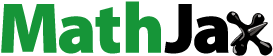
ABSTRACT
Raphidiopsis (Cylindrospermopsis) raciborskii is one of the most studied potentially harmful cyanobacteria. Single environmental factors such as increased temperature or light are reported to be promoters of R. raciborskii growth, but the interaction of two or more promoting factors is less understood. The performance of two strains of R. raciborskii (MVCC19, Uruguayan and LP1, Brazilian) were evaluated under acclimation and temperature shifts (25–32°C) in combination with two transitions from phosphorus (P) sufficiency to limiting growth conditions. When subjected to transition from high P sufficiency to a P-limiting state, strains were able to grow only at the warmer temperature if previously acclimated. The MVCC19 strain showed higher specific growth rates and a shorter growth phase than LP1. Morphological differences were also found: the MVCC19 strain produced shorter filaments, while the LP1 strain increased in length and the number of cells per filament. The results show the positive effect of high temperature on the ability of R. raciborskii to withstand P-limiting conditions, which may confer resilience of populations to periods of severe nutrient limitation in warm lakes. This finding contributes to the understanding of the success of this species in diverse environmental conditions. The effect of temperature on the tolerance of nutrient deficiency and the performance of strains under lake conditions suggests the need for a drastic reduction in nutrient loads to avoid R. raciborskii dominance in warmer lakes.
Introduction
It is well known that variations in aquatic community structure as a response to changes in abiotic conditions drive ecosystem functioning (Chase, Citation2010; Hunter-Cevera et al., Citation2016). The ability to predict species composition is particularly important in managed ecosystems providing human resources, such as drinking water supplies. Determining the factors driving the frequency, intensity and duration of potentially harmful algal blooms in aquatic ecosystems is particularly important (Mur et al., Citation1999). In order to adequately control and manage blooms, it is necessary to understand the ecophysiological behaviour of harmful algae in response to environmental changes, as well as dispersal and invasion trends (Carey et al., Citation2012; Thomas & Litchman, Citation2016).
One of the most-studied toxic and widespread distributed cyanobacteria in fresh waters is Raphidiopsis raciborskii (Wołonszyńska) Aguilera, Berrendero Gómez, Kastovsky, Echenique & Salerno (basionym Cylindrospermopsis raciborskii Wołoszyńska Seenaya & Subba Raju) (Aguilera et al., Citation2018). Recent phylogeographic studies using genetic markers have indicated that R. raciborskii originated in tropical America, spreading to Africa while these continents were connected, then to Australia and Asia and finally to Europe (Moreira et al., Citation2015). Several physiological characteristics have been reported as responsible for its worldwide success: wide thermal tolerance (Briand et al., Citation2004; Bonilla et al., Citation2016; Kovács et al., Citation2016), adjustment to low phosphate concentrations (Istvánovics et al., Citation2000; Amaral et al., Citation2014), large internal storage of polyphosphate reserves due to a wide range of cell nutrient quotas (Istvánovics et al., Citation2000), atmospheric nitrogen-fixing capacity (Kenesi et al., Citation2009; Yema et al., Citation2016), broad light tolerance (Bonilla et al., Citation2016), formation of dormant cells (i.e. akinetes; Padisák & Istvánovics, Citation1997) and production of cyanotoxins (Sinha et al., Citation2014).
Burford et al. (Citation2016), in a recent review, suggest that more detailed studies are needed to understand the complex physiology of R. raciborskii, and consequently forecast blooms. Increasing temperature has been pointed out as an important factor favouring the increase in frequency and intensity of blooms of R. raciborskii in temperate regions (Briand et al., Citation2004; Sinha et al., Citation2012; Antunes et al., Citation2015). Nutrient load increase also has been highlighted as a key factor in promoting R. raciborskii development (Posselt et al., Citation2009). However, some studies have reported high biomass of R. raciborskii even in areas with low phosphorus (P) (Bonilla et al., Citation2012; Prentice et al., Citation2015) and concomitantly with low nitrogen (N) concentrations (Briand et al., Citation2002).
At present, few studies have explored the interaction between the effects of temperature and nutrients on growth in R. raciborskii. These have reported a positive relationship between temperature and P uptake rates in phytoplankton dominated by R. raciborskii (Prentice et al., Citation2015); a reduction in thermal tolerance (i.e. higher Tmin and lower Tmax) under N-depletion in three R. raciborskii strains (Thomas & Litchman, Citation2016); the dominance of R. raciborskii in natural assemblage experiments under P limitation (1 µmol l–1) irrespective of temperature tested (25°C and 30°C; Ryan et al., Citation2017); and high growth rates under elevated temperature at different N:P ratios (4.5:1, 10:1 and 40:1), differing between two tested strains (Miotto et al., Citation2017). Considering that nutrient availability fluctuates in aquatic ecosystems, the regulation of phosphate uptake systems in a given environment can represent an adaptive advantage and consequently a significant trait (Litchman & Klausmeier, Citation2008; Aubriot et al., Citation2011; Prentice et al., Citation2015). Both P storage ability per se and the adjustment of cellular uptake systems to the exposed external nutrient concentration are important for growth optimization (Falkner et al., Citation2006; Amaral et al., Citation2014). Temperature fluctuations or acclimation may alter the P-metabolism of R. raciborskii. Therefore, it is unclear if the interactions between variable temperatures and flexible P metabolism are determining factors for R. raciborskii growth.
