ABSTRACT
Recent morphology-based investigations of freshwater Arctic diatoms suggest that many species remain to be discovered, reflecting a unique polar flora. During a survey of the freshwater diatom flora of northern Billefjorden, including the Petuniabukta fjord region (Spitsbergen), several morphodemes belonging to the Achnanthidium minutissimum species complex were recorded. Molecular phylogenies based on rbcL, 28S and 18S sequences, including single cells from Canada and strains from Marion Island (sub-Antarctica) and GenBank revealed the presence of 12 distinct A. minutissimum complex lineages, of which three contained strains from Spitsbergen. One Arctic lineage is described as a new species. Achnanthidium digitatum sp. nov. is morphologically characterized by narrow, linear to only slightly lanceolate valves and usually two areolae per stria. The two remaining Arctic lineages are in need of a more complete morphological and molecular comparison with other representatives of the A. minutissimum complex to clarify their taxonomic identity. It is argued that implementation of molecular data in the taxonomy of Achnanthidium will be essential to solve the taxonomic problems associated with this group, eventually resulting in a better understanding of the biogeography and niche differentiation of different species belonging to the A. minutissimum complex. Ideally, this should be based on more variable genes than the currently widely used 18S, which does not have a species level resolution in the A. minutissimum complex.
Introduction
During the 20th century, several large-scale studies were carried out on the freshwater diatom flora of the High Arctic including Spitsbergen (e.g. Foged, Citation1964; for a detailed overview see Douglas & Smol, Citation2010). However, several recent investigations using a refined morphotaxonomy and modern technologies (e.g. high resolution scanning electron microscopy) have resulted in the discovery of a large number of taxa new to science (e.g. Van de Vijver et al., Citation2004; Antoniades et al., Citation2008, Citation2009; Pinseel et al., Citation2014, Citation2015). This suggests that the Arctic diatom flora is more species-rich than previously reported and that many more species are yet to be discovered (Pinseel et al., Citation2016). Foged (Citation1981) previously observed that extensive taxonomic surveys of the High Arctic show a higher diversity than observed in most ecological studies. Genera with small-celled representatives such as Achnanthidium Kützing are thought to be especially prone to species diversity underestimation, as subtle (ultrastructural) morphological differences were often not distinguished in past surveys. In this regard, A. minutissimum s.l. (Kützing) Czarnecki is especially relevant since it is one of the most dominant diatoms in Arctic freshwater benthic environments (e.g., Pinseel et al., Citation2016; Zgrundo et al., Citation2016).
Achnanthidium, as defined by Round & Bukhtiyarova (Citation1996), is a widespread diatom genus in various freshwater habitats and comprises two morphologically distinct groups of species. The A. pyrenaicum (Hustedt) Kobayasi group is characterized by distal raphe fissures that are unilaterally deflected or hooked whereas the A. minutissimum group has simple, straight distal raphe endings (Round & Bukhtiyarova, Citation1996; Kobayasi, Citation1997). In general, taxonomy and species identifications in Achnanthidium are problematic due to: (1) the small valve size impeding the observation of diagnostic morphological characters in light microscopy, (2) the lack of clear morphological criteria for species delineation in both LM and SEM and (3) the limited number of studies addressing type material (i.e. Hlúbiková et al., Citation2011; Morales et al., Citation2011). This is especially the case for A. minutissimum, considered to be one of the most common diatom taxa in the world, occurring in all types of freshwater habitats (Potapova & Hamilton, Citation2007). However, Krammer & Lange-Bertalot (Citation1991) already argued that A. minutissimum comprises various morphodemes that presumably correspond with separate species and Potapova & Hamilton (Citation2007) noted that different morphodemes show distinct ecological preferences. Detailed study of ultrastructural variation offers opportunities to separate different morphodemes from A. minutissimum s.l. and in recent years, several taxa have already been described or redefined in the A. minutissimum complex (among others, Hlúbiková et al., Citation2011; Kulikovskiy et al., Citation2011; Morales et al, Citation2011; Novais et al., Citation2011; Taylor et al., Citation2011; Van de Vijver et al., Citation2011a, Citationb; Wojtal et al., Citation2011; Witkowski et al., Citation2012; Van de Vijver & Kopalová, Citation2014; Novais et al., Citation2015).
Although several Achnanthidium sequences (mainly of the A. minutissimum complex) are available on GenBank, molecular-based investigations into the species boundaries of Achnanthidium are entirely lacking. In contrast, all currently available studies using Achnanthidium sequences almost exclusively focus on understanding the phylogenetic relationships within the Monoraphidae or the diatoms in general (e.g. Bruder & Medlin, Citation2007; Rimet et al., Citation2011; Nakov, Citation2014; Kulikovskiy et al., Citation2016). However, molecular phylogenies based on DNA sequences from fast-evolving molecular markers are highly useful for species discovery (e.g. Evans et al., Citation2008; Vanormelingen et al., Citation2013; Lefevbre & Hamilton, Citation2015; Pinseel et al., Citation2017) and are likely to be a valuable tool for species level taxonomy in Achnanthidium. Use of molecular markers demonstrates that species boundaries based solely on morphology can be inaccurate (e.g. De Clerck et al., Citation2005; Quijano-Scheggia et al., Citation2009; Trobajo et al., Citation2009; Moniz et al., Citation2012). This is sometimes due to unrecognized phenotypic plasticity (Mann, Citation1999). However in most cases there are problems in visually recognizing subtle morphological discontinuities between species (e.g. Amato et al., Citation2007) or there is a complete lack of distinguishing morphological characters (e.g. Souffreau et al., Citation2013; Vanormelingen et al., Citation2013). Recently, species have been defined as separately evolving metapopulation lineages that acquire differences in molecular, morphological, ecological, reproductive, physiological and behavioural properties at different times during the speciation process and not necessarily in the same order (De Queiroz, Citation2007). In this regard, the existence of distinct lineages in molecular phylogenies, i.e. clusters of sequences showing little intracluster divergence preceded by a relatively long and statistically highly supported branch, can give primary indications of the presence of different species (Vanormelingen et al., Citation2013). Further, when the studied molecular markers do not have a species level resolution, they can indicate trajectories of speciation where sequence clusters comprise several closely related but distinct species. This evidence for the presence of different species can be strengthened by the correlation between different molecular markers and other properties such as morphology and ecology (e.g. Burns et al., Citation2008; Vanelslander et al., Citation2009). Therefore, except for a few algal groups such as diatoms, species are today rarely defined by morphological characteristics alone (Leliaert et al., Citation2014).
The present paper discusses several representatives of the A. minutissimum complex found in freshwater ponds and lakes in northern Billefjorden, including Petuniabukta (Spitsbergen, High Arctic). Availability of cultured material allowed us to examine molecular differences in species and include a molecular phylogeny. Both morphological and molecular data support the description of Achnanthidium digitatum Pinseel, Vanormelingen, Hamilton & Van de Vijver sp. nov. as a new species.
Materials and methods
Field sampling
The study area is located in the northern part of Billefjorden, the inner branch of Isfjorden in the central part of Spitsbergen (Svalbard Archipelago, High Arctic) and includes the Petuniabukta fjord area (78°33′–78°45′N and 16°25′–16°40′E). Details of the study area are given by Pinseel et al. (Citation2016). During the boreal summer seasons of 2011 and 2013, more than 80 epilithon, epipelon and epiphyton samples of the littoral zones from 53 lakes and ponds were taken for diatom analysis. For each water body, the epilithon of five to 10 stones originating from the littoral zone was scraped off using a knife. Epipelon samples were taken by scraping off the upper 0.5 cm of the sediments in the littoral zone using a Falcon tube. For the epiphyton, above-ground parts of mosses were cut and transferred to a Falcon tube. In order to prevent cross-contamination between samples, all sampling equipment was washed before and after sampling. Diatom samples for morphological analysis were fixed with 96% ethanol for preservation immediately upon collection. Samples for live diatom material were transported dark and cool (< 10°C). Water temperature, pH and conductivity were measured using a HANNA Combo pH/EC Tester. Supplementary Table 1 lists all samples of which material was used in the present study together with their GPS positions and measured ecological parameters.
Culture establishment
Clonal cultures of Achnanthidium were established from the natural samples. Upon arrival in the lab, small quantities of the natural material were incubated for a week in WC medium (Guillard & Lorenzen, Citation1972), without pH adjustment or vitamin addition, at 4°C, 20–30 μmol photons m–2 s–1 and a 12:12h light:dark cycle. Diatom cells were isolated from the natural samples under a stereomicroscope using a needle and a micro-pipette and grown at PAE in WC medium at standard culture conditions of 18°C, 20–30 μmol photons m–2 s–1 and a 12:12h light:dark cycle. The cultures were re-inoculated every 2–3 weeks when reaching the late exponential phase. In total, 12 Achnanthidium strains were established but long-term preservation was unsuccessful and the cultures are no longer available.
Sample preparation for morphological analysis
Small sub-samples of the fixed natural samples were prepared for LM observation following the method described in Van der Werff (Citation1955). Small parts of the samples were cleaned by adding 37% H2O2 and heating to 80°C for about 1 h. The reaction was completed by addition of KMnO4. Following digestion and centrifugation (three times 10 min at 3700 × g), cleaned material was diluted with distilled water to avoid excessive concentrations of diatom valves on the slides. Cleaned diatom material was dried on coverslips and mounted onto slides using Naphrax®.
Subcultures for morphological characterization were harvested in late exponential phase with a micropipette and cleaned by adding 65% nitric acid and heating to 60°C for 3 days. Samples were subsequently washed eight times with distilled water and mounted onto slides using Naphrax®.
All slides were studied at ×1000 magnification under oil immersion using an Olympus® BX51 microscope equipped with Differential Interference Contrast (Nomarski) optics. For scanning electron microscopy (SEM), parts of the oxidized suspensions were filtered through a 1 µm Isopore™ polycarbonate membrane filter (Merck Millipore). The stubs were sputter-coated with a Gold-Palladium layer of ~10 nm and studied in a Zeiss Ultra SEM microscope (Natural History Museum London, UK), a JEOL-JSM71001 (Botanic Garden Meise, Belgium) and a JEOL-JSM5600LV (Ghent University, Belgium). Oxidized material of the cultures is kept at PAE and samples and slides are stored in the BR-collection, property of the Belgian federal government and given on permanent loan to the Botanic Garden Meise (Belgium). All material is available upon request.
