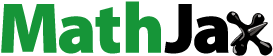
ABSTRACT
The aim of this paper was to examine the characteristics of Eucheuma cottonii waste in order to analyse its potential as renewable material. The morphology of Eucheuma cottonii (raw and wastes) was investigated through scanning electron microscopy (SEM), the thermal behaviour through thermogravimetric analysis (TGA) and the physical properties through FT-IR, XRD, gas pycnometer, particle size analyser, water absorption and moisture content analysis. The chemical compositions were determined by using acid detergent fibre (ADF), neutral detergent fibre (NDF) and acid detergent lignin (ADL) analysis. It was found that Eucheuma cottonii wastes have better thermal stability, higher crude fibre content, lower moisture content and similar density to the raw Eucheuma cottonii, which suggests that these biomass wastes have good potential as renewable filler material.
Introduction
Waste disposal of non-renewable materials has created serious environmental problems for many countries in the world. This issue is getting worse for small countries with limited landfill area. A waste management hierarchy has been proposed in order to achieve sustainable management, which includes reduction in waste, recycling, reuse and lastly landfill (Peddireddy et al., Citation2015). New environmental legislation has forced manufacturing industries to look for alternative materials for their products, which are more sustainable than conventional materials such as glass fibres and petroleum-based polymer composites. In recent years, the application of agricultural wastes as renewable filler materials has gained interest. The known advantages of these resources are low cost, low density, and reduced tool wear, which favour the manufacturing industry.
Many types of natural wastes have been investigated for their suitability as reinforcements in polymer composites, including coconut shell particles, oil palm empty fruit bunches and others (Sastra et al., Citation2006; Jawaid et al., Citation2011). Recent studies have advanced the feasibility of other fibres for polymer composites, such as roselle (Hibiscus sabdariffa) (Razali et al., Citation2015) and date palm (Phoenix dactylifera) (Al-Oqla et al., Citation2014). Mechakra et al. (Citation2015) extracted vegetable fibre, namely alfa (Stippa tenacissima), and investigated its microstructure and potential as filler in polymer. Although the natural fibre of terrestrial plants has been studied widely for their potential as reinforcement in polymer composite, only a few research studies have used aquatic plants as reinforcement material. A recent study by Bulota et al. (Citation2015) used raw algae obtained from the north coast of France as fillers and prepared poly(lactic) acid (PLA) composite via injection moulding. Enhancement in the modulus was observed upon algae loading in the composite. The algae Gelidium elegans and Laminaria japonica were also used as fillers for polymer and improvements in the thermal and mechanical properties were reported with their incorporation (Lee et al., Citation2008; Jang et al., Citation2013).
The red alga Eucheuma cottonii (also known as Kappaphycus alvarezii) can be found in abundance on the inner sides of coral reefs around the Philippines, Indonesia and the island coasts of East Africa (Chan et al. Citation2013). This macroalga is a very promising biomass owing to its high growth rate, high yield per area and high efficiency in CO2 capture (Sanchez-Silva et al., Citation2013). Moreover, East Malaysia, particularly the east coast of Sabah, is among the main cultivators of this species in the South East Asia Region. This species has been cultivated for 40 years in tropical regions for its hydrocolloids, namely carrageenan (Santos, Citation1989). Carrageenan is a marine-based polysaccharide which is extracted from red seaweed, in which it is the major structural component of the cell walls (Pereira & Van De Velde, Citation2011). Carrageenan is used widely as a thickening agent for food and non-food applications. Due to the high demand for these hydrocolloids, the seaweed processing industry has grown rapidly. In the most recent decade, the world business sector for carrageenan has expanded by around 4% every year yielding US $527 million in 2009 (Zhang et al., Citation2015).
