ABSTRACT
The use of microalgae in a number of sectors, including biodiesel, feed and food production, is proving to be of great interest. An evaluation was made of the possible biostimulant effects on Chlorella vulgaris and Scenedesmus quadricauda of humic-like substances (HLs) extracted from agro-industrial wastes. These included digestate from the waste of an agro-livestock farm (D-HL), oil extraction residues from rape (B-HL, Brassica napus L.) and tomato residues (T-HL). The microalgal growth medium (BG11) was supplemented with HLs to evaluate their effect on biomass yield as well as carbohydrate, chlorophylls a and b, lipid and fatty acid contents. Our results showed that the HLs used in the test are effective biostimulants of C. vulgaris and S. quadricauda. The biostimulant effect seems to depend on the type of extract used for cultivating the microalgae, the concentration and the species treated. Among the extracts applied to the growth medium, D-HL and T-HL seem to have a significant effect on microalgal biomass and lipid production. Although B-HL showed no significant effect on the biomass and lipid content of C. vulgaris and S. quadricauda, its presence in the growth medium increased the saturated:unsaturated fatty acid ratio (SFA/UFA) and stimulated the sugar metabolism of the microalgae by increasing their carbohydrate and chlorophyll content.
Introduction
Microalgae are photosynthetic organisms, and can be autotrophic or mixotrophic. They can attain a high growth rate under very simple conditions and are not subject to seasonal restrictions (Mata et al., Citation2010). Microalgae can produce variable quantities of lipids, carbohydrates and proteins depending on the species and growing conditions. As a result, they are a very interesting option for use in various sectors. Indeed, the biomass can potentially be used to produce biofuels (biodiesel and bioethanol) and also other biochemicals such as oligopeptides, proteins, oligosaccharides and carbohydrates for the food sector (Maurya et al., Citation2016). For example, there has been increased recent interest in diet supplementation with microalgae to introduce essential fatty acids. While most fish have a limited capacity to synthesize omega-3 (n3), they accumulate polyunsaturated fatty acids (PUFA) if they are fed a diet based on microalgae (Matos et al., Citation2016).
A further use for cultivated microalgae is in wastewater treatment as diverse microalgae can remove pollutants from industrial, agricultural and municipal waste water (Hu et al., Citation2008; Hunt et al., Citation2010; Solana et al., Citation2014; Tao et al., Citation2017). Baglieri et al. (Citation2016) evaluated the capacity of Chlorella vulgaris and Scenedesmus quadricauda to remove pesticides (fenhexamid, metalaxyl, triclopyr, pyrimethanil and iprodione) from waste water, demonstrating that compared with sterile conditions and with data reported in the literature, they increased the degradation rate of all these compounds (Baglieri et al., Citation2009, Citation2011; Gennari et al., Citation2007).
Given the versatility of microalgae, several studies have focused on the possibility of simultaneously increasing biomass production and the yield of specific high-value products. In effect, a number of organic compounds can increase microalgal growth when added to a culture medium. Czerpak et al. (Citation2003) demonstrated that diamines (agmatine and putrescine) and polyamines (spermine and spermidine) stimulate mitosis and increase the protein, chlorophyll and monosaccharide content in C. vulgaris. Moreover, some growth stimulants such as phytohormones, vegetable extracts and polyamides are potentially useful in improving the productivity of microalgae (Czerpak et al., Citation2003). Hunt et al. (Citation2010) studied Chlorella sorokiniana to evaluate how 12 biochemical stimulants influenced its growth and chlorophyll, protein and lipid contents. A higher biomass yield (c. 0.145 g l–1 d–1) was obtained by adding a combination of 1-naphthaleneacetic acid (5 ppm), gibberellic acid (10 ppm) and zeatin (1 ppm) to the culture medium. Interesting results were also obtained by adding humic acid (20 ppm) which induced a significant increase (P<0.05) in biomass (c. 0.030 g l–1 d–1) and chlorophyll content (0.5 g l–1 d–1) as compared with the control (c. 0.018 g l–1 d–1 and 0.18 g l–1 d–1, respectively).
Biostimulants, also called metabolism inducers, are a vast range of compounds that promote plant growth when used in small quantities in the soil or applied directly onto the leaf surface (Ertani et al., Citation2009). Biostimulants belong to three main groups: humic substances, algal extracts and products containing amino acids. Both the humic substances and algal extracts contain compounds that are similar to growth hormones such as auxin and gibberellin, which influence the physiology of the plants when applied in small quantities (Ertani et al., Citation2009). Furthermore, when humic-like substances (HLs) extracted from Brassica napus, Ricinus communis and Linum usitatissimum agro-industrial residues, and from a digestate, were used to treat corn seedlings, they proved effective in increasing both protein content and the activity of enzymes involved in the assimilation of nitrogen and in photosynthesis (Ertani et al., Citation2013). Similarly, it has been shown that extracts from tomato plant residues obtained by alkaline hydrolysis act as a biostimulant on bean plants, generally leading to an increase in the quantity of nitrogen assimilated (Baglieri et al., Citation2014; Monterumici et al., Citation2015).
