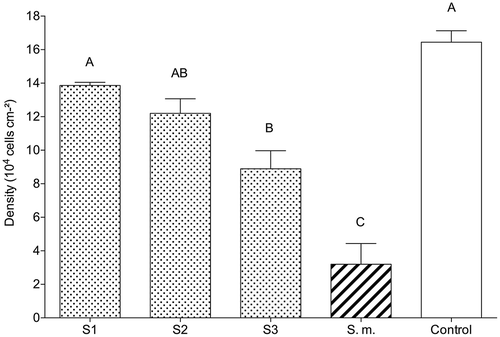
Anti-quorum sensing (QS) assay
None of the extracts inhibited QS of the reporter C. violaceum CV017 at natural tissue concentrations (). However, extracts of the invasive S. muticum inhibited QS of the reporter at a four-fold natural tissue concentration (0.115 µg µl−1). Other Sargassum spp. extracts did not interfere with QS of C. violaceum CV017 even at six-fold natural tissue concentrations ().
Table 2. Comparison of extracts of local Sargassum sp. 1 (S1), Sargassum sp. 2 (S2), Sargassum sp. 3 (S3) and invasive S. muticum in quorum sensing (QS) inhibition bioassay using the reporter Chromobacterium violaceum CV017 and the toxicity test using the strain Chromobacterium violaceum CV026.
Anti-diatom assay
There were visible differences between the morphology of the diatom Cylindrotheca closterium growing on extracts of invasive S. muticum, local Sargassum species and the control (). The diatoms growing on S. muticum extracts were pale in colour and hardly visible (). Their frustules were broken, releasing chloroplasts which covered the bottom of the wells. In contrast to these results, diatoms growing on extracts of the three local Sargassum species were largely intact (). Frustules appeared to break about 20% more often in the presence of extracts of S3 than in the presence of extracts of S1 and S2. In the control, C. closterium cells were healthy and only few broken cells (<10%) and loose chloroplasts were found ().
During the assay, diatom cell densities varied between 16.4 × 104 ± 0.7 cells cm−2 (control) and 3.2 × 104 ± 0.2 cells cm−2 (S. muticum; ). Diatom growth was significantly inhibited by all extracts when compared with the controls (Tukey test, P < 0.001). The lowest diatom density (five-fold lower than the control one) was observed in the presence of S. muticum extract ().
Anti-larval settlement bioassay
At natural tissue concentration all extracts significantly inhibited settlement of Bugula neritina larvae compared with controls (Mann–Whitney U, P < 0.05; A). After 20 h almost all larvae either settled or died (). All extracts at natural tissue concentration caused significant mortality of larvae (Mann–Whitney U, P < 0.05; ). After 20 h, extracts of Sargassum S1, S2 and S3 resulted in the death of more than 90% of larvae at natural tissue concentrations. Extracts of S. muticum had the strongest effect, since 100% of the larvae died after exposure for 1 h (). The ratio of unsettled and dead larvae significantly differed between S. muticum, local Sargassum spp. (S1, S2 and S3) extracts and the control (Mann–Whitney U, P < 0.05; ). After 8 and 20 h, the lowest ratio of settled/unsettled larvae was found in trials with S. muticum extracts (0) and the highest ratio was recorded for the control (4.08 and 12.2). S1, S2 and S3 had a moderate ratio (0.05–0.44 and 0.07–0.2) of settled/unsettled larvae.
Fig. 4. Percentage (mean ± SE, n = 4) of settled (A), unsettled (B) and dead (C) larvae of Bugula neritina treated with natural tissue concentrations (black bars) and three-fold diluted concentrations (white bars) of crude extracts of local Sargassum sp. 1 (S1), Sargassum sp. 2 (S2), Sargassum sp. 3 (S3) and invasive S. muticum (S.m.). C is the solvent control (ethyl acetate/methanol, 1:1). Larvae were counted 1, 8 and 20 h after the start of the experiment. Significant differences in settlement between natural (capital letters) and three-fold diluted (lower case letters) extract concentrations are indicated by different letters above the bars (P < 0.05 according to the Mann–Whitney U test).
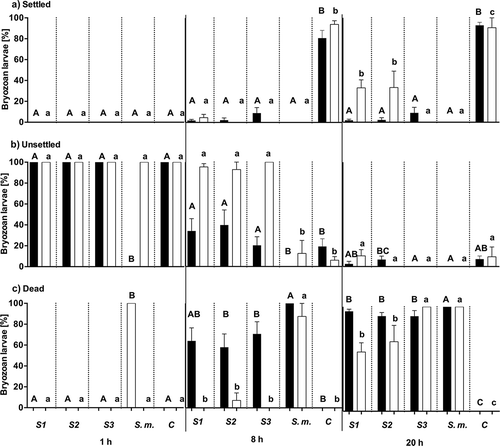
Sargassum extracts showed no activity against larval settlement after 1 h at three-fold diluted natural tissue concentration (Mann–Whitney U, P > 0.05), however, after 8 h more than 90% of all larvae had settled in the controls whereas in wells with three-fold diluted Sargassum extracts from Omani waters more than 90% of larvae remained swimming (). Furthermore, 80–90% of larvae exposed to three-fold diluted S. muticum extracts died after 8 h. In the presence of S1 and S2 extracts more than 30% of larvae settled and started metamorphosis and growth after 20 h (). In the presence of S3 and S. muticum extracts, all larvae died after 20 h.
Field experiment
Light microscopy revealed that the bacterial communities on all gels (controls and treatments) were dominated by rod-shaped and coccoid bacterial cells. Two out of five gels containing extracts of S1 and S3 were lost during the experiment. Bacterial densities on the gel surfaces varied from 11.9 × 104 cells cm−2 (S. muticum extracts) to 71.9 × 104 cells cm−2 (control; ). The density of bacteria was significantly higher on gels with S. muticum extracts in comparison with the control (LSD test, P < 0.05, ). Densities of bacteria on the gels with Sargassum S1, S2 and S3 extracts and controls did not differ from each other (LSD test, P > 0.05). Microalgal communities were dominated by the diatoms Cylindrotheca spp. and Amphora spp., as well as some species of Cyanobacteria. These groups accounted for more than 90% of species in all biofilms. Mean densities of diatoms fluctuated from 2.76 × 104 cells on gels with S. muticum extracts to 18.46 × 104 cells on the control gels (). Extracts of S. muticum and Sargassum S1 significantly (LSD test, P < 0.05) reduced densities of diatoms in comparison to the controls. There were no significant differences of diatom densities between Sargassum species (LSD test, P > 0.05).