Another important issue regarding the range expansion of R. raciborskii is the biological differences between strains. Several studies have reported distinct biological features in strains isolated from different water bodies with respect to growth rate, morphology and toxin production (Chonudomkul et al., Citation2004; Stucken et al., Citation2009; Piccini et al., Citation2011; Pierangelini et al., Citation2014; Willis et al., Citation2015). Furthermore, several strains isolated from a single sample also showed differences in growth rate, morphology and toxin production (Willis et al., Citation2016; Miotto et al., Citation2017). The high physiological flexibility of R. raciborskii has been highlighted (Briand et al., Citation2004; Bonilla et al., Citation2012; Amaral et al., Citation2014) but there is still no consensus as to whether its success in diverse ecosystems is explained by the phenotypic plasticity or differences among strains, reinforcing the need for detailed physiological investigations of strains (Burford et al., Citation2016, Citation2018).
Here, the acclimation capability of two strains of R. raciborskii to changes in abiotic conditions was evaluated experimentally in order to help explain its success in a wide range of climates and trophic levels. Ecophysiological flexibility and strain performance were evaluated in relation to temperature changes alone and in combination with transitions from P sufficiency to P-limiting conditions. We hypothesized that simultaneous changes in nutrient concentration and temperature would affect growth more severely than changes in a single factor. The experimental design emulated alternative realistic scenarios to understand the role of multiple interacting factors in bloom behaviour, persistence and invasion capability.
Materials and methods
Raphidiopsis raciborskii cultures
Strains of R. raciborskii were isolated from a Uruguayan lake, Lago Javier, 34°51′S, 56°02′W (MVCC19) and a Brazilian lake, Lagoa do Peri, 27°44′S, 48°31′W (LP1); and kindly provided by Prof. Leonardo Rörig, Universidade Federal de Santa Catarina. Cultures of both strains were maintained in N-free BG11 medium at 25°C (typical for maintenance of strains in laboratories) and a constant irradiance of 80 µmol photons m–2 s–1 before the experiments. The cultures were not axenic, but were routinely checked for low abundance of heterotrophic microorganisms.
Experimental design
We performed three experiments, one manipulating only the temperature and two manipulating temperature and P status. All experiments consisted of placing aseptically 80 ml of culture in 100 ml sterilized flasks in thermostatic baths. When the experimental temperature differed from the previous culture conditions, we called this ‘no acclimation’; otherwise (i.e. no change in temperature previously set) the cultures were called ‘acclimated’. Temperature shifts were from 25°C to 32°C, and from 32°C to 25°C. This warming and cooling range is within the estimated optimum temperature range proposed for R. raciborskii (Lürling et al., Citation2013; Recknagel et al., Citation2014; Kovács et al., Citation2016). Shifts in phosphate concentration modified cultures from P-sufficiency to P-limiting growth conditions.
Three replicates were used in each temperature and P concentration treatment (i.e. 24 flasks for each experiment). In experiment 1, the goal was to test for differences in performance of R. raciborskii strains in response to temperature shifts (i.e. warming and cooling with and without acclimation). Strains were grown in modified BG11 (N-free) medium and the initial biomass for all cultures was ~0.12 measured as optical density. In experiment 2, the objective was to test for differences between R. raciborskii strains under temperature shifts concomitantly with transitions from P-sufficiency to P-limiting growth conditions. P-limitation was achieved by lowering the phosphate concentration in the growth medium (Amaral et al., Citation2014). The P-limiting state was confirmed when the growth rate approached stationary phase and by the low phosphate concentration in the medium (close to or below the detection limit). At the given lower P concentration, the culture attains lower biomass, which may lead to culture collapse if no new P is supplied. Also, P-limitation was confirmed by renewed growth after addition of fresh medium containing the corresponding P concentration. P-sufficient cultures were subjected to two phosphate transitions: (2a) drastic transition: from 7 mg total P l–1 (225.8 µM; P-sufficiency) to 0.31 mg total P l–1 (10 µM; P-limiting sensu Amaral et al., Citation2014) through the dilution of 21.8 ml of P-sufficient culture with 478.2 ml of N- and P-free BG11 medium; and (2b) moderate transition: from 1 mg total P l–1 grown culture (32.2 µM; P-sufficient) to 0.31 mg total P l–1 (P-limiting) through the dilution of 155 ml of the P-sufficient culture with 345 ml of N- and P-free BG11 medium. Both P transitions were performed for each strain culture and temperature shift. The cultures were kept in batch conditions under the corresponding treatments. A summary of experimental design is available in Supplementary fig. S1.
Given the possibility of collapse of cultures (i.e. when approaching stationary phase), on day 5 of experiment 2a and on day 6 of experiment 2b, the culture replicates were diluted with fresh BG11 (N-free) medium (1:1 volume) containing the corresponding 0.31 mg K2HPO4–P l–1. Every day all flasks were manually shaken and 3 ml was sampled for optical density measurements (absorbance at 750 nm, spectrophotometer Thermo Evolution 60). The optical density data were used for growth rate calculation according to Andersen (Citation2005): , where ODi and ODf are the optical density at the beginning and the end of exponential phase, respectively; and the Ti and Tf are the interval of days during exponential growth. Given the variability in growth patterns, the interval of days to calculate growth rate was selected separately for each strain (see Supplementary Material). In vivo chlorophyll a fluorescence (excitation wavelength 395 ± 65 nm, emission wavelength ≥ 660 nm, AquaFluor® Handheld Fluorometer, Turner Designs, Sunnyvale, California, USA) was measured in order to verify the growth detected in optical density data. An aliquot was conditioned in Eppendorf’s with acid Lugol’s solution for further counts, biovolume estimates and morphological analysis. These counts and calculations were made only for experiment 2a because this represented the larger shifts in temperature and P concentration. Quantitative analysis of trichomes was performed by counting a minimum of 100 trichomes in each replicate in an inverted microscope (Olympus CKX41) with a Sedgewick-Rafter chamber. Then, the concentration was estimated as number of trichomes l–1, and the biovolume was calculated by multiplying the concentration of trichomes and the median volume per trichome calculated according to Hillebrand et al. (Citation1999), using measures of length (μm) and width (μm). We also quantified the number of heterocysts and number of cells per trichome in 10 trichomes from each replicate using an optical microscope (Olympus BX40) at 1000× magnification.