The aim of this work was to evaluate the bio-stimulant effect of HLs on C. vulgaris and S. quadricauda (Chlorophyta). The HLs were obtained through alkaline hydrolysis of different sources, including a digestate of agro-livestock residues (D-HL), Brassica napus biomass residues after extraction of oil (B-HL) and tomato cultivation residues (T-HL). The extracts were added to the growth medium of the microalgae and their effect on the quantity of biomass produced and the carbohydrate, chlorophylls a and b, lipid and fatty acid contents was evaluated.
Materials and methods
Humic-like substances (HLs)
The humic-like substances (HLs) were extracted from agroindustrial wastes including: digestate from the waste of an agro-livestock farm (D-HL), rape oil extraction residues from Brassica napus L. (B-HL) and tomato residues from agroindustrial waste (T-HL). Alkaline hydrolysed extracts were obtained as described by Ertani et al. (Citation2013). Briefly, a 200 g sample of finely ground waste was treated with aqueous 0.1 mol l−1 KOH at a 1:5 w/v (waste/solution ratio). The suspension was shaken under N2 for 20 h, allowed to settle overnight and centrifuged at 3000 rpm for 20 min. In order to solubilize and totally remove the HLs, three treatments with 0.1 mol l−1 KOH were carried out on the solid residue. The supernatant collected was freeze-dried and used for further analysis. These fractions had already been characterized in previously published works and have been summarized in (Ertani et al., Citation2013; Baglieri et al., Citation2014).
Table 1. Characterization of HLs fractions as reported in Ertani et al. (Citation2013) (D-HL and B-HL) and Baglieri et al. (Citation2014) (T-HL).
Microalgal culture and experimental conditions
The microalgae used in this study were Chlorella vulgaris (Beijerinck, CCAP 211/11C) and Scenedesmus quadricauda (isolated from an algal company raceway pond, located in Borculo, Gelderland, the Netherlands in 2011) obtained by and maintained in the algal collection of Swansea University (Wales, UK). All the experiments were conducted in triplicate using a 250 ml flask containing 150 ml of sterile BG11 culture medium at pH 8.4 (Baglieri et al., Citation2016). The culture medium was inoculated with 50 ml of algal suspension, containing 75 mg of cells calculated as dry mass at 60°C, from the centrifuged pellet obtained from the homogeneous algal suspension. For each microalgal species two different concentrations of HLs (1 = 100 mg l–1 and 2 = 200 mg l–1) were added. The HLs used in all the experiments were: B-HL1, B-HL2, D-HL1, D-HL2, T-HL1 and T-HL2. BG11 without HLs was used as the control. The flasks were closed with a cotton plug and incubated on a mechanical shaker (100 rpm) at 25–30°C, illuminated by a 3500-lx, average photon flux (PPF) 100 μmol photons m−2 s−1 light source (PHILIPS SON-T AGRO 400) with a 12-h photoperiod. The cultures were aerated by pumps with 20 l h–1 1.5 % CO2-enriched sterile humidified air during the light periods. Weekly, BG11 was added to compensate for water lost through evaporation. All analyses were performed on the microalgal samples after 46 days in the exponential growth phase (Baglieri et al., Citation2016).
Biomass determination
The total algal biomass grown in each flask was determined in cultures maintained for 46 days in all the experimental conditions. The microalgae were harvested and centrifuged at 2500 rpm for 10 min and the pellet was oven-dried at 60°C until constant mass was reached.
Carbohydrate content determination
The dried algal samples (100 mg) were treated with 3 ml of 72% H2SO4 (w/w) at 30°C for 20 min. The hydrolysate was diluted with 84 ml of distilled water and 4% H2SO4 (w/w), then autoclaved at 121°C for 20 min (Moxley & Zhang, Citation2007).
Carbohydrate determination was performed by ion exchange chromatography using a Dionex DX500 system, equipped with CarboPac PA20, using an isocratic elution of 20 mM NaOH. The detector (pulsed amperometric-EDet1) used the Gold Standard PAD waveform with an AgCl reference electrode which included the following electrode potentials set as waveform A; E1: +0.1 V for 400 ms, E2: −2.0 V for 1 ms, E3: +0.6 V for 1 ms, E4: −0.1 V for 6 ms.
The total carbohydrate content was expressed as a percentage of all the sugar detected in the microalgal biomass (dry mass).
Chlorophylls a and b content determination
The determination of chlorophylls a and b was performed on the 46th day algal cultures by extraction in ethanol. The extracts were analysed by spectrophotometry (Jasco V-530 UV-vis spectrophotometer) at a wavelength of 665 nm for chlorophyll a and 649 nm for chlorophyll b (Jeffrey & Humphrey, Citation1975; Lichtenthaler, Citation1987). Chlorophylls a and b concentrations were calculated according to Wellburn & Lichtenthaler (Citation1984).
