ABSTRACT
The morphology and ontogenetic allometric trends of a rare diatom Fragilaria heidenii Østrup and the morphologically related Staurosira tabellaria (W. Smith) Leuduger-Fortmorel were compared using conventional and semilandmark-based geometric morphometric analyses. Fragilaria heidenii was studied in detail by light and electron microscopy using type material and recent samples from Lake Ladoga and Lake Ilmen (north-western Russia). The taxon is transferred into the genus Staurosira Ehrenberg as Staurosira inflata comb. nov. on the basis of its valve morphology. This taxon is characterized by the absence of rimoportulae, lack of perforated copulae, spines located on the interstriae, internal vola occlusion in the areolae and the features of the areolae and apical pore fields. Conventional morphometric analysis showed considerable overlapping of S. inflata and S. tabellaria in their frustule characteristics such as length, width, length-to-width ratio and striae density. Moreover, at later stages of the vegetative life cycle, S. inflata has a tendency to resemble S. tabellaria by its valve outline that makes it difficult to separate these two taxa. The geometric morphometric analysis revealed two shape groups corresponding to S. inflata and S. tabellaria that were separated by a clear gap. Semilandmarks representing shape of the middle part of the valve were primarily responsible for discrimination between species. Apart from differences in valve shapes, S. inflata and S. tabellaria also differed significantly in their ontogenetic allometric trajectories. Overall, our results demonstrate that the semilandmark-based geometric morphometrics is sensitive enough to distinguish species by their outlines, when traditional morphometric parameters are not able to discriminate them with confidence.
Introduction
Fragilaria heidenii Østrup is a rare diatom, poorly cited in the literature. It was originally described by Heiden as Synedra inflata (Heiden, Citation1900, p. 14, fig. 19). Østrup (Citation1910) transferred this species to the genus Fragilaria and gave it the new name Fragilaria heidenii (the epithet ‘inflata’ was already taken by Fragilaria inflata Pantocsek; Pantocsek, Citation1902) arguing that it grew forming ribbon-shaped colonies like other taxa included in the genus (Østrup, Citation1910, p. 191, fig. 118). In 1926, Schulz provided the combination Fragilaria virescens var. inflata (Heiden) Schulz, meaning that he considered Heiden’s taxon (Synedra inflata) to be simply a variation instead of a species on its own (Schulz, Citation1926). Hustedt (Citation1931) provided yet another combination, Fragilaria inflata (Heiden) Hustedt, which is not correct because, as stated previously, the name inflata was already taken by F. inflata Pantocsek.
Fig. 1. Map of Lake Ladoga and Lake Ilmen (north-western Russia) and the location of sampling points. Additional geographic information is provided in .
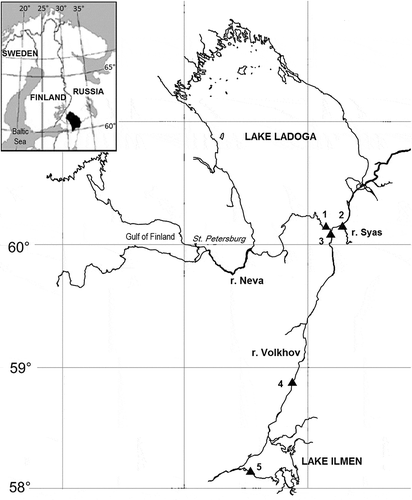
Fig. 2. Consensus configuration for Fragilaria heidenii and Staurosira tabellaria representing the placement of landmarks. Landmarks 1–4 are fixed. Semilandmarks 5–12 slide between 1 and 2. Semilandmarks 13–20 slide between 2 and 3. Semilandmarks 21–28 slide between 3 and 4. Semilandmarks 29–36 slide between 4 and 1.
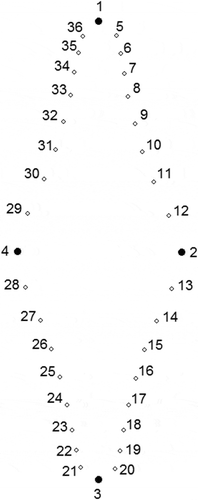
Figs 3–7. Fig. 3. Synedra inflata, original drawing produced by Heiden (1900). Fig. 4. Fragilaria heidenii, original drawing produced by Østrup (1910). Fig. 5. LM image of S. inflata from type slide made by Heiden (Rostock 0,15 Conventer See 3,2 m tief, B, Herbarium Berolinense). Fig. 6. LM image of broken valve of F. heidenii from original slide made by Østrup (3466, 20:190, C, Botanical Museum Copenhagen U096/2006 No. 1). Fig. 7. LM image of F. heidenii colonies in girdle view also from the slide made by Østrup. Scale = 10 µm.
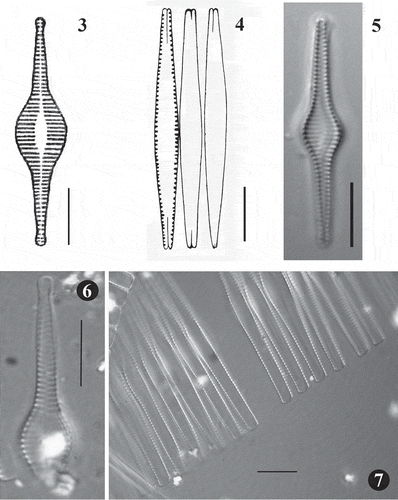
Figs 8–15. SEM images of Fragilaria heidenii Østrup from raw material collected by Østrup in Frederiksværk (Denmark) (C, Østrup Collection, Raw material 384.I Rant. 4244). Fig. 8. Broken frustules of a colony showing tight linkage between contiguous cells. Fig. 9. Inner view of central area showing details of the striae. Also, notice the spatulate spines linking neighbouring valves. Fig. 10. Outer view of valve showing striae, position of spines and lanceolate axial area. Fig. 11. Detail of central area in outer view showing the areolae on the mantle and broken off spines located on the interstriae. Fig. 12. Central area in outer view showing spatulate spines and small, elliptical to round areolae. Fig. 13. Side view of two neighbouring frustules showing unperforated girdle bands. Notice tapered spine structure toward valve apex and loose attachment between valves. Fig. 14. Inner view of apex covered by a film deposit. Notice structure of apical pore field on the valve below. Fig. 15. Inner view of valve. Scale = 10 µm (, , ), 5 µm (, , ), 2.5 µm (), 1 µm ().
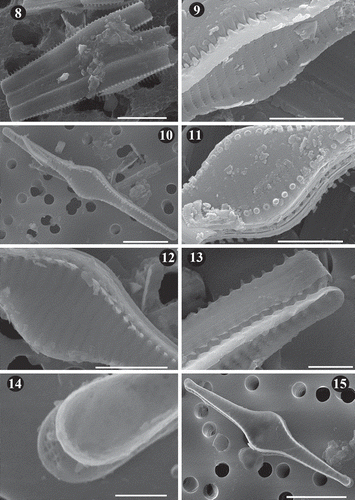
Figs 16–19. SEM images of Fragilaria heidenii Østrup from raw material collected by Østrup in Frederiksværk (Denmark) (C, Østrup Collection, Raw material 384.I Rant. 4244). Fig. 16. Side view of two large contiguous valves. . Outer view of mid-region of valve showing lanceolate axial area, areolae varying from elliptical to round, location of spines and raised interstriae. Fig. 18. Inner view of mid-region of valve showing vola occlusion in areolae. Notice raised interstriae. Fig. 19. Middle part of two broken neighbouring frustules. Notice internal vola occlusion of areolae and striae extending onto the valve mantle. Scale = 4 µm (), 1 µm (–).
