ABSTRACT
Brassinosteroids are part of the hormonal network that regulates growth processes and stress responses in plants. There is evidence for a similar hormonal network in microalgae. In the present study, six microalgae (Chlorococcum ellipsoideum, Gyoerffyana humicola, Nautococcus mamillatus, Acutodesmus acuminatus, Protococcus viridis and Chlorella vulgaris) were subjected to salt and low temperature stress with the addition of 36 g l–1 NaCl and transfer from 25°C to 15°C. There was a rapid response to salt stress with the brassinosteroid content (mainly castasterone with lower amounts of brassinolide, homocastasterone and typhasterol) increasing within 30 min of the salt treatment and remaining at these elevated levels after 7 h. The decrease in temperature had little effect on the brassinosteroid content. This was the first study to show that endogenous brassinosteroids increase in response to abiotic stress in a number of microalgae species.
Introduction
Brassinosteroids are polyhydroxylated steroid hormones with a ubiquitous distribution in the Plant Kingdom, occurring in angiosperms, gymnosperms (Bajguz & Tretyn, Citation2003) and non-seed plants, e.g. in the strobilus of Equisetum arvense (pteridophyte; Takatsuto et al., Citation1990) and Marchantia polymorpha (bryophyte; Park et al., Citation1999). They are also present in seaweed, e.g. Ecklonia maxima (Phaeophyceae; Stirk et al., Citation2014), freshwater Chlorophyceae, e.g. Hydrodictyon reticulatum (Hydrodictyaceae; Yokota et al., Citation1987) and microalgae (Bajguz, Citation2009a; Stirk et al., Citation2013a).
Brassinosteroids consist of a 5α-cholestane skeleton with structural variations in the type and position of the A- and B- rings and side chain (Oklestková et al., Citation2015). Variations include the number of hydroxyl (OH) groups on the A-ring, the oxidation stage of the B-ring (7-oxalactone, 6-ketone and non B-ring oxidized types) and substituents at mainly C-22, C-23 and C-24 on the side chain (Bajguz & Tretyn, Citation2003). There are two interconnected biosynthetic pathways for C28 brassinosteroids, namely the early C-6 oxidation and late C-6 oxidation pathways, with campersterol the precursor for both pathways (Fujioka & Yokota, Citation2003). C29 brassinosteroids follow a different biosynthetic pathway beginning with the precursor sitosterol and C27 brassinosteroids are synthesized from the precursor cholesterol (Fujioka & Yokota, Citation2003; Oklestková et al., Citation2015). While over 70 brassinosteroid metabolites have been identified to date (Bajguz & Tretyn, Citation2003; Bajguz, Citation2009a), only a few are biologically active. Brassinolide (BL) and its direct biosynthetic precursor castasterone (CS) and 24-epibrassinolide show the highest biological activity (Bartwell et al., Citation2013). Levels of active brassinosteroids are regulated by their modification mainly via hydroxylation, oxidation and epimerization reactions, and conjugation with either glucose and/or fatty acids (Fujioka & Yokota, Citation2003).
Brassinosteroids regulate many physiological and developmental processes in plants. They promote cell division, expansion and differentiation at the cellular level and influence root and shoot growth, reproductive processes and seed germination at the whole plant level (Fariduddin et al., Citation2014; Oklestková et al., Citation2015; Rajewska et al., Citation2016). There is evidence that brassinosteroids have a similar regulatory role in microalgae. Exogenous application of two brassinosteroids i.e. BL and CS stimulated cell division, increased net photosynthetic rate and chlorophyll content and increased monosaccharide and organic and inorganic phosphorus content in Chlorella vulgaris (Bajguz & Czerpak, Citation1998). Brassinazole, an inhibitor of brassinosteroid biosynthesis, suppressed the growth of C. vulgaris with a decrease in RNA synthesis, and protein, carotenoid and sugar content. This inhibitory effect was partially reversed with the co-application of BL (Bajguz & Asami, Citation2004).