Determination of lipid content and fatty acids analysis
Lipids were extracted from freeze-dried samples using dichloromethane following the method described by Folch et al. (Citation1957). Total lipids content was expressed as g l–1.
Extraction of the oleic fraction from the algal biomass was performed by a direct transesterification (DT) procedure as described by Alves et al. (Citation2008). Briefly, 250 mg of samples were treated with 1 ml of toluene and 3 ml of 5% HCl solution in methanol (prepared by adding acetyl chloride to the methanol). After homogenization by vortexing, the samples were incubated for 2 h at 70°C in a water bath. The solution was then cooled at room temperature and subsequently neutralized with 5 ml of 6% K2CO3. Fatty acid methyl esters (FAME) were extracted with 2 ml of hexane and 1 g of an activated carbon/Na2SO4 mixture. Samples were centrifuged at 2500 rpm for 5 min, the supernatant was recovered and the solvent removed under nitrogen at 37°C. Finally, the samples were purified on silica gel cartridges (500 mg ml–1) in a SPE column (Solid Phase Extraction). The final residue was dissolved in 1 ml of hexane, and stored for gas chromatography analysis. Gas chromatography was performed according to Coppa et al. (Citation2015) in a GC-System 7890A (Agilent Technologies Inc., Santa Clara, California, USA) equipped with an ion trap mass detector and a CP-Sil 88 capillary column (100 m × 0.25 mm) (Chrompack Nederland BV, Middelburg, the Netherlands) using hydrogen as the carrier gas. The injector and detector temperatures were 250°C and 255°C, respectively. The initial temperature was 70°C for 1 min, increased at a rate of 5°C/min to 100°C for 2 min, then raised to 140°C at 10°C/min and held for 40 min. Finally, the temperature was increased to 225°C for 15 min. The carrier gas was hydrogen and the pressure was maintained at a constant (158.6 kPa) during analysis. The peaks were routinely identified by comparing retention times against commercial authentic standards. The FA composition was expressed as g 100 g–1 FA and as g kg–1 of dry matter (DM).
Statistical analysis
Data regarding biomass, carbohydrate, chlorophyll, lipid and fatty acid composition were analysed by one-way ANOVA (P<0.05) followed by Tukey’s test for multiple comparison procedures.
Results
Biomass and total lipid determination
The total quantity of cells obtained at the end of the experimental period was determined in order to evaluate the effect that the treatments had on the production of biomass (). As compared with the control, a significant increase (P<0.05) in the biomass produced was found in both species following treatment with D-HL1 (41% and 31% for C. vulgaris and S. quadricauda, respectively) and treatment with T-HL1 (29% and 21% for C. vulgaris and S. quadricauda, respectively). In all other cases the differences in biomass production were not significant.
Table 2. Biomass and total lipids of microalgae grown in the absence or presence of different humic-like substances for 46 days.
The total lipid content of both species increased after treatment under all experimental conditions, presenting a similar trend to that seen for the accumulation of biomass (). A slight decrease (c. 10%), in comparison with the control (), was observed only in C. vulgaris treated with B-HL (both concentrations) and D-HL 2. In S. quadricauda, treatment with all extracts led to an increase in the lipid content. This increase was much higher than that found for C. vulgaris, when compared with the control. However, S. quadricauda had a lower total lipids content than C. vulgaris.
Carbohydrate and chlorophyll content
shows the composition of the microalgae in terms of galactose, glucose, xylose, total carbohydrates and chlorophylls a and b after 46 days of growth, in the presence of the HLs. Significant variability (P<0.05) was found in the monosaccharide content of both microalgal species when the various HLs were present (). When sugar content was analysed, there was a greater amount of glucose compared with other sugars, with percentages between c. 11% and 19% of total biomass in C. vulgaris and between c. 11% and 17% in S. quadricauda. This was followed by xylose (c. 4–8% in C. vulgaris and c. 5–8% in S. quadricauda) and galactose (from totally absent to c. 2% in C. vulgaris and c. 0.4–4% in S. quadricauda).
Table 3. Carbohydrate and chlorophyll composition of microalgae grown in the absence or presence of different humic-like substances for 46 days.
In both microalgal species, treatment with B-HL mostly led to an increase in total carbohydrates and their fractions. In particular, B-HL induced an increase in the total carbohydrate content of both C. vulgaris and S. quadricauda at both doses. With regard to monosaccharides, in C. vulgaris the glucose content increased significantly after treatment with B-HL1 and B-HL2 (19.18% and 17.79% of biomass, respectively) as compared with the control (15.27%). Moreover, the addition of B-HL2 led to a large increase in galactose content. However the response of the S. quadricauda strain to B-HL was slightly different: there was again an increase at both concentrations in regard to glucose, while an increase in galactose and xylose occurred only at the lowest and highest concentrations, respectively. Similar to the results obtained for the total carbohydrates, there was an increase in both chlorophyll a and b concentrations in both microalgae, as compared with the control, after 46 days of growth with both B-HL doses ().