Diatom terminology on valve outline and raphe structures followed Round et al. (Citation1990) and Krammer & Lange-Bertalot (Citation1991). For comparison, the following publications were consulted: Kobayasi et al. (Citation1986), Potapova & Ponader (Citation2004), Reichardt (Citation2004), Cantonati & Lange-Bertalot (Citation2006), Potapova (Citation2006), Ponader & Potapova (Citation2007), Potapova & Hamilton (Citation2007), Compère & Van de Vijver (Citation2011), Hlúbiková et al. (Citation2011), Jüttner & Cox (Citation2011), Jüttner et al. (Citation2011), Kulikovskiy et al. (Citation2011), Morales et al. (Citation2011), Novais et al. (Citation2011), Taylor et al. (Citation2011), Van de Vijver et al. (Citation2011a, b), Witkowski et al. (Citation2012), Van de Vijver & Kopalová (Citation2014), Novais et al. (Citation2015) and Liu et al. (Citation2016).
DNA extraction and sequencing
Subcultures for genetic characterization were harvested in late exponential phase and centrifuged. DNA extraction was performed following Zwart et al. (Citation1998) using a bead-beating method with phenol extraction and ethanol precipitation. Extracted DNA samples of the cultures are stored at PAE. Standard PCR primers and protocols were used to amplify sequences of the plastid gene rbcL (Daugbjerg & Anderson, Citation1997; Jones et al., Citation2005), the D1–D3 region of the nuclear 28S (LSU rDNA) (Scholin et al., Citation1994; Hamsher et al., Citation2011), a partial sequence of the nuclear 18S (SSU rDNA) including the V4 region (van Hannen et al., Citation1998; Huss et al., Citation1999; Quillou et al., Citation1999; Moon-van Der Staay et al., Citation2000) and the mitochondrial gene cox1 (Evans et al., Citation2007). However, all PCRs of cox1 failed due to amplification of bacterial DNA instead of diatom cox1. PCR products were transported to and sequenced by Macrogen, Inc.
Phylogenetic analysis
Psammothidium was chosen as outgroup for the A. minutissimum complex based on Nakov (Citation2014) and Kulikovskiy et al. (Citation2016) and sequences obtained from clonal cultures identified as P. abundans (Manguin) Bukhtiyarova & Round and P. papilio (D.E.Kellogg, M.Stuiver, T.B.Kellogg & G.H.Denton) Kopalová & Van de Vijver originating from Marion Island (sub-Antarctica) and Schirmacher Oasis (Continental Antarctica) respectively (Supplementary Table 1). In order to expand the molecular phylogeny and provide material for comparison, A. minutissimum complex strain material from clonal cultures established from Marion Island (sub-Antarctica) and two single cell A. minutissimum complex isolates from Ottawa (Canada) were sequenced and included in the phylogeny (Supplementary Table 1), and additional sequences of strains belonging to the A. minutissimum complex were downloaded from GenBank (Bruder & Medlin, Citation2007; Rimet et al., Citation2011; Larras et al., Citation2014; Zimmermann et al., Citation2014; Brinkmann et al., Citation2015; Keck et al., Citation2016; Kulikovskiy et al., Citation2016; Nakov et al., unpublished data; Yamaguchi et al., unpublished data, Supplementary Table 2). GenBank sequences that were distinctly shorter than the overall dataset were removed from further analysis. GenBank strains that belonged to the A. pyrenaicum group were not included in the analysis since Nakov (Citation2014) showed that these are distinct from the A. minutissimum group, clustering together with Psammothidium. This resulted in 36 (rbcL), 20 (28S) and 33 (18S) sequences, outgroup included. Information on sampling methods for Marion Island can be found in Van Nieuwenhuyze (Citation2015) and sampling at Schirmacher Oasis was performed as described in Verleyen et al. (Citation2012). Single cell collection and analysis was performed according to Hamilton et al. (Citation2015). Since the present study only aims to contribute to the Achnanthidium flora of Spitsbergen, the newly obtained sequences from other regions will not be discussed. All newly generated sequences have been deposited in GenBank and the Barcode of Life Database: http://www.boldsystems.org/index.php/Public_SearchTerms?query=DS-ACHN (Ratnasingham & Hebert, Citation2007) (Supplementary Table 2). The latter additionally lists the sampling dates, origin and LM/SEM micrographs.
All sequences were individually edited and automatically aligned using the Clustal-W algorithm (Thompson et al., Citation1994) as implemented in BioEdit 7.2.5 (Hall, Citation1999). All sequences aligned unambiguously without any distinct gaps. In order to minimize the number of missing characters, the ends of all alignments were trimmed. For the phylogeny reconstructions of rbcL, 28S and 18S, respectively 1434, 895 and 1019 sites were used. The best-fit models for nucleotide substitution for each individual locus were chosen in jModelTest2 based on the Bayesian information criterion (Posada, Citation2008; Darriba et al., Citation2012) as implemented in the CIPRES Science Gateway (Miller et al., Citation2010). Due to an underrepresentation of several substitution models in various programs for phylogenetic inference, different models were used in Bayesian analysis (BI) and maximum likelihood phylogenetic inference (ML) in order to choose the most optimal model possible for a given program. Several runs to test the performance of different models on the datasets yielded similar results. In BI the individual gene datasets of rbcL, 28S and 18S were analysed under the GTR+I+G (Tavare, Citation1986), HKY+I (Hasegawa et al., Citation1985) and HKY+I+G models respectively. In ML, GTR+I+G was used for rbcL and GTR+I was chosen for 28S and 18S. For the concatenated analysis of rbcL + 28S, only the rbcL sequences for which a corresponding 28S sequence was available were used, resulting in the GTR+I model for both genes under ML and the HKY+I model for both genes under BI. In order to avoid basal branching due to missing data (mainly 28S), we did not perform a concatenated three marker analysis of the dataset. A concatenated analysis of rbcL + 18S was not included in the study since it yielded no extra information due to the relatively low sequence divergence of 18S and missing data for both genes. All loci were analysed as a single partition. The individual gene and the concatenated datasets were analysed by ML in raxmlGUI (Silvestro & Michalak, Citation2012) using 10 independent runs and 1000 pseudoreplicates. In the concatenated dataset, both genes were partitioned to allow for independent parameter estimates. BI was performed in MrBayes v.3.2.4 (Huelsenbeck & Ronquist, Citation2001; Ronquist & Huelsenbeck, Citation2003). One run with four incrementally heated Metropolis-coupled Monte-Carlo Markov Chains was completed on the single gene datasets of 28S and 18S for 20 million generations and on the concatenated dataset and the single gene dataset of rbcL for 50 million generations. The concatenated dataset was partitioned by gene. Runs were sampled every 1000th generation and convergence and stationarity of the log-likelihood and parameter values were assessed using Tracer v.1.6 (Rambaut et al., Citation2014). The first 25% generations were discarded as burn-in. The post-burn-in tree was summarized and posterior-probabilities (PPs) were calculated in MrBayes using the sumt command. The sequence divergences between different lineages were calculated in MEGA 6.0 (Tamura et al., Citation2013) using p-distance estimation or number of base pair (bp) differences for both transitions and transversions and with pairwise deletion for treatment of gaps and missing data. The single gene alignments used for the phylogenetic analysis are available from TreeBASE (http://purl.org/phylo/treebase/phylows/study/TB2:S19961).
Results
Morphological analysis of natural material
Light microscopy study of the natural samples (Supplementary Table 1) revealed a large number of valves resembling A. minutissimum s.s. with linear to linear-lanceolate valve margins and protracted (slightly) subrostrate, rostrate or subcapitate apices. Detailed SEM observations revealed slight ultrastructural differences. Two morphodemes were most common: one was characterized by linear to slightly linear-lanceolate valves, (slightly) subrostrate apices and two (rarely three) areolae per stria (morphodeme 1: MD1) (–), whereas the second exhibited more clearly linear-lanceolate valves, rostrate, occasionally subcapitate apices and usually three to five areolae per stria (morphodeme 2: MD2) (–). Next to MD1 and MD2, several small-sized (< 10 μm) valves with elliptical margins and rounded apices were observed. It was not always clear from visual LM and SEM analysis whether these valves belonged to small specimens of MD1 and/or MD2 or if they represent one or more other smaller species.
Figs 1–10. Achnanthidium digitatum sp. nov. (MD1). Scanning electron micrographs of a natural sample (SPITS2013/029). Figs 1–3. SEM external view of a raphe valve. Fig. 4. SEM internal view of a raphe valve. Figs 5–8. SEM external view of a rapheless valve. Figs 9–10. SEM internal view of a rapheless valve. Scale= 5 μm.
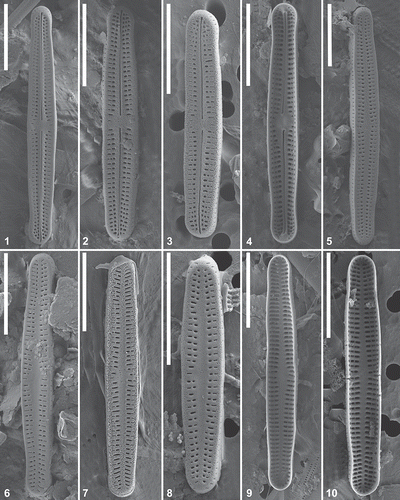
Figs 11–16. Achnanthidium minutissimum complex lineage A (MD2). Scanning electron micrographs of natural material. Fig 11. SEM external view of a raphe valve. Figs 12–13. SEM internal view of a raphe valve. Figs 14–15. SEM external view of a rapheless valve. Fig. 16. SEM internal view of a rapheless valve. Scale = 5 μm.