Brassinosteroids also play an important role in alleviating abiotic stresses in plants though interactions with the hormonal network and by stimulating the antioxidant system, modifying both antioxidant enzymes and non-enzymatic antioxidants in plants (Fariduddin et al., Citation2014; Oklestková et al., Citation2015, Rajewska et al., Citation2016). For example, brassinosteroids increased antioxidant enzyme activity and carotenoid content in drought-stressed maize seedlings (Fariduddin et al., Citation2014); brassinosteroids applied to salinity-stressed rice, Arabidopsis thaliana, Brassica napus, Vigna radiata and Cicer arietinum modified the accumulation of proline and the activity of various antioxidant enzymes and altered gene expression (reviewed in Fariduddin et al., Citation2014) and brassinosteroids improved the tolerance and recovery of tomato seedlings to high temperatures by increasing the accumulation of heat-shock proteins as well as providing protection from cold stress (Fariduddin et al., Citation2014). Similar responses occured in Chlorella vulgaris where exogenous application of BL partially overcame the inhibitory effects of heavy metal stress on growth, this was accompanied by an increase in both enzymatic (superoxide dismutase, catalase, ascorbate peroxidase and glutathione reductase) and non-enzymatic (ascorbate and glutathione) systems in a concentration-dependent manner (Bajguz, Citation2010).
There is interest in using cell culture techniques for the in vivo production of bioactive plant metabolites as it allows for a continuous and reliable source of natural products (Bartwell et al., Citation2013). Brassinosteroids have promising therapeutic properties such as antiviral (herpes, measles, polio), antimicrobial, anti-angiogenic, anti-proliferative (anti-cancer) and neuroprotective (antioxidant) activities (reviewed in Bajguz et al., Citation2013; Oklestková et al., Citation2015) combined with a favourable safety profile with low toxicity (Bajguz et al., Citation2013). Due to similarities between brassinosteroids and animal steroids, with both being cholesterol derivatives, brassinosteroids have potential to provide novel leads for drug development. Understanding the production of brassinosteroids in microalgae in response to induced stress can enhance the value of the biomass.
The aim of the present study was to quantify changes in the endogenous brassinosteroid content in microalgae exposed to salt and cold temperature stress.
Materials and methods
Microalgae cultures, growth conditions and experimental design
Six axenic microalgae strains from different taxonomic groups were selected from the Mosonmagyaróvár Algal Culture Collection (MACC) for the study. Details of the strains are shown in . Each strain was inoculated from agar cultures into two flasks containing 250 ml liquid Bristol nutrient medium (Bold, Citation1949). The cultures were grown at 25 ± 2°C in a 14:10 h light:dark photoperiod and illuminated from below with 130 μmol photons m–2 s–1 and aerated with 20 l h–1 1.5% CO2-enriched sterile air during the light phase.
Table 1. Taxonomic details and culture origin of the six microalgae strains used in the study.
After 7 days, the suspension cultures from both flasks were combined and used to inoculate 24 flasks each containing 250 ml Bristol medium. The starting biomass was 10 mg l–1. These cultures were grown in the above-mentioned culture conditions for a further 4 days.
At the beginning of the light period (7.00 am) on day 4, the flasks were divided into four groups (6 flasks per treatment) and treated as follows:
30 ml distilled water added and flasks incubated at 25°C;
30 ml sterile NaCl solution added to give a concentration of 36 g l–1 NaCl and flasks incubated at 25°C;
30 ml distilled water added and flasks incubated at 15°C;
30 ml sterile NaCl solution added to give a concentration of 36 g l–1 NaCl and flasks incubated at 15°C.
After 30 min and again at 7 h (2.00 pm), 10 ml samples were collected from the flasks for each treatment. A portion from each sample (2 ml) was stored deep-frozen for brassinosteroid quantification and another 2 ml were preserved with Lugol’s solution for cell number counting. The remaining sample (5 ml) was used for dry weight determination.
Cell number
Cell number was counted using an Olympus BX60 microscope (Olympus Optical Co. Ltd, Tokyo, Japan) and analysed with analySYS software (Software Imaging GmbH, Münster, Germany). There were six replicates per treatment. One-way ANOVA followed by post hoc Tukey’s test were used to detect significant differences (P > 0.05) between the treatments (SigmaPlot v.13).