Fig. 5. Bacterial (a) and diatom (b) cell densities (mean ± SD) on gels with embedded natural tissue concentration extracts of the local (dotted bars) Sargassum sp. 1 (S1), Sargassum sp. 2 (S2), Sargassum sp. 3 (S3), invasive (striped bar) S. muticum (S.m.), and the solvent control (open bar) after 7 days exposure in the sea. Significant differences (ANOVA, LSD, P < 0.05) are indicated by different letters.
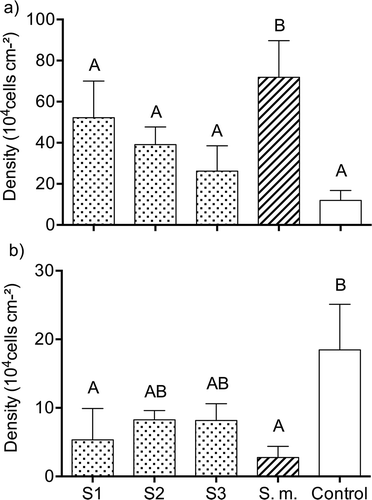
Phlorotannin content
Phlorotannin content (TPC) of invasive S. muticum was significantly higher compared with native Sargassum spp. (Tukey test, P < 0.001). TPC differed between minimal values of 0.15 ± 0.045% DWalgae for Sargassum S1 and maximal values of 5.87 ± 0.97% DWalgae for S. muticum ().
Metabolomic comparison of Sargassum extracts
Cluster analysis demonstrated that composition of S. muticum extracts shared less than 30% similarity with other Sargassum species extracts (). The composition of S2 and S3 extracts were similar (similarity > 70%). The composition of the extract of S1 was different to all other extracts (). Sargassum muticum had overall fewer compounds (n = 15) compared with the native Sargassum S1 (n = 21), S2 (n = 24) and S3 (n = 17) species. The most dominant compounds in extracts of Sargassum S1, S2 and S3 were neophytadiene and hexadecanoic fatty acids. Sargassum muticum extract was dominated by neophytadiene as well but it also contained four unidentified compounds (with retention times of 22.3, 30.04, 45.01 and 64.05 min) which were missing in the native Sargassum species. All Sargassum spp. extracts were dominated by unidentified fatty acids (). In total, most of the compounds (> 50%) did not have a close match with the NIST GC-MS library.
Table 3. Results of gas chromatography-mass spectrometry (GC-MS) analysis of crude extracts of Sargassum sp. 1 (S1), Sargassum sp. 2 (S2), Sargassum sp. 3 (S3) and invasive S. muticum (S.m.).
Fig. 7. Chemical comparison of crude extracts from different Sargassum species by cluster analysis. The dendrogram is based on a Bray–Curtis similarity matrix containing data on presence or absence of peaks in individual extract samples. Data represent samples of Sargassum sp. 1 (S1), Sargassum sp. 2 (S2), Sargassum sp. 3 (S3) and invasive S. muticum (S.m.).
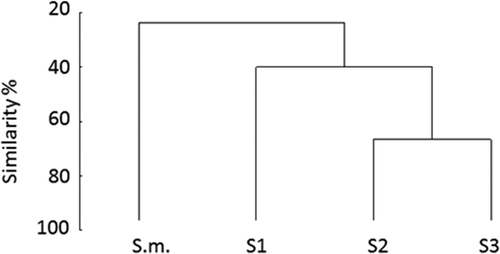
Discussion
Invasive species are considered as one of the greatest threats to local communities and global diversity in addition to climate change and habitat loss (Mack et al., Citation2000). The introduction of alien species can affect native community structure significantly by competitive interactions or even replacement (Mack et al., Citation2000; Thomsen et al., Citation2009). There is experimental evidence that chemically mediated interactions are an important factor facilitating the successful invasion of alien species (Wikström et al., Citation2006; Cassano et al., Citation2008). Here we demonstrate that the invasive Sargassum muticum has a more effective antibacterial, anti-quorum sensing, anti-diatom and anti-larval defence in comparison to three native Sargassum species from Omani waters. These advantages in S. muticum chemical defence might enable a possible future invasion of this alga into the Arabian Gulf region.
Macroalgae of the genus Sargassum contain a large number of compounds that showed antifouling activity in laboratory bioassays (Ganti et al., Citation2006; Bazes et al., Citation2009; Silkina et al., Citation2009, Citation2012; Plouguerné et al., Citation2010a, Citation2010b; Gerasimenko et al., Citation2014). Most of the evidence for antifouling activity of Sargassum species came from laboratory experiments. In this study the antifouling activity of different Sargassum species was tested both in laboratory and, for the first time, field experiments.
Laboratory antibacterial assays showed that the natural level concentration of crude extract from the invasive S. muticum was the only one inhibiting bacterial growth of all four tested bacteria. The inhibitory effect of S. muticum extracts was almost as strong as the antibiotic control and in the case of E. coli and P. putida, even stronger. The low antibacterial activity of the local Sargassum crude extracts from Omani waters contradicts previous results with other Sargassum species. Plouguerné et al. (Citation2010a) tested inhibitory activity of polar- and non-polar extracts of Sargassum vulgare against five biofilm-forming marine bacteria and found antibacterial activity in different extract fractions, whereby the methanol fraction was the most active one with a minimum inhibitory concentration of 0.2 µg ml−1 against Vibrio aestuarianus and Pseudoalteromonas elyakovii. The difference between our results and other researchers’ could be due to differences in test bacteria and concentrations of bioactive compounds and fractions. The pronounced antibacterial activity of S. muticum reported in this study is congruent with previous reports (Plouguerné et al., Citation2008, Citation2010b). Sargassum muticum chloroform extracts inhibited bacterial growth of five bacterial strains with MICs of 100 µg ml−1. Saturated and unsaturated linear hydrocarbons, arachidonic acid, palmitic, linolenic, palmitoleic acids and galactoglycerolipids were investigated as main components of the active fractions (Plouguerné et al., Citation2008). Additionally, antibacterial experiments with isolated galactoglycerolipids showed activity against the growth of two out of four tested bacterial strains (Plouguerné et al., Citation2010b). In another study, palmitic acid was identified as the main compound in S. muticum extract and its antibacterial activity at 44 µg ml−1 had been confirmed (Bazes et al., Citation2009). While we did not quantify the above-mentioned compounds in our extracts, we did however analyse phlorotannin content as a potentially bioactive metabolite. Phlorotannin concentrations differed between 0.151 ± 0.045% DWalgae for Sargassum sp. S1 and maximal values of 5.868 ± 0.968% DWalgae for S. muticum. These values were in the range of previously reported phlorotannin contents of sargassacean species (< 10% DWalgae, Ragan & Glombitza, Citation1986; 0.66 ± 0.15 – 3.40 ± 0.91% DWalgae, Tanniou et al., Citation2014). The activity of phlorotannins against a broad range of bacteria has been shown in previous studies. Nagayama et al. (Citation2002) detected bactericidal activity of phlorotannins from the brown alga Ecklonia kurome (phlorotannins ~ 3% DWalgae) against 35 bacterial strains with minimum bactericidal concentrations ranging from 50 mg l−1 to 400 mg l−1. Similarly, phlorotannins of Sargassum wightii strongly inhibited the growth of E. coli and Aeromonas hydrophila and showed moderate activity against Bacillus subtilis and Pseudomonas aeruginosa (Vijayabaskar & Shiyamala, Citation2011).
