ABSTRACT
Red snow caused by dinoflagellates is a phenomenon rarely reported, described from the European Alps from 1914 onwards, and subsequently observed outside Europe on several occasions in Ontario, Canada. Considerable taxonomic confusion exists regarding the identity of the organism(s) causing red snow, but the most recent occurrence in 2016 in Ontario has now allowed detailed studies, including LM, SEM, TEM and molecular sequencing of the causative species. We conclude that the two species originally described as the cause of red snow, Glenodinium pascheri and Gyrodinium nivale, are synonymous and that the appropriate name for the organism is Borghiella pascheri (syn. Woloszynskia pascheri) as suggested by Moestrup & Calado in the recent volume of the Süsswasserflora. The central part of Borghiella pascheri cells is tomato red and filled with numerous organelles, whose ultrastructure indicates modified chloroplasts. Lack of cultures has prevented chemical characterization of the red pigment. Formation of temporary cysts was common in the samples. Transformation of the motile cells into temporary cysts was followed in detail, and the cysts were shown to be surrounded by the fused inner membranes of the amphiesmal vesicles, which became the cell membrane of the cysts, covered by the fused pellicle precursors. The cell membrane from the motile cell was discarded together with the outer amphiesmal vesicle membrane and the thin thecal plates, and the temporary cysts were therefore not surrounded by any pattern of vesicles. Sexual reproduction resulted in the formation of hypnozygotes. Although the species possessed several unusual features, DNA sequencing showed it to belong to Borghiella. The culture established in 1965 from the Botanical Garden in Göttingen, Germany and generally identified as Woloszynskia pascheri belongs to a separate species of Borghiella, to be described separately.The occurrence of red snow caused by dinoflagellates is discussed.
Introduction
Red snow is usually associated with green algae (e.g. Chlamydomonas nivalis), but reports of dinoflagellates causing red snow or red ice have occurred intermittently in the literature from 1914 onwards (Traunsteiner, Citation1914), initially from Europe (references below) but also from Canada (Gerrath & Nicholls, Citation1974). Two species have been identified as causing red ice or snow: Gyrodinium nivale Lindemann Citation1929 and Glenodinium pascheri Suchlandt Citation1916. The first species has received little subsequent attention, but the latter has had variable taxonomic treatment: Lindemann (Citation1928) renamed it Gyrodinium pascheri (Suchlandt) Lindemann; Schiller (Citation1954) named it as Gymnodinium pascheri (Suchlandt) Schiller and finally it was transferred to Woloszynskia pascheri (Suchlandt) von Stosch (Citation1973).
During the winters and early springs of 1971–2016, extensive patches of red ice occurred in several frozen ponds and ditches in Ontario, Canada. The organism causing the red colour was identified as Woloszynskia pascheri (Nicholls, Citation2017). The species generally occurs as a non-motile stage in the ice, but readily transforms into a flagellated cell when brought to the lab, swimming and multiplying in the melted ice. Small unarmoured dinoflagellates can be difficult to identify and this applied to the present species, which offered rather few morphological features for identification. To solve some of the questions related to its complicated taxonomy, and to study phylogeny and ultrastructure, ice samples were shipped from Ontario to Copenhagen in February 2016. The transfer succeeded and numerous cells were swimming in the sample upon arrival in Copenhagen. Cells were processed for light- and electron microscopy and for molecular sequencing of single cells. Culturing was also attempted but cells failed to grow. Here we present the results and include a discussion of the taxonomy of dinoflagellates causing red snow, the literature of which is unusually confusing. DNA sequences previously deposited in GenBank as Woloszynskia pascheri belong to a different species, which is not red and which was isolated from a pond in the Botanical Garden in Göttingen, Germany in 1965.
Materials and methods
Material studied
A chunk of ice containing high numbers of Borghiella pascheri was mailed to University of Copenhagen. The sample was collected by KHN in February 2016 from a small pond 82 km north-east of Toronto, 6 km west of Cannington, Canada (). For comparison, we used strain CCAC 0075, presently thought to represent Woloszynskia pascheri but not associated with ice, kindly supplied by Barbara Melkonian, Cologne, Germany in 2004.
Light, scanning and transmission electron microscopy
Live cells were observed using a Carl Zeiss Axio Imager.M2 with differential interference contrast and a 63x/1.4 Plan Apochromat oil immersion objective lens. Digital images were captured with Zeiss AxioCam HRc (–) and 506 mono cameras (–). For visualization of chlorophyll autofluorescence, cells were excited with a Zeiss filter set 09 (excitation band pass 450–490 nm and emission 515 nm long pass). VECTASHIELD® Mounting Medium with DAPI (Vector Laboratories, Burlingame, California, USA) was used to stain the nucleus in glutaraldehyde-fixed cells (1% final concentration). Cells were excited with a Zeiss filter set 49 (excitation band pass 365 nm and emission 445 nm).
Figs 2–12. Borghiella pascheri, Nomarski interference microscopy (Figs 2–7) and fluorescence microscopy (Figs 8–12). The central part of the motile cell is filled with a mass of red pigment bodies that obscure other cell details except the nucleus (N) in the epicone (Fig. 4). Figs 2–6 show swimming cells, and the characteristic and species-specific angular shape of the hypocone is visible in several cells, particularly the cell in Fig. 5. Fig. 7 shows the smooth, thick-walled resting cyst (hypnozygote). Figs 8–10 illustrate the network of sausage-shaped chloroplasts in the periphery of the cell. The centrally located pigment bodies characteristic of this species do not fluoresce, indicating that they lack chlorophyll. Figs 11–12. Chloroplast fluorescence in resting cysts left at 4°C for almost one year.
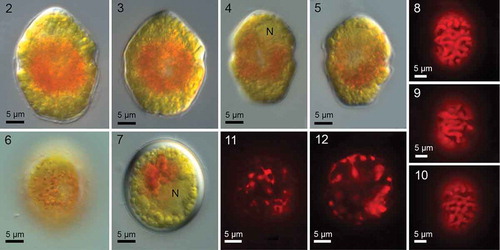
Before fixation for electron microscopy, the sample was left in the cold room (4ºC) for 8 days (16:8 h L:D), by which time numerous motile vegetative cells and planozygotes were actively swimming.
A 400 µl sample was mixed with a 400 µl fixation cocktail, made by mixing 100 µl saturated HgCl2 solution, 150 µl 4% osmium tetroxide in de-ionized water and 150 µl de-ionized water. The sample was fixed for 15 min at room temperature, transferred to an injection tube mounted on a Swinnex holder (containing a 8 µm membrane Millipore filter) and dehydrated in an ethanol series of 30, 50, 70 and 90% ethanol (c. 20 min in each change), followed by 99% ethanol (25 min). The injection tube was then unscrewed and the Swinnex holder rapidly transferred to a beaker with absolute ethanol. It was left in the covered beaker overnight and critical-point dried the following morning in a Bal-Tec CPD 030 freeze drier (Bal-Tec AG, Balzers, Liechtenstein). The dried filters were glued to metal stubs, sputter coated with gold-palladium in a Jeol JFC-2300 HR sputter (Jeol Ltd, Tokyo, Japan) and examined in a Jeol JSM field emission scanning electron microscope operated at 7 kV.