However, these multi-million dollar industries also produce industrial wastes from processing. Since the content of carrageenan in seaweed varies from only 25–35% of dry weight, an enormous amount of waste product is left behind during the extraction process, which is usually referred to as seaweed solid wastes (Tan & Lee, Citation2014). Only a small proportion of the seaweed solid wastes are utilized as organic fertilizer and most is discarded as waste, eventually causing environmental pollution. It is recorded that 1 million tonnes of seaweed is required to produce only 50 000 tonnes of hydrocolloids, leaving a huge amount of waste which is yet to be utilized (Kang et al., Citation2011).
Although there have been many studies on natural-based materials, it is clear from the literature that no study has been carried out on the potential of seaweed solid wastes from Eucheuma cottonii species as filler material. Thorough evaluations of the properties of new natural materials will be beneficial for new potential applications of bio-based materials (Al-Oqla et al., Citation2014). Since carrageenan extraction is a worldwide industry, the discovery of a new potential application for solid wastes from the process could be beneficial to society. The present research work has been undertaken with the objective of exploring the characteristics of seaweed solid wastes of E. cottonii species from Sabah, Malaysia.
Materials and methods
Waste from Eucheuma cottonii species was obtained from seaweed extraction, and raw E. cottonii was collected from the east coast of Sabah, Malaysia as a control. Approximately 2 kg of solid wastes were obtained after a hot alkaline extraction process used to obtain carrageenan. & show the raw form of E. cottonii seaweed and its waste. Both the waste and raw seaweed were cleaned with water and dried at 80°C for 24 h in a drying oven. The dried seaweeds were ground and sieved then kept in zip-locked bags until further characterization.
Physical properties
Particle size
The particle size was analysed using a Mastersizer 2000 E (Malvern Instruments, Malvern, UK) fitted with a Qspec Dry powder Feeder.
Scanning electron microscope (SEM)
The morphology of the samples was observed under a scanning electron microscope (SEM), model Hitachi S-3400N with acceleration voltage of 10 kV.
Moisture content
Five samples were prepared for the moisture content investigation. All samples were heated in an oven for 24 h at 105°C. The weight of the samples before (Mi) and after (Mf) heating was obtained in order to calculate the moisture content. The moisture content of the seaweed was determined using EquationEq. 1(1)
(1) .
Water absorption
The water absorption capacity was measured using the centrifugation method proposed by Jamsiah et al. (Citation2013). The samples (1.0 g) were dispersed in 20 ml of distilled water and placed in centrifuge tubes. Then, the dispersions were stirred and left for 24 h, followed by centrifugation for 25 min at 6000 rpm. The supernatant was discarded and the sediment weighed. The water absorption capacity was expressed as grams of water bound per gram of the sample on a dry basis. The data were collected in triplicate to obtain a mean value.
Density
The density was measured using gas intrusion under a helium gas flow with an AccuPyc 1340 pycnometer. Five measurements were conducted at 27°C and the average value was computed.
Chemical composition
The chemical composition was analysed according to neutral detergent fibre (NDF), acid detergent fibre (ADF), acid detergent lignin (ADL) and ash content analysis, as in Razali et al. (Citation2015).
Thermogravimetric analysis (TGA)
Thermogravimetric analysis was used to characterize the thermal degradation behaviour of the material with respect to weight loss due to increase in temperature. TGA was performed with a Q series thermal analysis machine from TA Instruments (New Castle, DE, USA). The analysis was carried out in aluminium pans under a dynamic nitrogen atmosphere in the temperature range 25–900°C at a heating rate of 10°C min−1.
FT-IR analysis
Fourier transform infrared (FT-IR) spectroscopy was used to detect the presence of functional groups existing in the seaweed. Spectra of the material were obtained using an IR spectrometer (Nicolet 6700 AEM). Approximately 2 mg of the sample, in powder form, was mixed with potassium bromide (KBr) and pressed into a disc ~1 mm thick. FT-IR spectra of the sample were collected in the range of 4000–400 cm−1.