Sargassum muticum crude extract was the only extract exhibiting a positive anti-quorum sensing (QS) activity in the agar-diffusion assay with a MIC of four times natural tissue concentration. This was probably due to toxicity of S. muticum extracts which was demonstrated in the antibacterial assay with C. violaceum CV026 at natural tissue concentration. The low anti-QS activity of Sargassum species in this study contrasts with the high inhibitive activity of macroalgal extracts found in previous studies (Goecke et al., Citation2010; Jha et al., Citation2013; Batista et al., Citation2014). Recently, Batista et al. (Citation2014) screened extracts of 11 macroalgal species from the Brazilian coast for their ability to inhibit QS of the reporter C. violaceum CV017. The researchers found anti-QS activity in all tested species, including Sargassum furcatum, with MICs ranging from 0.28 µg ml−1 to 2730 µg ml−1. The MIC of QS inhibition by S. muticum (4 × natural tissue concentration) examined in this study far exceeded the reported MIC of S. furcatum (0.1 × natural tissue concentration) (Batista et al., Citation2014). The low anti-QS activity found in this study might have been due to differences in extraction techniques. Batista et al. (Citation2014) stated that non-polar extracts of S. furcatum inhibited QS below the natural tissue concentration whereas polar compounds of this alga had no inhibitive effect. In the present study Sargassum species were extracted with a mixture of EtOAc/MeOH (1:1), which might only partially extract non-polar QS inhibitors such as long-chain furanones. Alternatively, differences in microbial communities associated with different Sargassum species could explain low anti-QS activity in our study (Goecke et al., Citation2010; Batista et al., Citation2014; Busetti et al., Citation2015).
The stronger antibacterial activity of the invasive S. muticum in comparison to non-invasive Sargassum spp. from Omani waters was not confirmed in our field experiment. Bacterial settlement and growth on gels containing Sargassum extracts at natural tissue concentration was not inhibited, but on the contrary, bacterial densities were 2.5–20-fold higher on gels containing extracts compared with controls. These results are not surprising since macroalgae are known to release large amounts of organic carbon into the surrounding environment, providing nutrients for microorganisms and hence represent a nutrient-rich habitat favourable for marine bacterial settlement and growth (Haas et al., Citation2011). Furthermore, if phlorotannins are the antimicrobial agents, their effect could have been diminished in the field, since they are large, water-soluble compounds, which probably dissolve quickly in the surrounding seawater and are gone within a few days. However, the associated bacterial community could have had synergistic effects and might protect the algal surface from additional fouling organisms by releasing antifouling substances into the surrounding seawater (Dobretsov & Qian, Citation2002; Goecke et al., Citation2013). Algae might be able to control their attached biofilm by promoting the attachment of certain bacteria which prevent other organisms from settling on their host’s surface (reviewed by Wahl et al., Citation2012). Lachnit et al. (Citation2010) demonstrated that the bacterial community which had developed on a substratum containing Fucus vesiculosus metabolites at natural tissue concentration was distinct from one formed on the control substratum without metabolites. This showed the strong selective force of algal metabolites on formation of epibiotic microbial communities. Saha et al. (Citation2012) did show that common macroalgal metabolites, such as dimethylsulphopropionate (DMSP) and the amino acids proline and alanine inhibited surface attachment by some bacteria (e.g. Cytophaga sp.), while enhancing the attachment of others (e.g. Rheinheimera baltica). Thus, the low antibacterial activity of Sargassum extracts found in our field experiment and the high antibacterial activity of S. muticum in the laboratory could suggest that S. muticum metabolites might enhance attachment of some bacteria with antifouling properties and inhibit attachment of others.
A strong antifouling activity of invasive S. muticum was observed in the laboratory assays with diatoms. The growth of C. closterium was significantly inhibited and their morphology changed in the presence of extracts from invasive S. muticum in contrast to extracts from non-invasive Sargassum and solvent controls. This anti-diatom activity of S. muticum extracts was supported by the results of the field experiment. Gel surfaces containing S. muticum crude extracts revealed more than 85% reduction in diatom densities relative to control gels. Similarly, extracts of native Sargassum species reduced diatom densities on the gels by 50% in comparison to the control. These results are consistent with previous laboratory studies showing antifouling properties of S. muticum crude extracts against certain diatom species (Silkina et al., Citation2009, Citation2012). In their studies S. muticum compounds reduced growth and pigment content of the tested diatoms Fragilaria pinnata, Cylindrotheca closterium and Thalassiosira pseudonana (Silkina et al., Citation2009, Citation2012). The anti-diatom activity of S. muticum has been attributed to palmitic acid which inhibited the growth of C. closterium at concentrations of 45.5 µg ml−1 under controlled conditions (Bazes et al., Citation2009). Thus, palmitic acid might be responsible for the anti-diatom effect of S. muticum in our study but could not be identified by GC-MS and may therefore be referred to as unidentified fatty acid. In another laboratory study, the growth of C. closterium was inhibited by Sargassum vulgare extract fractions of different polarity with minimum inhibitory concentrations ranging from 0.2 to 500 µg ml−1 (Plouguerné et al., Citation2010a). Wang et al. (Citation2006, Citation2007) reported an inhibitory effect of Sargassum thunbergii against the diatom Skeletonema costatum and dinoflagellates. The mechanisms underlying the diatoms’ response to algal extract are not yet fully understood. However, S. muticum crude extract has been shown to alter the photosynthetic activity (Silkina et al., Citation2009), which might be the reason for the changed morphology of C. closterium growing on S. muticum extract in our study.