Conversely, all the other treatments induced, in general, a reduction in the content of carbohydrates and chlorophylls in both species. In fact, D-HLs and T-HLs negatively affected total carbohydrates as well as monosaccharides, in comparison with the control, except for T-HL 1 which caused a slight increase in total carbohydrates, glucose and xylose in S. quadricauda and D-HL2 with a slight increase in glucose in the same species ().
Similarly, D-HL and T-HL treatments generally induced a decrease in chlorophylls in each microalgal species, at both concentrations. However, similar chlorophyll b values to the control were obtained in C. vulgaris and S. quadricauda treated with D-HL1 and T-HL2, respectively ().
Fatty acids composition
Growth conditions influenced the distribution of saturated (SFA) and unsaturated FA (UFA), particularly in C. vulgaris (). Indeed, treatment with D-HL and T-HL induced a greater production of UFA than SFA in C. vulgaris. The quantities of SFA and UFA in S. quadricauda grown on BG11 (control) were equally distributed (50.5% and 49.5% respectively) (). Interestingly, only the treatment with D-HL1 caused a significantly different distribution (P<0.05) compared with that of the control (35% SFA acids and 64% UFA).
Fig. 1. Fatty acids (UFA: unsaturated fatty acids; SFA: saturated fatty acids) composition, as total fatty acid distribution, in the biomass of Chlorella vulgaris (A) and Scenedesmus quadricauda (B) grown in the absence or in the presence of different humic-like substances (HLs: D= digestate; B= Brassica; T= tomato; HL= humic-like substance; 1: 100 mg l–1; 2: 200 mg l–1) for 46 days. The values are means of data from three replicates. Values for each species and fatty acid followed by different letters (in italics for UFA and bold for SFA) are significantly different (P<0.05).
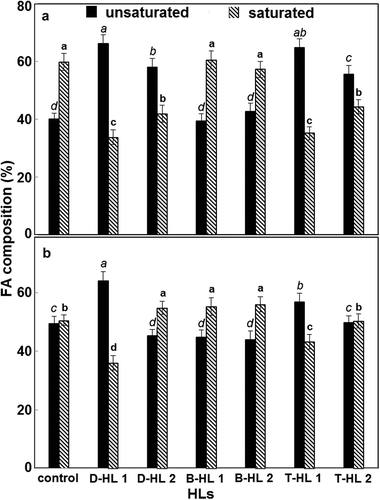
Chlorella vulgaris cultivated in BG11 contained mainly palmitic (34.5%), oleic (11.7%), stearic (7.6%), linoleic (5.87%), elaidic (5.47%) and alpha-linoleic (4.41%) acids as a percentage of total FA (). D-HL and T-HL in C. vulgaris were actively involved in decreasing the FA palmitic, stearic and oleic acids, even if in the latter the negative effect was seen only at the higher concentration. On the other hand in C. vulgaris, D-HL and T-HL increased the quantity of UFA (principally linoleic and alpha-linolenic acids) inducing an average production three times greater than the control. Moreover these treatments positively affected the content of minor FA, such as gamma-linolenic acid. Conversely, C. vulgaris cultivated in the presence of B-HL1 and B-HL2 contained mainly palmitic (31.28% and 28.17%, respectively), oleic (12.10% and 11.26%, respectively), linoleic (7.21% and 8.89%, respectively), stearic (6.97% and 5.07%, respectively) and alpha-linoleic (3.65% and 4.27%, respectively) acids (). However the percentage contents of these FA were rather different with respect to the control. In fact B-HLs resulted in a decrease in palmitic and stearic acids while linoleic acid increased with respect to the control. On the other hand, oleic and alpha-linolenic were similar to the control. Finally, HLs in C. vulgaris induced a general reduction in the content of elaidic acid with respect to the control, even if it was less relevant for B-HL. Conversely, in C. vulgaris, all the treatments with HLs, especially D-HL and T-HL, resulted in an increase in the SFA nonadecylic acid, as compared with the control.
Table 4. Fatty acids composition of Chlorella vulgaris grown in the absence or presence of different humic-like substances for 46 days.