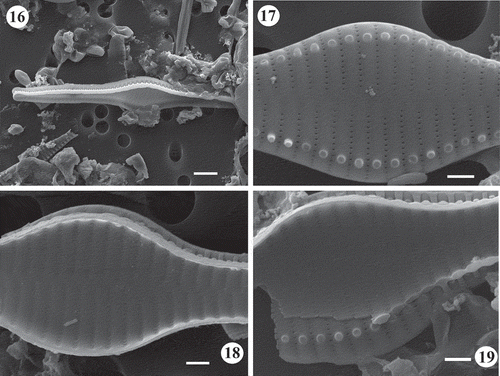
Krammer & Lange-Bertalot (Citation2000) considered both Synedra inflata Heiden and Fragilaria inflata (Heiden) Hustedt as synonyms of Fragilaria heidenii Østrup. Working on ‘lectotype’ material of F. heidenii from Østrup collection, Lange-Bertalot made reference to the unresolved relation between F. heidenii and F. hungarica Pantocsek (Krammer & Lange-Bertalot, Citation2000, p. 133, pl. 116, figs 8, 9). Later, in a detailed morphological and taxonomic study of F. hungarica, Ács et al. (Citation2009) showed this taxon should be transferred to the genus Staurosira sensu Williams & Round (Citation1987) under the new name Staurosira grigorszkyi Ács, E. Morales & Ector. This study provided evidence that although the valve outline of larger specimens of S. grigorszkyi resemble F. heidenii, colonies do not possess widely open apices between contiguous valves (Ács et al., Citation2009), a feature characteristic for F. heidenii. Recently, based on investigation of type material from the W. Smith collection of Odontidium tabellaria W. Smith (Smith, Citation1856, p. 17, pl. 34, fig. 291), Morales et al. (Citation2015) presented the combination Staurosira tabellaria (W. Smith) Leuduger-Fortmorel in Leuduger Fortmorel & Petit (1878, p. 179) as a more appropriate and valid name for S. grigorszkyi. Whether F. heidenii and S. tabellaria should be considered as taxonomically distinct or conspecific species remains to be resolved by the analysis of type material of the former and through morphological comparison of both taxa.
Valve shape is one of the important morphological characteristics used for distinction among diatom species. Shape is directly inherited during the vegetative phase of the diatom life cycle as a result of the moulding of the daughter valve mantles by the parent frustules (Mann, Citation1984). Therefore, diatom shape is inextricably linked to valve ontogeny, in the course of which the shape of a new cell physically determines the shape of its progeny (Mann, Citation1994). However, an exception exists for initial cells produced through sexual reproduction, which often differ from the normal vegetative cells in various aspects of valve morphology (Mann & Chepurnov, Citation2005, and references therein). In vegetative reproduction, most diatoms undergo size reduction, which is accompanied by changes in frustule shape. A subtle deformation of daughter cells with subsequent cell divisions leads to the well-known phenomenon that toward the end of size reduction the diatom valves could become more elliptical in outline and less undulated (Geitler, Citation1932). Thus, towards the end of the size diminution series, diatom valves tend to lose their diagnostic features, making them less distinguishable. Hence, for a reliable distinction between diatom species, we need to know full ranges of intraspecific shape variations determined by allometric changes relating to the size diminution of frustules. The ratios of quantitative morphological characters to valve length (or valve diameter in centric diatoms) are often used for distinguishing closely related diatom taxa (e.g. Edgar & Theriot, Citation2004; Potapova & Ponader, Citation2004; Chudaev & Gololobova, Citation2012; Edgar et al., Citation2015). These ontogenetic allometric trends are utilized as additional morphological characteristics of diatom taxa in order to reveal their developmental sequences, which are a basic attribute of gene expression embodied in form (Stoermer et al., Citation1986). However, the application of ratios of taxonomic variables as morphological characteristics should be made cautiously because they are often interdependent and non-linearly related to the valve size (Theriot, Citation1988). In this regard, the technique of geometric morphometric shape analysis, which allows visualizing ontogenetic allometric trajectories in the multivariate morphospace, has proved to be a useful and effective extension of the traditional morphometric approach (e.g. Stoermer & Ladewski, Citation1982; Mou & Stoermer, Citation1992; English & Potapova, Citation2012; Edgar et al., Citation2015; Kulichová & Fialová, Citation2016).
During a monitoring survey of Lake Ladoga (north-western Russia) and its tributaries in 2010 and 2014–2015, sympatric populations of two species identified as F. heidenii and S. tabellaria were found in samples collected from different environments (lentic and lotic) and habitats (plankton and periphyton). We made detailed investigations of the valve morphology of F. heidenii based on light microscopy (LM) and scanning electron microscopy (SEM) of type material and recent north-western Russian populations of this species, in order to revise its taxonomy and nomenclature. To reveal morphological differences between F. heidenii and S. tabellaria we used conventional and geometric morphometric approaches. In addition, to better clarify the differences between these species we compare their ontogenetic allometric trends.
Materials and methods
Type material of Synedra inflata was obtained from the Fricke collection no. XLI 19 at the Herbarium Berolinense (B) in the Botanic Garden and Botanical Museum Berlin-Dahlem. The permanent slide (Dr. Heiden Rostock 0,15 Conventer See 3,2 m tief) was examined. No raw material was available for analysis.
Reference material of Fragilaria heidenii was obtained from the Østrup collection in the Botanical Garden and Museum of the University of Copenhagen (C). The permanent slide (3466, 20:190, Botanical Museum Copenhagen U096/2006 No. 1) and raw material (Østrup 384.I Rant. 4244) were examined.
Samples of recent epiphytic material containing F. heidenii and S. tabellaria were collected at five sampling sites in July–August of 2010 and 2014–2015 from two large lakes in north-western Russia – Lake Ladoga and Lake Ilmen, and the Volkhov river belonging to the Lake Ladoga catchment (). Details of the sampling sites are presented in . In addition, for the geometric morphometric analysis, we used several published images of S. tabellaria (syn: S. grigorszkyi) taken from type material in the Pantocsek collection (BP) and from recent material collected in Lake Balaton (Hungary) (Ács et al., Citation2009).
Table 1. General information of sites sampled for the present study.
Recent plankton samples from north-western Russia were collected from the surface water layer, preserved with Lugol’s solution, and then concentrated by sedimentation in the laboratory. Recent periphytic material (epiphyton) was collected from living reed stems (Phragmites australis (Cav.) Trin. ex Steud.). Stems were chosen randomly and at least five 10–15 cm long stem sections were collected at the sampling site. In the field, the epiphyton was gently brushed from plant pieces with a toothbrush, and the resulting algal suspensions were then put in a small plastic bottle and fixed in 4% formaldehyde solution.