X-ray diffraction (XRD)
The structures of the seaweed solid waste were evaluated with an X-ray diffractometer (APD 2000, Italy), operated at 40 kV and 30 mA, equipped with Cu Kα radiation at a wavelength of 0.15406 nm. The samples were scanned over the range of diffraction angle 2ϴ = 5 to 60° with a scanning rate of 2° min−1 at room temperature.
Results and discussion
Physical properties
The dried and ground seaweeds were analysed for particle size. It was found that the mean diameter of seaweed waste was 120 μm, whereas raw seaweed showed a smaller mean diameter of 115 μm (). The morphology of the seaweed particles was analysed using SEM. Both raw seaweed and seaweed waste have similar flake-like morphologies, with a higher number of smaller particles in raw seaweed compared with seaweed waste (, ). A similar microstructure was observed for seaweed particles being dried using the sun-drying method (Jamsiah et al., Citation2013). This finding is in agreement with the results from the particle size analysis. Particle size is an important characteristic that may influence the physicochemical properties of a material, such as water absorption and swelling characteristics (Singh et al., Citation2003).
Fig. 3. Particle size distribution of Eucheuma cottonii (a) raw seaweed particles (b) solid waste particles.
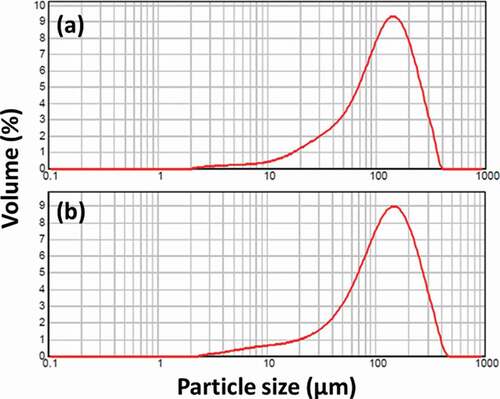
Moisture content is an important characteristic that should be taken into account before considering a new natural material as a potential filler for polymer composites. High moisture content may undermine the stability of the composite in terms of dimensions, tensile strength and porosity formation (Razali et al., Citation2015), therefore, lower moisture content is desirable. The moisture content of both raw seaweed and seaweed waste from this study (), is low (1.13 and 0.75 wt% respectively) compared with other natural fibres (kenaf and jute), which have around 3–5% (Saheb & Jog, Citation1999). This might be attributed to preliminary heating of the seaweed, which removes some of the moisture. Raw seaweed has a slightly higher moisture content than seaweed waste, suggesting that the loss of volatile compounds during seaweed extraction also reduced the moisture content. The low moisture content of seaweed is a good characteristic of this waste as a potential filler for polymer composites.
Table 1. Physical properties of seaweeds and other materials
The water absorption of seaweed is shown in . The results indicate a higher water absorption by seaweed waste (8.65 g g−1) compared with raw seaweed (5.21 g g−1). These results are comparable with the water absorption of the green seaweed species, Ulva lactuca, which has 6.6 g g−1 water absorption capacity (Yaich et al., Citation2011). The higher water absorption capacity of seaweed waste might be attributed to larger particle size, which favours absorption. In addition, cellulose content is another notable factor that affects the water absorption of the material. This might be attributed to the existence of a free hydroxyl group in the cellulose, which attract water molecules from the surrounding environment through hydrogen bonding, causing higher water absorption kinetics (Islam et al., Citation2012). Thus, higher cellulose content in seaweed waste causes an increase in water absorption compared with raw seaweed.
The weight of the material is one of the most important factors in the material selection process, since it may affect the performance of the end products. Density of the material is the main criterion that directly correlates to this property. Both types of seaweed (raw and waste) have comparable densities of 1.70 and 1.73 g cm−3 respectively (). These values are similar to other brown seaweed species, namely Laminaria hyperborea (1.70 g cm−3) and Sargassum muticum (1.70 g cm−3) (Freitas et al., Citation2008). Nevertheless, the density of seaweed is lower than conventional glass fibre (2.5 g cm−3) but slightly higher than other natural fibres such as flax (1.6 g cm−3) and roselle (1.4 g cm−3) (Razali et al., Citation2015). In addition, it was observed that seaweed waste had a slightly higher density than raw seaweed. This might be attributed to the lower moisture content of seaweed waste, since the increase in mass due to moisture gain was lower than the accompanying volumetric expansion of the sample (Kibar & Esen, Citation2010). Similar findings have been reported for rice seed (Kibar & Esen, Citation2010).