Dry weight determination
Samples were filtered through Whatman GF/C glass fibre filters (5 cm diameter) that had previously been dried at 105°C for 2 h, cooled in a desiccator and weighed. Following filtration, the algae-loaded filters were again dried, cooled and weighed. The biomass of the suspension was calculated as mg DW l–1. Owing to the high salt content in the NaCl-treated cultures, their DW was adjusted according to a factor determined by filtering a similar volume of 36 g l–1 NaCl solution. These measurements were used to determine the brassinosteroid content in the samples.
Brassinosteroid quantification
The brassinosteroid content in the samples was analysed using the previously described method (Tarkowská et al., Citation2016) with a few minor modifications. Briefly, 0.4–2.4 mg DW samples, each combined with 10 pmol of internal standard mixture comprising [2H3]brassinolide, [2H3]castasterone, [2H3]24-epibrassinolide, [2H3]24-epicatasterone, [2H3]28-norbrassinolide, [2H3]28-norcastasterone and [2H3]typhasterol (OlChemim, Olomouc, Czech Republic), were extracted in ice-cold 60% acetonitrile overnight at 4°C with stirring. The crude extracts were subsequently centrifuged and the obtained supernatants were further purified on polyamine SPE columns (Supelco, Bellefonte, Pennsylvania, USA) and finally analysed by UHPLC-MS/MS (Waters, Milford, USA; Waters MS Technologies, Manchester, UK). Data analysis was carried out using MasslynxTM 4.1 software (Waters, Manchester, UK) and brassinosteroids were quantified by the standard isotope-dilution method using six technical replicates per one biological sample.
Results
Over the 7 h experimental period, three species i.e. Chlorococcum ellipsoideum (), Acutodesmus acuminatus () and Protococcus viridis () continued to divide as indicated by a significant increase in cell number from 30 min to 7 h in the 25°C and 15°C treatments. Addition of NaCl had a negative effect on cell division with no significant changes in cell number over the 7 h period in the salt-stressed cultures. The other three species i.e. Gyoerffyana humicola (), Nautococcus mamillatus () and Chlorella vulgaris () did not undergo cell division over the 7 h experimental period with no significant change in cell number in the 25°C and 15°C treatments. Addition of NaCl stimulated cell division in G. humicola at 25°C () and N. mamillatus at 15°C ().
Fig. 1. Change in cell number in six microalgae exposed to high salt and cold temperature stress. Results are presented as mean ± SE (n = 6). Different letters indicate significant differences (P > 0.05).
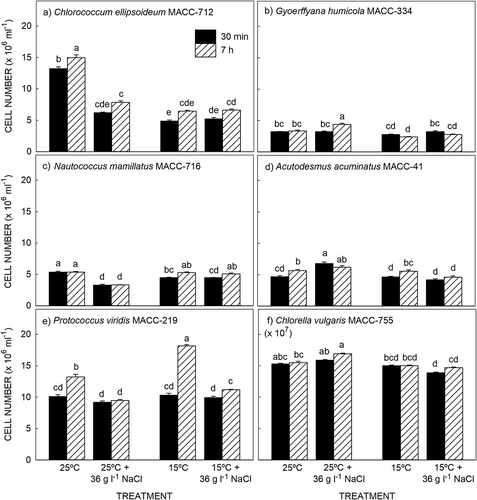
Four endogenous brassinosteroids – BL, CS, homocastasterone (homoCS) and typhasterol (TY) were detected in the six microalgae. In all species, regardless of the treatment, CS was present in the highest concentration. In N. mamillatus, A. acuminatus, P. viridis and C. vulgaris the other three brassinosteroids were present in similar, lower amounts. In G. humicola homoCS occurred in moderate amounts with BL and TY present in the lowest concentrations, while in C. ellipsoideum BL and TY occurred in similar amounts with homoCS present in the lowest concentration (Supplementary table).