Another batch of material was fixed and embedded at the same time for transmission electron microscopy. Five ml were mixed with 5 ml cold 6% glutaraldehyde in 0.15 M phosphate buffer at pH 7.2. The mixture was left in the fridge for 2 h before centrifugation at 2000 rpm (769 × g), which resulted in the formation of a pellet of cells in the bottom of the centrifuge tube. The pellet was retained during the following steps, which included rinsing in three changes of cold buffer over 1 h, post-osmication in cold 2% osmium tetroxide in water for 2.5 h, a brief rinse in cold buffer, and dehydration in an ethanol series, 20–25 min in each change of 30, 50, 70 and 90% cold ethanol. The tube with the material was then removed from the fridge, and dehydration was completed at room temperature: 30 min in absolute ethanol from a solution containing molecular sieves (2 changes), followed by 10 min in propylene oxide (2 changes). The material was left overnight in a 1:1 mixture of propylene oxide and Epon 812 in a fume hood to allow evaporation of the propylene oxide. The cocktail was replaced with Epon 812, left for 3.5 h in the fume hood, and the pellet was finally removed from the centrifuge tube with a pipette and transferred to Epon 812 in a polymerization mould. It was polymerized at 60°C overnight.
The polymerized block was trimmed and sectioned using a Leica Super Nova ultramicrotome (Leica Microsystems GmbH, Wetzlar, Germany). The sections (50–60 nm in thickness) were collected on slot grids and stained at room temperature for 10 min in 2% uranyl acetate, followed by 10 min in Reynold’s lead citrate. They were examined in a Jeol-1010 transmission electron microscope (Jeol Ltd, Tokyo, Japan), working at 80 kV, and photographed using a Gatan Orius digital camera (Gatan Inc., Pleasanton, California, USA).
Cell disruption and DNA extraction
Single cells of B. pascheri were isolated using capillary pipettes and transferred to 0.2 ml thin-walled tubes. Prior to PCR, cells were treated with glass beads as described in Boutrup et al. (Citation2016). A 10 ml sample of exponentially growing CCAC 0075 was centrifuged at 1500 rpm for 10 min. The pellet was transferred to a 1.5 ml Eppendorf tube and kept frozen at −18ºC until extraction of total genomic DNA. DNA extraction followed Doyle & Doyle (Citation1987) but with modifications as outlined in Hansen et al. (Citation2003).
PCR amplification conditions and sequencing
PCR conditions and sequencing of the nuclear-encoded LSU rDNA gene have been described previously (Hansen & Daugbjerg, Citation2011). The LSU rDNA sequence of Borghiella pascheri and strain CCAC 0075 was determined in both directions. To test for reproducibility, the LSU rDNA sequence of CCAC 0075 was determined independently from two individual cells. As the sequences of these cells were identical only one was used in the phylogenetic analyses.
Alignment and phylogenetic analyses
The LSU rDNA sequence of Borghiella pascheri was added to the alignment used by Daugbjerg et al. (Citation2014) when describing Borghiella andersenii. Hence, the alignment used here comprised all Borghiella species for which LSU rDNA sequences are available.
Sequence divergence
PAUP (ver. 4.0a152; Swofford, Citation2003) was used to estimate sequence divergence in per cent between four species of Borghiella, strain CCAC0075 and Baldinia anauniensis.
Results
Light microscopy
Motile cells were 27.4 ± 2.3 µm (24.4–32.6 µm) long and 21.5 ± 2.2 µm (17.8–26.3 µm) wide (n=21) (–). The epicone was rounded conical, and usually slightly smaller than the hypocone, which was characteristically angular (–). Cells were dorsoventrally flattened, most pronounced on the hypocone (shown better in SEM, see below). The appearance of the cell was dominated by the large central area of red pigmentation (–, ), which obscured observation of details such as the eyespot (see below) and the cingular displacement. The chloroplasts were located mainly in the periphery of the cell, and appeared as single units or a continuous network (–). When the material arrived in Denmark, it was dominated by planozygotes, and red, smooth ovoid–oval resting cysts soon formed (); these were still present in the cold room in the glass container and showing autofluorescence (, ) one year after the material arrived from Canada. Cyst measurements: length 28.3 ± 1.5 µm (25.7–30.5 µm) and width 25.1 ± 1.6 µm (22.2–27.7 µm) (n=13).
Temporary cysts, the dominant stage in the ice, were not studied here, but were measured by Nicholls (Citation2017) as 19–35 µm long and 16–26 µm wide. In other words, motile cells, temporary cysts and resting cysts had very similar dimensions.
Scanning electron microscopy
The scanning electron microscope showed the cell to be covered by numerous amphiesmal vesicles, whose rims were usually indicated by small droplets (–). However, the arrangement and number of vesicles on the epi- and hypocone were somewhat variable. Typically, the epicone amphiesma comprised 4–5 (6) latitudinal series of amphiesmal vesicles, each vesicle pentagonal, or sometimes 4- or 6-sided (–). The epicone vesicles lining the cingulum formed a well-marked line (–).
Figs 13–18. Borghiella pascheri, SEM. Figs 13, 14 cells in ventral view, Figs 15, 16 cells in dorsal view (vr = ventral ridge, tf and lf = transverse and longitudinal flagellum, respectively). The arrow indicates the sometimes slightly pronounced left edge of the sulcus. Figs 17, 18 show the apical furrow, seen from the ventral side of the cell, the asterisks indicate the amphiesmal vesicles lining the apical furrow.
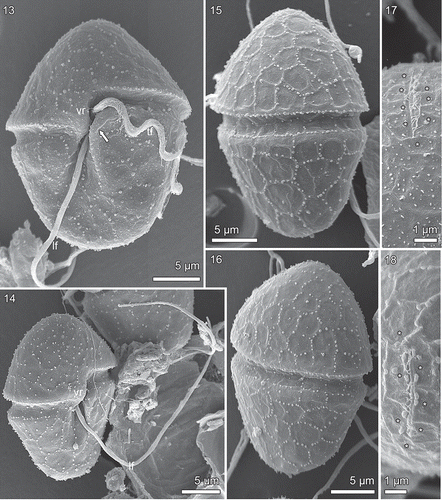
In dorsal view, the hypocone commonly showed c. 4–6 horizontal rows of amphiesmal vesicles (–). The postcingular row appeared to be distinct, but the postcingular row of plates actually curved over the posterior cingulum rim into the cingulum ().
A short (4–5 µm), straight apical furrow extended over the apex of the cell (–). On the ventral side of the cell, it was directed obliquely to the right of the sulcus, rather than towards the confluence point of sulcus and cingulum (). The furrow comprised two parallel vesicles but details were difficult to see in the SEM. However, a row of fibrillar structures, probably mucilaginous, extended from the left side of the apical furrow complex (the left vesicle), and a shallow furrow was present on the right side of the row of fibrils (). The pair of vesicles was lined on each side by 3–4 larger amphiesmal vesicles (, , ).
Figs 19–21. Planozygotes of Borghiella pascheri, SEM. Fig. 19. Planozygote with its two markedly long longitudinal flagella (lf). Fig. 20. Planozygote in which the apical furrow has been marked by arrows. Fig. 21. Planozygote in lateral view, showing the flat ventral side of the hypocone. lf and tf = longitudinal and transverse flagellum, respectively.