Scenedesmus quadricauda cultivated in BG11 contained mainly palmitic (23.44%), oleic (12.42%), alpha-linoleic (10.86%) and linoleic (8.85%) acids as a percentage of total FA (). The changes in the FA composition of S. quadricauda were less relevant than in C. vulgaris. HLs were associated with a general decrease in palmitic and oleic acids, except for the lower concentration of B-HL, which resulted in a slight increase in the content of palmitic acid. As regards the linoleic acid of S. quadricauda, both doses of D-HL positively affected its content (D-HL1, 9.03%; D-HL2, 10.13%), whereas T-HLs (T-HL1, 8.37%; D-HL2, 5.75%) and the higher dose of B-HL (9.01%) decreased the percentage of linoleic acid. Moreover, the alpha-linoleic acid content increased in the treatment with T-HLs (T-HL1, 13.43%; T-HL2, 11.23%) and the lower dose of D-HL (12.66%), whereas it decreased with B-HLs (B-HL1, 10.05%; B-HL2, 9.87%) and the higher dose of D-HL (9.23%). It should be noted that in S. quadricauda gamma-linolenic acid was not found in the control, but significant quantities were found in the treated samples, the most active being the D-HL and T-HL treatments. Overall, in S. quadricauda the lower dose of D-HL induced the greatest change in FA composition, resulting in a higher increase in UFA gamma-linolenic, alpha-linolenic and linoleic acids with respect to the control. Conversely, the increase observed in the SFA of S. quadricauda with the addition of B-HL1, as compared with the control, was mostly due to palmitic (24.01%), behenic (2.41%), myristic (1.93%) and stearic (1.37%) acids (). Regarding the other fatty acids, although the variations were statistically significant they are difficult to explain, probably being related to upstream factors.
Table 5. Fatty acids composition of the Scenedesmus quadricauda grown in the absence or in the presence of different humic-like substances for 46 days.
Discussion
Our results showed that extracts obtained by alkaline hydrolysis from a variety of food industry waste can have a biostimulant effect on C. vulgaris and S. quadricauda. D-HL and T-HL mainly affected biomass and lipid composition, whereas B-HL positively influenced carbohydrates and chlorophylls. The correlation coefficient, calculated between the parameters ‘total lipids produced’ and ‘biomass accumulated’, resulted in values of 0.73 and 0.75 (P=0.05) for C. vulgaris and S. quadricauda, respectively. Our results agree with those given by Talebi et al. (Citation2013), who observed a strong correlation between the total lipids produced and the amount of biomass.
The differences in biostimulant effect depending on the type of extract used may be explained by the different chemical characteristic of the extracts. The most relevant differences in the composition of the extracts are related to the aromatic carbon and indolacetic acid contents, which are about twice as high in D-HL and T-HL as in B-HL, as well as the nitrogen content, which is higher in B-HL than in D-HL and T-HL (Ertani et al., Citation2013; Baglieri et al., Citation2014, ). It is well known that humic substances (HS) are characterized by an auxin-like effect, and the low molecular mass of HS fractions characterized by a high carboxyl and aromatic C content positively affects the metabolic parameters of plants (Ertani et al., Citation2013, Citation2016; Muscolo et al., Citation2013). Moreover, it has been demonstrated that the auxin-like behaviour of the B-HL and D-HL effectively leads to an increase in the protein content and activity of the enzymes involved in nitrogen assimilation and photosynthesis in corn seedlings (Ertani et al., Citation2013). At the same time, it has also been shown that alkaline hydrolysate from residues of tomato cultivation promotes growth in bean plants (Baglieri et al., Citation2014).
Our results suggest that the HLs under examination can also influence the growth and development of microalgae, with variable physiological responses depending on the algal species and type of extract used. Furthermore, we demonstrated that there is a greater effect on biomass production when the extract concentrations are lower, in particular in the presence of D-HL and T-HL. Different outcomes observed at high concentrations could be due either to increased turbidity of the medium which affected light penetration, or the presence of molecules in the extract which in some way inhibited the hormone-like activity, as observed by Hunt et al. (Citation2010). According to this hypothesis, many phenolic compounds can be toxic for plants at high concentrations while at lower concentrations some phenolic acids can have a similar effect to that of indoleacetic acid or gibberellic acid (Hrubcovà et al., Citation2000; Pizzeghello et al., Citation2006). Interestingly, both doses of B-HL, characterized by a lower level of IAA and aromatic compounds as compared with D-HL and T-HL, exerted a similar effect on almost all of the parameters evaluated.
Microalgae contain complex carbohydrates composed of a mixture of neutral sugars, amino sugars and uronic acid whose composition varies greatly between species and growth conditions (Templeton et al., Citation2012). Therefore, it is crucial to know the carbohydrate profile and the photosynthetic pigment content of the different microalgal species.
As regards the sugar composition, the values obtained in this study agree with those reported by Brown et al. (Citation1997) in Chlorella sp. – an exception was the quantity of xylose which was higher than in Brown et al. (Citation1997).
Our results highlighted that, of the substances tested, B-HL was the most effective in increasing the carbohydrate and chlorophyll content of both microalgal species (). Likewise, Ertani et al. (Citation2013) found that corn plants treated with B-HL accumulated greater quantities of chlorophylls a and b compared with those seen in the control plants. More recently, in order to select the best microalgal species for food applications, Matos et al. (Citation2016) studied the biochemical composition of a number of species, among which C. vulgaris proved to be the best candidate as a source of low fibre carbohydrates. Treatment of microalgae with B-HL could increase the carbohydrate content which would be very useful for potential food or animal feed industry applications.
