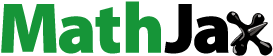
ABSTRACT
Kelps are globally important bioengineering species with high ecological and economic value, but are increasingly threatened by climate-driven geographic range shifts. The inheritance of economically important traits from parents to offspring is poorly understood in kelps but it is of utmost interest to seaweed farmers wishing to select strains with superior performance and resilience to environmental change. For two allopatric kelp species (N-Atlantic Laminaria digitata and S-Atlantic L. pallida), we compared the speed of gametogenesis and reproductive success in parental gametophytes, and produced intraspecific and reciprocal interspecific crosses of female × male gametophyte parents isolated from the two species. We then compared the upper thermal resilience of microscopic and macroscopic sibling sporophytes in an exposure experiment over two weeks. The upper thermal limit of the sporophytes resulting from intraspecific crosses of the two species deviated by 1°C. In contrast, sporophytes from both interspecific hybrid crosses had a 2–3°C higher upper thermal tolerance than single species sporophytes, indicating heterosis for thermal tolerance. This phenotypic response appears partially sex-dependent in our study, with female parents being more important in determining the thermal-response phenotype than male parents. The presence of male gametophytes generally enhanced female reproductive success. Both gametogenesis rate and reproductive success differed among the types of reciprocal crosses. Although the interspecific crosses were artificial in an ecological sense, they may provide a tool for understanding the molecular basis of heterosis and thermal tolerance in kelps (e.g. by investigating species-specific gene expression), or for aquaculture breeding programmes against a background of rapid environmental change.
Introduction
Hybridization between individuals from different species or from genetically distinct populations might produce hybrid offspring with increased or reduced fitness (Barton & Hewitt, Citation1985). Heterosis (or hybrid vigour), the superior phenotypic performance of the first generation hybrid offspring relative to their parents (Shull, Citation1908), has an ancestral role in the fitness of natural populations by providing genetic variation to adapt to environmental changes (Lippman & Zamir, Citation2007). Thus, heterosis has been widely explored in agriculture and proven to be an efficient way to develop crops and livestock hybrids with increased productivity (Longin et al., Citation2012; Fu et al., Citation2014), contributing to world food production.
Global warming has become a serious worldwide threat to biodiversity and ecosystems, imposing negative effects on food production (Lunt et al., Citation2016). Breeding programmes searching for heterosis in thermal traits for developing heat-tolerant hybrids are in high demand (Driedonks et al., Citation2016). This has already been exploited in some crops such as wheat (Farooq et al., Citation2011), cotton (Song et al., Citation2015), maize (Gazala et al., Citation2017), canola (Koscielny et al., Citation2018) and recently also in some kelp species (Zhang et al., Citation2011; Zhao et al., Citation2016).
Brown algae of the order Laminariales (kelps) play important habitat-structuring roles in the marine environment throughout temperate and polar regions worldwide, providing a variety of goods and services that are of high ecological, social and economic importance (Steneck et al., Citation2002; Bennett et al., Citation2016). However, coastal kelp forests are currently threatened by multiple anthropogenic factors, including ocean warming (e.g. Bartsch et al., Citation2013; Wernberg et al., Citation2013).
In contrast to Asia where 99% of seaweed production for commercial use is derived from aquaculture (FAO, Citation2016), non-Asian countries mainly exploit seaweeds by harvesting wild stocks (Buschmann et al., Citation2017; Charrier et al., Citation2017). As increasing current and future demand outstrips the supply from natural resources, aquaculture production is an excellent approach to cope with the growing market requirements for algal biomass, protecting natural kelp beds from overexploitation (Duarte et al., Citation2009; Mazarrasa et al., Citation2014). However, research into breeding brown algal strains for aquaculture lags far behind that of land plants, although algae have been traditionally cultivated in Asia for centuries (Dillehay et al., Citation2008; Buchholz et al., Citation2012). Since strong hybrid vigour is usually observed in crosses between individuals of different species (Fu et al., Citation2014), interspecific hybridization has developed as an effective strategy for producing heterotic hybrids between Saccharina spp. with desirable agronomic traits, greatly promoting the development of kelp cultivation in China (Zhang et al., Citation2007; Li et al., Citation2007, Citation2008a). Heterosis breeding strategies have been applied with success in kelp mariculture to obtain hybrids with increased thermal tolerance (Li et al., Citation2008a; Zhang et al., Citation2011). For example, the daily loss of blades was lower in the high temperature resistant hybrid between ‘Yuanza No. 10’ (hybrid between S. japonica and S. latissima) and ‘Fujian’ (S. japonica) than in the respective non-hybrid control cultivars; growth continued until seawater temperature reached 18–21°C, compared with about 15°C in the control (Zhang et al., Citation2011).