Statistical analysis
Statistical analyses were carried out in the R environment (R Core Team) and STATISTICA 7.1 (StatSoft). Plots were made using SigmaPlot version 11.0 (Systat Software), STATISTICA 7.1 and R. Mann–Whitney tests were used to compare growth rates between the strains in all experiments, given that assumptions of parametric tests were never met. Aiming to contrast strains under the same conditions and environmental shifts, the growth rates were compared: (i) between strains under the same acclimation condition in order to evaluate if strains differ in their response to temperature or temperature + nutrient shifts; and (ii) in each strain between acclimation conditions in order to evaluate if acclimation affects the response to temperature or temperature + nutrient shifts.
Morphological traits, cell and trichome counts, biovolume and chlorophyll a fluorescence were compared using a Mann–Whitney test (experiment 2a). Traits in strains were compared at the beginning of the exponential phase and during exponential growth, by summing trait values of trichomes within each replicate and subtracting this from the summed values at the end of exponential phase. Thus, three values of trait variation over time were obtained for each strain and compared between strains. For trichome counts, biovolume and chlorophyll a fluorescence data, the comparisons were made between strains at the beginning and at the end of the experiment separately. Furthermore, the relationships between trichome length and number of heterocysts for each of the two strains were compared using Kruskal–Wallis and the post hoc Nemenyi test.
Results
Growth rate responses of strains to temperature shifts
In experiment 1, the strains showed distinct growth curves (). MVCC19 showed exponential growth during the experiment. LP1 had a variable growth and lag phase in some treatments, so the cultures were discontinued. No difference was found between the growth rates of acclimated strains at 25°C (average: 0.161 ±0.005 d–1 and 0.158 ±0.014 d–1, p > 0.05; MVCC19 and LP1, respectively). In contrast, in the no-acclimation treatment (i.e. 32–25°C), the growth rates between strains were different (p = 0.049, Z = −1.963). The averaged growth rates were 0.158 ±0.002 d–1 and 0.187 ±0.018 d–1, MVCC19 and LP1, respectively. Acclimation had no effect on growth rates within each strain (p > 0.05).
Fig. 1. Growth curves of experiment 1 (with phosphorus sufficiency). Temperature shifts were performed at time zero. The curves were obtained with the mean values (n = 3, ± standard deviation; error bars are smaller than the size of the symbols if not visible) of optical density for each strain and temperature shift.
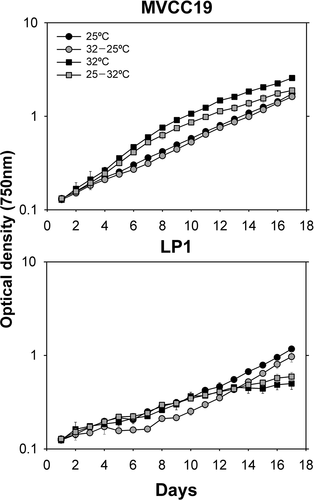
At 32°C, MVCC19 had higher growth rates than LP1, regardless of acclimation (p = 0.049, Z = 1.963). The growth rates of MVCC19 and LP1 were, respectively, 0.245 ±0.038 d–1 and 0.127 ±0.032 d–1 when acclimated, and 0.230 ±0.010 d–1 and 0.096 ±0.007 d–1 with no acclimation. Acclimation had no effect in either strain (p > 0.05).
Growth rate responses of strains to temperature and phosphate shifts
In experiment 2a, the cultures were submitted to a 22-fold P decrease in the medium through the dilution of cultures with P-free BG11 (see Supplementary fig. S1). In both strains only the acclimated cultures at 32°C entered exponential growth (). MVCC19 had a higher growth rate than LP1 (average: 0.381 ±0.003 d–1 and 0.232 ±0.009 d–1, MVCC19 and LP1, respectively; p = 0.049, Z = 1.963) and also entered the log phase earlier. In both strains, the next highest growth rates achieved were at 32°C without acclimation. One replicate of LP1 in this treatment kept growing, causing the increased variation compared with the other curves. Finally, LP1 showed a prolonged stationary phase when compared with MVCC19.
Fig. 2. Growth curves of experiment 2a (transition from 7 mg total P l−1 to 0.31 mg total P l−1). Temperature shifts were performed at time zero. The curve was obtained with the mean values (n=3, ± standard deviation; error bars are smaller than the size of the symbols if not visible) of optical density for each strain and temperature + phosphate shift. The dashed line separates the data before and after the 1:1 growth medium renewal.