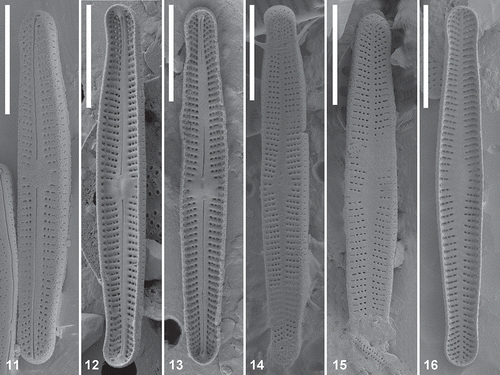
Molecular and morphological analysis of culture material
The plastid gene rbcL was the most variable marker in this study: Achnanthidium strains differed from each other by 0–63 bp (0.0–4.4%) with a mean of 25.2 bp (2.0%). The nuclear encoded 28S gene showed distinctly less variation with Achnanthidium strains only differing by 0–9 bp (0.0–1.7%) with a mean of 4.7 bp (0.6%), whereas they differed by 0–22 bp (0.0–4.2%) with a mean of 8.7 bp (1.1%) for the nuclear encoded 18S gene. However, when only taking into account the strains that were shared between the datasets of both nuclear genes, the variation between the Achnanthidium strains dropped to 0–4 bp (0.0–0.6%) and a mean of 1.5 bp (0.2%) for 18S. The variation of 28S remained the same. rbcL remained relatively variable in the smaller dataset showing 0–46 bp (0.0–3.2%) difference with a mean of 20.3 (1.5%) bp. Therefore, the rbcL and 28S genes were primarily used to delimit different lineages, whereas the larger single marker datasets of rbcL and 18S were studied to gain a wider perspective of the phylogenetic placement of the Arctic strains. An overview of all base pair differences and p-distances between all strains for rbcL, 28S and 18S is given in Supplementary Table 3.
ML and BI phylogenetic analysis on the single marker and concatenated datasets produced identical results, apart from the single marker rbcL and 18S datasets, where an incongruence between tree topologies of ML and BI phylogenetic analysis existed for two or one node(s), indicated as ‘-‘ in the Bayesian node support (; Supplementary Figs 1–2). In the molecular phylogenies, different lineages were defined as groups of sequences with a small number of nucleotide substitutions, in relation to the large number of substitutions between groups. In the rbcL dataset, 11 Achnanthidium lineages (A–K) were recovered with moderately high support, of which several can potentially be further subdivided (, lineages A and F). Three of the lineages contained Arctic Achnanthidium strains (, lineages A, D and I). In the smaller concatenated dataset of rbcL and 28S, four lineages were recovered, all of which correspond with the rbcL phylogeny (Supplementary Fig. 1). The same lineages were recovered from the individual gene dataset of 28S. In the single marker dataset of 18S, six lineages were recovered, including Arctic lineage I (Supplementary Fig. 2). Lineage L was represented by strains not present in the rbcL phylogeny and five sister lineages of the rbcL dataset were collapsed into one lineage in the 18S phylogeny (Supplementary Fig. 2, lineages A, B, D, F and G).
Fig. 17. Maximum likelihood phylogeny of the A. minutissimum complex based on rbcL sequences with indication of statistical support (ML bootstrap proportions/BI posterior probabilities). The different lineages are indicated with grey boxes. The dark grey boxes represent the Arctic lineages. The geographic regions where each lineage has been found are indicated.
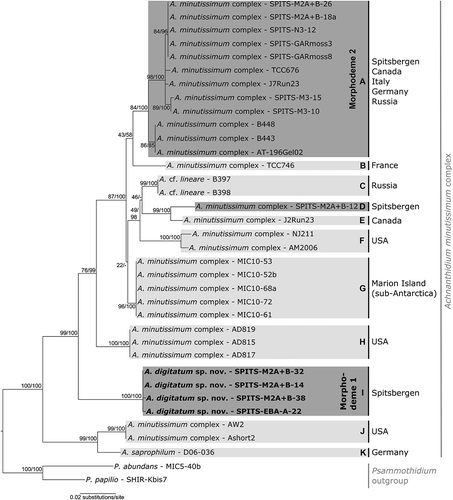
Morphological analysis of culture material in LM () and SEM (–, Supplementary Figs 3–26) showed that two of the three Arctic Achnanthidium lineages corresponded with MD1 (, lineage I, four strains) and MD2 (, lineage A, seven strains). The third lineage (, lineage D) was represented by only one strain with smaller valves and a linear-elliptical valve outline. The other lineages in the phylogeny consisted of sequences of strains from Marion Island (, lineage G), a single cell isolate from Canada (, lineage E) or GenBank (; Supplementary Figs 1–2, lineages B, C, F, H, J, K and L). Several sequences from GenBank identified as A. minutissimum or A. saprophilum (Kobayashi & Mayama) Round & Bukhtiyarova belong to different lineages in the phylogenies. The Arctic lineages differed distinctly from each other: lineage A (MD2) and lineage I (MD1) differed by 40–43 bp (2.8–3.0%) for rbcL, 9 bp (1.0%) for 28S and 1–4 bp (0.2–0.6%) for 18S. The third smaller morphodeme (lineage D) differed from lineage A (MD2) by 26–28 bp (1.8–2.0%) for rbcL, 4 bp (0.8%) for 28S and 0–2 bp (0.0–0.2%) for 18S and from lineage I (MD1) by 45–46 bp (3.1–3.2%) for rbcL, 7 bp (0.4–0.5%) for 28S and 3–4 bp (0.4–0.6%) for 18S. Only the Arctic strains were taken into account for these calculations.
Figs 18–37. Achnanthidium digitatum sp. nov. (MD1) and A. minutissimum complex lineage A (MD2). Light microscopy pictures of culture material. Figs 18–22. Achnanthidium digitatum sp. nov. (MD1) LM view of a raphe valve (Figs 18–19: strain SPITS-M2A+B-32 (type material), Figs 20–22. strain SPITS-M2A+B-38). Figs 23–27. Achnanthidium digitatum sp. nov. (MD1) LM view of a rapheless valve (Figs 23–25: strain SPITS-M2A+B-32 (type material), Figs 26–27: strain SPITS-M2A+B-38). Fig 28–32. A. minutissimum complex lineage A (MD2) LM view of a raphe valve (strains SPITS-M3-15, SPITS-N3-12, SPITS-M2A+B-18a, SPITS13-GARmoss8 and SPITS13-GARmoss3 respectively). Figs 33–37. A. minutissimum complex lineage A (MD2) LM view of a rapheless valve (strains SPITS-M3-15, SPITS-N3-12, SPITS-M2A+B-18a, SPITS13-GARmoss8 and SPITS13-GARmoss3 respectively). Scale = 10 μm.
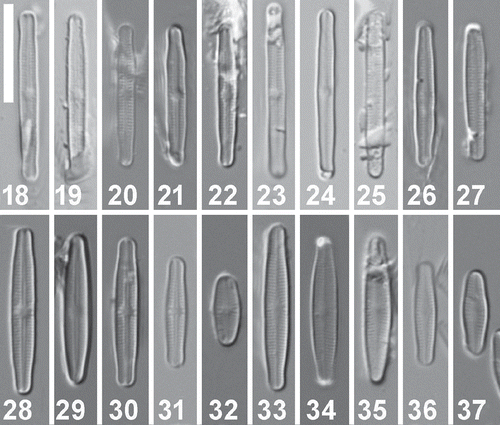
Figs 38–49. Achnanthidium digitatum sp. nov. (MD1). Scanning electron micrographs of culture material. Figs 38–40. SEM external view of a raphe valve (strains SPITS-M2A+B-38, SPITS-M2A+B-32 (type material) & SPITS-EBA-A-22 respectively). Figs 41–43. SEM internal view of a raphe valve (strains SPITS-M2A+B-38, SPITS-M2A+B-32 (type material) and SPITS-EBA-A-22 respectively). Figs 44–46. SEM external view of a rapheless valve (strains SPITS-M2A+B-32 (type material), SPITS-EBA-A-22 & SPITS-M2A+B-14 respectively). Figs 47–49. SEM internal view of a rapheless valve. Fig. 47 represents strain SPITS-M2A+B-32 (type material) and Figs 48–49 represent strain SPITS-EBA-A-22). Scale = 5 μm.
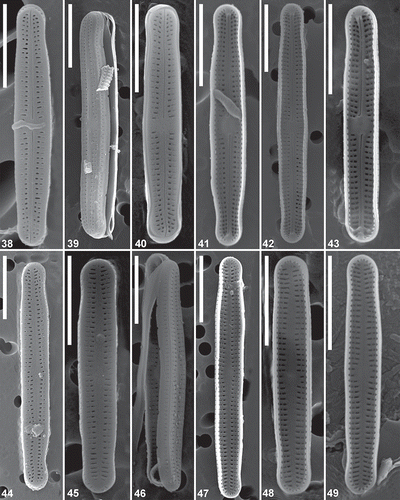
Detailed SEM analysis of the MD2 (lineage A) culture material showed small but consistent morphological differences between different strains revealing two distinct groups. Whereas one was characterized by linear to linear-lanceolate valves, three to four areolae per stria and a small rounded central area on the raphe valve, the other showed clearly strictly linear-lanceolate valves, three to five areolae per striae and a central area on the raphe valve that either forms a broad rectangular fascia or is small and rounded. In the phylogenetic trees, both morphodemes of MD2 turned up as two sublineages within lineage A, which might suggest the presence of more than one species (; Supplementary Fig. 1). However, both sublineages had almost identical sequences for rbcL (3–4 bp difference) and showed no difference in 28S and 18S. Moreover, two strains from Italy (TCC676) and Canada (J7Run23) clustered basally between both Arctic sublineages (). Consequently, all MD2 strains are presently treated as one single lineage potentially showing intraspecific phenotypic variation.
New species
Based on distinct morphological differences from other taxa in the A. minutissimum complex and its presence as a distinct lineage in the molecular phylogenies of all three markers, lineage I (MD1) is described as Achnanthidium digitatum Pinseel, Vanormelingen, Hamilton & Van de Vijver sp. nov. The new species differs from its closest sister lineage (lineage H) by 35–36 bp (2.4–2.6%) for rbcL and 4–5 bp (0.8%) for 18S. Achnanthidium digitatum is morphologically characterized by the combination of narrow, linear to only slightly lanceolate valves with protracted, (slightly) subrostrate apices in the larger specimens and usually two (sometimes three) areolae per stria (–, –). Apart from some occasional deformities in valve shape, the cultures of A. digitatum did not show structural differences compared to A. digitatum valves from the natural material. Lineages A (MD2) and D (from here on referred to as MD3) are in need of a more thorough morphological and molecular examination, as they show close morphological affinities with several species within the complex (–; Supplementary Figs 3–26).