An effective anti-larval activity of S. muticum extracts against larvae of the bryozoan Bugula neritina was observed. While all tested Sargassum extracts were toxic to bryozoan larvae at tissue-level concentrations, only three-fold diluted extracts of S. muticum and S3 killed 100% of larvae. Sargassum muticum was also the only extract causing immediate death (after < 1 h) of larvae in our study. The effective anti-larval defensive properties of Sargassum species have been shown using other invertebrate species. Thabard et al. (Citation2011) showed that Sargassum polyceratium surface molecules significantly affect embryonic development of three tropical invertebrates (Codakia orbicularis, Diadema antillarum and Pseudonereis sp.). Furthermore, their embryo toxicity assays revealed lethal concentrations ranging between 10–200 µg ml−1 (100% mortality after 30 h exposure), depending on the test species. Six structurally similar compounds, isolated from the brown alga Sargassum horneri, inhibited larval settlement of the mussel Mytilus edulis with an EC50 of 0.11–3.34 μg ml−1 (Cho, Citation2013). Phlorotannins extracted from Sargasssum tenerrimum were highly effective against Hydroides elegans larval settlement with an EC50 and LC50 of 0.526 ppm and 13.984 ppm respectively (Lau & Qian, Citation1997). Similarly, a strong and significant negative relationship between phlorotannin concentration and larval settlement had been reported from field and laboratory experiments with Fucus vesiculosus (Brock et al., Citation2007). Thus, the higher phlorotannin concentration of S. muticum in our study compared with native Sargassum spp. might again contribute to its antifouling activity. Waterborne chemicals exuded by Sargassum echinocarpum and Sargassum polyphyllum, reduced settlement of larvae of the polychaete H. elegans, but had no effect on settlement of B. neritina larvae (Walters et al., Citation1996). The different defence activities of other Sargassum species against B. neritina larvae in the above studies demonstrate the variability of Sargassum defences against certain foulers, which was also observed in our experiments between S. muticum and native Sargassum species from Oman waters.
In our study extracts were tested at tissue-level concentrations. Although we used a best estimate of ecologically relevant concentrations of extracts in our assay, the concentrations used were based on the assumption that chemical constituents in the extracts were produced by the alga itself and that their concentrations were distributed evenly throughout the algal tissue. Although we recognize the limitation of this assumption, chemical ecologists have traditionally used whole-tissue extracts as a starting point until more detailed knowledge of compound localization within the organism becomes available (Henrikson & Pawlik, Citation1998; Nylund et al., Citation2005; Gochfeld & Aeby, Citation2008; Feng et al., Citation2011). To account for possible variable concentrations of substances on the algal surface compared with inside its thallus (Sudatti et al., Citation2006; Lima et al., Citation2008), we also tested lower and higher concentrations of Sargassum spp. extracts.
GC–MS analysis of crude extracts revealed that the identified compounds of all Sargassum spp. extracts were dominated by fatty acids and neophytadiene. Their potential role as antifouling agents has been discussed before. Rosell & Srivastava (Citation1987) highlighted the antibacterial activity of unsaturated fatty acids from the brown alga Desmarestia ligulata. In a more recent study Ganti et al. (Citation2006) demonstrated the antifouling activity of fats and phthalic acid derivatives isolated from Sargassum confusum. Similarly, Plouguerné et al. (Citation2010b) identified saturated and unsaturated linear hydrocarbons (C12–C27) in anti-microfouling active fractions of S. muticum chloroform extracts. Additionally they detected several major metabolites (i.e. arachidonic acid, palmitic, linolenic, palmitoleic acids, and galactoglycerolipids) that were not found in the S. muticum extract analysed in this study. The highly non-polar compounds found in the chloroform extract of Plouguerné et al. (Citation2010b) might be missing in the S. muticum crude extract of the present study due to the use of different solvents for extraction.
Cluster analysis of GC–MS detected compounds demonstrated the differences (<30% similarity) in the chemical composition between the extracts of the invasive S. muticum and the native Sargassum species. These differences were reflected in laboratory and field bioassays, where S. muticum extract showed stronger antifouling defences than native Sargassum sp against bacteria, settling diatoms and bryozoan larvae. The uniqueness of secondary compounds in invasive species and their potential role in invasion success has been demonstrated in several studies previously (reviewed by Cappuccino & Arnason, Citation2006). Macel et al. (Citation2014) compared a broad range of metabolites of six successful exotic plant species and their native congeners of the family Asteraceae. The authors found highly species-specific and diverse plant chemistry among both exotic and native species. However, exotic species had on average more species-unique metabolites compared with their native congeners (Cappuccino & Arnason, Citation2006).
Although it is known that different developmental stages of algal species might contain different metabolite compositions (Kamiya et al., Citation2010), it was not possible to collect Japanese and Omani Sargassum spp. at the same developmental stage due to weather conditions and the seasonal occurrence of Sargassum spp. in Oman. Invasive S. muticum was collected in Japan before maturation and native Sargassum spp. in Oman during maturation. It has been shown that the phenolic content of S. muticum in Brittany peaked during the reproductive period which was hypothesized as to provide maximum protection of the fertile receptacles from both grazing and solar radiation (Plouguerné et al., Citation2006). Thus, our approach was rather conservative since Sargassum spp. from Oman might be expected to be at its highest level of chemical defence, whereas the invasive S. muticum might have a lower level of its chemical defence.
Laboratory and field experiments demonstrated strong differences in the antifouling defence of invasive and non-invasive Sargassum species. Invasive S. muticum was better chemically defended against diatoms than native Sargassum species. Additionally S. muticum was more effective against larval settlement and the only species inhibiting bacterial QS and bacterial growth of all tested strains in the laboratory study. While the efficient antifouling defence of S. muticum could be demonstrated and is probably contributing to its invasive success, the specific chemical mode of operation still needs further investigation. This study supports the hypothesis that invasive species are better defended from biofouling and more competitive than local species. Due to its strong chemical defence S. muticum might be able to invade the Arabian Gulf region and should be included in future monitoring programmes for invasive species assessments in this area.
Acknowledgements
We gratefully acknowledge Mr Jamal Al-Sabahi (Oman) for assistance with GC–MS analysis and compound identification and Dr Hiromori Shimabukuro (Japan) for algal sampling. We would like to thank Fabian Heinke and Johanna Meinecke (ICBM, Germany) for phlorotannin extraction, Samuel Nietzer and Tessa Clemens (ICBM, Germany) for algal DNA extraction and PCR and the Pathology department, College of Medicine, SQU for providing silicone moulds for the experiments.