Chemical composition
Whereas the crude fibre contents (cellulose, hemicellulose and lignin) of many terrestrial biomass types have been studied intensively, little work has been done on seaweed. shows the chemical composition of Eucheuma cottonii (raw and waste) and other red seaweeds in published work including Sarconema scinaioides, Gracilaria edulis and Gelidiella acerosa. Generally, higher amounts of cellulose, hemicellulose and ash were observed for seaweed waste as compared with the raw sample. This finding might be attributed to the dissolution of some extractives from seaweed during processing, leaving a higher fraction of the non-soluble part in the seaweed waste (Tan & Lee, Citation2014). The result also shows that increase of cellulose and hemicelluloses content is accompanied by a decrease of lignin. This finding is in good agreement with that of Razali et al. (Citation2015). A lower cellulose content (2.0 wt%) was reported for E. cottonii from India (Siddhanta et al., Citation2009), this might be attributed to the different environment for growth, which affects the chemical composition of the seaweed (Harley et al., Citation2012).
Table 2. Comparison of the chemical composition of seaweed
In addition, the cellulose content of E. cottonii in this study was higher than other seaweed species, namely S. scinaioides and Gracilaria edulis, but lower than Gelidiella acerosa (Siddhanta et al., Citation2009). The low crude fibre content of seaweed as compared with other terrestrial biomass corresponds to the higher amount of organic extractives in the composition, such as carbohydrates (42–66 wt%), proteins (8–43 wt%), and lipids (0.2–1.8 wt%) (Hong et al., Citation2014). In contrast, terrestrial biomass produces cell walls that mainly consist of lignocellulose material.
Thermal analysis
The thermal degradation characteristics of Eucheuma cottonii are shown on the TG and DTG curves (). It was observed that the thermal degradation onset temperature of raw seaweed occurs at 211°C and the secondary degradation temperature occurs at 699°C. Meanwhile, the DTG curve shows that the onset temperature of seaweed waste shifted to a higher temperature (246°C), but a slight decrease in the secondary degradation temperature (696°C) was observed. The higher thermal stability of seaweed waste might be attributed to the dissolution of polysaccharide (carrageenan) into the aqueous solution during the hot alkaline extraction process. Thus, the amounts of volatile components in the seaweed waste were lower than in raw seaweed. The slight decrease in the final degradation temperature might be attributed to the lower lignin content in the seaweed waste. The thermal degradation onset temperature of seaweed waste is comparable with other natural fibres such as kenaf (244°C) and roselle (220°C); however, seaweed possesses advantages in terms of a higher secondary degradation temperature compared with kenaf (356°C) and roselle (350°C) (Lee et al., Citation2013; Razali et al., Citation2015).