As suggested by Hunt et al. (Citation2010), algal biomasses with lower pigment contents are potentially interesting for the production of biofuels, given that chlorophyll is known to interfere with the extraction of oils and affect the subsequent production of biodiesel. Therefore, D-HL and T-HL which induced a greater increase in algal biomass and lipids () while at the same time reducing the production of pigments () could be used to produce microalgae for biofuel extraction.
Microalgae synthesize FA to form a variety of lipids. Different growth conditions can lead to considerable variation in the lipid content and the composition of FA in both C. vulgaris and S. quadricauda (Hu et al., Citation2008; Han et al., Citation2016). FA characterization is therefore fundamental, as the use of microalgae in a number of sectors (for example the production of biofuels or for use in the food industry) depends directly on FA composition. It has been demonstrated that in C. vulgaris SFA, mono-unsaturated (MUFA), C16:0 and C18:0 were the main groups synthesized (Hu et al., Citation2008). In agreement with these data, C. vulgaris cultivated in BG11 contained mainly palmitic, stearic and oleic acids (). Similarly, in S. quadricauda, under all growth conditions, there was a predominance of FA with chains between C16 and C18, of which palmitic acid, oleic acid, linoleic acid and alpha linolenic acid were the most representative (), in agreement with Kandimalla et al. (Citation2016). Our results have shown that, among the substances added to the growth medium for both the microalgal species, D-HL and T-HL resulted in a greater increase in lipid content. This suggests that treatment with different HLs could determine a diverse distribution of lipid and FA contents and could therefore support multiple applications. It is difficult to fully understand how the composition of HLs may influence the different distribution of FA in the microalgal species. Moreover, it is very probable that different compounds working together may affect microalgal composition.
The FA composition of human food is extremely important; PUFA include essential FA which cannot be produced by the human body. Foods with a PUFA/SFA ratio below 0.45 are considered undesirable for the human diet due to the fact that they can potentially induce an increase in the level of cholesterol in the blood (FAO/WHO, Citation2008). It is interesting to note that the PUFA/SFA ratios calculated for C. vulgaris (0.98) and S. quadricauda (0.84) treated with D-HL1 increased considerably compared with the values seen in the control (0.22 for C. vulgaris and 0.55 for S. quadricauda). Similar PUFA/SFA ratios, 0.96 for C. vulgaris and 0.76 for S. quadricauda, were also found when T-HL1 was present. Moreover, a preference for foods characterized by an omega-3/omega-6 (n3/n6) ratio ≥0.2 has been recommended, given that n3 and n6 FA are necessary for human health (Armenta & Valentine, Citation2013). It should be underlined that the n3/n6 ratio calculated for S. quadricauda in all the experimental growth conditions varied from 1.3 to 1.6 while for C. vulgaris treatment with D-HL1 determined an increase in the n3/n6 ratio (1.0) compared with the control (0.83). D-HL and T-HL could therefore prove useful in the production of microalgae for lipid extraction for eventual use in the food or animal feeds industries.
The type and quantity of lipids produced by the microalgae can directly affect the quality of the biodiesel obtained from them. Duong et al. (Citation2015) demonstrated that Chlorella sp. NT8a and Scendesmus dimorphus NT8a accumulate the greatest quantity of triglycerides and can be considered suitable for producing biodiesel after further optimization of their growth conditions. The FA profile and the SFA/UFA ratio are of overriding importance in biodiesel production (Radakovits et al., Citation2010). Indeed, SFA are more resistant to degradation and therefore increase the longevity of the biodiesel and its resistance to oxidation. Higher saturation levels determine higher density and viscosity of the fuel (Talebi et al., Citation2013). It is interesting to note that the highest SFA/UFA ratios were obtained from treatment with B-HL. The ratio in S. quadricauda in particular increased from 1.0 to 1.27. Therefore, adding B-HL to the growth medium of microalgae for biodiesel production could lead to a higher yield.
This study demonstrated that HLs from agro-industrial waste exert a biostimulant effect on C. vulgaris and S. quadricauda. In particular, D-HL and T-HL positively affected biomass and lipid composition, mostly at lower concentrations, whereas B-HL increased carbohydrates and chlorophylls regardless of the concentration. The capacity to increase biomass, lipids and carbohydrates in microalgae, on the basis of the HLs used, might reduce production costs and broaden the applications of microalgae to include the food, animal feeds and biofuels sectors. Our results provide the basis for developing a range of ideal mixtures either made up of different HLs applied to the same microalgae or of a mixture of different microalgae treated with the same HL.
Author contributions
Ivana Puglisi: writing and editing manuscript, data analysis, statistical analysis; V. Barone: carbohydrate determination, lipid and fatty acids determination, pigment analysis, strain cultivation; S. Sidella: strain cultivation, pigment analysis, statistical analysis; M. Coppa: lipid and fatty acids determination, statistical analysis; C. Broccanello: Humic-like substances extraction: M. Gennari: writing and editing manuscript; A. Baglieri: original concept, experimental design, writing and editing manuscript.