The total brassinosteroid content ranged from 52 to 670 pg mg–1 DW, being lowest in P. viridis () and highest in G. humicola (). Time of day and sample treatment had an effect on the brassinosteroid content. With the exception of N. mammillatus () and salt-stressed C. ellipsoideum at 25°C (), the endogenous brassinosteroid content decreased over the course of the light period regardless of the treatment ().
Fig. 2. Endogenous brassinosteroid content in six microalgae exposed to high salt and low temperature stress. Results are presented as mean ± SE (n = 6).
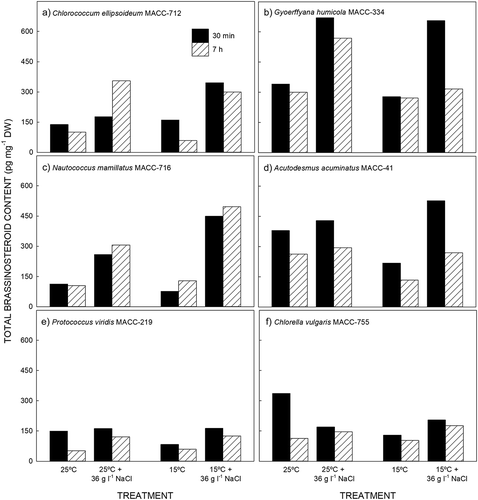
The effect of salinity stress was pronounced, with generally higher brassinosteroid content in samples treated with 36 g l–1 NaCl compared with the corresponding untreated cultures. The response to salinity stress was rapid, with samples harvested after 30 min exposure to high salinity generally having an increased brassinosteroid content compared with the corresponding untreated cultures (). In G. humicola and A. acuminatus, the content of brassinosteroids increased in the short term (30 min harvest) and then decreased over time (7 h harvest; , ). Conversely, the brassinosteroid content increased more slowly in C. ellipsoideum in response to salinity stress when cultured at 25°C (). In the other strains, the brassinosteroid content initially increased and remained at these elevated levels after 7 h.
In four strains, temperature had no effect on the brassinosteroid content while in A. acuminatus () and C. vulgaris (), brassinosteroid levels decreased when transferred from 25 to 15°C. There was a salt-and-temperature accumulative effect in G. humicola where the brassinosteroid level was lower in the salt-stressed culture at 15°C () and higher in N. mamillatus ().
Discussion
CS is the most widely distributed brassinosteroid in the plant kingdom, followed by BL with TY, 6-deoxocastasterone (6-deoxoCS), teasterone (TE) and 28-norcastasterone (28-norCS) also being commonly identified (Bajguz & Tretyn, Citation2003). The brassinosteroid profiles of the six microalgae analysed in the present study consisted of these common brassinosteroids with CS, BL, TY and homoCS being detected in all strains (Supplementary table). This is in agreement with previous studies where CS and BL were identified in 24 microalgae strains from diverse taxonomic divisions Chlorophyceae, Trebouxiophyceae, Ulvophyceae (all Chlorophyta) and one strain from the Streptophyta clade (Stirk et al., Citation2013a); seven brassinosteroids, including CS, BL and TY as well as TE, 6-deoxoteasterone (6-deoxoTE), 6-deoxotyphasterol (6-deoxoTY) and 6-deoxoCS were isolated from Chlorella vulgaris (Bajguz, Citation2009a); two brassinosteroids with a C-24 configuration were present in the freshwater green alga Hydrodictyon reticulatum (Hydrodictyaceae; Yokota et al., Citation1987); and CS and BL were isolated from the stipes and fronds of the kelp Ecklonia maxima (Stirk et al., Citation2014).
In agreement with previous studies on non-vascular plants, the brassinosteroids identified in the six microalgae strains analysed in the present study are the last intermediates in the early C-6 oxidation pathway where TY is converted via CS to BL (Fujioka & Yokota, Citation2003). Feeding experiments where TE is converted via 3-dehydroteasterone to TY suggest that brassinosteroids are formed via the early C-6 oxidation pathway in the liverwort Marchantia polymorpha (Park et al., Citation1999). This limited evidence suggests that the early C-6 biosynthetic pathway is the favoured pathway in non-vascular species although there is also evidence of the late C-6 oxidation pathway in C. vulgaris (Bajguz, Citation2009a). In contrast, the late C-6 oxidation pathway is the predominate route in angiosperms such as Arabidopsis, tomato and tobacco (Fujioka & Yokota, Citation2003).