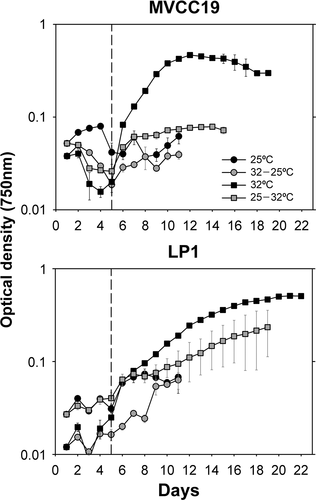
In experiment 2b, following 3-fold P reduction in the culture medium, both strains grew when acclimated at 32°C (), with no significant difference between the growth rates (p > 0.05; 0.137 ±0.069 d–1 and 0.139 ±0.003 d–1, MVCC19 and LP1, respectively). In this treatment, MVCC19 replicates were more variable than LP1 replicates (). Additionally, MVCC19 grew when shifted from 32 to 25°C (average: 0.112 ±0.017 d–1), and LP1 when acclimated at 25°C (0.113 ±0.006 d–1) and when shifted from 25 to 32°C (0.112 ±0.002 d–1). The LP1 replicates acclimated at 32°C had higher growth rates (p = 0.049, Z = 1.96) than non-acclimated treatments.
Fig. 3. Growth curve of the experiment 2b (transition from 1 mg total P l−1 phosphate sufficiency to 0.31 mg total P l−1). Temperature shifts were performed at time zero. The curve was obtained with the mean values (n=3, ± standard deviation; error bars are smaller than the size of the symbols if not visible) of optical density for each strain and temperature + phosphate shift. The dashed line separates the data before and after the 1:1 growth medium renewal.
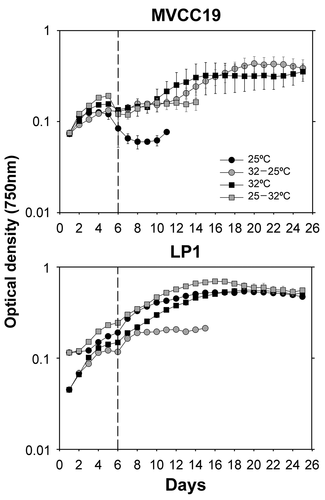
Effect of temperature and phosphate shifts on morphological traits
shows the median number of trichomes counted, the in vivo chlorophyll a fluorescence and the calculated biovolumes in experiment 2a. In this experiment, the sixth day was considered the beginning of exponential growth for both strains (see figures in Supplementary Material). MVCC19 had more trichomes than LP1 at the beginning (p < 0.05), but accumulated fewer trichomes at the end of the exponential phase, even with greater growth rate due to a shorter exponential phase. The same can be observed in in vivo chlorophyll a fluorescence and biovolume values (p < 0.05).
Table 1. Median values of trichome counts, in vivo chlorophyll a fluorescence (Chl a) measures and calculated total biovolume (BV) at initial and final exponential phase for acclimated strains at 32°C in experiment 2a
The morphological traits (i.e. length, width, number of heterocysts and number of cells per trichome) differed between strains (). LP1 had longer trichomes and more heterocysts and cells per trichome, hence greater biovolume, than MVCC19. MVCC19 varied less than LP1 between the initial and final days of exponential growth (). MVCC19 had higher numbers of trichomes with similar sizes and biovolume, and with fewer heterocysts. The temporal variation in the length of trichomes and the number of heterocysts is shown in . When analysing the trichomes according to the number of heterocysts, there was a clear pattern in increasing length and the number of heterocysts in LP1 cultures, but not in MVCC19 (; Nemenyi test, p < 0.05).
Fig. 4. Median (1st–99th percentiles) of the difference between the final and first day of exponential growth phase for several traits of LP1 and MVCC19 acclimated strains at 32°C. The difference between final and initial trait value was calculated for each replicate and for each strain by subtracting the summed value of 10 trichomes measured. Therefore, three values of trait difference were used for strain comparisons. Variation was considered non-significant when percentiles crossed zero. Type I error of Mann–Whitney tests comparing the trait changes between the strains are also shown. Significant p values are highlighted in bold.
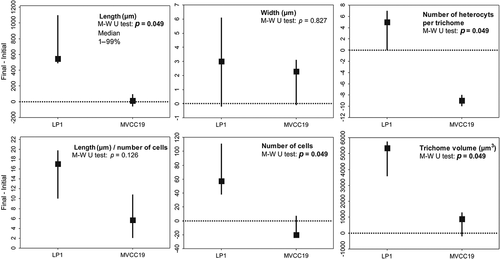
Discussion
This study experimentally demonstrated that high temperatures enhanced the ability of R. raciborskii to withstand phosphate deficiency, which helps to explain the success of this species in P-limited and warm environments. We provide support for the hypothesis that simultaneous changes in nutrient concentration and temperature affect growth more severely than changes in a single factor. The evidence of synergistic effects of temperature on the characteristic P-metabolism of R. raciborskii contributes to the understanding of the species’ success in relatively low P environments (Bonilla et al., Citation2012; Prentice et al., Citation2015), beyond the known physiological adjustments to low phosphate concentrations (Wu et al., Citation2012; Amaral et al., Citation2014) and large intracellular polyphosphate storage capacity (Istvánovics et al., Citation2000). In addition, the marked differences between strains regarding growth kinetics, and the variable morphology, suggest that MVCC19 and LP1 are adapted to different lake conditions. The physiological flexibility and morphological variability of R. raciborskii found here reinforce the need to study the effect of concomitant acclimation to environmental factors in order to better understand growth behaviour and improve realistic predictive models.