To explore the application of heterotic hybrids with improved upper thermal tolerance, a thorough understanding of the transmission mode of the thermal traits from parent to offspring is essential, both to establish an effective method for parental selection and to guide future mechanistic analyses of the genetic/genomic bases of tolerance. However, knowledge regarding the inheritance patterns of thermal tolerance is very scarce in kelps and macroalgae in general. As in land plants, most studies in Laminariales have focused on the transmission of morphological traits. Sporophyte length was maternally inherited in reciprocal crosses of L. digitata × L. abyssalis (tom Dieck & de Oliveira, Citation1993), while blade width was paternally inherited in hybrids between S. longicruris and S. latissima (=L. longicruris and L. saccharina; Lüning et al., Citation1978), and between S. longissima and S. japonica (=L. longissima and L. japonica; Zhang et al., Citation2007). Crosses between the non-bullate and bullate forms of S. latissima and S. longicruris revealed that the presence of bullae is genetically dominant and not sex-linked, as hybrids always possessed bullae (Lüning et al., Citation1978; Bolton et al., Citation1983).
The South Atlantic species Laminaria pallida Greville ex J. Agardh occurs in the upper subtidal along the South African and Namibian coastlines (Dieckmann, Citation1980; Stegenga et al., Citation1997), while the North Atlantic species L. digitata (Hudson) J.V. Lamouroux occurs from the Arctic to cold-temperate southern Brittany, France and Massachusetts, USA in the NE and NW Atlantic, respectively (Lüning, Citation1990). Although allopatric in their distribution, both species are still able to hybridize and form viable F1 sporophytes in culture (tom Dieck & de Oliveira, Citation1993). In addition, the upper survival limit reportedly deviates by 1°C (L. digitata: 21°C, L. pallida: 22°C; tom Dieck, Citation1992; tom Dieck & de Oliveira, Citation1993). Thus, these two species were selected as model organisms to study the inheritance of thermal traits. To accomplish this, we compared rates of gametogenesis, reproductive success and thermal resilience of microscopic and macroscopic F1 sporophyte offspring resulting from intraspecific and interspecific reciprocal crosses using female and male gametophyte parents isolated from the two species.
Materials and methods
Algal material
Unialgal clonal female and male gametophytes of Laminaria digitata, isolated from Helgoland, North Sea (AWI seaweed culture collection: ♂ 3157, ♀ 3158) and of L. pallida, isolated from Oudekraal, South Africa (AWI seaweed culture collection: ♂ 3378, ♀ 3379; as in tom Dieck & de Oliveira, Citation1993) were maintained in a vegetative state at 4°C and 15°C, respectively. Irradiance was set to 3 μmol photons m–2 s–1 of red light (LED Mitras daylight 150 controlled by ProfiLux 3, GHL Advanced Technology, Kaiserslautern, Germany), 16:8 h light:dark (LD) cycle, in sterile full strength Provasoli enriched seawater (PES; Provasoli, Citation1968). Female and male gametophyte material from both species was acclimated for six days to 8, 12 and 15°C (± 0.5°C) before the start of the experiments.
For experiments 1–3, vegetative male and female gametophytes of L. digitata and L. pallida were gently fragmented using a pestle and mortar, sieved (diameter = 50 and 100 µm) and suspended in half-strength PES to produce stock solutions of gametophytes with lengths of 50–100 µm. These were used to set up intraspecific (L. digitata ♂ × ♀; L. pallida ♂ × ♀) and reciprocal interspecific crosses (L. digitata ♀ × L. pallida ♂; L. pallida ♀ × L. digitata ♂), as well as ‘female unisexual’ cultures (Supplementary table S1) in each temperature. Each multicellular fragment was considered as one separate gametophyte irrespective of size.
Experiment 1: Gametogenesis and reproductive success
Intraspecific and interspecific crosses with a gametophyte density of ~400 multicellular gametophytes cm–2, were prepared from gametophyte stock solutions. Material was sown into five replicate Petri dishes (5.3 cm diameter, height 1.5 cm), filled with 12 ml of half-strength PES, and subjected to 8, 12 and 15°C (± 0.5°C) which spans the optimal temperature for gametogenesis in L. pallida (tom Dieck & de Oliveira, Citation1993) and L. digitata (tom Dieck, Citation1992; Martins et al., Citation2017). Irradiance was set to 15 μmol photons m–2 s–1 (white LED light; MITRAS lightbars 150 Daylight, GHL Advanced Technology, Kaiserslautern, Germany) measured with a LI-COR LI-185B Photometer (LI-COR-Biosciences, Lincoln, NE, USA) in a 16:8 h LD cycle. Cultures containing only female gametophytes (L. digitata ♀; L. pallida ♀) were used to track the rate of gametogenesis in the absence of males and the development and morphological status of parthenogenetic sporophytes. The culture medium was changed every 10 days by the replacement of 5 ml of half-strength PES per Petri dish.
1a: Quantification of ontogenetic stages: The relative presence of four ontogenetic stages (1: vegetative gametophytes, 2: gametophytes with oogonia, 3: gametophytes with released eggs, 4: gametophytes with attached sporophytes) was quantified every 7 days over a period of 21 days in ≥ 300 female gametophytes per replicate (n = 5) using an Olympus CKX41 inverted microscope (Olympus Co., Tokyo, Japan). For each female gametophyte the latest developmental stage was recorded if at least one cell per multicellular gametophyte had entered the respective developmental stage. Juvenile sporophytes were differentiated from released eggs if a first cell division was visible.