The brassinosteroid concentration ranges in the six strains analysed in the present study were similar to those recorded in the 24 microalgae strains previously analysed (118–978 pg mg–1 DW; Stirk et al., Citation2013a) but higher than reported in C. vulgaris (1.91 ng g–1 DW; Bajguz, Citation2009a) and the kelp Ecklonia maxima (14–29 pg mg–1 DW; Stirk et al., Citation2014). The higher brassinosteroid content measured in the present study and the previously analysed 24 microalgae strains may be due to the cultures being harvested on day 4 while in an exponential growth phase. Brassinosteroids generally occur in higher concentrations in young, actively growing plant tissues compared with older tissues (Bajguz & Tretyn, Citation2003) as they are essential for cell elongation, influencing the mechanical properties of the cell wall during cell elongation (Bartwell et al., Citation2013).
Of the four brassinosteroids detected in the six microalgae, CS, homoCS and TY have a 6-ketone B-ring and BL has a 7-oxolactone B-ring (Bagjuz & Tretyn, Citation2003). In general, the 7-oxalactone brassinosteroids have the strongest biological activity followed by the 6-ketone brassinosteroids with the non-oxidized brassinosteroids having no activity (Bajguz & Tretyn, Citation2003). This has also been shown in microalgae where exogenous application of three BL and three CS analogues stimulated growth and increased protein and nucleic acid content in C. vulgaris with the three BL analogues being more effective than the CS analogues (BL > 24-epiBL > homoBL > CS > 24-epiCS > homoCS; Bajguz, Citation2000). In the present study, CS was consistently the predominant endogenous brassinosteroid in the six microalgae species, suggesting that brassinosteroids were actively involved in regulating physiological processes in the cells.
Previous studies investigating the role of brassinosteroids in growth and stress responses in microalgae used C. vulgaris as the model organism. Exogenous brassinosteroids were applied and changes in physiological processes and biochemical content measured. The present study is the first to report on changes in brassinosteroids in other microalgae species and to quantify changes in the endogenous brassinosteroids in response to external stimuli.
In the present study, addition of NaCl induced salt stress as seen by the decline in cell numbers in three species. There was a rapid response to salt stress with a significant increase in the endogenous brassinosteroid content within 30 min in all six species. The brassinosteroid content generally remained at elevated concentrations after 7 h in the light period. In contrast, a slower decrease in temperature from 25°C to 15°C had less effect on the brassinosteroid content compared with the rapid salt-shock treatment in the six species. A similar rapid response was measured with exogenous application of brassinosteroids to heavy metal-stressed C. vulgaris where the synthesis of phytochelatins (organic ligands involved in chelation of heavy metals for detoxification) was rapid, with changes in the phytochelatin content occurring within the first few hours of brassinosteroid application (Bajguz, Citation2002).
In vascular plants, brassinosteroids are an integral part of the hormonal signalling network where they are actively involved in crosstalk with other phytohormones influencing certain physiological responses to environmental stimuli (Fariduddin et al., Citation2014; Oklestková et al., Citation2015; Rajewska et al., Citation2016). In algae, crosstalk has been demonstrated in C. vulgaris where exogenous BL increased the thermotolerance of heat-stressed cultures by increasing the biosynthesis of abscisic acid (ABA) within 3 h of application (Bajguz, Citation2009b); exogenous BL also partially overcame the inhibitory effects of heavy metals on C. vulgaris, decreasing the accumulation of heavy metals in the cells and increasing ABA, indole-3-acetic acid (IAA) and zeatin content although there was no change in the endogenous BL content (Bajguz, Citation2011). Furthermore, brassinosteroids stimulated the levels of endogenous auxins in C. vulgaris and co-application of auxins and brassinosteroids acted synergistically to stimulate growth and the accumulation of proteins, chlorophylls and monosaccharides (Bajguz & Piotrowska-Niczyporuk, Citation2013); exogenous cytokinins stimulated the levels of endogenous brassinosteroids with a synergistic effect between exogenous cytokinins and exogenous BRs where there was an increase in cell number, proteins, chlorophylls and monosaccharides in a dose-effect relationship (Bajguz & Piotrowska-Niczyporuk, Citation2014).