Acknowledgements
We thank Mr. Salvatore Gulino for his technical support during the laboratory work.
Disclosure statement
No potential conflict of interest was reported by the author(s).
References
- Alves, S.P., Cabrita, A.R.J., Fonseca, A.J.M. & Bessa, R.J.B. (2008). Improved method for fatty acid analysis in herbage based on direct transesterification followed by solid-phase extraction. Journal of Chromatography A, 1209: 212–219.
- Armenta, R.E. & Valentine, M.C. (2013). Single-cell oils as a source of omega-3 fatty acids: an overview of recent advances. Journal of the American Oil Chemists’ Society, 90: 167–182.
- Baglieri, A., Abbate, C., Borzì, D. & Gennari, M. (2009). Adsorption of pyrimethanil on soil and some of its colloids. Fresenius Environmental Bulletin, 18: 578–584.
- Baglieri, A., Gennari, M., Arena, M. & Abbate, C. (2011). The adsorption and degradation of chlorpyriphos-methyl, pendimethalin and metalaxyl in solid urban waste compost. Journal of Environmental Science and Health Part B, 46: 454–460.
- Baglieri, A., Cadili, V., Mozzetti Monterumici, C., Gennari, M., Tabasso, S., Montoneri, E., Nardi, S. & Negre, M. (2014). Fertilization of bean plants with tomato plants hydrolysates. Effect on biomass production, chlorophyll content and N assimilation. Scientia Horticulturae, 176: 194–199.
- Baglieri, A., Sidella, S., Barone, V., Fragalà, F, Silkina, A., Nègre, M. & Gennari, M. (2016). Cultivating Chlorella vulgaris and Scenedesmus quadricauda microalgae to degrade inorganic compounds and pesticides in water. Environmental Science and Pollution Research, 23: 18165–18174.
- Brown, M.R., Jeffrey, S.W., Volkman, J.K. & Dunstan, G.A. (1997). Nutritional properties of microalgae for mariculture. Aquaculture, 151: 315–331.
- Coppa, M., Ferlay, A., Borreani, G., Revello-Chion, A., Tabacco, E., Tornambé, G., Pradel, P. & Martin, B. (2015). Effect of phenological stage and proportion of fresh herbage in cow diets on milk fatty acid composition. Animal Feed Science and Technology, 208: 66–78.
- Czerpak, R., Bajguz, A., Piotrowska, A., Dobrogowska, R., Matejczyk, W. & Wielawski, W. (2003). Biochemical activity of di- and polyamines in the green alga Chlorella vulgaris Beijerinck (Chlorophyceae). Acta Societatis Botanicorum Poloniae, 72: 19–24.
- Duong, V.T., Ahmed, F., Thomas-Hall, S.R., Quigley, S., Nowak, E. & Schenk, P.M. (2015). High protein- and high lipid-producing microalgae from northern Australia as potential feedstock for animal feed and biodiesel. Frontiers in Bioengineering and Biotechnology, 3: 53.
- Ertani, A., Cavani, L., Pizzeghello, D., Brandellero, E., Altissimo, A., Ciavatta, C. & Nardi, S. (2009). Biostimulant activity of two protein hydrolyzates in the growth and nitrogen metabolism of maize seedlings. Journal of Plant Nutrition and Soil Science, 172: 237–244.
- Ertani, A., Pizzeghello, D., Baglieri, A., Cadili, V. & Tambone, F. (2013). Humic-like substances from agro-industrial residues affect growth and nitrogen assimilation in maize (Zea mays L.) plantlets. Journal of Geochemical Exploration, 129: 103–111.
- Ertani, A., Pizzeghello, D., Francioso, O., Tinti, A. & Nardi, S. (2016). Biological activity of vegetal extracts containing phenols on plant metabolism. Molecules, 21: 205.
- FAO/WHO (2008). Fats and Fatty Acids in Human Nutrition – Report of an Expert Consultation. Food and Agriculture Organization of the United Nations – FAO, Geneva.
- Folch, J., Lees, M. & Sloane Stanley, G.H. (1957). A simple method for the isolation and purification of total lipids from animal tissues. Journal of Biological Chemistry, 226: 497–509.
- Gennari, M., Messina, C., Abbate, C., Baglieri, A. & Nègre, M. (2007). Adsorption of chlorpyrifos-methyl on some soil components. Influence of pH, ionic strength and organic acids. Fresenius Environmental Bulletin, 7: 764–769.
- Han, F., Pei, H., Hu, W., Han, L., Zhang, S. & Ma, G. (2016). Effect of high-temperature stress on microalgae at the end of the logarithmic phase for the efficient production of lipid. Environmental Technology, 37: 2649–2657.
- Hrubcová, M., Cvikrová, M., Eder, J., Zon, J. & Machácková, I. (2000). Effect of inhibition of phenylpropanoid biosynthesis on peroxidase and IAA-oxidase activities and auxin content in alfalfa suspension cultures. Plant Physiology and Biochemistry, 38: 949–956.