Samples from north-western Russia were treated with potassium dichromate in hot sulphuric acid according to Balonov (Citation1975), washed three times in distilled water, and centrifuged at 2000 rpm for 10 min for decantation. Samples of raw material from the Østrup collection were cleaned with hydrogen peroxide and hydrochloric acid (according to CEN, 2003), and subsequently washed three times with distilled water. For SEM analysis, portions of clean material were filtered through a polycarbonate Millipore membrane filter (3 µm pore size), mounted on aluminium stubs using double-sided carbon tape, and coated with gold using a rotary-pumped spatter coater Quorum Q150R. Stubs were examined using Zeiss EVO MA 10 SEM operated at 15 kV and 10 mm distance using secondary electrons (SE). For LM analysis, diatoms were mounted on glass slides with Naphrax® mounting medium and observed with a Zeiss AxioImager D1 microscope equipped with Nomarski optics. LM photos were obtained using an AxioCam MRm digital camera.
Conventional morphometric analysis was performed using the following quantitative characteristics: valve length and width, length-to-width ratio, and striae density in 10 µm. To compare the characteristics of F. heidenii with the morphologically closest taxon S. tabellaria, we measured 30 valves of each taxon from Russian samples using LM images.
Semilandmark-based geometric morphometric analysis (Bookstein, Citation1997) was used to assess whether there were distinct shape groups corresponding to the two investigated taxa. This approach is more effective than landmark-based method in capturing information about shape curvature, because semilandmarks are able to quantitatively slide along an outline (Bookstein, Citation1997), whereas landmarks represent discrete homologous points of shape (Bookstein, Citation1991). For the semilandmark-based shape analysis, we used 30 specimens of F. heidenii and 30 specimens of S. tabellaria from Russian samples: one from the Heiden’s type slide of Synedra inflata in the Fricke collection (B), four from reference raw material of F. heidenii in the Østrup collection (C), five from type material of S. tabellaria (syn: S. grigorszkyi) in the Pantocsek collection (BP) (Ács et al., Citation2009), and seven specimens of S. tabellaria (syn: S. grigorszkyi) from Hungarian samples (Ács et al., Citation2009). To perform semilandmark-based shape analysis, we used the TPS-series software (Rohlf, Citation2007) available from http://life.bio.sunysb.edu/morph/index.html. The TpsDig2 ver. 2.16 program was used to digitize landmarks on the valve outline using LM images. Of these 36 landmarks, four were fixed and remaining landmarks were semilandmarks that were allowed to slide along the outline curve (Bookstein, Citation1997). The four fixed landmarks were positioned at the intersections of the valve outline with the apical and transapical axes and four sets of eight sliding semilandmarks were placed between the four fixed landmarks ().
The generalized Procrustes analysis was used to superimpose configurations of both landmarks and semilandmarks in all specimens to a common coordinate system and produce a set of shape variables (Rohlf & Slice, Citation1990). The Procrustes superimposition involves least squares algorithm to fit each configuration to the average configuration which is usually called ‘consensus’ configuration. This iterative procedure scales all specimens to unit centroid size, shifts them to the same centre of gravity, and rotates them to align all configurations in a dataset to the consensus configuration.
Partial warp scores that describe positions of specimens relative to the consensus configuration in the morphospace were obtained using tpsRelw ver. 1.49. The relative warp (RW) analysis, an analogue of principal component analysis of partial warps, which characterizes directions of shape deformations within a dataset (Bookstein, Citation1991; Rohlf, Citation1993), was performed using the same program. Because we expected that small-scale morphological characteristics would be more important for discrimination of specimens, we set the parameter α to –1 (Rohlf, Citation1993). The first two relative warps are the most important principal components capturing shape variability in a dataset. To examine allometry, size-related shape changes within F. heidenii and S. tabellaria populations from north-western Russia, we regressed scores of the first two relative warps on their valve length. In order to visualize the ontogenetic allometric trends in the two studied populations, the first two relative warps were plotted against valve length. Analysis of covariance (ANCOVA) was used to assess the relationships of valve length to length-to-width ratio and to the first two relative warps. The homogeneity of slopes model was used to test whether the continuous predictor variable (valve length) had a different effect at different levels of categorical independent variables (species).
Morphological terminology follows Anonymous (1975), Ross et al. (Citation1979) and Round et al. (Citation1990).
Results
Comparison of specimens of Fragilaria heidenii from lectotype material with those found in recent material from north-western Russia
Line drawings of F. heidenii published by Heiden (Citation1900) and Østrup (Citation1910) are presented in and , respectively. LM micrographs of specimens of F. heidenii from Hustedt collection were presented by Krammer & Lange-Bertalot (2000, pl. 116, figs 8–10). We examined the original type slide of Synedra inflata made by Heiden (Citation1900), stored in the Botanic Garden and Botanical Museum Berlin-Dahlem (B). In the type slide of Synedra inflata, we could only find one valve in valve view (). We analysed the slide containing F. heidenii and raw material collected by Østrup from Frederiksværk (Hovedstaden Region, Zealand, Denmark) on 30 July 1892. In the slide, we could only find one broken valve in valve view () and many frustules in girdle view (). Preparations based on raw material showed that this taxon was not very abundant – we could find only 20 specimens that were imaged and measured. Comparison of images of specimens from the Heiden’s slide () and the Østrup collection (, , ) with images of specimens from recent samples collected in north-western Russia (, –) show the same taxon.
Figs 20–25. SEM images of Staurosira inflata. Lake Ladoga and Lake Ilmen material. Fig. 20. Side view of two neighbouring frustules showing unperforated girdle bands. Notice longer spines at the central part of the valve. Fig. 21. General internal view of frustule. Notice lack of rimoportulae. Fig. 22. Inner view of frustule. Notice vola occlusion of areolae and raised interstriae. Fig. 23. Inner view of apex. The small apical pore field can be seen. Fig. 24. General external view of frustule. Notice striae perpendicular to the apical axis at the valve centre and slightly radial at the ends. Fig. 25. Close up of valve in showing striae that extend onto the valve mantle. Scale = 5 µm (), µm (), 2 µm (), µm (, ), 0.5 µm ().
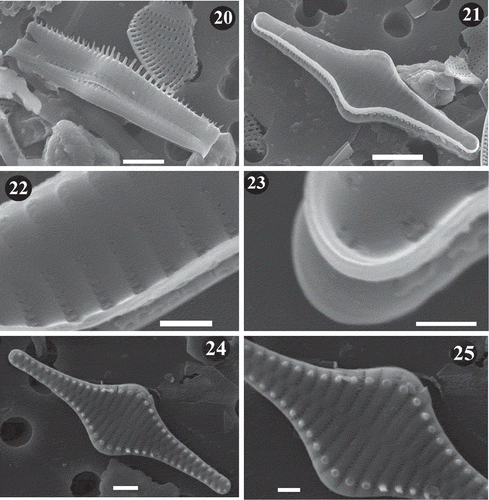
Figs 26–29. SEM images of Staurosira tabellaria. Lake Ladoga and Lake Ilmen material. Fig. 26. General external view of frustule showing details of striae and location of spines on the interstriae. Fig. 27. Internal view of frustule showing mantle at apexes. Notice the absence of rimoportulae and lack of ornamentation on girdle bands. Fig. 28. External view of large frustule. Notice raised interstriae and spines located on them. Fig. 29. Close up of apex of valve in . Notice details of apical pore field. Scale = 2 µm (–), 0.5 µm ().