The six microalgae strains analysed in the present study were part of a previous study where endogenous auxins, cytokinins, gibberellins and brassinosteroids were detected and quantified in 4-day old cultures (Stirk et al., Citation2013a, Citation2013b). Thus it is likely that the rapid increase in endogenous brassinosteroids in response to salt stress in the present study would have been accompanied by changes in the other hormone concentrations. A more detailed investigation on the effects of external stimuli on hormone homeostasis is required in order to understand the role of hormones in regulating abiotic defence mechanisms in microalgae.
In conclusion, this was the first study to show that endogenous brassinosteroids increase in response to abiotic stress in a number of microalgae species. This response was rapid with increased brassinosteroid levels within 30 min of the salt shock. Understanding the role of brassinosteroids in the stress responses of microalgae could enhance the value of in vivo production of bioactive microalgae metabolites.
Supplementary information
The following supplementary material is accessible via the Supplementary Content tab on the article’s online page at https://doi.org/10.1080/09670262.2018.1441447
Supplementary table. Brassinosteroids identified in the six microalgae strains exposed to salt and cold temperature stress and harvested after 30 min and 7 h exposure.
Acknowledgements
This research was funded by the project SABANA (Grant no. 727874) from the European Union Horizon 2020 Research and Innovation Program, the National Research Foundation of South Africa (grant number 105997) and the Ministry of Education, Youth and Sports, Czech Republic (grant LO1204 from the National Program of Sustainability I and Agricultural Research).
Disclosure statement
No potential conflict of interest was reported by the authors.
Additional information
Funding
Notes on contributors
Wendy A. Stirk
WA Stirk: experimental design, wrote and edited manuscript; P Bálint: culture experiments; D Tarkowská: brassinosteroid analysis, drafting and editing manuscript; M Strnad: drafting and editing manuscript; J van Staden: drafting and editing manuscript; V Ördög: original concept; drafting and editing manuscript.
References
- Bajguz, A. (2000). Effect of brassinosteroids on nucleic acids and protein content in cultured cells of Chlorella vulgaris. Plant Physiology and Biochemistry, 38: 209–215.
- Bajguz, A. (2002). Brassinosteroids and lead as stimulators of phytochelatins synthesis in Chlorella vulgaris. Journal of Plant Physiology, 159: 321–324.
- Bajguz, A. (2009a). Isolation and characterization of brassinosteroids from algal cultures of Chlorella vulgaris Beijernck (Trebouxiophyceae). Journal of Plant Physiology, 166: 1946–1949.
- Bajguz, A. (2009b). Brassinosteroid enhanced the level of abscisic acid in Chlorella vulgaris subjected to short-term heat stress. Journal of Plant Physiology, 166: 882–886.
- Bajguz, A. (2010). An enhancing effect of exogenous brassinolide on the growth and antioxidant activity in Chlorella vulgaris cultures under heavy metal stress. Environmental and Experimental Botany, 68: 175–179.
- Bajguz, A. (2011). Suppression of Chlorella vulgaris growth by cadmium, lead and copper stress and its restoration by endogenous brassinolide. Archives of Environmental Contamination and Toxicology, 60: 406–416.
- Bajguz, A. & Asami, T. (2004). Effects of brassinazole, an inhibitor of brassinosteroid biosynthesis, on light- and dark-grown Chlorella vulgaris. Planta, 218: 869–877.
- Bajguz, A. & Czerpak, R. (1998). Physiological and biochemical role of brassinosteroids and their structure-activity relationship in the green alga Chlorella vulgaris Beijerinck (Chlorophyceae). Journal of Plant Growth Regulation, 71: 131–139.