In general, three stages of thermal decomposition were observed. This finding agrees with the thermal characteristics of other seaweed species, namely Myriogramme manginii, Plocamium cartilagineum and Gigartina skottsbergii (Kebelmann et al., Citation2013). Weight loss during the first stage of decomposition (below 200°C) corresponds to the dehydration and evaporation of highly volatile compounds (Kebelmann et al., Citation2013). During the second stage (180–450°C), various volatile components were decomposed, and the decomposition is attributed to the degradation of carbohydrate and protein, where the main weight loss at this temperature corresponds to the high amount of these two components (Sanchez-Silva et al., Citation2013). A previous study on the nutrient content of E. cottonii confirmed that this species has large proportions of carbohydrate (57 wt%) and protein (4.5 wt%) in its composition (Abirami & Kowsalya, Citation2011). Apart from that, decomposition of hemicellulose and the initial stage of the degradation of cellulose also take place between 200°C and 270°C, while the degradation of lignin and the final degradation of cellulose occur between 270°C and 370°C (Ross et al. Citation2008). The degradation above 500°C might be attributed to carbonate decomposition, and as the temperature increases, the residue continues to decompose, leading to char formation (Ross et al., Citation2008). Finally, the last stage corresponds to the oxidation of the remaining char formed (Sanchez-Silva et al. Citation2013). It was observed that approximately 17 wt% and 15 wt% of the char was not completely oxidized for the control and waste seaweed respectively. The residual char represents the remaining materials after all the volatile materials have been eliminated through the pyrolysis process (Razali et al., Citation2015). For terrestrial biomass, lignin was reported to be the main constituent of the residual char; however, for macroalgae, carbonate composition represents the main constituent in addition to lignin (Chan et al., Citation2013). The thermal degradation characteristics of E. cottonii are in agreement with those reported in previous works focused on the study of the pyrolytic behaviour of macroalgae (Li et al., Citation2011; Kebelmann et al., Citation2013; Sanchez-Silva et al., Citation2013).
FT-IR analysis
The FT-IR spectra for raw seaweed and seaweed waste were almost identical (). Seven predominant peaks were presented by the raw seaweed spectra, at 3443 cm−1, 2927 cm−1, 1648 cm−1, 1520 cm−1, 1378 cm−1, 1265 cm−1 and 1071 cm−1. The intense peaks at 3443 cm−1 indicated the presence of O-H groups in all parts of the filler, due to the hydroxyl groups found in celluloses, hemicelluloses and lignin (Sahari et al., Citation2012). These peaks also represent O-H and N-H stretching (Kang et al., Citation2011). Peaks at 1648 cm−1 represent carbonyl groups stretching (C=O) in hemicelluloses and lignin (Kang et al., Citation2011). The strong peaks at 2927 cm−1 and 1071 cm−1 can be assigned to C-H stretching and C-O stretching respectively (Kang et al., Citation2011). The peaks at 1520 cm−1 correspond to the stretching of the aromatic groups present in lignin (Sahari et al., Citation2012). The peaks at 1378 cm−1 and 1265 cm−1 correspond to methyl (CH3) bending and C-O-C stretching respectively (Kang et al., Citation2011).
The other functional groups correspond to seaweed polysaccharides, namely 3,6-anhydro-D-galactose at 925–935 cm−1 and D-galactose-4-sulphate at 840–850 cm−1. In addition, the spectra of methyl group also indicate the presence of seaweed polysaccharide (Pereira et al., Citation2003).
Meanwhile, the seaweed waste shows slight differences in the projected spectra compared to the raw seaweed. The change of peak at 1500–1300 cm−1 for the seaweed wastes indicates that the methyl group (CH3) and seaweed hydrocolloids were involved during seaweed processing. The peak at 1265 cm−1 has shifted to 1260 cm−1 indicating the involvement of C-O-C groups in the process. In addition, the peak at 1071 cm−1 shifted to 1068 cm−1 which shows that C-O groups were involved during the processing as well. Furthermore, the functional groups of seaweed polysaccharide, namely 3,6-anhydro-D-galactose and D-galactose-4-sulphate, also experience peak shifting from 848 to 846 cm−1 and 926 to 930 cm−1 respectively. The existence of residual polysaccharides in seaweed waste might contribute to additional strengthening effects when used as a filler in polymer due to the high gel strength possessed by this material.