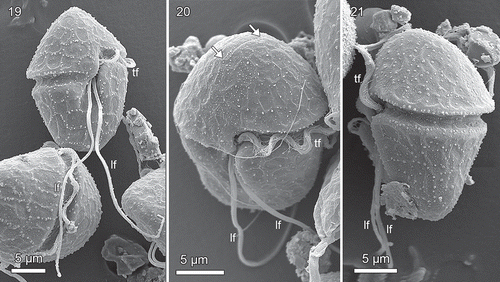
The cingulum was well marked, its anterior edge more sharply defined than the posterior edge (–). The left flange of the sulcus often appeared slightly swollen (, arrow). The amphiesmal vesicles of the cingulum could not be seen in detail in the SEM, but were readily identified in the thin sections (see below), one forming the roof of the cingulum, the second forming most of the cingulum floor, while the vesicles of the third row bent over the rim of the cingulum to continue onto the hypocone (compare with ). The cingulum ends were displaced by c. 1.5 cingulum widths (, , ). The sulcus did not extend onto the epicone. On the hypocone it continued almost to the antapex, becoming less pronounced at the antapical end of the hypocone (, ). The longitudinal flagellum was notably long, in c. 45 µm long, in the planozygote in c. 35 µm long, in fact longer than the cell length and extending as far as 20 µm behind the cell ().
Figs 22–24. Borghiella pascheri, TEM. Fig. 22. Longitudinal section through the cell showing the main organelles, the cingulum (c), sulcus (s), nucleus (N) in the epicone, profiles of chloroplasts (chl) in the periphery of the cell, and the centrally located pigment bodies (p). Fig. 23. Transverse section though the epicone. The cell is somewhat flattened dorsoventrally; the nucleus (N) is located on the right side of the figure along the dorsal side of the cell. Several relatively large lipid bodies (l) are present in the area between the chloroplasts (chl) and the nucleus. Fig. 24. Transverse section through the hypocone, which is strongly flattened dorsoventrally. The section illustrates the sulcus (s), with the eyespot (e, barely visible at this magnification), the central group of pigment bodies (p), starch grains (st) and chloroplasts (chl) in the outer part of the cell.
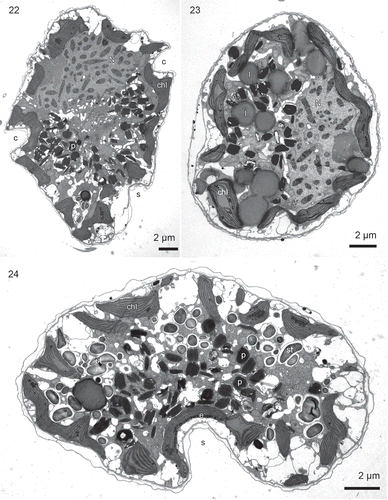
Figs 25–28. The red pigment bodies of Borghiella pascheri, TEM. Fig. 25. Transverse section of the cell showing the central part occupied by numerous pigment bodies (p). The cell periphery is occupied by the chloroplasts (chl). Starch grains (st) also abound. Fig. 26. Pigment body at higher magnification. Each body is lined by a membrane and the internal part comprises a stack of membranes, in this case with thylakoid-like structures stacked in threes like chloroplast lamella (arrow). Various pieces of membrane-like material are also present in the vesicle. Fig. 27. Thin section through the central group of pigment bodies (p). The inner part of each body is taken up by parallel thylakoid-like membranes, sometimes single (single arrow), sometimes separate but still parallel (two arrows), sometimes close together (three arrows). cDNA, probably chloroplast DNA, m = mitochondrion. Fig. 28. The original drawing of Glenodinium pascheri by Suchlandt (Citation1916) of material from the European Alps, illustrating cell shape and the many pigment bodies (drawn rod shaped) that occupy the central part of the cell.
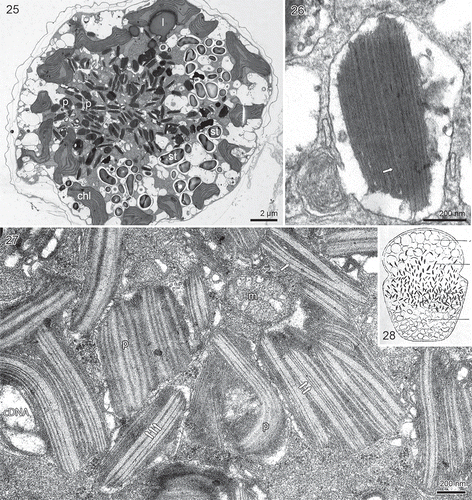
Figs 29–31. Borghiella pascheri, TEM. Fig. 29. The cingulum (c) is lined by three amphiesmal vesicles, two confined to the cingulum (I, II) and one curving over the posterior rim of the cingulum to the hypocone (III). Each amphiesmal vesicle contains two plate-like structures (arrows). Fig. 30. The sulcus region (s) contains the eyespot, formed by a layer of pigment globules (e) within a chloroplast (chl). On the outside of the eyespot is a row of vesicles containing brick-like material, thus confirming that the eyespot belongs to Type B sensu Moestrup & Daugbjerg (Citation2007). Cytoskeletal microtubules may be seen in the area between the vesicles and the amphiesma, and some of these represent flagellar root r1. m = mitochondrion, p = pigment bodies. Fig. 31. The brick-like material (arrows) near the eyespot globules (e) in more detail. chl = chloroplast.
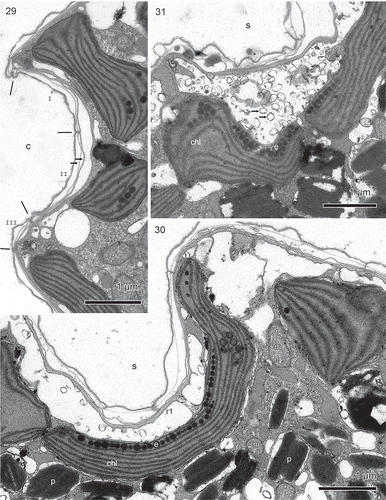
A few pores were observed in the amphiesma (), see Discussion for further information.
Planozygotes are illustrated in –. The only difference noted between flagellated single cells and the numerous planozygotes was the presence of two longitudinal flagella of the planozygotes (–). Somewhat unexpectedly, we did not notice planozygotes to be larger and covered by more amphiesmal vesicles than the single cells (detailed measurements were not made, however).
Transmission electron microscopy
, show the cell at low magnification. The epicone was only slightly dorsoventrally flattened (), in contrast to the hypocone, which was strongly flattened (). The nucleus was located mainly in the epicone () along the dorsal side of the cell (). Chloroplast profiles were visible along the entire periphery of the cell (–). An eyespot, not noticed in previous studies and not visible to us using the light microscope, was nevertheless present in the sulcal region (, , ), see further below. The entire central part of the cell was taken up by vesicles (–), responsible for giving the cells their red colour (compare with ). The cell also contained starch grains and a number of lipid globlets (–). Trichocysts were absent.
The red bodies were c. 1 µm long and contained numerous membranes. In some cases a stack of membranes was visible (), and thylakoid-like triplets were occasionally visible within the stack (). In other cases the stack(s) of thylakoid-like structures had broken up, resulting in many parallel, single or paired structures (). We interpret electron-translucent areas to represent the nucleoids ().