- Bajguz, A. & Piotrowska-Niczyporuk, A. (2013). Synergistic effect of auxin and brassinosteroids on the growth and regulation of metabolite content in the green alga Chlorella vulgaris (Trebouxiophyceae). Plant Physiology and Biochemistry, 71: 290–297.
- Bajguz, A. & Piotrowska-Niczyporuk, A. (2014). Interactive effect of brassinosteroids and cytokinins on growth, chlorophyll, monosaccharide and protein content in the green alga Chlorella vulgaris (Trebouxiophyceae). Plant Physiology and Biochemistry, 80: 176–183.
- Bajguz, A. & Tretyn, A. (2003). The chemical characteristic and distribution of brassinosteroids in plants. Phytochemistry, 62: 1027–1046.
- Bajguz, A., Bajguz, A.J. & Tryniszewska, E.A. (2013). Recent advances in medicinal applications of brassinosteroids, a group of plant hormones. In Studies in Natural Products Chemistry, vol. 40 (Atta-ur-Rahman, F.R.S., editor), 33–49. Elsevier, Amsterdam.
- Bartwell, A., Mall, R., Lohani, P., Guru, S.K. & Arora, S. (2013). Role of secondary metabolites and brassinosteroids in plant defense against environmental stresses. Journal of Plant Growth Regulation, 32: 216–232.
- Bold, H.C. (1949). The morphology of Chlamydomonas chlamydogma sp. nov. Bulletin of the Torrey Botanical Club, 76: 101–108.
- Fariduddin, Q., Yusuf, M., Ahmad, I. & Ahmad, A. (2014). Brassinosteroids and their role in response of plants to abiotic stress. Biologia Plantarum, 58: 9–17.
- Fujioka, S. & Yokota, T. (2003). Biosynthesis and metabolism of brassinosteroids. Annual Review of Plant Biology, 54: 137–164.
- Oklestková, J., Rárová, L., Kvasnica, M. & Strnad, M. (2015). Brassinosteroids: synthesis and biological activities. Phytochemical Reviews, 14: 1053–1072.
- Park, S.H., Han, K.S., Kim, T.W., Shim, J.K., Takatsuto, S., Yokoto, T. & Kim, S.K. (1999). In vivo and in vitro conversion of teasterone to typhasterol in cultured cells of Marchantia polymorpha. Plant and Cell Physiology, 40: 955–960.
- Rajewska, I., Talarek, M. & Bajguz, A. (2016). Brassinosteroids and response of plants to heavy metal action. Frontiers in Plant Science, 7: 629.
- Stirk, W.A., Bálint, P., Tarkowská, D., Novák, O., Strnad, M., Ördög, V. & van Staden, J. (2013a). Hormone profiles in microalgae: Gibberellins and brassinosteroids. Plant Physiology and Biochemistry, 70: 348–353.
- Stirk, W.A., Ördög, V., Novák, O., Rolčík, J., Strnad, M., Bálint, P. & van Staden, J. (2013b). Auxin and cytokinin relationships in 24 microalgal strains. Journal of Phycology, 49: 459–467.
- Stirk, W.A., Tarkowská, D., Turečová, V., Strnad, M. & van Staden, J. (2014). Abscisic acid, gibberellins and brassinosteroids in Kelpak®, a commercial seaweed extract made from Ecklonia maxima. Journal of Applied Phycology, 26: 561–567.
- Takatsuto, S., Abe, H. & Gamoh, K. (1990). Evidence for brassinosteroids in strobilus of Equisetum arvense L. Agricultural and Biological Chemistry, 54: 1057–1059.
- Tarkowská, D., Novák, O., Oklestková, J. & Strnad, M. (2016). The determination of 22 natural brassinosteroids in a minute sample of plant tissue by UHPLC-MS/MS. Analytical and Bioanalytical Chemistry, 408: 6799–6812.
- Yokota, T., Kim, S.K., Fukui, Y., Takahashi, N., Takeuchi, Y. & Takematsu, T. (1987). Brassinosteroids and sterols from a green alga, Hydrodictyon reticulatum: configuration at C-24. Phytochemistry, 26: 503–506.