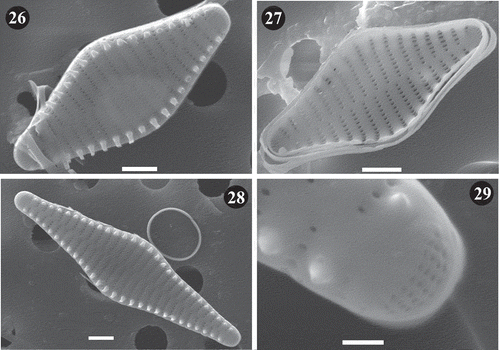
Figs 30–43. Staurosira inflata, Lake Ladoga and Lake Ilmen populations. LM photographs showing size diminution series. Scale = 10 µm.
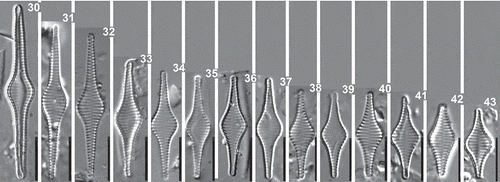
Krammer & Lange-Bertalot (Citation2000) working on ‘lectotype’ material of F. heidenii from the Østrup collection gave the following measurements for F. heidenii: length 20–50 µm, width 6–10 µm and striae density between 13 and 15 in 10 µm. In our examination of reference raw material of F. heidenii, the dimensions of specimens were more or less in the same range of variation: length varied between 33–56 µm, width 7–8 µm and striae density ranged between 13 and 15 in 10 µm. Somewhat smaller specimens were found in recent samples collected in north-western Russia. Their length varied from 16 to 43 µm, their breadth from 6 to 7 µm. The density of striae was 13 to 15 in 10 µm. A total of 30 specimens were observed and measured using LM (–).
The examined material of F. heidenii reveals that frustules are rectangular in girdle view and they form ribbon-like chains (, ). Frequently, neighbouring frustules have separated ends (), similar to colonies of F. crotonensis Kitton. Valves are lanceolate with a conspicuously inflated central portion and strongly narrowed subcapitate ends (, , , , , –). Striae are alternate, perpendicular to the apical axis and parallel at the valve centre, and slightly radial at the ends (, , , ). SEM shows that striae lie along depressions due to raised interstriae (, , ). A slight elevation of the interstriae with respect to the striae can be observed also in internal view (, , ). The areolae are arranged in uniseriate rows that extend onto the valve mantle (, , , ). They are mostly elliptic and become smaller and round as they approach the ends of striae on the valve surface and mantle (, , ). The number of areolae along the striae is around 50 in 10 µm. Because the areolae are very small, the volae are difficult to observe but they can be seen in the inner side of some valves (, , ). The axial area is narrow and lanceolate, wider at the central area (, , ). Apical pore fields are greatly reduced and composed of around two-three rows of single, round poroids (, ). Spines are solid, and located on the interstriae at the valve face-mantle junction (, , , ). They are spatulate at the mid-region of the valve and become tapered toward the apex (, , ). The valve face-mantle junction gently drops beyond the point of insertion of the spines (, , ). Images of girdle elements are not clear probably due to the state of preservation of the material, but we could observe that the copulae are open, narrow and lack perforations (, , ). The valvocopula is wider and also lacks perforations (). Rimoportulae are absent (, ).
The SEM information we provide here for F. heidenii, such as the absence of rimoportulae, lack of perforated copulae, internal vola occlusion of areolae, and spines located on the interstriae, makes it evident that it is a member of the genus Staurosira. We propose the following combination for this organism:
Staurosira inflata (Heiden) A.Rusanov, Ács, E.Morales & Ector comb. nov.
Basionym: Synedra inflata Heiden in Mitth. Mecklenburg. Geol. Landesanst. 10(21): 14, fig. 19. 1900. ≡ Fragilaria heidenii Østrup Danske Diatoméer: 190, tab. 5, fig. 118. 1910.
Morphological examination of Staurosira tabellaria in recent material from north-western Russia
In recent samples collected in north-western Russia, a total of 30 specimens of S. tabellaria were observed and measured using LM. The cell length of S. tabellaria varied from 11 to 23 µm, the cell width from 6 to 7 µm. The density of striae was 13 to 16 in 10 µm. These measurements were within the range determined by Ács et al. (Citation2009) and Morales et al. (Citation2015).
In LM, valve outline of S. tabellaria varies from rhomboid to elliptical with tapering ends, ending in narrowly rounded apices (–). Striae are alternate, parallel and perpendicular to the apical axis. The axial area varies from linear to narrow lanceolate. In SEM, areolae vary from slightly apically elongated to round (, ). Uniseriate rows of areolae continue from valve face to the mantle (). Apical pore fields are well developed with approximately four rows of poroids, and 5–8 poroids per row (). Spines are located on interstriae (, ) that are slightly elevated (, ). Copulae are open, narrow and lack perforations (). Rimoportulae are absent (). SEM confirmed that S. tabellaria specimens from recent Russian samples have characteristic features such as the absence of rimoportulae, lack of perforated copulae, spines located on the interstriae and the characteristics of the areolae which are the main features of the genus Staurosira.
Conventional and geometric morphometric analyses of Staurosira inflata and Staurosira tabellaria
Conventional morphometric analysis showed considerable overlapping of S. inflata and S. tabellaria from north-western Russian samples in their frustule characteristics such as length, width, length-to-width ratio and striae density (). Comparison of size diminution series of S. inflata and S. tabellaria showed that at low valve length S. inflata had a tendency to resemble S. tabellaria by its valve outline (–, –). The overlap in aspect ratios between both species is illustrated by the plot of length-to-width ratio against valve length where the convergence of the late ontogenetic stages of S. inflata and early stages of S. tabellaria is clearly evident (). No distinct lines of growth trajectories corresponding to change in length-to-width ratio were found: ANCOVA showed that equality of slopes could not be rejected (F = 1.01; P = 0.32). Results from ANCOVA also showed that regression lines did not significantly differ in their intercepts between different species (, ), indicating equal rates of change of the length-to-width ratio in respect to length for both taxa.
Table 2. Results of the analysis of covariance (ANCOVA), testing relationships among valve length and length-to-width ratio at different levels of categorical independent variables (species). Analysis tests whether the regression lines differ in their intercepts.
Fig. 59. Box-plot diagrams showing variation in valve length (A), width (B), length-to-width ratio (C), and striae density (D) of S. inflata (SI) and S. tabellaria (ST). Point represents mean, whiskers indicate max and min of the data.
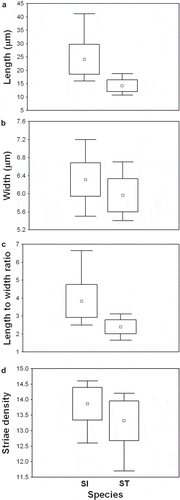
Fig. 60. Plot of length-to-width ratio against valve length for S. inflata and S. tabellaria. Each taxon is represented by linear regression line reflecting aspect ratio variation with length. The morphospace was contoured (dashed horizontal lines) by set of standard shape descriptors based on length-to-width ratios proposed by Cox (Citation1995). The ratios are shown on the left side of the plot; morphological characters (lanceolate, elliptical etc.) corresponding to these ratios are shown on the right side.