The eyespot belonged to type B sensu Moestrup & Daugbjerg (Citation2007). The sulcus was lined on the inside by a half circle (in transverse sections of the cell) of pigment globules of the eyespot located in a chloroplast near the sulcus (, ). The area between the sulcus cell membrane and the eyespot was occupied by a row of vesicles, each containing what in other species has been termed brick-like material (, ). These vesicles were often somewhat swollen, probably an artefact introduced during processing for electron microscopy (, ). The amphiesmal vesicles were underlined by sheets of microtubules, which almost certainly contained also the microtubular flagellar root R1, which typically occupies this position in dinoflagellates (, ).
The apical furrow was rarely seen in the thin sections and although details may be difficult to discern in the figure, shows two narrow apical furrow vesicles, subtended by microtubules. The vesicle on the right is subtended by two somewhat separated microtubules, the one on the left by two microtubules located close together. This resembles the situation in B. dodgei, in which the apical furrow vesicle is subtended by two separate microtubules, the other vesicle, the knob vesicle, by 2–3 microtubules located close together.
Figs 32–35. Borghiella pascheri, TEM. Fig. 32. Thin section through the apical furrow, which comprises two narrow amphiesmal vesicles (arrows), underlain by a group of 1+1+2 microtubules. The vesicle on the right is the furrow vesicle. pp = pellicle precursor, t = thecal plate. Figs 33–35. Details of the amphiesma at higher magnification. Fig. 33. Amphiesmal vesicle containing thin but typical thecal plate (t), while the inner component of the vesicle has coiled up (pp). The tri-layered construction of the inner component, the pellicle precursor (pp), is very distinct. Fig. 34. High magnification showing the cell membrane (cm), and two amphiesmal vesicles (av), lined by the outer (oa) and the inner (ia) amphiesmal membrane. A thin thecal plate (t) is located within each amphiesmal vesicle, together with the underlying pellicle precursor (pp). The two pellicle precursors are very close together and would soon have started fusing with each other, in preparation for the formation of a temporary cyst. The inner membranes of each amphiesmal vesicle are likewise very close, probably fusing or fused. Fig. 35. Formation of a temporary cyst. The cell discards the original cell membrane (cm), the outer amphiesmal vesicle membranes (oa) and the thecal plates (t), but retains the pellicle precursors (pp) which fuse to become the pellicle. The inner amphiesmal membranes likewise fused, to become the cell’s new cell membrane. Labelling as in Fig. 34.
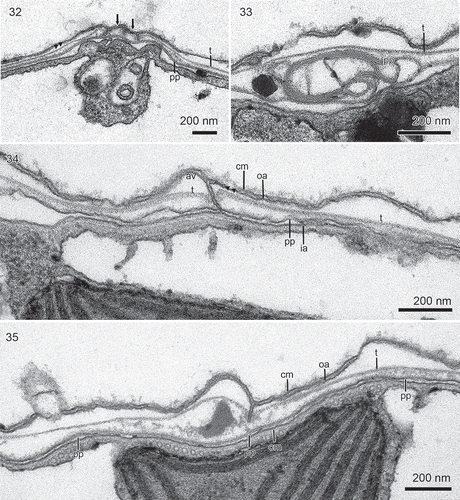
The amphiesmal vesicles, formation of temporary cysts
Cells of B. pascheri are lined along the periphery by the amphiesma, each amphiesmal vesicle containing two components: an outer component without any visible structure and an inner component, which from some angles is distinctly three layered. shows a case in which part of the inner component is irregularly folded and its three-layered structure is very distinct. The structure is morphologically different from the structure of cell membranes such as those lining the amphiesmal vesicles. The inner component we will term the pellicle precursor (pp). As Borghiella pascheri readily forms temporary cysts in the ice-water interface, the changes that occur in the ultrastructure of the amphiesma during this process were often visible in our material (–). When formation of temporary cysts begins, the amphiesmal vesicles are closely aligned and located close together (). The amphiesmal vesicles then break up and all the inner amphiesmal membranes start fusing with each other. The pellicle precursors, no longer confined to the vesicles, likewise make contact with each other and start fusing. The remaining part of the amphiesma, i.e. the old cell membrane and the outer amphiesmal membranes plus the thecal plates now break away from the cell and are discarded (). Once the process is complete, all the inner amphiesmal membranes have fused to become the new cell membrane and the pellicle precursors have fused to become the pellicle. At some angles of sectioning, the three-layered structure of the pellicle was still clearly visible (, pp), while at other angles the pellicle was very similar to and could not be distinguished from the underlying, new cell membrane (, left and right). Having discarded the thecal plates, the temporary cysts were without amphiesmal vesicles and lined only by the new cell membrane and a wall formed by the pellicle.
An unusual structure took the form of worm-like bodies, sometimes several together (–). Each body was surrounded by a single membrane but lined on the outside by vesicles (). This recalls the structure of the pusule in other species of dinoflagellates. Several profiles of more typical pusule canals were often visible near the worm-like structures (), indicating that the two structures are both components of the pusule system.
Figs 36–38. Borghiella pascheri. Fig. 36. The pusule region which in addition to typical pusule canals contains elongate, snake- or tube-like components, believed to be part of the pusule system. Fig. 37. A snake-like component measuring 2 µm in length. Fig. 38. Both the pusule canals (pus) and the snake-like components are lined by vesicles. m = mitochondrion, cDNA = chloroplast DNA, p = pigment body.
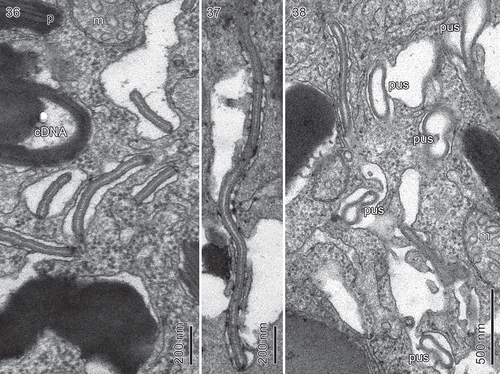
Phylogeny
Based on LSU rDNA sequences the genus Borghiella was monophyletic and highly supported by posterior probability (pp = 1.0) and bootstrap (BS = 100%) (). Borghiella spp. formed a sister group to a lineage comprising Baldinia anauniensis and Polarella/Biecheleriopsis/Biecheleria/Symbiodinium/Protodinium. The relationship between these dinoflagellates was highly supported by pp=1.0 and BS=86%. Among the Borghiella species B. pascheri formed a close relationship with B. dodgei, highly supported by pp=1.0 and BS = 95%. Borghiella tenuissima formed a sister taxon to B. pascheri/B. dodgei but this relationship received less support (pp=0.78 and BS=58%). Strain CCAC 0075, previously identified as Woloszynskia pascheri, clustered within the species of Borghiella. It did not form a sister taxon with B. pascheri but rather was part of an unresolved trifurcation with B. andersenii and B. dodgei/B. pascheri/B. tenuissima ().