Disclosure statement
No potential conflict of interest was reported by the authors.
Additional information
Funding
References
- Al-Yamani, F.Y., Skryabin, V. & Durvasula, S.R.V. (2015). Suspected ballast water introductions in the Arabian Gulf. Aquatic Ecosystem Health & Management, 18: 282–289.
- Barbosa, J.P., Fleury, B.G., Gama, B.A.P. da, Teixeira, V.L. & Pereira, R.C. (2007). Natural products as antifoulants in the Brazilian brown alga Dictyota pfaffii (Phaeophyta, Dictyotales). Biochemical Systematics and Ecology, 35: 549–553.
- Batista, D., Carvalho, A.P., Costa, R., Coutinho, R. & Dobretsov, S. (2014). Extracts of macroalgae from the Brazilian coast inhibit bacterial quorum sensing. Botanica Marina, 57: 441–447.
- Bazes, A., Silkina, A., Douzenel, P., Faÿ, F., Kervarec, N., Morin, D., Berge, J.P. & Bourgougnon, N. (2009). Investigation of the antifouling constituents from the brown alga Sargassum muticum (Yendo) Fensholt. Journal of Applied Phycology, 21: 395–403.
- Borenstein, M., Hedges, L. V, Higgins, J.P.T. & Rothstein, H.R. (2009). Effect sizes based on correlations. In Introduction to Meta-Analysis. John Wiley & Sons, Ltd, Chichester, UK. 21–32. doi: 10.1002/9780470743386.ch4.
- Bouarab, K., Potin, P., Weinberger, F., Correa, J. & Kloareg, B. (2001). The Chondrus crispus–Acrochaete operculata host-pathogen association, a novel model in glycobiology and applied phycopathology. Journal of Applied Phycology, 13: 185–193.
- Brock, E., Nylund, G. & Pavia, H. (2007). Chemical inhibition of barnacle larval settlement by the brown alga Fucus vesiculosus. Marine Ecology Progress Series, 337: 165–174.
- Buschbaum, C., Lackschewitz, D. & Reise, K. (2012). Nonnative macrobenthos in the Wadden Sea ecosystem. Ocean and Coastal Management, 68: 89–101.
- Busetti, A., Shaw, G., Megaw, J., Gorman, S.P., Maggs, C.A. & Gilmore, B.F. (2015). Marine-derived quorum-sensing inhibitory activities enhance the antibacterial efficacy of tobramycin against Pseudomonas aeruginosa. Marine Drugs, 13: 1–28.
- Cappuccino, N. & Arnason, J.T. (2006). Novel chemistry of invasive exotic plants. Biology Letters, 2: 189–193.
- Cassano, V., De-Paula, J.C., Fujii, M.T., Gama, B.A.P. Da & Teixeira, V.L. (2008). Sesquiterpenes from the introduced red seaweed Laurencia caduciramulosa (Rhodomelaceae, Ceramiales). Biochemical Systematics and Ecology, 36: 223–226.
- Cen-Pacheco, F., Santiago-Benítez, A.J., García, C., Álvarez-Méndez, S.J., Martín-Rodríguez, A.J., Norte, M., Martín, V.S., Gavín, J.A., Fernández, J.J. & Daranas, A.H. (2015). Oxasqualenoids from Laurencia viridis: combined spectroscopic-computational analysis and antifouling potential. Journal of Natural Products, 78: 712–721.
- Chernin, L., Winson, M., Thompson, J., Haran, S., Bycroft, B., Chet, I., Williams, P. & Stewart, GSAB. (1998). Chitinolytic activity in Chromobacterium violaceum: substrate analysis and regulation by quorum sensing. Journal of Bacteriology, 180: 4435–4441.
- Cho, J.Y. (2013). Antifouling chromanols isolated from brown alga Sargassum horneri. Journal of Applied Phycology, 25: 299–309.
- Coleman, A. (1980). Enhanced detection of bacteria in natural environments by fluorochrome staining of DNA. Limnology and Oceanography, 25: 948–951.
- Critchley, A.T. (1983). Sargassum muticum – a taxonomic history including world-wide and western Pacific distributions. Journal of the Marine Biological Association of the United Kingdom, 63: 617–625.
- Culioli, G., Ortalo-Magné, A., Valls, R., Hellio, C., Clare, A.S. & Piovetti, L. (2008). Antifouling activity of meroditerpenoids from the marine brown alga Halidrys siliquosa. Journal of Natural Products, 71: 1121–1126.
- Dahms, H.U., Dobretsov, S. & Qian, P.Y. (2004). The effect of bacterial and diatom biofilms on the settlement of the bryozoan Bugula neritina. Journal of Experimental Marine Biology and Ecology, 313: 191–209.
- Davies, D.G. (1998). The involvement of cell-to-cell signals in the development of a bacterial biofilm. Science, 280: 295–298.
- Devienne, K.F. & Raddi, M.S.G. (2002). Screening for antimicrobial activity of natural products using a microplate photometer. Brazilian Journal of Microbiology, 33: 166–168.
- Dixon, J., Schroeter, S. & Kastendiek, J. (1981). Effects of the encrusting bryozoan, Membranipora membranacea, on the loss of blades and fronds by the giant kelp, Macrocystis pyrifera (Laminariales). Journal of Phycology, 17: 341–345.
- Dobretsov, S. & Qian, P.Y. (2002). Effect of bacteria associated with the green alga Ulva reticulata on marine micro- and macrofouling. Biofouling, 18: 217–228.
- Dobretsov, S., Dahms, H.U. & Qian, P.Y. (2006). Inhibition of biofouling by marine microorganisms and their metabolites. Biofouling, 22: 43–54.
- Dobretsov, S., Dahms, H.U., Yili, H., Wahl, M. & Qian, P.Y. (2007). The effect of quorum-sensing blockers on the formation of marine microbial communities and larval attachment. FEMS Micriobiology Ecology, 60: 177–188.
- Dobretsov, S., Teplitski, M. & Paul, V. (2009). Mini-review: quorum sensing in the marine environment and its relationship to biofouling. Biofouling, 25: 413–427.
- Dobretsov, S., Abed, R.M.M., Maskari, S.M.S. Al, Sabahi, J.N. Al & Victor, R. (2011). Cyanobacterial mats from hot springs produce antimicrobial compounds and quorum-sensing inhibitors under natural conditions. Journal of Applied Phycology, 23: 983–993.