Both strains were able to withstand a period of P-limitation only when acclimated at the highest temperature. Temperature, therefore, plays a decisive role in the capability of R. raciborskii to overcome stressful P-limiting conditions and thus avoid the collapse of the whole population. In agreement with our findings, similar relationships between nutrients and temperature were previously described for cyanobacteria and other phytoplankton groups (Ahlgren, Citation1987, Citation1988; Thrane et al., Citation2017). However, other studies reported a negligible effect of temperature under low P conditions (Ryan et al., Citation2017), possibly because a critical P-limiting condition may not have been reached. Furthermore, altered nutrient acquisition machinery due to the reduction in membrane fluidity by suboptimal temperatures was reported in marine cyanobacteria (Varkey et al., Citation2015), which can decrease photosynthetic efficiency (quantum yield of PSII Fv/Fm) and increase nutrient requirements for cell maintenance. The increase in nutrient requirements as temperature decreases could also explain the reduction in thermal tolerance of R. raciborskii strains under N deficiency found by Thomas & Litchman (Citation2016). In addition, low temperatures can increase the susceptibility to photoinhibition (Mehnert et al., Citation2012; Kovács et al., Citation2016). Nonetheless, Várkonyi et al.(Citation2000) reported higher tolerance to photoinhibition in R. raciborskii at 25°C than at 35°C, due to more polyunsaturated fatty acids at the lower temperature. Further, Bonilla et al. (Citation2012) found that at high light intensity (135 µmol photons m–2 s–1) and warm temperatures, the growth rate of R. raciborskii was higher than that of Planktothrix agardhii. The greater effect of temperature together with the high light intensity could be a competitive advantage in environments with abiotic conditions (Bonilla et al., Citation2012; Prentice et al., Citation2015). All these findings show the complexity of R. raciborskii growth when considering concomitant variations in temperature ranges, light intensities and nutrient conditions, in addition to the acclimation ability and strain environmental adequacy.
The physiological flexibility and morphological variability between strains found here are in line with previous studies, suggesting that R. raciborskii strains have distinct growth rates, morphology and light acclimation (Piccini et al., Citation2011; Amaral et al., Citation2014; Pierangelini et al., Citation2014; Willis et al., Citation2016; Miotto et al., Citation2017) under different temperatures (Chonudomkul et al., Citation2004) and nutrient concentrations (Amaral et al., Citation2014; Thomas & Litchman, Citation2016). Besides the physiological flexibility, the strain differences may help explain how the species withstands P limitation in warmer environments and its expansion capacity in different lake conditions.
The morphological and physiological differences found between strains are still not completely understood. Recently, Willis et al. (Citation2018) compared the whole genome of nine strains from the same water sample and found that the genes responsible for nutrient acquisition were in a genome region common to all strains. Thus, differences in gene copies and expression might be responsible for strain differentiation (Willis et al., Citation2018). In our case, despite cultivation of the two strains for at least one year under the same laboratory conditions, they still responded differently when subjected to the same experimental settings (phosphorus limitation and temperature changes). For instance, MVCC19 grew through producing short filaments (relative to LP1) and few heterocysts (, ), which suggest that, compared with LP1, MVCC19 resembles an ‘r strategist’ (Reynolds, Citation2006) and exhibits invasive behaviour. In addition, MVCC19 is able to increase pigment content, a photosynthetic apparatus adjustment demonstrated to occur in other R. raciborskii strains that results in the improvement of light absorption (Mehnert et al., Citation2012; Pierangelini et al., Citation2014, Citation2015). Therefore, the small trichomes, short lag period and higher growth rates of MVCC19 allow it to overcome fluctuations in light and nutrient availabilities, and short-term favourable growth periods.
On the other hand, the larger trichomes of LP1 can favour buoyancy (Reynolds et al., Citation1987; Padisák et al., Citation2003), and consequently exposure to high light, which can favour nitrogen fixing; and the reduced chlorophyll a content could be indicative of acclimation to high light conditions. Taken together, the more heterocysts per trichome produced in LP1 and the apparently higher pigment content in MVCC19 may reflect a trade-off between energy investment in pigment production or N2 fixing, given the high cost of the last process (Kenesi et al., Citation2009; Yema et al., Citation2016; Burford et al., Citation2018). Conversely, increased chlorophyll a content has been associated with N2 fixing in order to produce the required ATP (Kenesi et al., Citation2009). The balance between these processes and the responses that each strain develops are not simple, and probably several successful strains co-occur in the same lake according to given environmental conditions. In fact, Miotto et al. (Citation2017) found even more larger trichomes than those of LP1 in another isolate from Lagoa do Peri (LP2). However, one can hypothesize that the larger filament length and lower photosynthetic pigment content of LP1 may be more suited to a stable, less enriched environment with higher light availability. Although we compared only two strains, MVCC19 and LP1 differences could be a result of the conditions in the lakes they originate from. Lago Javier (the origin of MVCC19) is subjected to more environmental variation in terms of temperature, trophic state and water column mixing compared with Lagoa do Peri (where LP1 was isolated) (see Bonilla et al., Citation2016 for an environmental description of Lago Javier; and Hennemann & Petrucio, Citation2011 and Brentano et al., Citation2016 for an environmental description of Lagoa do Peri). We advocate that hypotheses regarding the relationship between morphological features among strains and the original environments need to be further explored, using strains from numerous lakes of different trophic state and mixing regimes.
This study shows the positive effect of high temperature on the capability of R. raciborskii to withstand P-deficiency and the variation in strains regarding this growth condition. Our study may help to explain the persistence of R. raciborskii during summer stratified periods in which phosphate has been depleted to low or undetectable levels at the euphotic zone, and thus part of the population may survive periods of severe P-limiting conditions. In addition, the results reinforce the role of interacting factors which probably act synergistically to promote the success of this species. The combined positive effect of temperature on the tolerance of nutrient deficiency and the strain adequacy to lake conditions, may imply a drastic reduction in nutrient loads is required to avoid R. raciborskii dominance in warmer aquatic environments under restoration programmes.