Fig. 39. Phylogeny of Borghiella pascheri (boldface) based on nuclear-encoded partial LSU rDNA (1574 base pairs including introduced gaps) and inferred from Bayesian analysis. The ingroup of dinoflagellates (46 genera and 75 species) was polarized using ciliates (3 taxa), apicomplexans (4 taxa) and Perkinsus. Posterior probabilities (≥0.5) from Bayesian analysis and bootstrap values (≥50%) from maximum likelihood analyses with 1000 replications are shown at internodes. Posterior probabilities <0.5 and bootstrap values <50% are indicated by –. GenBank accession numbers are provided in parentheses following species names. The genus Borghiella is indicated and the branch lengths are proportional to the number of character changes.
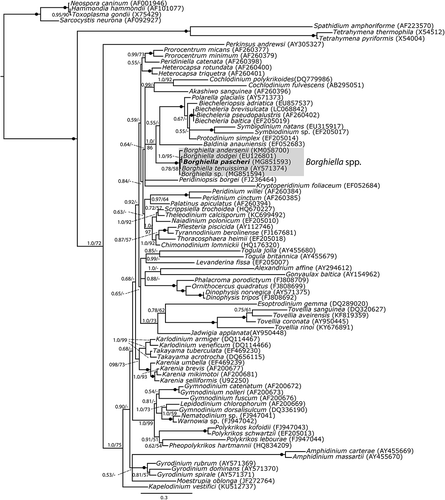
Sequence divergence
The sequence divergence between Baldinia anauniensis and species of Borghiella varied between 14.1 and 14.4% (uncorrected p distances) and between 15.8 and 16.0% (absolute distances) (). Within the genus Borghiella the smallest sequence divergence was between B. dodgei and B. pascheri (0.3% using both uncorrected ‘p’ and absolute distances) and the largest divergence was between B. andersenii and B. dodgei (2.0% using both uncorrected ‘p’ and absolute distances) ().
Table 1. Sequence divergence (in percentage) between species of Baldinia and Borghiella based on 1336 base pairs of the LSU rDNA gene. Estimates of sequence divergence were based on uncorrected (‘p’) (above diagonal) and absolute distances (below diagonal) using PAUP*.
Discussion
‘Red snow’ is usually due to volvocalean green algae living on and in the snow, and as such the phenomenon has been reported from most if not all continents. However, red snow due to dinoflagellates is rare and has presently been described only from Canada, Austria and Switzerland (see Nicholls, Citation2017, for references). In addition, we have unpublished information from Portugal, where red snow caused by a dinoflagellate was seen on 11 April 2014, covering the ice on a pond at Lagoas das Salgadeiras, at c. 1795 m altitude in the Serra da Estrela, Portugal (Mariana Pandeirada, personal communication to ØM). The dinoflagellate causing this red snow was photographed but could not be cultured, and its identity therefore needs to be confirmed. Morphologically, the Canadian material examined by us is identical to the Central European material described 100 years ago, indicating conspecificity of the materials on the two continents. A phylogenetic analysis of material from the European Alps is needed to determine whether any difference exists in the DNA sequences. As the cells are present in/on the ice as temporary cysts or as thick-walled resting cysts produced by sexual reproduction, these are more resistant than flagellated cells and it is therefore not unlikely that the species can/has spread over large distances by wind and/or water. It should be sought in the mountains of South America, Africa and Asia. The most characteristic feature of Glenodinium pascheri, when first described from Switzerland, is the flattened antapex, a feature not seen in the other species of Borghiella, illustrated first by Suchlandt (Citation1916, and reproduced here as ) and in the Canadian material (Nicholls, Citation2017, his ) and in our – and in particular in . Morphologically the Canadian and European materials also agreed in the concentration of the red bodies in the central part of the cell, a feature drawn by both Traunsteiner (Citation1914, figs I–IV) and Suchlandt (Citation1916, figs A–F). These authors mention that the epicone was slightly smaller than the hypocone, a feature we also recognized in our material, although the size difference was small. Regarding size, Traunsteiner (Citation1914) gave cell length of motile cells as 30–40 µm, Suchlandt (Citation1916) as 26 µm and Lindemann (Citation1929) as 18–33 µm, and in our material 24–33 µm. We observed no difference between the Canadian and the European material.
In many respects the ultrastructure of B. pascheri agrees with what has been observed in other species of Borghiella, B. dodgei and B. andersenii (Moestrup et al., Citation2008; Daugbjerg et al., Citation2014, respectively). Thus, the amphiesmal vesicles of all three species contain two different components, an outer, more or less even, plate-like component, and an inner, more irregular, but also plate-like structure. The inner component we will term the pellicle precursor. The term pellicle has been used differently by different authors and this has caused considerable confusion. We follow Morrill & Loeblich (1983, p. 158), the pellicle being a continuous, non-membranous layer, formed by fusion of pellicle presursors. The precursors (by Morrill & Loeblich termed osmiophilic material) are located within amphiesmal vesicles. Our observations in Borghiella pascheri support the observations of Morrill & Loeblich (Citation1983). The outer component of the amphiesmal vesicles in Borghiella is probably homologous with the thecal plates in thecate dinoflagellates, while the inner component, the pellicle precursors and the subsequently formed pellicle, is different, both chemically (e.g. Loeblich, Citation1970; Morrill & Loeblich, Citation1981) and morphologically, as shown in the high-magnification section in . As illustrated in , the amphiesmal vesicles in B. pascheri fuse in preparation for formation of temporary cysts, followed by shedding of the cell membrane, the outer amphiesmal membrane and the outer ‘thecal’ plate, while the inner component of the amphiesmal vesicle fuse with those from adjacent vesicles to form the pellicle. The lowermost amphiesmal membranes fuse to become the new cell membrane. In B. andersenii, the amphiesmal vesicle contained a 50 nm thick thecal plate, with two membrane-like structures beneath (Daugbjerg et al., Citation2014, ). The latter appeared to be continuous around the cell, a feature also seen in illustrations of many other dinoflagellates (e.g. in Kryptoperidinium foliaceum, Dodge & Crawford, Citation1970, plate 3F, as Glenodinium foliaceum), and we have come to the conclusion that the lowermost membrane represents the fused lower amphiesmal membranes while the uppermost structure is the pellicle wall. Fusion of the lowermost components readily occurs, also in flagellated cells.
The eyespots of all species of Borghiella examined by TEM are similar, constituting Type B of Moestrup & Daugbjerg (Citation2007), in which the eyespot is located close to the outer membranes of a chloroplast or chloroplast lobe and accompanied on the chloroplast external side by cisternae containing what has been termed brick-like material. An eyespot has not been seen in B. pascheri before, nor could we see it in the light microscope, the whole central area of the cell being reddish and obscuring cell details. It was readily visible in the thin sections studied here.
The apical furrow of the three species appear to be very similar, and it was shown well in B. dodgei to be composed of two narrow, parallel vesicles, supported on the inside by c. 5 microtubules (Moestrup et al., Citation2008, ). In B. pascheri we saw this structure rarely in the sections, but shows the presence of four microtubules beneath the two vesicles of the apical furrow.
Thin sections of the cells showed B. pascheri as lacking trichocysts, nor have trichocysts been reported in the other species of Borghiella examined in detail, B. dodgei (Moestrup et al., Citation2008) and B. andersenii (Daugbjerg et al., Citation2014). Scanning electron microscopy, however, showed the presence of a few pores in the amphiesma (), and the same feature, although not commented on by the authors, is visible in some of the published micrographs of B. dodgei (Moestrup et al., Citation2008, , ) and B. andersenii (Daugbjerg et al., Citation2014, , ). Such pores are unlikely to be trichocyst pores, but we have no suggestions about their nature.