- Hu, Q., Sommerfeld, M., Jarvis, E., Ghirardi, M., Posewitz, M., Seibert, M. & Darzins, A. (2008). Microalgal triacylglycerols as feedstocks for biofuel production: perspectives and advances. The Plant Journal, 54: 621–639.
- Hunt, R.W., Chiannsamy, S., Bhatnagar, A. & Das, K.C. (2010). Effect of biochemical stimulants on biomass productivity and metabolite content of the microalga Chlorella sorokiniana. Applied Biochemistry and Biotechnology, 162: 2400–2414.
- Jeffrey, S.W. & Humphrey, G.F. (1975). New spectrophotometric equations for determining chlorophyll a, b, c1 and c2 in higher plants and natural phytoplankton. Biochemie und Physiologie der Pflanzen, 165: 191–194.
- Kandimalla, P., Desi, S. & Vurimindi, H. (2016). Mixotrophic cultivation of microalgae using industrial flue gases for biodiesel production. Environmental Science and Pollution Research, 23: 9345–9354.
- Lichtenthaler, H.K. (1987). Chlorophylls and carotenoids: pigments of photosynthetic apparatus biomembranes. Methods in Enzymology, 148: 349–382.
- Mata, T.M., Martinsa, A.A. & Caetanob, N.S. (2010). Microalgae for biodiesel production and other applications: a review. Renewable and Sustainable Energy Reviews, 14: 217–232.
- Matos, A.P., Feller, R., Moecke, E.H.S., de Oliveira, J.V., Junior, A.F., Derner, R.B. & Sant’Anna, E.S. (2016). Chemical characterization of six microalgae with potential utility for food application. Journal of the American Oil Chemists’ Society, 93: 963–972.
- Maurya, R., Paliwal, C., Chokshi, K., Pancha, I., Ghosh, T., Satpati, G.G., Pal, R., Ghosh, A. & Mishra, S. (2016). Hydrolysate of lipid extracted microalgal biomass residue: an algal growth promoter and enhancer. Bioresource Technology, 207: 197–204.
- Monterumici, C.M., Rosso, D., Montoneri, E., Ginepro, M., Baglieri, A., Novotny, E.H., Kwapinski, W. & Negre, M. (2015). Processed vs. non-processed biowastes for agriculture: effects of post-harvest tomato plants and biochar on radish growth, chlorophyll content and protein production. International Journal of Molecular Sciences, 16: 8826–8843.
- Moxley, G. & Zhang, Y.H.P. (2007). More accurate determination of acid-labile carbohydrates in lignocellulose by modified quantitative saccharification. Energy Fuels, 21: 3684–3688.
- Muscolo, A., Sidari, M. & Nardi, S. (2013). Humic substance: relationship between structure and activity. deeper information suggests univocal findings. Journal of Geochemical Exploration, 129: 57–63.
- Pizzeghello, D., Zanella, A., Carletti, P. & Nardi, S. (2006). Chemical and biological characterization of dissolved organic matter from silver fir and beech forest soils. Chemosphere, 65: 190–200.
- Radakovits, R., Jinkerson, R.E., Darzins, A. & Posewitz, M.C. (2010). Genetic engineering of algae for enhanced biofuel production. Eukaryotic Cell, 9: 486–501.
- Solana, M., Rizza, C.S. & Bertucco, A. (2014). Exploiting microalgae as a source of essential fatty acids by supercritical fluid extraction of lipids: comparison between Scenedesmus obliquus, Chlorella protothecoides and Nannochloropsis salina. Journal of Supercritical Fluids, 92: 311–318.
- Talebi, A.F., Mohtashami, S.K., Tabatabaei, M., Tohidfar, M., Bagheri, A., Zeinalabedini, M., Hadavand Mirzaei, H., Mirzajanzadeh, M., Malekzadeh Shafaroudi, S. & Bakhtiari, S. (2013). Fatty acids profiling: a selective criterion for screening microalgae strains for biodiesel production. Algal Research, 2: 258–267.
- Tao, Q., Gaoa, F., Qiana, C.-Y., Guoa, X.-Z., Zhou, Z. & Yang, Z.-H. (2017). Enhanced biomass/biofuel production and nutrient removal in an algal biofilm airlift photobioreactor. Algal Research, 21: 9–15.
- Templeton, D.W., Quinn, M., Van Wychen, S., Hyman, D. & Laurens, L. M. L. (2012). Separation and quantification of microalgal carbohydrates. Journal of Chromatography A, 1270: 225–234.
- Wellburn, A.R. & Lichtenthaler, H. (1984). Formulae and program to determine total carotenoids and chlorophyll a and b of leaf extracts in different solvents. In Advances in Photosynthesis Research (Nijhoff, M.E., editor), 272–284. W. Junk, The Hague.