- Engelen, A.H., Henriques, N., Monteiro, C. & Santos, R. (2011). Mesograzers prefer mostly native seaweeds over the invasive brown seaweed Sargassum muticum. Hydrobiologia, 669: 157–165.
- Engelen, A.H., Serebryakova, A., Ang, P., Britton-Simmons, K., Mineur, F., Pedersen, M. F., Arenas, F., Fernández, C., Steen, H., Svenson, R., Pavia, H., Toth, G.B., Viard, F. & Santos, R. (2015). Circumglobal invasion by the brown seaweed Sargassum muticum. Oceanography and Marine Biology: An Annual Review, 53: 81–126.
- Feng, D., Ke, C., Lu, C. & Li, S. (2011). Antifouling activity of marine sessile organisms from China against barnacle settlement. Journal of the Marine Biological Association of the United Kingdom, 91: 1073–1079.
- Fernandes, N., Case, R.J., Longford, S.R., Seyedsayamdost, M.R., Steinberg, P.D., Kjelleberg, S. & Thomas, T. (2011). Genomes and virulence factors of novel bacterial pathogens causing bleaching disease in the marine red alga Delisea pulchra. PLoS ONE, 6.
- Flagella, M.M., Verlaque, M., Soria, A. & Buia, M.C. (2007). Macroalgal survival in ballast water tanks. Marine Pollution Bulletin, 54: 1395–1401.
- Flemming, H.C. (2009). Why microorganisms live in biofilms and the problem of biofouling. In Marine and Industrial Biofouling (Flemming, H.C., Murthy, P.S., Venkatesan, R. & Cooksey, K., editors), 3–12. Springer, Berlin.
- Fuqua, W.C., Winans, S.C. & Greenberg, E.P. (1994). Quorum sensing in bacteria: the LuxR-LuxI family of cell density-responsive transcriptional regulators. Journal of Bacteriology, 176: 269–275.
- Gama, B. a. P. da, Carvalho, A.G.V., Weidner, K., Soares, A.R., Coutinho, R., Fleury, B.G., Teixeira, V.L. & Pereira, R.C. (2008). Antifouling activity of natural products from Brazilian seaweeds. Botanica Marina, 51: 191–201.
- Ganti, V.S., Kim, K.H., Bhattarai, H.D. & Shin, H.W. (2006). Isolation and characterisation of some antifouling agents from the brown alga Sargassum confusum. Journal of Asian Natural Products Research, 8: 309–315.
- Gerasimenko, N.I., Logvinov, S. V., Busarova, N.G. & Martyyas, E.A. (2013). Structure and biological activity of several classes of compounds from the brown alga Sargassum pallidum. Chemistry of Natural Compounds, 49: 927–929.
- Gerasimenko, N.I., Martyyas, E.A., Logvinov, S.V. & Busarova, N.G. (2014). Biological activity of lipids and photosynthetic pigments of Sargassum pallidum C. Agardh. Applied Biochemistry and Microbiology, 50: 73–81.
- Gochfeld, D.J. & Aeby, G.S. (2008). Antibacterial chemical defences in Hawaiian corals provide possible protection from disease. Marine Ecology Progress Series, 362: 119–128.
- Goecke, F., Labes, A., Wiese, J. & Imhoff, J. (2010). Chemical interactions between marine macroalgae and bacteria. Marine Ecology Progress Series, 409: 267–299.
- Goecke, F., Labes, A., Wiese, J. & Imhoff, J.F. (2013). Phylogenetic analysis and antibiotic activity of bacteria isolated from the surface of two co-occurring macroalgae from the Baltic Sea. European Journal of Phycology, 48: 47–60.
- Guillard, R. & Ryther, J. (1962). Studies of marine planktonic diatoms. 1. Cyclotella nana Hustedt, and Detonula confervacea (Cleve) Gran. Canadian Journal of Microbiology, 8: 229–239.
- Haas, A.F., Nelson, C.E., Wegley Kelly, L., Carlson, C.A., Rohwer, F., Leichter, J.J., Wyatt, A. & Smith, J.E. (2011). Effects of coral reef benthic primary producers on dissolved organic carbon and microbial activity. PloS ONE, 6: e27973.
- Hadfield, M.G. (2011). Biofilms and marine invertebrate larvae: what bacteria produce that larvae use to choose settlement sites. Annual Review of Marine Science, 3: 453–470.
- Hammann, M., Rempt, M., Pohnert, G., Wang, G., Boo, S.M. & Weinberger, F. (2016). Increased potential for wound activated production of Prostaglandin E2 and related toxic compounds in non-native populations of Gracilaria vermiculophylla. Harmful Algae, 51: 81–88.
- Hellio, C., Maréchal, J.-P., Gama, B.A.P. da, Pereira, R.C. & Clare, A.S. (2009). Natural marine products with antifouling activities. In Advances in Marine Antifouling Coatings and Technologies (Hellio, C. & Yebra, D.M., editors), 572–622. Woodhead Publishing Ltd, Cambridge.
- Henrikson, A.A. & Pawlik, J.R. (1995). A new antifouling assay method: results from field experiments using extracts of four marine organisms. Journal of Experimental Marine Biology and Ecology, 194: 157–165.
- Henrikson, A.A. & Pawlik, J.R. (1998). Seasonal variation in biofouling of gels containing extracts of marine organisms. Biofouling, 12: 245–255.
- Heo, S.-J., Jang, J., Ye, B.-R., Kim, M.-S., Yoon, W.-J., Oh, C., Kang, D.-H., Lee, J.-H., Kang, M.-C., Jeon, Y.-J., Kang, S.-M., Kim, D., Kim, K.-N. (2014). Chromene suppresses the activation of inflammatory mediators in lipopolysaccharide-stimulated RAW 264.7 cells. Food and Chemical Toxicology, 67: 169–75.
- Heo, S.-J. & Jeon, Y.-J. (2009). Protective effect of fucoxanthin isolated from Sargassum siliquastrum on UV-B induced cell damage. Journal of Photochemistry and Photobiology B – Biology, 95: 101–107.
- Jha, B., Kavita, K., Westphal, J., Hartmann, A. & Schmitt-Kopplin, P. (2013). Quorum sensing inhibition by Asparagopsis taxiformis, a marine macro alga: separation of the compound that interrupts bacterial communication. Marine Drugs, 11: 253–265.