However, in two respects B. pascheri differs, not only from the other species but also from all other dinoflagellates examined so far. The first is the appearance of the flat vesicles illustrated in –, surrounded on both sides by vesicles. They resemble the flattened tubes found in another, not closely related dinoflagellate, Theleodinium calcisporum (Craveiro et al., 2013), in which similar tubes are part of the pusule system. The second unusual feature of B. pascheri is the nature of the red bodies, which occupy most of the central part of the cell. Only a few red dinoflagellate species are known but we have had the opportunity to examine a further three species in detail, Tovellia coronata (Lindberg et al., Citation2005), Tovellia sanguinea (Moestrup et al., Citation2006) and Nottbeckia ochracea (Hansen et al., Citation2018). All three species differ from B. pascheri in the structure of the bodies, which give the cells their red colour. Cells of Tovellia coronata are more or less evenly red throughout, and in the electron microscope they were found to contain numerous scattered globules, resembling and interpreted by Lindberg et al. (Citation2005) as carotenoid globules, which are similar in appearance in the electron microscope. The chemical composition of the globules remains unknown (Lindberg et al., Citation2005). In the second species, Tovellia sanguinea, famous for colouring the water of Lake Tovel in Italy tomato red in summer (e.g. Flaim et al., Citation2004) the pigment commonly formed aggregates in the central part of the cell, and was identified as astaxanthin (Frassanito et al., Citation2006). The same applies to Tovellia diexiensis just described from the edge of the Tibetan Plateau (Zhang et al., Citation2016). The pigment bodies of the recently examined Nottbeckia ochracea, a species known only from a tiny rockpool in the Finnish Archipelago, resemble those of Tovellia sanguinea (Hansen et al., Citation2018) but the chemical nature of the pigments in this species has not been determined. In contrast to all these species, B. pascheri cells possess numerous organelles which accumulate in the central region of the cell and which we interpret to be of chloroplast origin. They are the size of chloroplasts and contain thylakoid-like membranes, but no lipid droplets. They also differ from the chloroplasts by being surrounded by a single membrane. Such a type of organelle is exceptional and indicates an unknown type of pigment. Some lipid droplets were present in the cytoplasm of B. pascheri but they were too few to explain the red colour of the cell. Borghiella pascheri also differs from the other species in being confined to ice and snow, at localities with very high light irradiance. Unfortunately, culturing of B. pascheri was not successful, and examination of the pigments must await availability of material from nature. Pigmented chloroplasts, also known as chromoplasts, occur in vascular plants (illustrated by thin sections for example in Gunning & Steer (Citation1975) in tomato fruits) and in the shield cells (capitulum) of the Chara male organ (Pickett-Heaps, Citation1975). They contain many pigment globules in addition to typical thylakoids, and the chloroplasts are filled up with pigment globules when the shield cells mature and turn red or orange. In green flagellates such as Chlamydomonas nivalis and Chlainomonas sp. the red pigments have been shown to be astaxanthin and astaxanthin derivatives, which in electron micrographs appear as opaque globules in the cell (Remias et al., Citation2016).
It appears probable that the modification of chloroplasts – in addition to the presence of typical chloroplasts in the cell – is a specialization, which has occurred as a response to the move from fresh water (other species of Borghiella) to the special biotope of ice and snow with its very strong light, which necessitates shielding to avoid cell damage.
On the identity of the two red-snow dinoflagellates presently known
As mentioned earlier, red snow caused by a dinoflagellate was first described by Marie Traunsteiner from Kitzbühel in the Austrian Alps in Tyrol in a short, difficult to access German-language note (Traunsteiner, Citation1914). Traunsteiner described brick-red patches of snow in the melting ice of a fishpond in Langau () at the end of February (probably 1914). Cells were said to be 30–40 µm long, the epicone somewhat smaller than the hypocone. Traunsteiner did not provide a name for her material, in which she described young cells to be completely naked while in older cells she noticed a thin cover when cells were treated with Fleming’s reagent, indicating that it was probably a new species of Glenodinium, characterized in particular by its red colour. The cells contained a conspicuous large central red area, while the cell periphery contained greenish chloroplasts or was colourless. Cells divided during swimming, cytokinesis starting at the antapex. They were strongly positively phototropic. Traunsteiner also observed ovoid cysts (temporary cysts). After a few hours at higher temperature, the cysts formed flagellated cells. In old cultures Traunsteiner observed red-green thick-walled cysts, probably hypnozygotes (resting cysts), which she also found in March on plants and pieces of ice in the pond. Traunsteiner sent material to Lindemann in Berlin, who described it as a new species, Gyrodinium nivale Lindemann (Lindemann, Citation1929). Lindemann was able to extend Traunsteiner’s observations and gave the cell length as 18–33 µm. He found the cells to be ovoid but somewhat variable in shape. Characteristic was the strongly dorsoventrally flattened hypocone, which in lateral view gave the cells a conical appearance. The epicone was slightly smaller than the hypocone. Lindemann observed the cingulum to be descending, the ends in long cells displaced c. 1/5 of the cell length. On the left hand side of the sulcus was a swelling. A stigma was not observed.
Only one year following Traunsteiner’s note, in December 1915, Otto Suchlandt found a dinoflagellate forming red snow and ice on the frozen Davos Lake () in Switzerland (Suchlandt, Citation1916) and described the Swiss material as the new species Glenodinium pascheri Suchlandt, honouring Prof. Pascher in Prague, who provided advice. Suchlandt was aware of Traunsteiner’s article but stated that his material was different. It is difficult, however, from Suchlandt’s description to get an understanding of what he thought the difference between the two materials was. Cells were stated by Suchlandt to be 26 µm in length, 20 µm wide, and although this is lower than the numbers given by Traunsteiner, it agrees with the numbers subsequently provided by Lindemann (18–33 µm), using Traunsteiner’s material. The epicone was described by Suchlandt as dorsoventrally flattened, semicircular, and the hypocone as trapezoid. The main difference between Traunsteiner’s and Suchlandt’s material appears to be cell size, as everything else is identical, even in detail. Lindemann followed Suchlandt in believing the two species to be different, and gave as the main difference the path of the cingulum, circular in Gl. pascheri and descending in Gyr. nivale. However, Lindemann stated that it was difficult to observe the details of the cingulum on the ventral side of the cell due to the strong red colour of the central part of the cells, the small cell size and their continuous swimming. Nevertheless, both Traunsteiner and Suchlandt illustrate their cells as having a circular cingulum! Lindemann observed, however, that the material he received from Traunsteiner possessed a descending cingulum. How difficult it can be to see the details on the ventral side of the cell was reinforced recently, when Nicholls (Citation2017) described the new material from Canada in a preliminary way and mentioned that the cingulum had very little displacement. When studying the material in Copenhagen we found it very difficult to see the ventral part of the cell, and we also came to the conclusion that the cingulum was probably more or less circular. However, SEM showed otherwise, the displaced cingulum was obvious, and the displacement amounted to c. 1.5 times the cingulum width. Nicholls (Citation2017) described the epicone as dome-shaped, and the hypocone truncate or rounded, consistently flattened dorsoventrally towards the antapex. This agrees with Traunsteiner, Suchlandt and Lindemann. Our observations using SEM showed some variation in the shape of the epicone, which appeared rounded to slightly conical. We observed no variation in the shape of the hypocone.