The morphological characterizations of A. digitatum and MD2 are based on natural samples and culture material, whereas MD3 is solely based on culture material as these valves could not be definitively linked to field material. Reliable distinction of different valve types in LM proved to be impossible, and thus we show only LM pictures of cultures (). All measurements were made on SEM pictures. Supplementary Table 4 gives an overview of the main morphological characteristics of the lineages from Spitsbergen, together with a morphological comparison with similar species.
Achnanthidium digitatum Pinseel, Vanormelingen, Hamilton & Van de Vijver sp. nov. (MD1, lineage I) (LM: Figs 18–27, SEM: –, –)
Description: Rectangular frustules arched in girdle view (, ). Cingulum composed of three copulae. Mantle areolae transapically elongated, slit-like (, ). Valves linear to slightly linear-lanceolate with almost parallel margins and protracted, (slightly) subrostrate apices. Apices becoming broadly rounded, not protracted, in smaller specimens (–, –). Valve dimensions (n=20): length 8.6–19.1 μm, width 1.8–2.3 μm. Raphe valves (–, , –) concave. Axial area narrow, linear, only slightly widening towards central area. Axial area almost absent near the apices. Central area small, rounded, bordered by two to three more widely spaced shortened striae (–, –) or forming a clear rectangular fascia (). External raphe straight, filiform with simple, short, slightly widened proximal raphe endings and simple straight distal raphe endings, terminating just beyond the last apical stria (–, –). Internal proximal raphe fissures bent in opposite directions. Internal distal raphe endings straight, terminating in small helictoglossae (, –). Striae (–, , –), 31–36 in 10 μm, moderately radiate throughout, usually becoming more strongly radiate towards the apices. Striae composed of two, rarely three areolae. Areolae close to the axial area rounded or broadly transapically elongated. Margin areolae mostly narrow slit-like. Shortened striae in the central area composed of one, usually narrow slit-like, areola. Near apices, areolae always rounded, organized in rows of two, sometimes three. External areolae on the apices rarely narrow slit-like. Rapheless valves (–, , –) convex with narrow linear axial area, slightly widening towards the central area. Central area almost non-existent, never forming a fascia or subfascia (–, –). Striae (–, –), 31–36 in 10 μm, almost parallel, becoming slightly radiate near the apices. Central striae more widely spaced, composed of two, rarely three, rounded or broadly transapically elongated areolae. Margin areolae broadly transapically elongated or narrow slit-like. Occasionally, shortened striae, composed of one, usually narrow slit-like areola, present in the central area. Near apices, rectangular and slit-like areolae occasionally present.
Holotype: Slide BR-4448 (oxidized material of culture strain SPITS-M2A+B-32), National Botanic Garden of Belgium, Meise.
Isotype: Slide PLP-302 (oxidized material of culture strain SPITS-M2A+B-32), University of Antwerp, Belgium.
Type locality: Epiphyton on moss from a wetland next to a lake at Mathiessondalen (78°33’47.4”N, 16°35’14.1”E), northern Billefjorden (Central Spitsbergen), collected by K. Kopalová, 20 July 2011 (sample SPITS2011/M2A+B).
Pictures of the Type Strain: LM pictures () and SEM pictures (, , , ) taken of culture strain SPITS-M2A+B-32. LM pictures were taken from the holotype slide. SEM pictures were taken from the same batch of oxidized material from which the holo- and isotype slides were prepared.
Sequence data: Nuclear encoded 28S (LSU rDNA) sequence (BOLD: ACHSP008-15, GenBank accession: KU565398), plastid gene rbcL sequence (BOLD: ACHSP008-15, GenBank accession: KU687471) and nuclear encoded 18S (SSU rDNA) sequence (BOLD: ACHSP008-15, GenBank accession: KX946582).
Representative specimens: Mathiessondalen, northern Billefjorden, Central Spitsbergen (78°33’47.4”N, 16°35’14.1”E), sample SPITS2011/M2A+B, 20 July 2011, coll. K. Kopalová, strains SPITS-M2A+B-14 () and SPITS-M2A+B-38 (Figs 20–22, 26, 27, , ). Ebbadalen, Petuniabukta, Central Spitsbergen (78°44’35.4”N, 16°36’50.4”E), sample SPITS-EBA-A, 17 July 2011, coll. K. Kopalová, strain SPITS-EBA-A-22 (, , , , ). BOLD and GenBank reference numbers are given in Supplementary table 2.
Etymology: The specific epithet digitatum refers to the finger-like appearance of the new taxon.
Ecology and associated diatom flora: Achnanthidium digitatum is described from a small lake characterized by an alkaline pH ((8.1–8.4)) with moderately high conductivity (550–761 μS cm−1). At the type locality, it was found in epiphyton (moss) and epilithon samples. Additional populations were observed in various epilithon, epiphyton and epipelon samples from > 40 other localities in the study area with alkaline pH (7.4–10.9, median 8.7) and variable conductivity values (67–1948 μS cm−1, median 319 μS cm−1). Achnanthidium digitatum was generally observed in rather high abundances together with A. minutissimum complex lineage A (MD2). The new species was often observed together with Rossithidium petersenii (Hustedt) Round & Bukhtiyarova, Denticula tenuis Kützing, Denticula kuetzingii Grunow incl. var. rumrichae Krammer, Cymbella botellus (Lagerstedt) A.W.F.Schmidt, Diatoma tenuis C.Agardh and several Nitzschia Hassall taxa resembling Nitzschia perminuta (Grunow) Peragallo.
Distribution and ecology of additional populations: Based on SEM micrographs, additional populations of A. digitatum have been observed in the Canadian Arctic Archipelago (Antoniades et al., Citation2008) and Franz Josef Land (Pla-Rabés et al., Citation2016). The Canadian Arctic ponds and lakes are characterized by an alkaline pH (7.4–8.5, depending on spring melt and sampling time), moderate conductivity values (198–620 μS cm–1), low concentrations of particulate organic carbon (0.3–0.5 mg l–1), dissolved organic carbon (6.3–21 mg l–1), dissolved inorganic carbon (19.5–52.9 mg l–1), particulate nitrogen (30–60 µg l–1), nitrite (9–11 µg l–1), ammonium (2–29 µg l–1), particulate phosphorus (4.8–9.8 µg l–1), total phosphorus (unfiltered) (9.2–20.2 µg l–1), total phosphorus (filtered) (4.4–10.4 µg l–1), calcium (22–36 mg l–1), potassium (1.7–7.6 mg l–1), sodium (6.9–41.3 mg l–1) and sulphate (1.6–14.9 mg l–1) (data taken from Antoniades et al. Citation2008: physicochemical sample preparation and analysis followed the methods of Antoniades et al. (Citation2003) and Environment Canada (Citation1994)).
Comparison with similar species: Several taxa within the A. minutissimum complex closely resemble A. digitatum in their valve morphology. Achnanthidium sublineare Van de Vijver, Jarlman & Ector and A. strictum E.Reichardt can be separated from A. digitatum based on the valve apices that are always acutely rounded in A. sublineare and A. strictum, whereas A. digitatum shows protracted, (slightly) subrostrate apices in the larger specimens. Furthermore, the central area of the raphe valves always forms a rectangular fascia without striae in A. sublineare and A. strictum, whereas in A. digitatum shortened striae usually border the central area. Achnanthidium lailae Van de Vijver has two to three areolae per stria on the valve face of the raphe valves whereas A. digitatum only very rarely exhibits three areolae per stria on the valve face of the raphe valve and the larger valves of A. digitatum are slightly protracted whereas those of A. lailae are always broadly rounded. When compared to all other taxa within the A. minutissimum complex, A. digitatum has a larger length-to-width ratio and more linear valves giving the valves of A. digitatum a very narrow, slender appearance when compared to the broader, more lanceolate valves of A. minutissimum s.l., altogether justifying the description of A. digitatum as a new species.
Achnanthidium minutissimum complex lineage A (MD2) (LM: Figs 28–37, SEM: Supplementary Figs 3–22)
MD2 can be distinguished from A. digitatum by its relatively broader more lanceolate valves and by usually having three to five areolae per stria as compared to two (rarely three) in A. digitatum. However, due to some overlap in valve outline (), reliable identification of both taxa in LM can be difficult. MD2 shares distinct phenotypic features with A. minutissimum s.s., A. neocryptocephalum Novais & Van de Vijver and A. microcephalum Naegeli when regarding valve dimensions, striation patterns and areola configurations. In MD2, the central area of the raphe valve shows different morphologies, either forming a clear rectangular fascia as seen in A. neocryptocephalum, or more often bordered by three shortened striae, which is sometimes seen in A. minutissimum s.s. More rarely, the central area on the raphe valve of MD2 is small, rounded and almost absent, similar to both A. minutissimum s.s. and A. microcephalum. Furthermore, the rapheless valves of MD2 generally exhibit a moderately broad lanceolate axial area with a rhombic lanceolate central area similar to A. neocryptocephalum, whereas A. minutissimum s.s. and A. microcephalum have a narrow linear axial area that only slightly widens towards the central area. Finally, the valves of MD2 cultures are clearly less lanceolate than those of A. neocryptocephalum and A. minutissimum s.s., whereas smaller specimens of MD2 show more affinity with small valves of A. microcephalum. Based on these results, it remains unclear whether MD2 is conspecific with A. minutissimum s.s., A. microcephalum or A. neocryptocephalum or whether it represents a new species. Therefore, a decision about the taxonomic status of MD2 is currently not established until more data for intraspecific morphological variation and molecular studies on Achnanthidium become available, a decision which is reinforced by the observations of clear morphological differences between different strains assigned to MD2 and the finding that several strains from other localities cluster within lineage A.