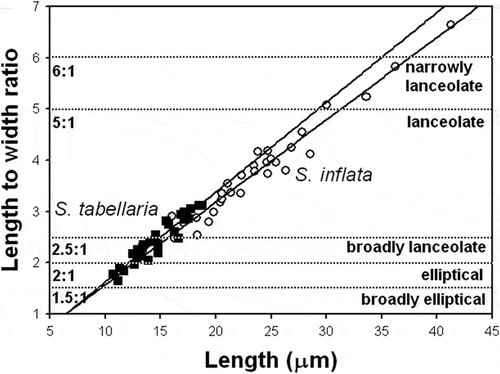
In order to define shape variation with standard shape descriptors we used a morphological terminology linked to the ratio of a shape’s major and minor axes, as proposed by Cox (Citation1995). According to this scheme, reference points define a certain zone for each category along the shape continuum: narrowly lanceolate (6:1), lanceolate (5:1), broadly lanceolate (2.5:1), elliptical (2:1) and broadly elliptical (1.5:1). Using this standard descriptive terminology for valve shape, all specimens of S. inflata basically fell into categories of lanceolate and narrowly lanceolate, while specimens of S. tabellaria varied from elliptical to broadly lanceolate and lanceolate (). The overlap between two species lay in the category lanceolate close to broadly lanceolate (). Actually, specimens of S. tabellaria, which fell into category lanceolate on the basis of their length-to-width ratios, belonged to the early ontogenetic stages with rhomboid shape (–).
In the relative warp analysis, the first and second warps accounted for 53.4 and 19.7% of shape variation, respectively. The plot of the first and second relative warps (RW1–2) showed two distinct groups of specimens, which were separated by a gap along the RW1 axis (). The specimens from the type population of S. inflata fell into the same group with specimens of S. inflata from recent north-western Russian samples. Likewise, specimens from the type population of S. tabellaria (Ács et al., Citation2009) fell into separate group with specimens of S. tabellaria from Hungarian samples (Ács et al., Citation2009) and recent Russian samples. RW1 scores produced a gradient from narrowly lanceolate valves with a conspicuously inflated middle section, to broadly lanceolate and elliptical valves with not-distinct middle section from the left to the right of the plot (). Thus, RW1 represented a dilation gradient in the middle region of the frustule. ANCOVA showed that equality of slopes of the relationships between RW1 and valve length of S. inflata and S. tabellaria was rejected (F = 53.5; P <0.0001). Both species had statistically distinct growth trajectories corresponding to shape variation captured by RW1 (, ). The rate of changes of this shape component with respect to valve length in S. tabellaria was much higher than in S. inflata.
Table 3. Linear regression-derived parameters for relationships of relative warps to valve length of Staurosira heidenii and S. tabellaria.
Fig. 61. Plot of the first and second relative warps (RW1–2) for S. inflata and S. tabellaria. Group outliers are connected by lines. (a) S. inflata collected in Lake Ladoga and Lake Ilmen (north-western Russia); (b) S. inflata from raw material in the Østrup collection; (c) S. inflata from the Heiden’s type slide; (d) S. tabellaria collected in Lake Ladoga and Lake Ilmen; (e) S. tabellaria (syn: S. grigorszkyi) from raw material in the Pantocsek collection collected in Lake Balaton (Ács et al., 2009); (f) S. tabellaria (syn: S. grigorszkyi) from recent samples collected in Lake Balaton (Ács et al., 2009). Illustrations inside the plot represent shape variation of S. inflata and S. tabellaria in the morphospace of the first two relative warps (RW1–2).
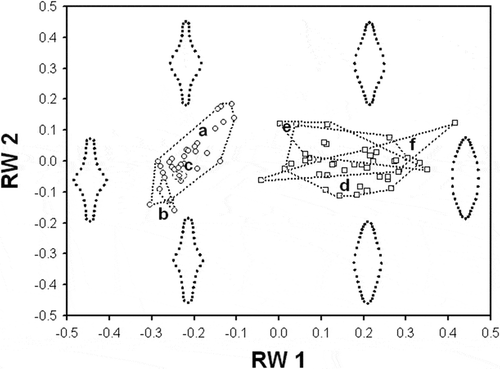
Fig. 62. Plot of the first relative warp (RW1) against valve length for S. inflata and S. tabellaria. Regression lines represent ontogenetic-allometric trends in S. inflata and S. tabellaria populations. Illustrations outside the plot represent extremes of the warp.
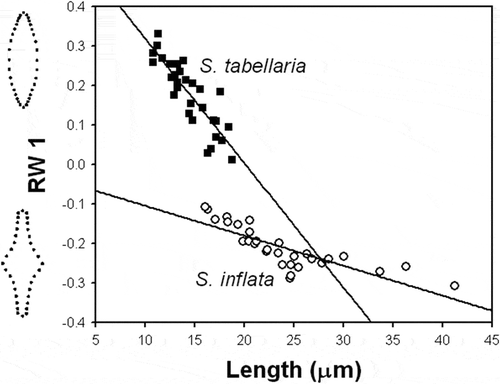
RW2 scores produced a gradient of variation in shape of the valve ends from broadly cuneate to narrowly attenuating from the bottom to the top of the plot (). Hence, RW2 reflected the manner in which the valve ends decrease in width as they progress toward the apices. Specimens of S. inflata varied in this shape character with respect to length, while specimens of S. tabellaria did not (, ). Although both species substantially overlapped in their RW2 scores, S. inflata had higher range of shape variation captured by RW2 than S. tabellaria ().
Discussion
Valve morphology of Staurosira inflata and related taxa
Staurosira inflata has several ultrastructural features that justify its placement into the genus Staurosira Ehrenberg as defined by Williams & Round (Citation1987). Among these features are a cingulum characterized by a lack of perforated copulae (, ) and larger valvocopulae also without perforations (); uniseriate striae composed of elliptical or round areolae (, , , ); spines located on the interstriae at the valve face-mantle junction (, , , ); and reduced apical pore fields consisting of 2–3 rows of poroids ().
Staurosira inflata shares with Fragilaria D.M. Williams & Round and closely related Fragilariforma D.M. Williams & Round a combination of morphological features such as frustules rectangular in girdle view and usually forming linear colonies, uniseriate striae extended onto the valve mantle, spines presented at the junction of valve face and mantle and apical pore fields (Williams & Round, Citation1987). The absence of rimoportula and the presence of unperforated copulae indicate that S. inflata does not belong to the genus Fragilaria since taxa in this genus should have at least one rimoportula lying at a pole (Tuji & Williams, Citation2006) and a single row of poroids presented on each copula (Williams & Round, Citation1987). An additional feature that distinguishes S. inflata from Fragilaria is the arrangement of linking spines on the interstriae, while taxa belonging to Fragilaria have spines interrupting the striae (Williams & Round, Citation1987). Likewise, S. inflata cannot be related to Fragilaria virescens (Fragilariforma virescens (Ralfs) D.M. Williams & Round) as suggested by Schulz (Citation1926) because taxa in the genus Fragilariforma have a single rimoportula present at one pole and perforated copulae (Williams & Round, Citation1987). In addition, S. inflata differs from Fragilariforma by its linear to narrowly lanceolate sternum that widens toward the valve centre, whereas Fragilariforma species possess very narrow and often indistinct sternum (Williams & Round, Citation1987).