The materials from Austria, Switzerland and Canada are morphologically and ecologically identical. Lindemann ignored Marie Traunsteiner’s drawing of the circular cingulum, but not Suchlandt’s very similar drawing of Gl. pascheri! We believe this to be a mistake and conclude that no difference exists between the two materials, Gyr. nivale therefore being a synonym of the 13-year-older Glenodinium pascheri.
In agreement with our interpretation, Schiller (Citation1933) also merged the two species, which he transferred to Gyrodinium, as Gyrodinium pascheri (Suchlandt) Schiller, although he stated that he had not seen Traunsteiner’s article. A few years later Jadwiga Wołoszyńska reported material she identified as belonging to this species from the Polish Tatra Mountains in January–February 1936 (Wołoszyńska, 1937). She provided a detailed description of her material, which agrees with previous observations except for one feature: Wołoszyńska described rapid multiplication of the cells in the non-motile stage, she did not see division in the motile stage. Cells in the latter stage lost the flagella but retained the furrows and became surrounded by a wall. Eventually non-motile cells appeared in Wołoszyńska’s mixed cultures. They lacked furrows and Wołoszyńska considered these to be temporary resting stages. Wołoszyńska collected her material from the plankton, not from ice or snow.
Considering that division of the motile cell has been reported many times, from Traunsteiner (Citation1914) to Nicholls (Citation2017), Woloszynska’s observations are striking and require further study. It seems likely that Wołoszyńska’s plankton dinoflagellate is a different species.
Many problems apply to Schiller’s subsequent reports of algae from ice and snow in Austria (Schiller, Citation1954, Citation1955). Schiller had by then changed his mind and transferred Gyr. pascheri to Gymnodinium, as Gymnodinium pascheri (Suchlandt) Schiller and merged into this name no less than five other Gymnodinium species. Schiller found his material in water and forming yellow-brown, not red, patches on ice and melting snow. The morphology of Schiller’s material agrees in other respects with the red-snow material of Traunsteiner and Suchlandt. Schiller described cell division in the motile stage, and his illustrations agree with those of Traunsteiner (Citation1914) and Nicholls (Citation2017). However, Schiller also describes cell division in the non-motile stage, and in material from a pond in Glinzendorf he describes how cells divide into two and subsequently four in the non-motile stage. This recalls the division of the genus Tovellia, which is morphologically very similar, and Schiller’s subsequent (1955) description of ‘horned cysts’ from Springer Park, Vienna, shows that his material is not Gymnodinium pascheri, but a species of Tovellia. Schiller therefore mixed up at least two species in his often very detailed reports. In conclusion, we are presently aware of only a single dinoflagellate species forming red snow.
On the identity of the culture identified as Woloszynskia pascheri (Suchlandt) von Stosch (CCAC 0075)
von Stosch (Citation1973) did not provide any new information when he transferred Gymnodinium pascheri to Woloszynskia, as W. pascheri (Suchlandt) von Stosch. von Stosch was obviously misled by Schiller’s description of horned cysts in the material from Austria. The same applies to Kusel-Fetzmann’s (Citation1979) description of material from Austria. The ‘Sammlung von Algenkulturen at the University of Göttingen’ for many years contained a culture identified as Woloszynskia pascheri (Suchlandt) von Stosch. It was sequenced by Logares et al. (Citation2007) and in the LSU found to be sister species to the cold-water species Borghiella tenuissima (Lauterborn) Moestrup, G. Hansen & Daugbjerg (as Woloszynskia tenuissima). The culture was isolated from the Botanical Garden at University of Göttingen by W. Koch in 1965. It is not a cold-water species, as we have obtained the culture and maintained it at 15°C for many years. Identification of the culture as representing Suchlandt-Traunsteiner’s ice alga has long been suspect. The culture is no longer listed in the Göttingen collection but can be obtained from the Algal Culture Collection at University of Cologne, still under the number CCAC 0075, but now identified as Borghiella dodgei.
Our studies have indicated that CCAC 0075 may be an undescribed species, but a fuller description of the species will be given separately, using TEM and SEM. We have collected material from southern Sweden (Karin Lindberg, MSc Thesis), which resembles CCAC 0075, indicating that this species is widespread but probably often overlooked. Material from Wisconsin, USA identified as Woloszynskia pascheri by Wilcox (Citation1986, Citation1989) does not agree with Borghiella pascheri as studied by us and belongs to a different, unknown, species.
Acknowledgements
We thank Lis M. Frederiksen for sectioning the material studied here. ND thanks the Carlsberg Foundation and Brødrene Hartmann for equipment grants. Our studies on the identity of Borghiella pascheri (as Woloszynskia pascheri) began several years ago but had to be discontinued due to lack of material that agreed with the morphology and ecology of the original material from the European Alps. The initial work was done by Karin Lindberg and we thank Villum-Kann Rasmussen Foundation for a grant supporting her during this work. We finally wish to thank Antonio Calado for comments on a previous version of the manuscript.
Disclosure statement
No potential conflict of interest was reported by the authors.
Additional information
Funding
Notes on contributors
Øjvind Moestrup
Ø. Moestrup. scanning and transmission electron microscopy, drafting and editing of manuscript; N.H. Nicholls, collection and shipping of material from Canada; N. Daugbjerg, molecular sequencing and analysis of data.
References
- Boutrup, P.V., Moestrup, Ø., Tillmann, U. & Daugbjerg, N. (2016). Katodinium glaucum (Dinophyceae) revisited: proposal of new genus, family and order, based on ultrastructure and phylogeny. Phycologia, 55: 147–164.
- Craveiro, S.C., Pandeirada, M., Daugbjerg, N., Moestrup, Ø. & Calado, A.J (2013). Ultrastructure and phylogeny of Theleodinium calcisporum gen. et sp. nov., a freshwater dinoflagellate that produces calcareous cysts. Phycologia, 52: 488–507.
- Daugbjerg, N., Andreasen, T., Happel, E., Pandeirada, M., Hansen, G., Craveiro, S.C., Calado, A.J. & Moestrup, Ø. (2014). Studies on woloszynskioid dinoflagellates VII. Description of Borghiella andersenii sp. nov.: light and electron microscopy and phylogeny based on LSU rDNA. European Journal of Phycology, 49: 436–449.
- Dodge, J.D. & Crawford, R.M. (1970). A survey of thecal fine structure in the Dinophyceae. Botanical Journal of the Linnean Society, 63: 53–67.
- Doyle, J.J and Doyle, J.L. (1987). A rapid DNA isolation procedure for small quantities of fresh leaf tissue. Phytochemical Bulletin, 19: 11–15.
- Flaim, G., Hansen, G., Moestrup, Ø., Corradini, F. & Borghi, B. (2004). Reinterpretation of the dinoflagellate ‘Glenodinium sanguineum’ in the reddening of Lake Tovel, Italian Alps. Phycologia, 43: 737–743.
- Frassanito, R., Flaim, G., Mancini, I. & Guella, G. (2006). High production of unexpected carotenoids in Dinophyceae. Astaxanthin esters from the freshwater dinoflagellate Tovellia sanguinea. Biochemical Systematics and Ecology, 34: 843–853.