X-ray diffraction (XRD) analysis
The XRD patterns of raw seaweed and seaweed waste are displayed to compare the peak transformation after seaweed processing (). Several crystalline peaks were found on the raw seaweed, indicating its crystalline nature. In raw seaweed, many potassium chloride (KCI) phases (2Ɵ = 28.38°, 40.52° and 50.10°) were detected and sodium chloride (NaCl) phases (2Ɵ =31.60° and 45.40°) were also confirmed. A previous study also reported the presence of KCl and NaCl in other seaweed species, namely Gracilaria cacalia, Enteromorpha clathrata and Sargassum natans (Wang et al., Citation2008). It can be seen that the height of crystalline peaks for seaweed waste were reduced compared with the raw seaweed, which indicates lower intensity of crystalline phases in the material. This might be attributed to dissolution of the crystalline phases into aqueous solution during processing. This finding is in agreement with Wang et al. (Citation2008). It was observed that KCl is the main substance that dissolved after NaCl. A previous study has reported KCl peaks on the X-ray diffractogram of seaweed polysaccharide, which confirms the dissolution of this component during the extraction process (Martins et al., Citation2012). From these results, it can be concluded that the structure of the seaweed wastes has been changed after the processing.
Conclusions
Seaweed wastes of Eucheuma cottonii species from Sabah, Malaysia were characterized by electron microscopy, XRD, TGA and FT-IR. The physical properties of this filler were assessed as well. Generally, seaweed wastes possess certain advantages in terms of higher crude fibre content and better thermal stability. Raw seaweed has a slightly lower density and water absorption capacity than seaweed wastes. FT-IR and XRD analysis showed that the seaweed wastes had undergone prior processing which resulted in changes in their structure. The characteristics shown by E. cottonii waste show that this marine alga also possesses potential as a renewable filler for eco-friendly materials.
Disclosure statement
No potential conflict of interest was reported by the author(s).
Additional information
Funding
Notes on contributors
R. Jumaidin
R. Jumaidin: original concept, drafting manuscript; S.M. Sapuan: original concept and editing manuscript; M. Jawaid: thermal analysis and editing manuscipt; M.R Ishak: experimental concept; J. Sahari: original concept.
S. M. Sapuan
R. Jumaidin: original concept, drafting manuscript; S.M. Sapuan: original concept and editing manuscript; M. Jawaid: thermal analysis and editing manuscipt; M.R Ishak: experimental concept; J. Sahari: original concept.
M. Jawaid
R. Jumaidin: original concept, drafting manuscript; S.M. Sapuan: original concept and editing manuscript; M. Jawaid: thermal analysis and editing manuscipt; M.R Ishak: experimental concept; J. Sahari: original concept.
M. R. Ishak
R. Jumaidin: original concept, drafting manuscript; S.M. Sapuan: original concept and editing manuscript; M. Jawaid: thermal analysis and editing manuscipt; M.R Ishak: experimental concept; J. Sahari: original concept.
J. Sahari
R. Jumaidin: original concept, drafting manuscript; S.M. Sapuan: original concept and editing manuscript; M. Jawaid: thermal analysis and editing manuscipt; M.R Ishak: experimental concept; J. Sahari: original concept.
References
- Abirami, R.G. & Kowsalya, S. (2011). Nutrient and nutraceutical potentials of seaweed biomass Ulva lactuca and Kappaphycus alvarezii. Journal of Agriculture Science and Technology, 5: 1–7.
- Al-Oqla, F.M., Sapuan, M.S., Ishak, M.R. & Aziz, N.A. (2014). Combined multi-criteria evaluation stage technique as an agro waste evaluation indicator for polymeric composites: date palm fibers as a case study. BioResources, 9: 4608–4621.
- Bulota, M. & Budtova, T. (2015). PLA/algae composites: morphology and mechanical properties. Composites Part A: Applied Science and Manufacturing, 73: 109–115.
- Chan, S.W., Mirhosseini, H., Taip, F.S., Ling, T.C. & Tan, C.P. (2013). Comparative study on the physicochemical properties of κ-carrageenan extracted from Kappaphycus alvarezii (Doty) Doty ex Silva in Tawau, Sabah, Malaysia and commercial κ-carrageenans. Food Hydrocolloids, 30: 581–588.