An extensive literature search revealed several taxa currently included in Staurosira that are morphologically similar to S. inflata by its valve outline expanded in the middle area. Besides S. tabellaria (W. Smith) Leuduger-Fortmorel (Ács et al., Citation2009; Morales et al., Citation2015), this group of morphologically similar taxa includes the following species: S. construens Ehrenberg (Krammer & Lange-Bertalot, Citation2000), S. stevensonii Manoylov, E. Morales & Stoermer (Manoylov et al., Citation2003), S. longwanensis Rioual, E. Morales & Ector (Rioual et al., Citation2014), S. incerta E. Morales (Morales, Citation2006), and S. sviridae Kulikovskiy, Genkal & Mikheyeva (Kulikovskiy et al., Citation2011; Chudaev & Gololobova, Citation2012). The main characteristics of all species in this group are presented in . One of the main features distinguishing S. inflata from morphologically similar taxa of Staurosira, excepting S. stevensonii, is the open apices between neighbouring frustules along the ribbon-like colonies and that can be clearly visible under LM. This feature is due to presence of longer spines at the middle part of the valve (). Other morphological characteristics that distinguish the group of closely related taxa from S. inflata include characteristics of valve outline, frustule dimensions and ultrastructural features of the valves.
Table 4. Comparison of S. inflata with other morphologically similar taxa within the genus Staurosira.
Staurosira construens differs from S. inflata by its distinct cruciate outline and more acute central inflation. The valve ends in S. construens are slightly incurved as they progress toward the broadly rounded apices. In contrast, S. inflata has valve ends that gradually decrease in width, ending in narrowly rounded apices. The axial area of S. construens is wide and varies from linear to broadly lanceolate or elliptic (Krammer & Lange-Bertalot, Citation2000), while S. inflata possesses a much narrower central sternum. Staurosira construens has a higher striae density (). Additionally, striae in S. construens are visibly curved, especially at the central area, whereas in S. inflata the striae are mostly parallel.
Staurosira stevensonii possesses a cruciate shape like S. construens. However, the valve ends of S. stevensonii decrease in width more gradually, in a manner that closely resembles those of S. inflata. These two taxa differ morphologically in the shape of the central area inflation, which is much more pronounced in S. stevensonii than in S. inflata. As noted previously, neighbouring cells in colonies of S. stevensonii are disconnected at the apices (Manoylov et al., Citation2003), a feature that is also present in S. inflata. Staurosira stevensonii has shorter valves and higher striae density (). Both species also differ in shape of the axial area: S. stevensonii possesses a linear sternum, while S. inflata has the axial area varying from linear to narrowly lanceolate.
Staurosira longwanensis is generally lanceolate in valve outline and has a very faint inflation in the central portion of the valve. This combination of morphological features clearly differentiates this taxon from S. inflata. Also S. longwanensis differs in valve dimensions, having shorter and narrower valves than S. inflata (). Areolae in S. longwanensis vary from small oval to transapically extended, while in S. inflata areolae are more variable in shape, varying from round to elliptical or transapically elongated. Staurosira longwanensis has apical pore fields that are well-developed even in small valves having up to 5 rows of poroids, whereas S. inflata possesses very small pore fields composed by few poroids. The specific feature of S. longwanensis that distinguishes it from S. inflata and other Staurosira species is a presence of perforations on the girdle band (Rioual et al., Citation2014).
Staurosira incerta has lanceolate valves with inflation in the central part that makes it similar in outline to smaller valves of S. inflata. In S. incerta the valve ends are subrostrate (Morales, Citation2006), while S. inflata has attenuated and narrowly rounded valve ends. Staurosira incerta differs from S. inflata by its lower valve length and width. There are also distinct ultrastructural differences between these taxa. Areolae are commonly more elongated and apical pore fields are more developed in S. incerta than those in S. inflata.
Staurosira sviridae is another taxon with valve shape comparable to that of S. inflata. Valves in S. sviridae are elliptic-lanceolate in outline with an inflated middle portion (Kulikovskiy et al., Citation2011; Chudaev & Gololobova, Citation2012). Staurosira sviridae is smaller than S. inflata in both length and width (). Apical pore fields in S. sviridae are well-developed and composed of 5–7 rows of poroids. The axial area is lanceolate and widened in the valve centre.
Morphological comparison of Staurosira inflata and Staurosira tabellaria
Our analysis of sympatric populations of S. inflata and S. tabellaria showed that the former was larger in valve length and length-to-width ratio than the latter. However, substantial overlapping of their quantitative valve characteristics including length and length-to-width ratio (), precluded the use of the morphometric characters for precise delineation of species boundaries between these two taxa. In the same way, our attempt to utilize the size-dependent variation in quantitative characteristics was impractical to distinguish between S. inflata and S. tabellaria. For example, the plot of length-to-width ratio against valve length demonstrated the overlap between S. inflata and S. tabellaria in the narrow length range of 15–20 µm (), indicating that small cells of S. inflata can be confused with S. tabellaria in regard to their valve outline. Geitler (Citation1932) showed that in pennate diatoms length reduces much more strongly than width during the life cycle, therefore the length-to-width ratios probably cannot be used for taxon separation. On the other hand, we found that landmark-based shape analysis and ontogenetic trajectories of valve shape were useful for clarifying taxa boundaries between sympatric populations of S. inflata and S. tabellaria.
Landmark-based shape analysis produced separation of specimens from Russian and Hungarian populations and type material into two distinct shape groups corresponding to S. inflata and S. tabellaria (). The separation of the two groups was along the first relative warp (RW) capturing the most important portion in shape variation. The first RW represented a dilation gradient in the middle section of the valve. The two taxa were localized within this gradient: S. inflata was positioned in the range of shape varying from conspicuously to slightly inflated mid-section, whereas S. tabellaria was located in the range altered from slightly inflated to not-distinct mid-section. Furthermore, S. inflata and S. tabellaria differed significantly in their ontogenetic trajectories with respect to this shape component (). Staurosira tabellaria had significantly higher slope of the ontogenetic trend than S. inflata. This indicated that valves of S. tabellaria had higher variability in shape which changed with decrease in size from rhombic to elliptical-lanceolate. Despite a larger size range, S. inflata varied less in valve shape and its changes over a decrease in size involved decline in degree of dilation of the mid-valve region. The second RW represented a gradient of shape alteration of the valve ends. Only S. inflata changed in this shape component with decrease in size in such a manner that larger cells have broadly cuneate ends, while smaller ones bear narrowly attenuated apices (). In contrast, S. tabellaria represented shape variability in the valve ends that was not related to valve size. The non-significant relationship between size of S. tabellaria and this shape component might be because there was an insufficient range of valve sizes. For example, Ács et al. (Citation2009) examined the population of S. tabellaria from Lake Balaton and reported length range, 8–37 µm, which is longer than the range in the Russian population we studied, 11–23 µm.
Apart from quantitative characterization of valve shape, ultrastructural examination of sympatric populations from north-western Russia under SEM showed that S. inflata may be distinguished from S. tabellaria by the structure of apical pore fields. As mentioned above, S. inflata has very small pore fields composed of few poroids (, ). In contrast, S. tabellaria possesses rather well-developed pore fields with approximately four rows of poroids, and 5–8 poroids per row (). However, it seems that the degree of development of apical pore fields in S. tabellaria may vary from one region to another and cannot be used as a reliable diagnostic feature, since S. tabellaria from south-eastern England in the Van Heurck collection possessed reduced apical pore fields (Morales et al., Citation2015), while S. tabellaria from Hungary (Lake Balaton) in the Pantocsek collection had well-developed pore fields (Ács et al., Citation2009).