- Gerrath, J.F. & Nicholls, K.H. (1974). A red snow in Ontario caused by the dinoflagellate, Gymnodinium pascheri. Canadian Journal of Botany, 52: 683–685.
- Gunning, B.E.S. & Steer, M.W. (1975). Plant Cell Biology: An Ultrastructural Approach. Edward Arnold, London.
- Hansen, G. & Daugbjerg, N. (2011). Moestrupia oblonga gen. et comb. nov. (syn.: Gyrodinium oblongum), a new marine dinoflagellate genus characterized by light and electron microscopy, photosynthetic pigments and LSU rDNA sequence. Phycologia, 50: 583–599.
- Hansen, G., Daugbjerg, N. & Franco, J.M. (2003). Alexandrium minutum (Dinophyceae) from Denmark – a study based on morphology, toxin composition and LSU rDNA sequences, with some morphological observations on other European strains. Harmful Algae, 2: 317–335.
- Hansen, G., Daugbjerg, N. & Moestrup, Ø. (2018). The rainwater rock-pool dinoflagellate Nottbeckia ochracea gen. et comb. nov. (syn.: Hemidinium ochraceum) – a fine-structural and molecular study with emphasis on the motile stage. Protist. 169: 280–306.
- Kusel-Fetzmann, E. (1979). The algal vegetation of Neusiedlersee. In Neusiedlersee: The Limnology of a Shallow Lake in Central Europe (Loeffler H., editor), 171–202. W. Junk Publishers, The Hague.
- Lindberg, K., Moestrup, Ø. & Daugbjerg, N. (2005). Studies on woloszynskioid dinoflagellates I: Woloszynskia coronata re-examined using light and electron microscopy and partial LSU rDNA sequences, with description of Tovellia gen. nov and Jadwigia gen. nov. (Tovelliaceae fam. nov.). Phycologia, 44: 416–440.
- Lindemann, E. (1928). Vorläufige Mitteilung. Archiv für Protistenkunde, 63: 259–260.
- Lindemann, E. (1929). Experimentelle Studien über die Fortpflanzungserscheinungen der Süßwasserperidineen auf Grund von Reinkulturen. Archiv für Protistenkunde, 68: 1–104.
- Loeblich A.R. III. (1970). The amphiesma or dinoflagellate cell covering. In Proceedings of the North American Paleontological Convention (Yochelson E.L., editor), 868–929. Allen Press, Lawrence, Kansas.
- Logares, R., Shalchian-Tabrizi, K., Boltovskoy, A. & Rengefors, K. (2007). Extensive phylogenies indicate infrequent marine-freshwater transitions. Molecular Phylogenetics and Evolution, 45: 887–903.
- Moestrup, Ø. & Daugbjerg, N. (2007). On dinoflagellate phylogeny and classification. In Unravelling the Algae: The Past, Present, and Future of Algae Systematics (Brodie, J. & Lewis, J., editors), 215–230. Systematics Association Special Volumes, Vol. 75. CRC Press, Taylor & Francis Group.
- Moestrup, Ø., Hansen, G., Daugbjerg, N., Flaim, G. & D’Andrea, M. (2006). Studies on woloszynskioid dinoflagellates II: on Tovellia sanguinea sp. nov., the dinoflagellate species responsible for the reddening of Lake Tovel, N. Italy. European Journal of Phycology, 41: 47–65.
- Moestrup, Ø., Hansen, G. & Daugbjerg, N. (2008). Studies on woloszynskioid dinoflagellates III: on Borghiella gen. nov., and B. dodgei sp. nov., a cold-water species from Lake Tovel, N. Italy, and on B. tenuissima comb. nov. (syn. Woloszynskia tenuissima). Phycologia, 47: 54–78.
- Morrill, L.C. & Loeblich, A.R. III (1981). The dinoflagellate pellicular wall layer and its occurrence in the division Pyrrhophyta. Journal of Phycology, 17: 315–323.
- Morrill, L.C. & Loeblich, A.R. III (1983). Ultrastructure of the dinoflagellate amphiesma. International Review of Cytology, 82: 151–180.
- Nicholls, K.H. (2017). Introduction to the biology and ecology of the freshwater cryophilic dinoflagellate Woloszynskia pascheri causing red ice. Hydrobiologia, 784: 305–319.
- Pickett-Heaps, J.D. (1975). Green Algae. Sinauer, Sunderland, Massachusetts.
- Remias, D., Pichrtová, M.,. Pangratz, M., Lütz, C. & Holzinger, A. (2016). Ecophysiology, secondary pigments and ultrastructure of Chlainomonas sp. (Chlorophyta) from the European Alps compared with Chlamydomonas nivalis forming red snow. FEMS Microbiology Ecology, 92: doi: 10.1093/femsec/fiw 030.
- Schiller, J. (1933). Flagellatae, Dinoflagellatae (Peridiniae). In Kryptogamen-Flora von Deutschland, Österreich und der Schweiz (Rabenhorst, L., editor), Bd. 10, Abth. 3, Teil 1. Akadem. Verlags., Leipzig. 617 pp.
- Schiller, J. (1954). Über winterliche pflanzliche Bewohner des Wassers, Eises und des daraufliegenden Schneebreies. I. Österreichische Botanische Zeitschrift, 101: 236–284.
- Schiller, J. (1955). Untersuchungen an den planktischen Protophyten des Neusiedlersees 1950–1954, I. Teil. Wissenschaftliche Arbeiten aus dem Burgenland, 9: 1–66.
- Suchlandt, O. (1916). Dinoflagellaten als Erreger von rotem Schnee. Berichte der Deutschen Botanischen Gesellschaft, 24: 242–246 plus Tafel III.
- Swofford, D.L. (2003). PAUP*. Phylogenetic Analysis Using Parsimony (*and Other Methods). Version 4. Sinauer Associates, Sunderland, Massachusetts.
- Traunsteiner, M. (1914). Rote Peridineen im Schnee. Kleinwelt nr 7: 104–106. Deutsche Mikrobiologische Gesellschaft. Bamberg Publ.
- von Stosch, H.A. (1973). Observations on vegetative reproduction and sexual life cycles of two freshwater dinoflagellates, Gymnodinium pseudopalustre Schiller and Woloszynskia apiculata. British Phycological Journal, 8: 105–134.
- Wilcox, L.W. (1986). Prokaryotic endosymbionts in the chloroplast stroma of the dinoflagellateWoloszynskia pascheri. Journal of Phycology, 135: 71–79.
- Wilcox, L.W. (1989). Multilayered structures (MLSs) in two dinoflagellates, Katodinium campylops and Woloszynskia pascheri. Journal of Phycology, 25: 785–789.
- Woloszynska, J. (1937). Die Algen der Tatraseen und Tümpel. III. Peridineen im Winterplankton einiger Tatraseen. Archivum Hydrobiologii i Rybactwa, 10: 188–196 plus Tab. IX.
- Zhang, Q., Zhu, H., Hu, Z. & Liu, G. (2016). Blooms of the woloszynskioid dinoflagellate Tovellia diexiensis sp. nov. (Dinophyceae) in Baishihai Lake at the eastern edge of the Tibetan Plateau. Algae, 31: 205–217.