Supplementary Information
The following supplementary material is accessible via the Supplementary Content tab on the article’s online page at https://doi.org/10.1080/09670262.2019.1567824
Supplementary fig. S1. Summary of the experimental design.
Supplementary fig. S2. Averaged optical density and in vivo chlorophyll a fluorescence measured during experiment 1 (n = 3, ± standard deviation; error bars are smaller than the size of the symbols if not visible). Temperature shifts were performed at time zero.
Supplementary fig. S3. Averaged optical density data of experiment 1 (n = 3, ± standard deviation; error bars are smaller than the size of the symbols if not visible). Days selected for calculation of growth rates in grey. The selection of days was based on linear regression. Corresponding averaged growth rate is shown.
Supplementary fig. S4. Averaged optical density and in vivo chlorophyll a fluorescence measured during experiment 2a (n = 3, ± standard deviation; error bars are smaller than the size of the symbols if not visible). The dashed line separates the data before and after the 1:1 growth medium renewal. Temperature shifts were performed at time zero.
Supplementary fig. S5. Averaged optical density data of experiment 2a) (n = 3, ± standard deviation; error bars are smaller than the size of the symbols if not visible). Days selected for calculation of growth rates in grey. The selection of days was based on linear regression. Corresponding averaged growth rate is shown. The dashed line separates the data before and after the 1:1 growth medium renewal.
Supplementary fig. S6. Averaged optical density and in vivo chlorophyll a fluorescence measured during experiment 2b (n = 3, ± standard deviation; error bars are smaller than the size of the symbols if not visible). The dashed line separates the data before and after the 1:1 growth medium renewal. Temperature shifts were performed at time zero.
Supplementary fig. S7. Averaged optical density data of experiment 2b (n = 3, ± standard deviation; error bars are smaller than the size of the symbols if not visible). Days selected for calculation of growth rates in grey. The selection of days was based on linear regression. Corresponding averaged growth rate is shown. The dashed line separates the data before and after the 1:1 growth medium renewal.
Author contributions
All authors conceived the original idea and contributed to the final version of the manuscript. E. Galvanese and L. Aubriot designed and conducted the experiments. E. Galvanese and A. Padial performed statistical analysis. E. Galvanese drafted the manuscript.
Supplementary Material
Download MS Word (1.5 MB)Acknowledgements
All experiments and counts were executed in Universidad de la República, Sección de Limnología. We also thank F. Martigani for technical support and help in all experiments and Dr L.F. Fernandes for assistance and use of microscopes. Finally, we are grateful to the anonymous reviewers for their helpful comments and to Prof. John Raven FRS and Prof. Christine Maggs for valuable suggestions.
Disclosure statement
No potential conflict of interest was reported by the authors.
Additional information
Funding
References
- Aguilera, A., Gómez, E.B., Kaštovský, J., Echenique, R.O. & Salerno, G.L. (2018). The polyphasic analysis of two native Raphidiopsis isolates supports the unification of the genera Raphidiopsis and Cylindrospermopsis (Nostocales, Cyanobacteria). Phycologia, 57: 130–146.
- Ahlgren, G. (1987). Temperature functions in biology and their application to algal growth constants. Oikos, 49: 177–190.
- Ahlgren, G. (1988). Phosphorus as growth-regulating factor relative to other environmental factors in cultured algae. In Phosphorus in Freshwater Ecosystems (48) (Persson, G. & Jansson, M., editors), 191–210. Hydrobiologia, Dordrecht.
- Amaral, V., Bonilla, S. & Aubriot, L. (2014). Growth optimization of the invasive cyanobacterium Cylindrospermopsis raciborskii in response to phosphate fluctuations. European Journal of Phycology, 49: 134–141.
- Andersen, R.A. (2005). Algal Culturing Techniques. 1st Ed. Academic Press, London.
- Antunes, J.T., Leão, P.N. & Vasconcelos, V.M. (2015). Cylindrospermopsis raciborskii: review of the distribution, phylogeography, and ecophysiology of a global invasive species. Frontiers in Microbiology, 6.
- Aubriot, L., Bonilla, S. & Falkner, G. (2011). Adaptive phosphate uptake behaviour of phytoplankton to environmental phosphate fluctuations. FEMS Microbiology Ecology, 77: 1–16.
- Bonilla, S., Aubriot, L., Soares, M.C.S., González-Piana, M., Fabre, A., Huszar, V.L.M., Lürling, M., Antoniades, D., Padisák, J. & Kruk, C. (2012). What drives the distribution of the bloom-forming cyanobacteria Planktothrix agardhii and Cylindrospermopsis raciborskii? FEMS Microbiology Ecology, 79: 594–607.
- Bonilla, S., González-Piana, M., Soares, M.C.S., Huszar, V.L.M., Becker, V., Somma, A., Marinho, M.M., Kokociński, M., Dokulil, M., Antoniades, D. & Aubriot, L. (2016). The success of the cyanobacterium Cylindrospermopsis raciborskii in freshwaters is enhanced by the combined effects of light intensity and temperature. Journal of Limnology, 75: 606–617.
- Brentano, D.M., Giehl, E.L.H. & Petrucio, M.M. (2016). Abiotic variables affect STX concentration in a meso-oligotrophic subtropical coastal lake dominated by Cylindrospermopsis raciborskii (Cyanophyceae). Harmful Algae, 56: 22–28.
- Briand, J.F., Robillot, C., Quiblier-Llobéras, C., Humbert, J.F., Couté, A. & Bernard, C. (2002). Environmental context of Cylindrospermopsis raciborskii (Cyanobacteria) blooms in a shallow pond in France. Water Research, 36: 3183–3192.