Ecology and distribution of Staurosira inflata
According to Krammer & Lange-Bertalot (Citation2000), the ecology of S. inflata is difficult to determine with certainty owing to possible identification errors in the available literature. The same authors state that the taxon is likely to be cosmopolitan, more common in larger lakes as reported previously by Hustedt (Citation1931), euryhaline, perhaps even mesohalobe as reported by Hustedt (Citation1939), common in brackish habitats as reported by Brockmann (Citation1950), and classified as a brackish/freshwater tychoplankton species by Vos & de Wolf (Citation1993). In Lake Ladoga, S. inflata was first recorded (as Fragilaria inflata (Heiden) Hustedt, nom illeg., non Pantocsek) in surface sediments from the southern coast where it was widely distributed at the end of the 1950s and commonly encountered as codominant with Tabellaria fenestrata (Lyngbye) Kützing, S. construens, Staurosirella pinnata (Ehrenberg) D.M. Williams & Round and Achnanthidium minutissimum (Kützing) Czarnecki (Davydova, Citation1968). Currently the distribution of S. inflata is tightly restricted to a few southern coast localities, mainly in Volkhov Bay which is the most eutrophic and polluted embayment of the Lake Ladoga. In our study, S. inflata was observed in periphyton samples at a very low relative abundance (no more than 1–2%). Periphytic diatom assemblages were dominated by A. minutissimum, Fragilaria gracilis Østrup, Eunotia minor (Kützing) Grunow, Cocconeis placentula Ehrenberg, Navicula cryptocephala Kützing, and Nitzschia fonticola (Grunow) Grunow. Sampling sites were characterized by mesotrophic and eutrophic conditions with elevated TP concentration (37–156 µg l–1), low conductivity (90–224 μS cm–1) and neutral pH (6.7–7.3).
Based on the results of the shape analysis of recent sympatric populations and the type material of S. inflata and S. tabellaria, we can conclude that these two taxa should be considered as taxonomically distinct species. The recognition of S. inflata and S. tabellaria as separate species is justified by a gap in the distribution of these taxa in the multidimensional morphospace and their distinct ontogenetic trajectories. Overall, our results demonstrate that the semilandmark-based geometric morphometrics is sensitive enough to distinguish between species when traditional morphometric parameters such as length, width, length-to-width ratio and striae density are not able to discriminate between them with confidence. In addition, our results showed that ontogenetic allometric trends can be a very useful diagnostic tool to distinguish between morphologically similar species.
Acknowledgements
We thank two anonymous reviewers for their constructive comments. We would like to express our gratitude to Dr Regine Jahn and Wolf-Henning Kusber of the Botanic Garden and Botanical Museum Berlin-Dahlem and Dr Nina Lundholm, Dr Ruth Nielsen and Dr Olof Ryding of the Botanical Garden and Museum of the University of Copenhagen for providing type material and permanent preparations, and Dr Jani Heino of Finnish Environmental Institute for help in obtaining of type material.
Disclosure statement
No potential conflict of interest was reported by the authors.
Additional information
Funding
Notes on contributors
Alexander G. Rusanov
A.G. Rusanov: original concept, sampling, data analysis and interpretation, drafting, writing and editing manuscript; L. Ector: editing manuscript; E.A. Morales: editing manuscript; K.T. Kiss: electron microscopy, editing manuscript; É. Ács: original concept, electron microscopy, drafting and editing manuscript.
References
- Ács, É., Morales, E.A., Kiss, K.T., Bolla, B., Plenković-Moraj, A., Reskóné, M.N. & Ector, L. (2009). Staurosira grigorszkyi nom. nov. (Bacillariophyceae) an araphid diatom from Lake Balaton, Hungary, with notes on Fragilaria hungarica Pantocsek. Nova Hedwigia, 89: 469−483.
- Anonymous (1975). Proposals for a standardization of diatom terminology and diagnoses. Beihefte zur Nova Hedwigia, 53: 323−354.
- Balonov, I.M. (1975). Podgotovka diatomovykh i zolotiskykh vodoroslej k elektronnoj mikroskopii [Preparation of diatoms and golden algae for electron microscopy]. In Metodika izucheniya biogeocenozov vnutrennikh vodoemov (Mordukhai-Boltovskoi, F.D., editor), 87−90. Nauka, Moscow [in Russian].
- Bookstein, F.L. (1991). Morphometric Tools for Landmark Data. Geometry and Biology. Cambridge University Press, Cambridge.
- Bookstein, F.L. (1997). Landmark methods for forms without landmarks: morphometrics of group differences in outline shape. Medical Image Analysis, 1: 225−243.
- Brockmann, C. (1950). Die Watt-Diatomeen der schleswig-holsteinischen Westküste. Abhandlungen der Senckenbergischen Naturforschenden Gesellschaft, 478: 1−26.
- CEN – European Committee for Standarization (2003). Water quality – Guidance standard for the routine sampling and pretreatment of benthic diatoms from rivers. – European Standard EN 13946. European Committee for Standardization, Brussels, 14 pp.
- Chudaev, D.A. & Gololobova, M.A. (2012). Frustule morphology of species of the genus Staurosira sensu stricto (Bacillariophyceae) from the Lake Glubokoe (Moscow Region). Novosti Sistematiki Nizshikh Rastenii [Novitates Systematicae Plantarum Non Vascularium], 46: 68−84, 5 pls [in Russian].
- Cox, E.J. (1995). Studies on the diatom genus Navicula Bory. VII. The identity and typification of Navicula gregaria Donkin, N. cryptocephala Kütz. and related taxa. Diatom Research, 10: 91−111.
- Davydova, N.N. (1968). Sostav i usloviya formirovaniya diatomovykh kompleksov v poverkhnostnom sloe donnykh otlozhenij Ladozhskogo ozera [Composition and condition of formation of diatom assemblages in surface sediments of Lake Ladoga]. In Rastitel’nye resursy Ladozhskogo ozera (Raspopov, I.M., editor), 131−174. Leningrad State University, Leningrad [in Russian].
- Edgar, R.K., Saleh, A.I. & Edgar, S.M. (2015). A morphometric diagnosis using continuous characters of Pinnunavis edkuensis, sp. nov. (Bacillariophyta: Bacillariophyceae), a brackish-marine species from Egypt. Phytotaxa, 212: 1−56.
- Edgar, S.M & Theriot, E.C. (2004). Phylogeny of Aulacoseira (Bacillariophyta) based on molecules and morphology. Journal of Phycology, 40: 772−788.
- English, J.D. & Potapova, M.G. (2012). Ontogenetic and interspecific valve shape variation in the Pinnatae group of the genus Surirella and the description of S. lacrimula sp. nov. Diatom Research, 27: 9−27.
- Geitler, L. (1932). Der Formwechsel der pennaten Diatomeen (Kieselalgen). Archiv für Protistenkunde, 78: 1−226.
- Heiden, H. (1900). Diatomeen des Conventer Sees bei Doberan von der Litorina bis zur Jetztzeit. Mittheilungen aus der Grossherzoglich Mecklenburgischen Geologischen Landesanstalt, 10: 1−32.