- Jin, W., Zhang, W., Wang, J., Yao, J., Xie, E., Liu, D., Duan, D. & Zhang, Q. (2014). A study of neuroprotective and antioxidant activities of heteropolysaccharides from six Sargassum species. International Journal of Biological Macromolecules, 67: 336–342.
- Kamiya, M., Nishio, T., Yokoyama, A., Yatsuya, K., Nishigaki, T., Yoshikawa, S. & Ohki, K. (2010). Seasonal variation of phlorotannin in sargassacean species from the coast of the Sea of Japan. Phycological Research, 58: 53–61.
- Karlsson, J. & Loo, L.-O. (1999). On the distribution and continuous expansion of the Japanese seaweed – Sargassum muticum – in Sweden. Botanica Marina, 42: 285–294.
- Kogame, K., Uwai, S., Shimada, S. & Masuda, M. (2005). A study of sexual and asexual populations of Scytosiphon lomentaria (Scytosiphonaceae, Phaeophyceae) in Hokkaido, northern Japan, using molecular markers. European Journal of Phycology, 40: 313–322.
- Kornmann, P. & Sahling, P.-H. (1994). Meeresalgen von Helgoland: Zweite Ergänzung. Helgoländer Meeresuntersuchungen, 48: 365–406.
- Lachnit, T., Wahl, M. & Harder, T. (2010). Isolated thallus-associated compounds from the macroalga Fucus vesiculosus mediate bacterial surface colonization in the field similar to that on the natural alga. Biofouling, 26: 247–255.
- Lane, C.E., Lindstrom, S.C. & Saunders, G.W. (2007). A molecular assessment of northeast Pacific Alaria species (Laminariales, Phaeophyceae) with reference to the utility of DNA barcoding. Molecular Phylogenetics and Evolution, 44: 634–648.
- Lau, S.C.K. & Qian, P.Y. (1997). Phlorotannins and related compounds as larval settlement inhibitors of the tube-building polychaete Hydroides elegans. Marine Ecology Progress Series, 159: 219–227.
- Lima, L.M. de S., Alor, R., Uriostegui, R., Murray, S.N. & Pereira, R.C. (2008). Within-plant variation in palatability and chemical defences in the green seaweed Avrainvillea elliottii. Botanica Marina, 51: 21–25.
- Low, N.H.N., Drouin, A., Marks, C.J. & Bracken, M.E.S. (2015). Invader traits and community context contribute to the recent invasion success of the macroalga Heterosiphonia japonica on New England rocky reefs. Biological Invasions, 17: 257–271.
- Macel, M., Vos, R.C.H. de, Jansen, J.J., Putten, W.H. van der & Dam, N.M. van. (2014). Novel chemistry of invasive plants: exotic species have more unique metabolomic profiles than native congeners. Ecology and Evolution, 4: 2777–2786.
- Mack, R.N., Simberloff, D., Lonsdale, W.M., Evans, H., Clout, M. & Bazzaz, F.A. (2000). Biotic invasions: causes, epidemiology, global consequences, and control. Ecological Applications, 10: 689–710.
- Manefield, M., Nys, R. de, Kumar, N., Read, R., Givskov, M. & Steinberg, P. (1999). Evidence that halogenated furanones from Delisea pulchra inhibit acylated homoserine lactone (AHL)-mediated gene expression by displacing the AHL signal from its receptor protein. Microbiology, 145: 283–291.
- Mieszkin, S., Callow, M.E. & Callow, J.A. (2013). Interactions between microbial biofilms and marine fouling algae: a mini review. Biofouling, 29: 1097–1113.
- Moyle, P.B. & Light, T. (1996). Fish invasions in California: do abiotic factors determine success? Ecology, 77: 1666–1670.
- Murphy, J.T., Johnson, M.P. & Viard, F. (2016). A modelling approach to explore the critical environmental parameters influencing the growth and establishment of the invasive seaweed Undaria pinnatifida in Europe. Journal of Theoretical Biology, 396: 105–115.
- Nagayama, K., Iwamura, Y., Shibata, T., Hirayama, I. & Nakamura, T. (2002). Bactericidal activity of phlorotannins from the brown alga Ecklonia kurome. Journal of Antimicrobial Chemotherapy, 50: 889–893.
- Nylund, G., Cervin, G., Hermansson, M. & Pavia, H. (2005). Chemical inhibition of bacterial colonization by the red alga Bonnemaisonia hamifera. Marine Ecology Progress Series, 302: 27–36.
- Nys, R. de, Givskov, M., Kumar, N., Kjelleberg, S. & Steinberg, P.D. (2006). Furanones. Progress in Molecular and Subcellular Biology, 42: 55–86.
- Paul, V.J. & Puglisi, M.P. (2004). Chemical mediation of interactions among marine organisms. Natural Product Reports, 21: 189–209.
- Pérez, M., Falqué, E. & Domínguez, H. (2016). Antimicrobial action of compounds from marine seaweed. Marine Drugs, 14: 52.
- Plouguerne, E., Le Lann K., Connan S., Jechoux G., Deslandes E. & Stiger V. (2006). Spatial and seasonal variations in density, maturity, length and phenolic content of the invasive brown macroalga Sargassum muticum along the coast of Western Brittany (France). Aquatic Botany, 8: 337–344.
- Plouguerné, E., Hellio, C., Deslandes, E., Véron, B. & Stiger-Pouvreau, V. (2008). Anti-microfouling activities in extracts of two invasive algae: Grateloupia turuturu and Sargassum muticum. Botanica Marina, 51: 202–208.
- Plouguerné, E., Hellio, C., Cesconetto, C., Thabard, M., Mason, K., Véron, B., Pereira, R.C. & Gama, B.A.P. (2010a). Antifouling activity as a function of population variation in Sargassum vulgare from the littoral of Rio de Janeiro (Brazil). Journal of Applied Phycology, 22: 717–724.
- Plouguerné, E., Ioannou, E., Georgantea, P., Vagias, C., Roussis, V., Hellio, C., Kraffe, E. & Stiger-Pouvreau, V. (2010b). Anti-microfouling activity of lipidic metabolites from the invasive brown alga Sargassum muticum (Yendo) Fensholt. Marine Biotechnology (New York, N.Y.), 12: 52–61.
- Qian, P.-Y., Lau, S.C.K., Dahms, H.-U., Dobretsov, S. & Harder, T. (2007). Marine biofilms as mediators of colonization by marine macroorganisms: implications for antifouling and aquaculture. Marine Biotechnology (New York, N.Y.), 9: 399–410.