- Freitas, O.M.M., Martins, R.J.E., Delerue-Matos, C.M. & Boaventura, R.A.R. (2008). Removal of Cd(II), Zn(II) and Pb(II) from aqueous solutions by brown marine macro algae: kinetic modelling. Journal of Hazardous Materials, 153: 493–501.
- Harley, C.D.G., Anderson, K.M., Demes, K.W., Jorve, J.P., Kordas, R.L., Coyle, T.A. & Graham, M.H. (2012). Effects of climate change on global seaweed communities. Journal of Phycology, 48: 1064–1078.
- Hong, I.K., Jeon, H. & Lee, S.B. (2014). Comparison of red, brown and green seaweeds on enzymatic saccharification process. Journal of Industrial and Engineering Chemistry, 20: 2687–2691.
- Islam, M.S., Hamdan, S., Rusop, M., Rahman, M.R., Ahmed, A.S. & Idrus, M.A.M.M. (2012). Dimensional stability and water repellent efficiency measurement of chemically modified tropical light hardwood. Bioresources, 7: 1221–1231.
- Jamsiah, S., Ramli, N., Daik, R. & Yarmo, M.A. (2013). Determination of the functional properties of Kappaphycus alvarezii seaweed powder. Malaysian Journal of Analytical Sciences, 17: 236–243.
- Jang, Y.H., Han, S.O., Sim, I.N. & Kim, H.-I. (2013). Pretreatment effects of seaweed on the thermal and mechanical properties of seaweed/polypropylene biocomposites. Composites Part A: Applied Science and Manufacturing, 47: 83–90.
- Jawaid, M., Abdul Khalil, H.P.S. & Abu Bakar, A. (2011). Woven hybrid composites: tensile and flexural properties of oil palm-woven jute fibres based epoxy composites. Materials Science and Engineering A, 528: 5190–5195.
- Kang, O.L., Ramli, N., Said, M., Ahmad, M., Yasir, S.M. & Ariff, A. (2011). Kappaphycus alvarezii waste biomass: a potential biosorbent for chromium ions removal. Journal of Environmental Sciences, 23: 918–922.
- Kebelmann, K., Hornung, A., Karsten, U. & Griffiths, G. (2013). Thermo-chemical behaviour and chemical product formation from Polar seaweeds during intermediate pyrolysis. Journal of Analytical and Applied Pyrolysis, 104: 131–138.
- Kibar, H. & Esen, B. (2010). The effect of moisture content on physical and mechanical properties of rice (Oryza sativa L.). Spanish Journal of Agriculture Reasearch, 8: 741–749.
- Lee, J.M., Mohd Ishak, Z.A., Mat Taib, R., Law, T.T. & Ahmad Thirmizir, M.Z. (2013). Mechanical, thermal and water absorption properties of kenaf-fiber-based polypropylene and poly(butylene succinate) composites. Journal of Polymers and the Environment, 21: 293–302.
- Lee, M.W., Han, S.O. & Seo, Y.B. (2008). Red algae fibre/poly(butylene succinate) biocomposites: the effect of fibre content on their mechanical and thermal properties. Composites Science and Technology, 68: 1266–1272.
- Li, D., Chen, L., Zhang, X., Ye, N. & Xing, F. (2011). Pyrolytic characteristics and kinetic studies of three kinds of red algae. Biomass and Bioenergy, 35: 1765–1772.
- Martins, J.T., Cerqueira, M.A., Bourbon, A.I., Pinheiro, A.C., Souza, B.W.S. & Vicente, A.A. (2012). Synergistic effects between κ-carrageenan and locust bean gum on physicochemical properties of edible films made thereof. Food Hydrocolloids, 29: 280–289.
- Mechakra, H., Nour, A., Lecheb, S. & Chellil, A. (2015). Mechanical characterizations of composite material with short Alfa fibers reinforcement. Composite Structures, 124: 152–162.