- Hustedt, F. (1931). Die Kieselalgen Deutschlands, Österreichs und der Schweiz unter Berücksichtigung der übrigen Länder Europas sowie der angrenzenden Meeresgebiete. In Kryptogamen Flora von Deutschland, Österreich und der Schweiz (Rabenhorst, L., editor), Band 7, Teil 2, Lief. 1. Akademische Verlagsgesellschaft m.b.h., Leipzig.
- Hustedt, F. (1939). Die Diatomeenflora des Küstengebietes der Nordsee vom Dollart bis zur Elbemündung. I. Die Diatomeenflora in den Sedimenten der unteren Ems sowie auf den Watten in der Leybucht, des Memmert und bei der Insel Juist. Abhandlungen herausgegeben vom naturwissenschaftlichen Vereine zu Bremen, 31: 571−677.
- Krammer, K. & Lange-Bertalot, H. (2000). Bacillariophyceae. 2/3. Teil: Centrales, Fragilariaceae, Eunotiaceae. In Süsswasserflora von Mitteleuropa. 2nd ed. (Ettl, H., Gerloff, J., Heynig, H. & Mollenhauer, D., editors). Spektrum Akademischer Verlag, Heidelberg, 599 pp.
- Kulichová, J. & Fialová, M. (2016). Correspondence between morphology and ecology: morphological variation of the Frustulia crassinervia-saxonica species complex (Bacillariophyta) reflects the ombro-minerotrophic gradient. Cryptogamie, Algologie, 37: 15−28.
- Kulikovskiy, M.S., Genkal, S.I. & Mikheyeva, T.M. (2011). New data on Bacillariophyta of Belarus. 2. Fam. Fragilariaceae (Kütz.) De Tony, Diatomaceae Dumort. and Tabellariaceae F. Schütt. Algologia, 21: 357−373, 13 pls [in Russian].
- Leuduger Fortmorel, G. & Petit, P. (1878). Des gisements siliceux fossiles de l’Auvergne employés à la préparation de la dynamite (1). Journal de Micrographie, 2: 173−180.
- Mann, D.G. (1984). An ontogenetic approach to diatom systematics. In Proceedings of the Seventh International Diatom Symposium Philadelphia, August 22–27,1982 (Mann, D.G., editor), 113−144. O. Koeltz, Koenigstein.
- Mann, D.G. (1994). The origins of shape and form in diatoms: the interplay between morphogenetic studies and systematics. In Shape and Form in Plants and Fungi (Ingram, D.S. & Hudson, A.J., editors), 17−38. Academic Press, London.
- Mann, D.G. & Chepurnov, V.A. (2005). Auxosporulation, mating system, and reproductive isolation in Neidium (Bacillariophyta). Phycologia, 44: 335–350.
- Manoylov, K.M., Morales, E.A. & Stoermer, E.F. (2003). Staurosira stevensonii sp. nov. (Bacillariophyta), a new taxon from Florida, USA. European Journal of Phycology, 38: 65−71.
- Morales, E.A. (2006). Staurosira incerta (Bacillariophyceae) a new fragilarioid taxon from freshwater systems in the United States with comments on the structure of girdle bands in Staurosira Ehrenberg and Staurosirella Williams et Round. In Advances in Phycological Studies. Festschrift in Honour of Prof. Dobrina Temniskova-Topalova (Ognjanova-Rumenova, N. & Manoylov, K., editors), 133−145. Pensoft Publishers & University Publishing House, Sofia-Moscow.
- Morales, E.A., Wetzel, C.E., Van de Vijver, B. & Ector, L. (2015). Morphological studies on type material of widely cited araphid diatoms (Bacillariophyta). Phycologia, 54: 455−470.
- Mou, D. & Stoermer, E.F. (1992). Separating Tabellaria (Bacillariophyceae) shape groups based on Fourier descriptors. Journal of Phycology, 28: 386−395.
- Østrup, E. (1910). Danske Diatoméer. C.A. Reitzels Boghandel, Bianco Lunos Bogtrykkeri, Kjøbenhavn, Copenhagen, 323 pp.
- Pantocsek, J. (1902). Kieselalgen oder Bacillarien des Balaton. Resultate der Wissenschaftlichen Erforschung des Balatonsees, herausgegeben von der Balatonsee-Commission der Ungarischen Geographischen Gesellschaft. Bd 2 (2). Commissionsverlag von Ed. Hölzel, Wien.
- Potapova, M.G. & Ponader, K.C. (2004). Two common North American diatoms, Achnanthidium rivulare sp. nov. and A. deflexum (Reimer) Kingston: morphology, ecology and comparison with related species. Diatom Research, 19: 33−57.
- Rioual, P., Morales, E.A., Chu, G., Han, J., Li, D., Liu, J., Liu, Q., Mingram, J. & Ector, L. (2014). Staurosira longwanensis sp. nov., a new araphid diatom (Bacillariophyta) from Northeast China. Fottea, 14: 91−100.
- Rohlf, F.J. (1993). Relative warp analysis and an example of its application to mosquito wings. In Contributions to Morphometrics (Marcus, L.F., Bello, E. & Garcia-Valdecasas, A., editors), 131−159. Museo Nacional de Ciencias Naturales (CSIC), Madrid.
- Rohlf, F.J. (2007). TPS Series. Department of Ecology and Evolution, State University New York at Stony Brook, New York.
- Rohlf, F.J. & Slice, D. (1990). Extensions of the Procrustes method for the optimal superimposition of landmarks. Systematic Zoology, 39: 40−59.
- Ross, R., Cox, E.J., Karayeva, N.I., Mann, D.G., Paddock, T.B.B., Simonsen, R. & Sims, P.A. (1979). An amended terminology for the siliceous components of the diatom cell. Nova Hedwigia, Beiheft, 64: 513−533.
- Round, F.E., Crawford, R.M. & Mann, D.G. (1990). The Diatoms. Biology and Morphology of the Genera. Cambridge University Press, Cambridge.
- Schulz, P. (1926). Die Kieselalgen der Danziger Bucht mit Einschluss derjenigen aus glazialen und postglazialen Sedimenten. Botanisches Archiv, 13: 149−327.
- Smith, W. (1856). Synopsis of the British Diatomaceae. Vol. 2. John Van Voorst, London.
- Stoermer, E.F. & Ladewski, T.B. (1982). Quantitative analysis of shape variation in type and modern populations of Gomphoneis herculeana. Beiheft zur Nova Hedvigia, 73: 347−386.
- Stoermer, E.F., Qi, Y.-Z. & Ladewski, T.B. (1986). A quantitative investigation of shape variation in Didymosphenia (Lyngbye) M. Schmidt (Bacillariophyta). Phycologia, 25: 494−502.
- Theriot E. (1988). An empirically based model of variation in rotational elements in centric diatoms with comments on ratios in phycology. Journal of Phycology, 24: 400−407.
- Tuji, A. & Williams, D.M. 2006. Typification of Conferva pectinalis O. F. Müll. (Bacillariophyceae) and the identity of the type of an alleged synonym, Fragilaria capucina Desm. Taxon, 55: 193−199.
- Vos, P.C. & de Wolf, H. (1993). Diatoms as a tool for reconstructing sedimentary environments in coastal wetlands; methodological aspects. Hydrobiologia, 269/270: 285−296.
- Williams, D.M. & Round, F.E. (1987). Revision of the genus Fragilaria. Diatom Research, 2: 267−288.