- Briand, J., Leboulanger, C., Humbert, J., Bernard, C. & Dufour, P. (2004). Cylindrospermopsis raciborskii (Cyanobacteria) invasion at mid-latitudes: selection, wide physiological tolerance, or global warming? Journal of Phycology, 40: 231–238.
- Burford, M.A., Beardall, J., Willis, A., Orr, P.T., Magalhaes, V.F., Rangel, L.M., Azevedo, S.M.F.O.E. & Neilan, B.A. (2016). Understanding the winning strategies used by the bloom-forming cyanobacterium Cylindrospermopsis raciborskii. Harmful Algae, 54: 44–53.
- Burford, M.A., Willis, A., Chuang, A., Man, X. & Orr, P.T. (2018). Recent insights into physiological responses to nutrients by the cylindrospermopsin producing cyanobacterium, Cylindrospermopsis raciborskii. Journal of Oceanology and Limnology, 36: 1032–1039.
- Carey, C.C., Ibelings, B.W., Hoffmann, E.P., Hamilton, D.P. & Brookes, J.D. (2012). Eco-physiological adaptations that favour freshwater cyanobacteria in a changing climate. Water Research, 46: 1394–1407.
- Chase, J.M. (2010). Stochastic community assembly causes higher biodiversity in more productive environments. Science, 328: 1388–1391.
- Chonudomkul, D., Yongmanitchai, W., Theeragool, G., Kawachi, M., Kasai, F., Kaya, K. & Watanabe, M.M. (2004). Morphology, genetic diversity, temperature tolerance and toxicity of Cylindrospermopsis raciborskii (Nostocales, Cyanobacteria) strains from Thailand and Japan. FEMS Microbiology Ecology, 48: 345–355.
- Falkner, R., Priewasser, M. & Falkner, G. (2006). Information processing by cyanobacteria during adaptation to environmental phosphate fluctuations. Plant Signaling and Behavior, 1: 212–220.
- Hennemann, M.C. & Petrucio, M.M. (2011). Spatial and temporal dynamic of trophic relevant parameters in a subtropical coastal lagoon in Brazil. Environmental Monitoring and Assessment, 181: 347–361.
- Hillebrand, H., Dürselen, C., Kirschtel, D., Zohary, T. & Pollingher, U. (1999). Biovolume calculation for pelagic and benthic microalgae. Journal of Phycology, 35: 403–424.
- Hunter-Cevera, K.R., Neubert, M.G., Olson, R.J., Solow, A.R., Shalapyonok, A. & Sosik, H.M. (2016). Physiological and ecological drivers of early spring blooms of a coastal phytoplankter. Science, 354: 326–329.
- Istvánovics, V., Shafik, H.M., Présing, M. & Juhos, S. (2000). Growth and phosphate uptake kinetics of the cyanobacterium Cylindrospermopsis raciborskii (Cyanophyceae) in throughflow cultures. Freshwater Biology, 43: 257–275.
- Kenesi, G., Shafik, H.M., Kovács, A.W., Herodek, S. & Présing, M. (2009). Effect of nitrogen forms on growth, cell composition and N2 fixation of Cylindrospermopsis raciborskii in phosphorus-limited chemostat cultures. Hydrobiologia, 623: 191–202.
- Kovács, A.W., Présing, M. & Vörös, L. (2016). Thermal-dependent growth characteristics for Cylindrospermopsis raciborskii (Cyanoprokaryota) at different light availabilities: methodological considerations. Aquatic Ecology, 50: 623–638.
- Litchman, E. & Klausmeier, C.A. (2008). Trait-based community ecology of phytoplankton. Annual Review of Ecology, Evolution and Systematics, 39: 615–639.
- Lürling, M., Eshetu, F., Faassen, E.J., Kosten, S. & Huszar, V.L.M. (2013). Comparison of cyanobacterial and green algal growth rates at different temperatures. Freshwater Biology, 58: 552–559.
- Mehnert, G., Rücker, J., Nicklisch, A., Leunert, F. & Wiedner, C. (2012). Effects of thermal acclimation and photoacclimation on lipophilic pigments in an invasive and a native cyanobacterium of temperate regions. European Journal of Phycology, 47: 182–192.
- Miotto, M.C., Costa, L.D.F., Brentano, D.M., Nader, C., dos Santos Souza, L., Gressler, P.D., Laudares-Silva, R., Yunes, J.S., Barufi, J.B. & Rörig, L.R. (2017). Ecophysiological characterization and toxin profile of two strains of Cylindrospermopsis raciborskii isolated from a subtropical lagoon in Southern Brazil. Hydrobiologia, 802: 97–113.
- Moreira, C., Fathalli, A., Vasconcelos, V. & Antunes, A. (2015). Phylogeny and biogeography of the invasive cyanobacterium Cylindrospermopsis raciborskii. Archives of Microbiology, 197: 47–52.
- Mur, L.R., Skulberg, O.M. & Utkilen, H. (1999). Cyanobacteria in the environment. In Toxic Cyanobacteria in Water: A Guide to their Public Health Consequences, Monitoring and Management (Chorus, I. & Bartram, J.editors,), 25–54. World Health Organization, London.
- Padisák, J. & Istvánovics, V. (1997). Differential response of blue-green algal groups to phosphorus load reduction in a large shallow lake: Balaton, Hungary. SIL Proceedings, 1922–2010, 26: 574–580.
- Padisák, J., Soróczki-Pinté, E. & Rezner, Z. (2003). Sinking properties of some phytoplankton shapes and the relation of form resistance to morphological diversity of phytoplankton – an experimental study. Hydrobiologia, 500: 243–257.