- Peddireddy, S., Longhurst, P.J. & Wagland, S.T. (2015). Characterising the composition of waste-derived fuels using a novel image analysis tool. Waste Management, 40: 9–13.
- Pereira, L., Sousa, A., Coelho, H., Amado, A.M. & Ribeiro-Claro, P.J.A. (2003). Use of FTIR, FT-Raman and 13C-NMR spectroscopy for identification of some seaweed phycocolloids. Biomolecular Engineering, 20: 223–228.
- Pereira, L. & Van De Velde, F. (2011). Portuguese carrageenophytes: carrageenan composition and geographic distribution of eight species (Gigartinales, Rhodophyta). Carbohydrate Polymers, 84: 614–623.
- Razali, N., Salit, M.S., Jawaid, M., Ishak, M.R. & Lazim, Y. (2015). A study on chemical composition, physical, tensile, morphological, and thermal properties of roselle fibre: effect of fibre maturity. Bioresources, 10: 1803–1823.
- Ross, A.B., Jones, J.M., Kubacki, M.L. & Bridgeman, T. (2008). Classification of macroalgae as fuel and its thermochemical behaviour. Bioresource Technology, 99: 6494–6504.
- Sahari, J., Sapuan, S.M., Ismarrubie, Z.N. & Rahman, M.Z.A. (2012). Physical and chemical properties of different morphological parts of sugar palm fibres. Fibres and Textiles in Eastern Europe, 91: 21–24.
- Saheb, D.N. & Jog, J.P. (1999). Natural fiber polymer composites: a review. Advances in Polymer Technology, 18: 351–363.
- Sanchez-Silva, L., López-González, D., Garcia-Minguillan, A.M. & Valverde, J.L. (2013). Pyrolysis, combustion and gasification characteristics of Nannochloropsis gaditana microalgae. Bioresource Technology, 130: 321–331.
- Santos, G.A. (1989). Carrageenans of species of Eucheuma J. Agardh and Kappaphycus Doty (Solieriaceae, Rhodophyta). Aquatic Botany, 36: 55–67.
- Sastra, H.Y., Siregar, J.P., Sapuan, S.M. & Hamdan, M.M. (2006). Tensile properties of Arenga pinnata fiber-reinforced epoxy composites. Polymer-Plastics Technology and Engineering, 45: 149–155.
- Siddhanta, A.K., Prasad, K., Meena, R., Prasad, G., Mehta, G.K., Chhatbar, M.U., Oza, M.D., Kumar, S. & Sanandiya, N.D. (2009). Profiling of cellulose content in Indian seaweed species. Bioresource Technology, 100: 6669–6673.
- Singh, N., Singh, J., Kaur, L., Sodhi, N.S. & Gill, B.S. (2003). Morphological, thermal and rheological properties of starches from different botanical sources. Food Chemistry, 81: 219–231.
- Tan, I.S. & Lee, K.T. (2014). Enzymatic hydrolysis and fermentation of seaweed solid wastes for bioethanol production: an optimization study. Energy, 78: 53–62.
- Truong, M., Zhong, W., Boyko, S. & Alcock, M. (2009). A comparative study on natural fibre density measurement. Journal of the Textile Institute, 100: 525–529.
- Wang, S., Jiang, X.M., Han, X.X. & Wang, H. (2008). Fusion characteristic study on seaweed biomass ash. Energy and Fuels, 22: 2229–2235.
- Yaich, H., Garna, H., Besbes, S., Paquot, M., Blecker, C. & Attia, H. (2011). Chemical composition and functional properties of Ulva lactuca seaweed collected in Tunisia. Food Chemistry, 128: 895–901.
- Zhang, Z., Pang, T., Li, Q., Zhang, L., Li, L. & Liu, J. (2015). Transcriptome sequencing and characterization for Kappaphycus alvarezii. European Journal of Phycology, 50: 400–407.