Other taxa in the A. minutissimum complex show some resemblance to MD2, but can be separated based on small but distinct differences in valve morphology. The sub-Antarctic A. sieminskae Witkowski, Kulikovskiy & Riaux-Gobin shows a high similarity to MD2 in terms of valve dimensions, but never forms a clear rectangular fascia on the raphe valves and often shows parallel to even convergent striae near the apices which is never seen in MD2. Achnanthidium maritimo-antarcticum Van de Vijver & Kopalová has similar valve dimensions as MD2, but possesses a much less pronounced axial area on the rapheless valves, never shows a rectangular fascia on the raphe valves and does not have slit-like areolae as seen in MD2. Achnanthidium pseudolineare Van de Vijver, Novais & Ector exhibits a larger stria density on the raphe valves and has linear-parallel valve margins. Achnanthidium indistinctum Van de Vijver & Kopalová has smaller valve dimensions and lacks axial and central areas on the rapheless valves when compared to MD2. Achnanthidium lineare W.Smith is smaller with broadly rounded, never protracted, apices, a slightly lower stria density and less areolae per striae.
Achnanthidium minutissimum complex lineage D (MD3) (Supplementary Figs 23–26)
MD3 is similar to A. acsiae Wojtal, E.Morales, Van de Vijver & Ector and A. nanum (F.Meister) Novais & Jüttner when comparing valve dimensions, valve outline and striation pattern. However, MD3 shows a broad lanceolate axial area on the rapheless valves and fewer areolae per striae of the rapheless valves than A. acsiae and A. nanum. Achnanthidium sibiricum Kulikovskiy, Lange-Bertalot, Witkowski & Khursevich is highly similar when comparing valve dimensions, striation pattern and areolae configuration but differs from MD3 in having a narrow lanceolate axial area on the rapheless valves. Achnanthidium duriense Novais & Ector is more elliptically rounded than MD3 and A. lusitanicum Novais & M.Morais shows subrostrate, protracted apices. Additionally, MD3 shows similarities with small valves of several larger species such as A. lineare, A. minutissimum s.s., A. sieminskae and A. indistinctum.
Discussion
Achnanthidium digitatum can easily be assigned to Achnanthidium based on its small, narrow valves, V-shaped frustules, uniseriate striae, a fine central raphe and straight or curved distal raphe fissures (Round & Bukhtiyarova, Citation1996). Moreover, the simple straight distal raphe fissures clearly place it in a complex around A. minutissimum (Round & Bukhtiyarova, Citation1996; Kobayasi, Citation1997). Achnanthidium digitatum shows a rather close resemblance with most typical representatives of the A. minutissimum complex and has in the past presumably been identified as A. minutissimum. The unique morphological character combination of A. digitatum, i.e. the narrow, linear to only slightly linear-lanceolate valves, low number of areolae (usually two) per stria and a usually small asymmetrical to rounded central area with shortened striae, separates it from other established taxa in the A. minutissimum complex using SEM. In LM, a consistent identification of A. digitatum is unfeasible due to the overlap in valve morphology and the low number of distinctive discernible characters compared to other taxa.
Both A. digitatum and A. minutissimum complex lineage A (MD2) were observed sympatrically in almost all (> 80) examined epiphyton, epilithon and epipelon samples of the littoral zone of freshwater lakes and ponds from the study area, and were consequently one of the most dominant freshwater diatoms. Due to confusion with small-celled representatives of MD2, the distribution of A. minutissimum complex lineage D (MD3) in the study area remains unclear. Photomicrographs of A. digitatum valves have been published before in a revision of the freshwater diatom flora of the Canadian Arctic Archipelago (northern Ellesmere Island and Prince Patrick Island; Antoniades et al., Citation2008). It was argued by the authors that these valves presumably represent a new species, as they show clear ultrastructural differences from the type of A. minutissimum s.s. Careful comparison with our specimens revealed identical morphometrics and ultrastructural characteristics to these specimens. An additional population of A. digitatum was observed on Franz Jozef Land (Pla-Rabés et al., Citation2016). The findings of A. digitatum in other Arctic regions suggest that the species is a common component of the circumpolar Arctic diatom flora. The identity of the other two Arctic Achnanthidium taxa remains unclear: MD2 shows close affinities with A. minutissimum s.s., A. microcephalum and A. neocryptocephalum, whereas only one strain was available for MD3. More extensive molecular investigations with addition of strains unambiguously identified as A. minutissimum s.s. and related species would help to resolve whether MD2 and MD3 are conspecific with already established taxa. The phylogenetic analysis of rbcL showed that several strains from various regions (Canada, Italy, Germany and Russia) probably belong to the same lineage as MD2. This indicates that the MD2 species (group) has a wider distribution outside the Arctic region and suggests it might already have been observed or described in the past. The observation of clear morphological variations in valve outline, central area and areolae numbers within strains assigned to MD2 furthermore indicates that at least some species of the A. minutissimum complex might show distinct intraspecific phenotypic variation. It can however not be excluded that more than one species is present within the strains assigned to A. minutissimum lineage A. Although rbcL and 28S have been shown to exhibit sufficient interspecific variation to be useful as DNA barcodes in diatoms (e.g. Hamsher et al., Citation2011), no studies currently exist on the taxonomic resolution and inter- and intraspecific variation of rbcL and 28S in Achnanthidium specifically and the rather low sequence divergence of 28S suggests it might not be variable enough to distinguish between closely related species within the A. minutissimum complex. Other studies suggested V4 18S as a potential barcode marker in diatoms (Zimmermann et al., Citation2011; Luddington et al., Citation2012), but the results of this study indicate this marker is even less variable than 28S in the A. minutissimum complex. Moreover, DNA barcoding, a technique where short DNA sequences are used to identify species rather than to define species limits (Mann et al., Citation2010), by definition requires less molecular variation than the variation necessary to delimit species. Clearly, 18S and probably also 28S do not seem suitable for molecular species delimitation between closely related species in this complex, due to very low sequence divergences resulting in an absence of a clear boundary between inter- and intraspecific variation. Consequently, it is necessary to sequence more variable fast evolving genes such as cox1 that might yield additional information on the species limits within Achnanthidium.
Clearly, A. minutissimum is a widespread species complex representing many highly similar taxa that can not be reliably discriminated in LM. The observation that GenBank strains identified as A. minutissimum and A. saprophilum belonged to different lineages in the phylogenies furthermore confirms that reliable identification is difficult. Without voucher specimens or cultures linked to these sequences, it is impossible to determine their true identity. The difficulty in identification is due to the large overlap in morphological features visible in LM and variability in phenotypic expression, but at least a number of species show (slight) ultrastructural differences that can be recognized in SEM. However, as no molecular species delimitation has yet been carried out on Achnanthidium, it is not clear whether all Achnanthidium species exhibit divergent (ultrastructural) morphologies or whether some species simply cannot be morphologically separated due to a limited number of available morphological traits (Verbruggen, Citation2014). This taxonomic problem is found in other common cosmopolitan species complexes such as Hantzschia amphioxys Ehrenberg and Pinnularia borealis Ehrenberg (Souffreau et al., Citation2013; Pinseel et al., Citation2017; Pinseel et al., unpublished results). In addition, the degree of intraspecific morphological variation in Achnanthidium is little known. This makes it difficult to identify new populations to species level and adds a distinctly subjective aspect to species level taxonomy in Achnanthidium, i.e. how many morphological differences between two populations are needed to assign them to different species and what is the level of intraspecific phenotypic variation?
Whereas this study showed that small morphological differences in Achnanthidium correspond to molecular species limits, it also showed that identification of strains and certainly of individual valves to species level remains very difficult when based solely on morphological characteristics. Molecular studies on a large number of A. minutissimum populations worldwide are therefore needed to establish a reference DNA (barcode) database for species identification and to reveal the degree of intraspecific phenotypic variation. The inclusion of DNA sequences in species descriptions in the A. minutissimum complex will ease species identifications in future DNA-based ecological, biogeographic and biomonitoring studies (Mann et al., Citation2010). However this only applies when based on a more variable marker than the currently widely used V4 18S, which does not have species-level resolution in the complex. Furthermore, molecular data have the potential to greatly facilitate and speed up species discovery in Achnanthidium as they can overcome the typical problems associated with morphology-based species taxonomy. This however implies that DNA sequences should be assigned to currently established taxa and as no DNA samples are available for the vast majority of established species, this can only be achieved by secondary assignment of DNA sequences from newly obtained cultures or single-cell isolates to established type materials (e.g. Lefebvre & Hamilton, Citation2015). As the types of several Achnanthidium species have recently been reinvestigated (e.g. Van de Vijver et al., Citation2011a; Novais et al., Citation2015), most species are morphologically well-known, which should help the assignment of DNA sequences to old species names in this genus.
Finally, despite the relatively restricted taxon sampling, 12 different lineages were recovered via the various genes. Most of these have only been recovered from a single geographic region, whereas lineage A seems to be present throughout the northern hemisphere. Much additional sampling will be necessary to shed light on the exact distributions of the different taxa and to reveal whether selected taxon flocks have restricted rather than cosmopolitan distributions. Moreover, although only based on one marker, the rbcL phylogeny suggests that multiple colonization events have occurred in or out of several regions, notably North America and the Arctic, which might suggest the presence of interesting phylogeographic patterns in this species complex. Geographic signals are likely to be associated with niche differentiation between different (pseudo)cryptic species (Souffreau et al., Citation2013), which might yield important consequences for applied sciences such as biomonitoring in which A. minutissimum is an important indicator taxon. Currently, A. minutissimum s.l. is regarded as a very generalized indicator species with a seemingly broad ecological tolerance. However, Potapova & Hamilton (Citation2007) previously showed that different North American Achnanthidium morphotypes exhibit different ecological preferences that could be useful in biomonitoring.
In conclusion, in order to reveal the species diversity, evolutionary history and geographic distributions of species in the A. minutissimum complex, further research should focus on an increased taxon sampling of the molecular phylogenies and the development of suitable primer sets for fast-evolving (mitochondrial) markers. Only when the species boundaries are well understood, can geographic distributions and ecological niche differentiation of different species be fully unravelled. Hopefully, this study can serve as a first step towards species-level molecular research in this common diatom complex.
Supplemental information
The following supplementary material is accessible via the Supplementary Content tab on the article’s online page at http://dx.doi.org/10.1080/09670262.2017.1283540
Supplementary table 1. List of all samples examined in this study with their geographic position and measured ecological parameters. N.D. = not determined.