- Piccini, C., Aubriot, L., Fabre, A., Amaral, V., González-Piana, M., Giani, A., Figueredo, C.C., Vidal, L., Kruk, C. & Bonilla, S. (2011). Genetic and eco-physiological differences of South American Cylindrospermopsis raciborskii isolates support the hypothesis of multiple ecotypes. Harmful Algae, 10: 644–653.
- Pierangelini, M., Stojkovic, S., Orr, P.T. & Beardall, J. (2014). Elevated CO2 causes changes in the photosynthetic apparatus of a toxic cyanobacterium, Cylindrospermopsis raciborskii. Journal of Plant Physiology, 171: 1091–1098.
- Pierangelini, M., Sinha, R., Willis, A., Burford, M.A., Orr, P.T., Beardall, J. & Neilan, B.A. (2015). Constitutive cylindrospermopsin pool size in Cylindrospermopsis raciborskii under different light and CO2 partial pressure conditions. Applied and Environmental Microbiology, 81: 3069–3076.
- Posselt, A.J., Burford, M.A. & Shaw, G. (2009). Pulses of phosphate promote dominance of the toxic cyanophyte Cylindrospermopsis raciborskii in a subtropical water reservoir. Journal of Phycology, 45: 540–546.
- Prentice, M.J., O’Brien, K.R., Hamilton, D.P. & Burford, M.A. (2015). High- and low-affinity phosphate uptake and its effect on phytoplankton dominance in a phosphate-depauperate lake. Aquatic Microbial Ecology, 75: 139–153.
- Recknagel, F., Orr, P.T. & Cao, H. (2014). Inductive reasoning and forecasting of population dynamics of Cylindrospermopsis raciborskii in three sub-tropical reservoirs by evolutionary computation. Harmful Algae, 31: 26–34.
- Reynolds, C.S. (2006). The Ecology of Phytoplankton. Cambridge University Press, Cambridge.
- Reynolds, C.S., Oliver, R.L. & Walsby, A.E. (1987). Cyanobacterial dominance: the role of buoyancy regulation in dynamic lake environments. New Zealand Journal of Marine and Freshwater Research, 21: 379–390.
- Ryan, C.N., Thomas, M.K. & Litchman, E. (2017). The effects of phosphorus and temperature on the competitive success of an invasive cyanobacterium. Aquatic Ecology, 51: 463–472.
- Sinha, R., Pearson, L.A., Davis, T.W., Burford, M.A., Orr, P.T. & Neilan, B.A. (2012). Increased incidence of Cylindrospermopsis raciborskii in temperate zones – is climate change responsible? Water Research, 46: 1408–1419.
- Sinha, R., Pearson, L.A., Davis, T.W., Muenchhoff, J., Pratama, R., Jex, A., Burford, M.A. & Neilan, B.A. (2014). Comparative genomics of Cylindrospermopsis raciborskii strains with differential toxicities. BMC Genomics, 15: 83.
- Stucken, K., Murillo, A.A., Soto-Liebe, K., Fuentes-Valdés, J.J., Méndez, M.A. & Vásquez, M. (2009). Toxicity phenotype does not correlate with phylogeny of Cylindrospermopsis raciborskii strains. Systematic and Applied Microbiology, 32: 37–48.
- Thomas, M.K. & Litchman, E. (2016). Effects of temperature and nitrogen availability on the growth of invasive and native cyanobacteria. Hydrobiologia, 763: 357–369.
- Thrane, J.-E., Hessen, D.O. & Andersen, T. (2017). Plasticity in algal stoichiometry: experimental evidence of a temperature-induced shift in optimal supply N:P ratio. Limnology and Oceanography, 62: 1346–1354.
- Varkey, D., Mazard, S., Ostrowski, M., Tetu, S.G., Haynes, P. & Paulsen, I.T. (2015). Effects of low temperature on tropical and temperate isolates of marine Synechococcus. ISME Journal, 10: 1252.
- Várkonyi, Z., Zsiros, O., Farkas, T., Garab, G. & Gombos, Z. (2000). The tolerance of cyanobacterium Cylindrospermopsis raciborskii to low-temperature photo-inhibition affected by the induction of polyunsaturated fatty acid synthesis. Biochemical Society Transactions, 28: 892–894.
- Willis, A., Adams, M.P., Chuang, A.W., Orr, P.T., O’Brien, K.R. & Burford, M.A. (2015). Constitutive toxin production under various nitrogen and phosphorus regimes of three ecotypes of Cylindrospermopsis raciborskii ((Wołoszyńska) Seenayya et Subba Raju). Harmful Algae, 47: 27–34.
- Willis, A., Chuang, A.W., Woodhouse, J.N., Neilan, B.A. & Burford, M.A. (2016). Intraspecific variation in growth, morphology and toxin quotas for the cyanobacterium, Cylindrospermopsis raciborskii. Toxicon, 119: 307–310.
- Willis, A., Woodhouse, J., Ongley, S., Jex, A., Burford, M. & Neilan, B. (2018). Genome variation in nine co-occurring toxic Cylindrospermopsis raciborskii strains. Harmful Algae, 73: 157–166.
- Wu, Z., Zeng, B., Li, R. & Song, L. (2012). Physiological regulation of Cylindrospermopsis raciborskii (Nostocales, Cyanobacteria) in response to inorganic phosphorus limitation. Harmful Algae, 15: 53–58.
- Yema, L., Litchman, E. & de Tezanos Pinto, P. (2016). The role of heterocytes in the physiology and ecology of bloom-forming harmful cyanobacteria. Harmful Algae, 60: 131–138.