- Ragan, M.A. & Glombitza, K.-W. (1986). Phlorotannins, brown algal polyphenols. In Progress in Phycological Research (Round, F.E. & Chapman, D.J., editors), 129–241. Biopress, Bristol.
- Reimann, B.E.F. & Lewin, J.C. (1964). The diatom genus Cylindrotheca rabenhorst. Journal of the Royal Microscopical Society, 83: 283–296.
- Rohde, S., Hiebenthal, C., Wahl, M., Karez, R. & Bischof, K. (2008). Decreased depth distribution of Fucus vesiculosus (Phaeophyceae) in the Western Baltic: effects of light deficiency and epibionts on growth and photosynthesis. European Journal of Phycology, 43: 143–150.
- Rosell, K.G. & Srivastava, L.M. (1987). Fatty acids as antimicrobial substances in brown algae. Hydrobiologia, 151-152: 471–475.
- Saha, M., Rempt, M., Gebser, B., Grueneberg, J., Pohnert, G. & Weinberger, F. (2012). Dimethylsulphopropionate (DMSP) and proline from the surface of the brown alga Fucus vesiculosus inhibit bacterial attachment. Biofouling, 28: 593–604.
- Salta, M., Wharton, J.A., Blache, Y., Stokes, K.R. & Briand, J.-F. (2013). Marine biofilms on artificial surfaces: structure and dynamics. Environmental Microbiology, 15: 2879–2893.
- Schmitt, T.M., Hay, M.E. & Lindquist, N. (1995). Constraints on chemically mediated coevolution: multiple functions for seaweed secondary metabolites. Ecology, 76: 107–123.
- Shibata, T., Yamaguchi, K., Nagayama, K., Kawaguchi, S. & Nakamura, T. (2002). Inhibitory activity of brown algal phlorotannins against glycosidases from the viscera of the turban shell Turbo cornutus. European Journal of Phycology, 37: 493–500.
- Silkina, A., Bazes, A., Vouvé, F., Tilly, V. Le, Douzenel, P., Mouget, J.-L & Bourgougnon, N. (2009). Antifouling activity of macroalgal extracts on Fragilaria pinnata (Bacillariophyceae): a comparison with Diuron. Aquatic Toxicology (Amsterdam, Netherlands), 94: 245–254.
- Silkina, A., Bazes, A., Mouget, J.-L. & Bourgougnon, N. (2012). Comparative efficiency of macroalgal extracts and booster biocides as antifouling agents to control growth of three diatom species. Marine Pollution Bulletin, 64: 2039–2046.
- Stæhr, P.A., Pedersen, M.F., Thomsen, M.S., Wernberg, T. & Krause-Jensen, D. (2000). Invasion of Sargassum muticum in Limfjorden (Denmark) and its possible impact on the indigenous macroalgal community. Marine Ecology Progress Series, 207: 79–88.
- Sudatti, D.B., Rodrigues, S.V. & Pereira, R.C. (2006). Quantitative GC-ECD analysis of halogenated metabolites: determination of surface and within-thallus elatol of Laurencia obtusa. Journal of Chemical Ecology, 32: 835–843.
- Tanniou, A., Vandanjon, L., Incera, M., Serrano Leon, E., Husa, V., Grand, J. Le, Nicolas, J. L., Poupart, N., Kervarec, N., Engelen, A., Walsh, R.D., Guerard, F., Bourgougnon, N. & Stiger-Pouvreau, V. (2014). Assessment of the spatial variability of phenolic contents and associated bioactivities in the invasive alga Sargassum muticum sampled along its European range from Norway to Portugal. Journal of Applied Phycology, 26: 1–16.
- Thabard, M., Gros, O., Hellio, C. & Mare, J. (2011). Sargassum polyceratium (Phaeophyceae, Fucaceae) surface molecule activity towards fouling organisms and embryonic development of benthic species. Botanica Marina, 54: 147–157.
- Thomsen, M.S., Wernberg, T., Tuya, F. & Silliman, B.R. (2009). Evidence for impacts of nonindigenous macroalgae: a meta-analysis of experimental field studies. Journal of Phycology, 45: 812–819.
- Vijayabaskar, P. & Shiyamala, V. (2011). Antibacterial activities of brown marine algae (Sargassum wightii and Turbinaria ornata) from the Gulf of Mannar biosphere reserve. Advances in Biological Research, 5: 99–102.
- Wahl, M. (1989). Marine epibiosis. I. Fouling and antifouling: some basic aspects. Marine Ecology Progress Series, 58: 175–189.
- Wahl, M. (2008). Ecological lever and interface ecology: epibiosis modulates the interactions between host and environment. Biofouling, 24: 427–438.
- Wahl, M., Goecke, F., Labes, A., Dobretsov, S. & Weinberger, F. (2012). The second skin: ecological role of epibiotic biofilms on marine organisms. Frontiers in Microbiology, 3: 1–21.
- Walters, L.J., Hadfield, M.G. & Smith, C.M. (1996). Waterborne chemical compounds in tropical macroalgae: positive and negative cues for larval settlement. Marine Biology, 126: 383–393.
- Wang, R., Tang, X., Feng, L., Xiao, H., Qu, L. & Cai, H. (2006). Inhibitory effect of Sargassum thunbergii on Heterosigma akashiwo and Skeletonema costatum. Journal of Applied Ecology, 17: 2421–2425.
- Wang, R., Xiao, H., Zhang, P., Qu, L., Cai, H. & Tang, X. (2007). Allelopathic effects of Ulva pertusa, Corallina pilulifera and Sargassum thunbergii on the growth of the dinoflagellates Heterosigma akashiwo and Alexandrium tamarense. Journal of Applied Phycology, 19: 109–121.
- Wikström, S.A., Steinarsdóttir, M.B., Kautsky, L. & Pavia, H. (2006). Increased chemical resistance explains low herbivore colonization of introduced seaweed. Oecologia, 148: 593–601.
- Zar, J.H. (1999). Biostatistical analysis. 4th edn. Prentice Hall, Upper Saddle River, NJ.
- Zhang, Q., Zhang, J., Shen, J., Silva, A., Dennis, D. A. & Barrow, C.J. (2006). A simple 96-well microplate method for estimation of total polyphenol content in seaweeds. Journal of Applied Phycology, 18: 445–450.
- Zimmer, R.L. & Woollacott, R. (1977). Metamorphosis, ancestrulae, and coloniality in bryozoan life cycles. In Biology of Bryozoans (Woollacott, R.M. & Zimmer, R.L., editors), 91–142. Academic Press, New York.