Supplementary table 2. Achnanthidium strain list with BOLD and GenBank accession numbers. Sequences that were not available in GenBank or that were not obtained are indicated with N.A.
Supplementary table 3. Distance matrices giving an overview of the p-values and number of base pair differences of rbcL, 28S, 18S and V4 18S (100 bp region and thus including some of the adjacent more conserved areas) for the datasets examined in this study. The taxon labels are ordered by lineage according to their respective phylogenies.
Supplementary table 4. Overview of the main morphological features of Achnanthidium taxa that can be confused with A. digitatum and A. minutissimum lineage A (MD2).
Supplementary fig. 1. Maximum likelihood phylogeny of the A. minutissimum complex based on rbcL and 28S sequences with indication of statistical support (ML bootstrap proportions/BI posterior probabilities). The different lineages are indicated with grey boxes. The dark grey boxes represent the Arctic lineages. The lineage numbers correspond with .
Supplementary fig. 2. Maximum likelihood phylogeny of the A. minutissimum complex based on 18S sequences with indication of statistical support (ML bootstrap proportions/BI posterior probabilities). The different lineages are indicated with grey boxes. The dark grey boxes represent the Arctic lineages. The lineage numbers correspond with . The rbcL lineages that were collapsed in the 18S phylogeny are indicated with all their corresponding lineage numbers.
Supplementary figs 3–12. Achnanthidium minutissimum complex lineage A (MD2). Scanning electron micrographs of culture material. –. SEM external view of a raphe valve (strains SPITS-M3-10, SPITS-M3-15, SPITS-M2A+B-26, SPITS13-GARmoss8 & SPITS13-GARmoss3 respectively). –. SEM external view of a rapheless valve (strains SPITS-M3-10, SPITS-M3-15, SPITS-M2A+B-26, SPITS13-GARmoss8 & SPITS13-GARmoss3 respectively). Scale bars: 5 μm.
Supplementary figs 13–22. Achnanthidium minutissimum complex lineage A (MD2). Scanning electron micrographs of culture material. –. SEM internal views of the entire raphe valve (strains SPITS-M3-10, SPITS-M2A+B-26, SPITS13-GARmoss8 & SPITS13-GARmoss3 respectively). . SEM view showing a frustule in girdle view (strain SPITS13-GARmoss8). Figs 18–21. SEM internal views of the entire raphe valve (strains SPITS-M3-10, SPITS-M3-15, SPITS13-GARmoss8 & SPITS13-GARmoss3 respectively). Fig 22. SEM view showing a frustule in girdle view (strain SPITS13-GARmoss8). Scale bars: 5 μm.
Supplementary figs 23–26. Achnanthidium minutissimum complex lineage D (MD3). Scanning electron micrographs of culture material (strain SPITS-M2A+B-12). Fig 23. SEM external view of a raphe valve. Fig 24. SEM internal view of a raphe valve. Fig 25. SEM external view of a rapheless valve. Fig 26. SEM internal view of a rapheless valve. Scale bars: 1 μm.
Supplementary_material.zip
Download Zip (83.1 MB)Acknowledgements
Dr Alex Ball and the staff of the IAC laboratory at the Natural History Museum are thanked for their help with the scanning electron microscopy. Sofie D’hondt and Keely Lefebvre are thanked for their help with the molecular analysis. Myriam de Haan and Renaat Dasseville are thanked for their help with additional SEM observations at the Botanic Garden Meise and Ghent University respectively. Dr Elie Verleyen and Dr Wim Van Nieuwenhuyze are thanked for the sample collection at Schirmacher Oasis and Marion Island. We thank two anonymous reviewers for their constructive comments that helped to improve the manuscript.
Disclosure statement
No potential conflict of interest was reported by the authors.
Additional information
Funding
Notes on contributors
Eveline Pinseel
E. Pinseel: original concept and study design, field sampling, LM and SEM microscopy, molecular work, data analysis, description of new species, preparation and editing of the manuscript. P. Vanormelingen: original concept and study design, culture work, editing the manuscript. P.B. Hamilton: study design, single cell analysis, LM and SEM microscopy of Canadian Arctic material, editing the manuscript. W. Vyverman: provided resources, editing the manuscript. B. Van de Vijver: SEM microscopy, editing the manuscript. K. Kopalová: field sampling, editing the manuscript.
Pieter Vanormelingen
E. Pinseel: original concept and study design, field sampling, LM and SEM microscopy, molecular work, data analysis, description of new species, preparation and editing of the manuscript. P. Vanormelingen: original concept and study design, culture work, editing the manuscript. P.B. Hamilton: study design, single cell analysis, LM and SEM microscopy of Canadian Arctic material, editing the manuscript. W. Vyverman: provided resources, editing the manuscript. B. Van de Vijver: SEM microscopy, editing the manuscript. K. Kopalová: field sampling, editing the manuscript.
Paul B. Hamilton
E. Pinseel: original concept and study design, field sampling, LM and SEM microscopy, molecular work, data analysis, description of new species, preparation and editing of the manuscript. P. Vanormelingen: original concept and study design, culture work, editing the manuscript. P.B. Hamilton: study design, single cell analysis, LM and SEM microscopy of Canadian Arctic material, editing the manuscript. W. Vyverman: provided resources, editing the manuscript. B. Van de Vijver: SEM microscopy, editing the manuscript. K. Kopalová: field sampling, editing the manuscript.
Wim Vyverman
E. Pinseel: original concept and study design, field sampling, LM and SEM microscopy, molecular work, data analysis, description of new species, preparation and editing of the manuscript. P. Vanormelingen: original concept and study design, culture work, editing the manuscript. P.B. Hamilton: study design, single cell analysis, LM and SEM microscopy of Canadian Arctic material, editing the manuscript. W. Vyverman: provided resources, editing the manuscript. B. Van de Vijver: SEM microscopy, editing the manuscript. K. Kopalová: field sampling, editing the manuscript.
Bart Van de Vijver
E. Pinseel: original concept and study design, field sampling, LM and SEM microscopy, molecular work, data analysis, description of new species, preparation and editing of the manuscript. P. Vanormelingen: original concept and study design, culture work, editing the manuscript. P.B. Hamilton: study design, single cell analysis, LM and SEM microscopy of Canadian Arctic material, editing the manuscript. W. Vyverman: provided resources, editing the manuscript. B. Van de Vijver: SEM microscopy, editing the manuscript. K. Kopalová: field sampling, editing the manuscript.
Kateřina Kopalova
E. Pinseel: original concept and study design, field sampling, LM and SEM microscopy, molecular work, data analysis, description of new species, preparation and editing of the manuscript. P. Vanormelingen: original concept and study design, culture work, editing the manuscript. P.B. Hamilton: study design, single cell analysis, LM and SEM microscopy of Canadian Arctic material, editing the manuscript. W. Vyverman: provided resources, editing the manuscript. B. Van de Vijver: SEM microscopy, editing the manuscript. K. Kopalová: field sampling, editing the manuscript.
References
- Amato, A., Kooistra, W.H.C.F., Ghiron, J.H.L., Mann, D.G., Pröschold, T. & Montresor, M. (2007). Reproductive isolation among sympatric cryptic species in marine diatoms. Protist, 158: 193–207.
- Antoniades, D., Douglas, M.S.V. & Smol, J.P. (2003). Comparative physical and chemical limnology of two Canadian High Arctic regions: Alert (Ellesmere Island, NU) and Mould Bay (Prince Patrick Island, NWT). Archiv für Hydrobiologie, 158: 485–516.
- Antoniades, D., Hamilton, P.B., Douglas, M.S.V. & Smol, J.P. (2008). Diatoms of North America. The freshwater floras of Prince Patrick, Ellef Ringnes and northern Ellesmere Islands from the Canadian Arctic Archipelago. Iconographia Diatomologica, 17: 1–649.
- Antoniades, D., Hamilton, P.B., Hinz, F., Douglas, M.S.V. & Smol, J.P. (2009). Seven new species of freshwater diatoms (Bacillariophyceae) from the Canadian Arctic Archipelago. Nova Hedwigia, 88: 57–80.
- Brinkmann, N., Hodač, L., Mohr, K.I., Hodačová, A., Jahn, R., Ramm, J., Hallmann, C., Arp, G. & Friedl, T. (2015). Cyanobacteria and diatoms in biofilms of two karstic streams in Germany and changes of their communities along calcite saturation gradients. Geomicrobiology Journal, 32: 255–274.
- Bruder, K. & Medlin, L. K. (2007). Molecular assessment of phylogenetic relationships in selected species/genera in the naviculoid diatoms (Bacillariophyta). I. The genus Placoneis. Nova Hedwigia, 85: 331–352.
- Burns, J.M., Janzen, D.H., Hajibabaei, M., Hallwachs, W. & Hebert, P.D.N. (2008). DNA barcodes and cryptic species of skipper butterflies in the genus Perichares in Area de Conservación Guanacaste, Costa Rica. Proceedings of the National Academy of Sciences of the United States of America, 105: 6350–6355.
- Cantonati, M. & Lange-Bertalot, H. (2006). Achnanthidium dolomiticum sp. nov. (Bacillariophyta) from oligotrophic mountain springs and lakes fed by dolomite aquifers. Journal of Phycology, 42: 1184–1188.
- Compère, P. & Van de Vijver, B. (2011). Achnanthidium ennediense (Compère) Compère et Van de Vijver comb. nov. (Bacillariophyceae), the true identity of Navicula ennediensis Compère from the Ennedi mountains (Republic of Chad). Algological Studies, 136/137: 5–17.
- Darriba, D., Taboada, G.L., Doalla, R. & Posada, D. (2012). jModelTest2: more models, new heuristics and parallel computing. Nature Methods, 9: 772.
- Daugbjerg, N. & Anderson, R.A. (1997). Phylogenetic analyses of the rbcL sequences from Haptophytes and heterokont algae suggest their chloroplasts are unrelated. Molecular Biology and Evolution, 14: 1242–1251.
- De Clerck, O., Gavio, B., Fredericq, S., Bárbara, I. & Coppejans E. (2005). Systematics of Grateloupia filicina (Halymeniaceae, Rhodophyta) based on rbcL sequence analysis and morphological evidence including the reinstatement of G. minima and the description of G. capensis sp. nov. Journal of Phycology, 41: 391–410.
- De Queiroz, K. (2007). Species concepts and species delimitation. Systematic Biology, 56: 879–886.
- Douglas, M.S.V. & Smol, J.P. (2010). Freshwater diatoms as indicators of environmental change in the High Arctic. In The Diatoms: Applications for the Environmental and Earth Sciences (Smol, J.P. & Stoermer, E.F., editors). Cambridge University Press, New York.
- Environment Canada (1994). Manual of Analytical Methods. The National Laboratory for Environmental Testing, Canadian Centre for Inland Waters, Burlington, Ontario.
- Evans, K.M., Wortley, A.H. & Mann, D.G. (2007). An assessment of potential diatom “barcode” genes (cox1, rbcL, 18S and ITS rDNA) and their effectiveness in determining relationships in Sellaphora (Bacillariophyta). Protist, 158: 349–364.
- Evans, K.M., Wortley, A.H., Simpson, G.E., Chepurnov, V.A. & Mann, D.G. (2008). A molecular systematic approach to explore diversity within the Sellaphora pupula species complex (Bacillariophyta). Journal of Phycology, 44: 215–231.
- Foged, N. (1964). Freshwater diatoms from Spitsbergen. Tromsö Museums Skrifter, 11: 1–204.
- Foged, N. (1981). Diatoms in Alaska. Bibliotheca Phycologica, 53: 1–317.
- Guillard, R.R.L. & Lorenzen, C.L. (1972). Yellow-green algae with chlorophyllide c. Journal of Phycology, 8: 10–14.
- Hall, T.A. (1999). BioEdit: a user-friendly biological sequence alignment editor and analysis program for Windows 95/98/NT. Nucleic Acids Symposium Series, 41: 95–98.
- Hamilton, P.B., Lefebvre, K.E. & Bull, R.D. (2015). Single cell PCR amplification of diatoms using fresh and preserved samples. Frontiers in Microbiology, 6: 1084.
- Hamsher, S.E., Evans, K.M., Mann, D.G., Poulickova, A. & Saunders, G.W. (2011). Barcoding diatoms: exploring alternatives to COI-5P. Protist, 162: 405–422.
- Hasegawa, M., Kishino, H. & Yano, T. (1985). Dating of human–ape splitting by a molecular clock of mitochondrial DNA. Journal of Molecular Evolution, 22: 160–174.
- Hlúbiková, D., Ector, L. & Hoffmann, L. (2011). Examination of the type material of some diatom species related to Achnanthidium minutissimum (Kütz.) Czarn. (Bacillariophyceae). Algological Studies, 136/137: 19–43.
- Huelsenbeck, J.P. & Ronquist, F. (2001). MrBayes: Bayesian inference of phylogenetic trees. Bioinformatics, 17: 754–755.
- Huss, V.A.R., Frank, C., Hartmann, E.C., Hirmer, M., Kloboucek, A., Seidel, B.M., Wenzeler, P. & Kessler, E. (1999). Biochemical taxonomy and molecular phylogeny of the genus Chlorella sensu lato (Chlorophyta). Journal of Phycology, 35: 587–598.
- Jones, H.M., Simpson, G.E., Stickle, A.J. & Mann, D.G. (2005). Life history and systematics of Petroneis (Bacillariophyta), with special reference to British waters. European Journal of Phycology, 40: 61–87.
- Jüttner, I. & Cox, E.J. (2011). Achnanthidium pseudoconspicuum comb. nov.: morphology and ecology of the species and a comparison with related taxa. Diatom Research, 26: 21–28.
- Jüttner, I., Chimonides, J. & Cox, E.J. (2011). Morphology, ecology and biogeography of diatom species related to Achnanthidium pyrenaicum (Hustedt) Kobayasi (Bacillariophyceae) in streams of the Indian and Nepalese Himalaya. Algological Studies, 136/137: 45–76.
- Keck, F., Rimet, F., Franc, A. & Bouchez, A. (2016). Phylogenetic signal in diatom ecology: perspectives for aquatic ecosystems biomonitoring. Ecological Applications, 26: 861–872.
- Kobayasi, H. (1997). Comparative studies among four linear-lanceolate Achnanthidium species (Bacillariophyceae) with curved terminal raphe endings. Nova Hedwigia, 65: 147–163.
- Kobayasi, H., Nagumo, T. & Mayama, S. (1986). Observations on the two rheophilic species of the genus Achnanthes (Bacillariophyceae), A. convergens H. Kob. and A. japonica H. Kob. Diatom, 2: 83–93.
- Krammer, K. & Lange-Bertalot, H. (1991). Bacillariophyceae 4. Teil Achnanthaceae. Kritischen Ergänzungen zu Navicula (Lineolatae) und Gomphonema. Süßwasserflora von Mitteleuropa, 4:1–437.
- Kulikovskiy, M., Lange-Bertalot, H., Witkowski, A. & Khursevich, G. (2011). Achnanthidium sibiricum (Bacillariophyceae) a new species from bottom sediments in Lake Baikal. Algological Studies, 136/137: 77–82.
- Kulikovskiy, M., Andreeva, S.A., Gusev, E.S., Kuznetsova, I.V. & Annenkova, N.V. (2016). Molecular phylogeny of Monoraphid diatoms and raphe significance in evolution and taxonomy. Biological Bulletin, 43: 398–407.
- Larras, F., Keck, F., Montuelle, B., Rimeet, F. & Bouchez, A. (2014). Linking diatom sensitivity to herbicides to phylogeny: a step forward for biomonitoring? Environmental Science and Technology, 48: 1921–1930.
- Lefebvre, K. & Hamilton P.B. (2015). Morphology and molecular studies on large Neidium species (Bacillariophyta) of North America, including an examination of Ehrenberg’s types. Phytotaxa, 220: 201–223.
- Leliaert, F., Verbruggen, H., Vanormelingen, P., Steen, F., López-Bautista, J.M., Zuccarella, G.C. & De Clerck, O. (2014). DNA-based species delimitation in algae. European Journal of Phycology, 49: 179–196.
- Liu, B., Blanco, S., Long, H., Xu, J. & Jiang, X. (2016). Achnanthidium sinense sp. nov. (Bacillariophyta) from the Wuling Mountains Area, China. Phytotaxa, 284: 194–202.
- Luddington, I.A., Kaczmarska, I. & Lovejoy, C. (2012). Distance and character-based evaluation of the V4 region of the 18S rRNA gene for the identification of diatoms (Bacillariophyceae). PLoS ONE, 7: e45664.
- Mann, D.G. (1999). The species concept in diatoms. Phycologia, 38: 437–495.
- Mann, D.G., Sato, S., Trobajo, R., Vanormelingen, P. & Souffreau, C. (2010). DNA barcoding for species identification and discovery in diatoms. Cryptogamie, Algologie, 31: 557–577.
- Miller, M.A., Pfeiffer, W. & Schwartz, T. (2010). Creating the CIPRES Science Gateway for inference of large phylogenetic trees, Proceedings of the Gateway Computing Environments Workshop (GCE), New Orleans, LA: 1–8.
- Moniz, M.B.J., Rindi, F., Novis, P.M., Broady, P.A. & Guiry, M.D. (2012). Molecular phylogeny of Antarctic Prasiola (Prasiolales, Trebouxiophyceae) reveals extensive cryptic diversity. Journal of Phycology, 48: 940–955.
- Moon-van Der Staay, S.Y., van der Staay, G.W.M., Guillou, L. & Vaulot, D. (2000). Abundance and diversity of prymnesiophytes in the picoplankton community from the equatorial Pacific Ocean inferred from 18S rDNA sequences. Limnology and Oceanography, 45: 98–109.
- Morales, E.A., Ector, L., Fernández, E., Novais, M.H., Hlúbiková, D., Hamilton, P.B., Blanco, S., Vis, M.L. & Kociolek, P. (2011). The genus Achnanthidium Kütz. (Achnanthales, Bacillariophyaceae) in Bolivian streams: a report of taxa found in recent investigations. Algological Studies, 136/137: 89–130.
- Nakov, T. (2014). Studies of phylogenetic relationships and evolution of functional traits in diatoms. PhD thesis, The University of Texas at Austin.
- Novais, M. H., Hlúbiková, D., Morais, M., Hofmann, L. & Ector L. (2011). Morphology and ecology of Achnanthidium caravelense (Bacillariophyceae), a new species from Portuguese rivers. Algological Studies, 136/137: 131–150.
- Novais, M. H., Jüttner, I., Van de Vijver, B., Morais, M.M., Hoffmann, L. & Ector L. (2015). Morphological variability within the Achnanthidium minutissimum species complex (Bacillariophyta): comparison between the type material of Achnanthes minutissima and related taxa, and new freshwater Achnanthidium species from Portugal. Phytotaxa, 224: 101–139.
- Pinseel, E., Kopalová K. & Van de Vijver, B. (2014). Gomphonema svalbardense sp. nov., a new freshwater diatom species (Bacillariophyceae) from the Arctic Region. Phytotaxa, 170: 250–258.
- Pinseel, E., Van de Vijver, B. & Kopalová, K. (2015). Achnanthidium petuniabuktianum sp. nov. (Achnan-thidiaceae, Bacillariophyta), a new representative of the A. pyrenaicum group from Spitsbergen (Svalbard Archipelago, High Arctic). Phytotaxa, 226: 63–74.
- Pinseel, E., Van de Vijver, B., Kavan, J., Verleyen, E. & Kopalová K. (2016). Diversity, ecology and community structure of the freshwater littoral diatom flora from Petuniabukta (Spitsbergen). Polar Biology, doi: 10.1007/s00300-016-1976-0.
- Pinseel, E., Hejduková, E., Vanormelingen, P., Kopalová, K., Vyverman, W. & Van de Vijver, B. (2017). Pinnularia catenaborealis sp. nov. (Bacillariophyceae), a unique chain-forming diatom species from James Ross Island and Vega Island (Maritime Antarctica). Phycologia, 56: 94–107.
- Pla-Rabés, S., Hamilton, P.B., Ballesteros, E., Gavrilo, M., Friedlander, A.M. & Sala, E. (2016). The diatom structure and diversity of freshwater diatom assemblages from Franz Josef Land Archipelago: a northern outpost for freshwater diatoms. PeerJ, 4:e1705. doi: 10.7717/peerj.1705
- Ponader, K.C. & Potapova M.G. (2007). Diatoms from the genus Achnanthidium in flowing waters of the Appalachian Mountains (North America): ecology, distribution and taxonomic notes. Limnologica, 37: 227–241.
- Posada, D. (2008). jModelTest: phylogenetic model averaging. Molecular Biology and Evolution, 25: 1253–1256.
- Potapova, M. (2006). Achnanthidium zhakovschikovii sp. nov. (Bacillariophyta) and related species from rivers of Northwestern Russia. Nova Hedwigia, 82: 3–4.
- Potapova, M. & Hamilton, P.B. (2007). Morphological and ecological variation within the Achnanthidium minutissimum (Bacillariophyceae) species complex. Journal of Phycology, 43: 561–575.
- Potapova, M.G. & Ponader, K.C. (2004). Two common North American diatoms: Achnanthidium rivulare sp. nov. and A. deflexum (Reimer) Kingston: morphology, ecology and comparison with related species. Diatom Research, 19: 33–57.
- Quijano-Scheggia, S.I., Gracés, E., Lundholm, N., Moestrup, Ø., Andree, K. & Camp, J. (2009). Morphology, physiology, molecular phylogeny and sexual compatibility of the cryptic Pseudo-nitzschia delicatissima complex (Bacillariophyta), including the description of P. arenysensis sp. nov. Phycologia, 48: 492–509.
- Quillou, L., Chrétiennot-Dinet, M.-J., Medlin, L.K., Claustre, H., Loiseaux-de Goër, S. & Vaulot, D. (1999). Bolidomonas: a new genus with two species belonging to a new algal class, the Bolidophyceae. Journal of Phycology, 35: 368–381.
- Rambaut, A., Suchard, M.A., Xie, D. & Drummond, A.J. (2014). Tracer v1.6, available at: http://beast.bio.ed.ac.uk/Tracer.
- Ratnasingham, S. & Hebert, P.D.N. (2007). BOLD: The Barcode of Life Data System (www.barcodinglife.org). Molecular Ecology Notes, 7: 355–364.
- Reichardt, E. (2004). Eine bemerkenswerte Diatomee-nassoziation in einem Quellhabitat im Grazer Bergland, Österreich. Iconographia Diatomologica, 13: 419–479.
- Rimet, F., Kermarrec, L., Bouchez, A., Hoffmann, L., Ector, L & Medlin, K. (2011). Molecular phylogeny of the family Bacillariaceae based on 18S rDNA sequences: focus on freshwater Nitzschia of the section Lanceolatae. Diatom Research, 26: 273–291.
- Ronquist, F. & Huelsenbeck, J.P. (2003). MrBayes 3: Bayesian phylogenetic inference under mixed models. Bioinformatics, 19: 1572–1574.
- Round, F.E. & Bukhtiyarova, L. (1996). Four new genera based on Achnanthes (Achnanthidium) together with a re-definition of Achnanthidium. Diatom Research, 11: 345–361.
- Round, F.E., Crawford, R.M. & Mann, D.G. (1990). The Diatoms: biology and morphology of the genera. Cambridge University Press, Cambridge.
- Scholin, C.A., Herzog, M., Sogin, M. & Anderson, D.M. (1994). Identification of group–specific and strain-specific genetic-markers for globally distributed Alexandrium (Dinophyceae). 2. Sequence analysis of a fragment of the LSU ribosomal RNA gene. Journal of Phycology, 30: 999–1011.
- Silvestro, D. & Michalak, I. (2012). raxmlGUI: a graphical front-end for RAxML. Organisms Diversity and Evolution, 12: 335–337.
- Souffreau, C., Vanormelingen P., Van de Vijver, B., Isheva, T., Verleyen, E., Sabbe, K. & Vyverman, W. (2013). Molecular evidence for distinct Antarctic lineages in the cosmopolitan terrestrial diatoms Pinnularia borealis and Hantzschia amphioxys. Protist, 164: 101–115.
- Tamura, K., Stecher, G., Peterson, D., Filipski, A. & Kumar, S. (2013). MEGA6: Molecular Evolutionary Genetics Analysis Version 6.0. Molecular Biology and Evolution, 30: 2725–2729.
- Tavare, S. (1986). Some probabilistic and statistical problems on the analysis of DNA sequences. American Mathematical Society: Lectures on Mathematics in the Life Sciences, 17: 57–86.
- Taylor, J.C., Morales, E.A. & Ector, L. (2011). Achnanthidium standeri (Cholnoky) comb. nov. and Achnanthidium taiaense (J. R. Carter et Denny) comb. nov., two new combinations of morphologically similar Achnanthidium species from Africa. Algological Studies, 136/137: 151–166.
- Thompson, J.D., Higgins, D.G. & Gibson, T.J. (1994). Clustal-W – improving the sensitivity of progressive multiple sequence alignment through sequence weighting, position-specific gap penalties and weight matrix choice. Nucleic Acids Research, 22: 4673–4680.
- Trobajo, R., Clavero, E., Chepurnov, V.A., Sabbe, K., Mann, D.G., Ishihara, S. & Cox, E.J. (2009). Morphological, genetic, and mating diversity within the widespread bioindicator Nitzschia palea (Bacillariophyceae). Phycologia, 48: 443–459.
- Vanelslander, B., Créach, V., Vanormelingen, P., Ernst, A., Chepurnov, V.A., Sahan, E., Muyzer, G., Stal, L.J., Vyverman, W. & Sabbe, K. (2009). Ecological differentiation between sympatric pseudocryptic species in the estuarine benthic diatom Navicula phyllepta (Bacillariophyceae). Journal of Phycology, 45: 1278–1289.
- Van de Vijver, B. & Kopalová, K. (2014). Four new Achnanthidium species (Bacillariophyta) formerly identified as Achnanthidium minutissimum from the Antarctic Region. European Journal of Taxonomy, 79: 1–19.
- Van de Vijver, B., Beyens, L. & Lange–Bertalot, H. (2004). The genus Stauroneis in the Arctic and (Sub-)Antarctic Regions. Bibliotheca Diatomologica, 51: 1–317.
- Van de Vijver, B., Ector, L., Beltrami, M. E., de Haan, M., Falasco, E., Hlúbiková, D., Jarlman, A., Kelly, M., Novais, M. H. & Wojtal, A. Z. (2011a). A critical analysis of the type material of Achnanthidium lineare W. Sm. (Bacillariophyceae). Algological Studies, 136/137: 167–191.
- Van de Vijver, B., Jarlman, A., Lange-Bertalot, H., Mertens, A., de Haan, M. & Ector, L. (2011b). Four new European Achnanthidium species (Bacillariophyceae). Algological Studies, 136/137: 193–210.
- Van der Werff, A. (1955). A new method for cleaning and concentrating diatoms and other organisms. Verhandlungen der Internationalen Vereinigung für Theoretische und Angewandte Limnologie, 12: 276–277.
- van Hannen, E.J., Mooij, W., van Agterveld, M.P., Gons, H.J. & Laanbroek, H.J. (1998). Detritus-dependent development of the microbial community in an experimental system: qualitative analysis by DGGE. Applied and Environmental Microbiology, 65: 2478–2484.
- Van Nieuwenhuyze, W. (2015). Reconstruction of Holocene paleoenvironmental changes in the sub-Antarctic region. PhD thesis, Ghent University.
- Vanormelingen, P., Evans, K.M., Chepurnov, V., Vyverman, W. & Mann, D.G. (2013). Molecular species discovery in the diatom Sellaphora and its congruence with mating trials. Fottea, 13: 133–148.
- Verbruggen, H. (2014). Morphological complexity, plasticity, and species diagnosability in the application of old species names in DNA-based taxonomies. Journal of Phycology, 50: 26–31.
- Verleyen, E., Hodgson, D.A., Gibson, J., Imura, S., Kaup, E., Kudoh, S., De Wever, A., Hoshino, T., McMinn, A., Obbels, D., Roberts, D., Roberts, S., Sabbe, K., Souffreau, C., Tavernier, I., Van Nieuwenhuyze, W., Van Ranst, E., Vindevogel, N. & Vyverman, W. (2012). Chemical limnology in coastal East Antarctic lakes: monitoring future climate change in centres of endemism and biodiversity. Antarctic Science, 24: 23–33.
- Witkowski, A., Kulikovskiy, M. & Riaux-Gobin, C. (2012). Achnanthidium sieminskae, a new diatom species from Kerguelen Archipelago (Austral Islands). In Current Advances in Algal Taxonomy and its Applications (Wołowski, K., Kaczmarska, I., Ehrman, J.M. & Wojtal, A.Z., editors): 61–68. Kraków: W. Szafer Institute of Botany.
- Wojtal, A. Z., Ector, L., Van de Vijver, B., Morales, E. A., Blanco, S., Piatek, J. & Smieja, A. (2011). The Achnanthidium minutissimum complex (Bacillariophyceae) in southern Poland. Algological Studies, 136/137: 211–238.
- Zgrundo, A., Wojtasik, B., Convey, P. & Majewska, R. (2016). Diatom communities in the High Arctic aquatic habitats of northern Spitsbergen (Svalbard). Polar Biology, doi: 10.1007/s00300-016-2014-y.
- Zimmermann, J., Jahn, R. & Gemeinholzer, B. (2011). Barcoding diatoms: evaluation of the V4 subregion of the 18S rRNA gene, including new primers and protocols. Organisms Diversity and Evolution, 11: 173.
- Zimmermann, J., Abarca, N., Enk, N., Skibbe, O., Kusber, W.-H. & Jahn, R. (2014). Taxonomic reference libraries for environmental barcoding: a best practice example from diatom research. PLoS ONE, 9: e108793.
- Zwart, G., Huismans, R., van Agterveld, M.P., Van de Peer, Y., De Rijk, P., Eenhoorn, H., Muyzer, G., van Hannen, E.J., Gons, H.J. & Laanbroek, H.J. (1998). Divergent members of the bacterial division Verrucomicrobiales in a temperate freshwater lake. FEMS Microbiology Ecology, 25: 159–169.