1b: Reproductive success: The reproductive success of female gametophytes was evaluated as follows: (1) percentage of female gametophytes with sporophytes after 21 days; (2) absolute number of sporophytes with normal morphology per female gametophyte after 33 days; and (3) absolute number of sporophytes with irregular morphology per female gametophyte after 33 days and possible partheno-sporophytes (sensu tom Dieck Citation1992). Female gametophyte density was counted after 21 days. Sporophytes with regular morphology (regular cell divisions, clear polar differentiation into basal rhizoid and proximal elongated blade), which normally stay attached to the respective female oogonium, were considered to represent fertilized diploid sporophytes. Conversely, unattached sporophytes with irregular morphology and often with missing rhizoids were considered to represent unfertilized partheno-sporophytes (sensu tom Dieck Citation1992, fig 9–14) that would not fully develop into healthy sporophytes and thus represent unsuccessful recruits. The sporophytes were counted in 40 fields of view (100× magnification) per replicate with an Olympus CKX41 inverted microscope.
Experiment 2: Thermal tolerance of microscopic F1 sporophytes
Four replicate Petri dishes, containing four cover slips each, were prepared per cross as described for experiment 1 and maintained at 12°C. When microscopic sporophytes had developed after 28 days and with length ~130 µm, cover slips inside each Petri dish were transferred individually to four larger Petri dishes (8.9 cm diameter, height 3.5 cm) containing 100 ml of half-strength PES. Three sets of four Petri dishes per cross were acclimated at 17°C for one day before transfer to the three upper target temperatures (21, 22 and 23°C ± 0.1°C), one control set consisting of four Petri dishes was left at 12°C (all n = 4 Petri dishes containing one cover slip each). The experiment lasted 13 days plus six days of recovery at 12°C. The upper temperatures of 21 and 22°C were chosen for experiment 2, and were considered to represent the upper survival temperatures for the L. digitata and L. pallida strains, respectively (tom Dieck, Citation1992, tom Dieck & de Oliveira, Citation1993). Experiments were conducted in temperature-controlled water-baths (Huber Variostat with Pilot ONE, Offenburg, Germany), provided with 15 μmol photons m–2 s–1 white LED light in a 16:8h LD photoperiod. The PES medium was replaced 75% weekly.
Sporophyte density: To assess the survival capacity of intraspecific and reciprocal interspecific F1 sporophytes to temperature, the absolute number of normal and malformed sporophytes per cm2 was evaluated at days 0, 6 and 13 and after recovery at 12°C. Forty fields of view were analysed per replicate using an inverted microscope representing ~0.4 cm2. Sporophyte density data were normalized as a proportion of the initial values.
Cultivation phase for experiment 3
After 33 days at 12°C (end of experiment 1) the sporophytes of all crosses were detached from the small Petri dishes (5.3 cm diameter, height 1.5 cm), transferred to larger Petri dishes (8.9 cm diameter, height 3.5 cm) and maintained under the same culture conditions. In addition, partheno-sporophytes arising from female gametophyte cultures (L. digitata ♀ and L. pallida ♀) were also kept in the same conditions for comparative purposes. After three weeks, sporophytes were transferred to sterile aerated 5 l glass bottles and the irradiance was increased to 30–40 μmol photons m–2 s–1 white LED light for 14 weeks until sporophytes reached a size of 3–5 cm length. Sterile PES was regularly changed 1–2 times per week. This material was used in experiment 3.
Experiment 3: Parentage analysis and thermal tolerance of macroscopic F1-sporophytes
3a: Parentage analysis: To confirm the presence of both Laminaria genotypes in hybrid sporophytes, 18 macroscopic sporophytes derived from each of the intraspecific and reciprocal interspecific crosses of L. digitata and L. pallida as well as from ‘female unisexual’ gametophyte cultures (L. digitata ♀ and L. pallida ♀) were randomly selected (108 sporophytes in total) before the start of the thermal tolerance experiment. The sporophytes were cleaned with paper towels, flash-frozen in liquid nitrogen, lyophilized and used to amplify the microsatellite marker LolVVIV-23 (Coelho et al., Citation2014). This marker amplifies a fragment with species-specific length variation, allowing us to identify the presence of the two Laminaria genotypes in the hybrids from banding patterns on an agarose gel. Genomic DNA was extracted using the Nucleospin 96 Plant II kit (Macherey-Nagel Duren, Germany). Polymerase chain reaction (PCR) conditions were those by Coelho et al. (Citation2014).
3b: Thermal tolerance of macroscopic F1 sporophytes: Fourteen macroscopic sporophytes from each of the crosses (see Supplementary table S1) were transferred into each of the four replicated aerated beakers (1.6 l; half-strength PES) per target temperature (n = 4). After acclimation for one day at 17°C, beakers were exposed to 21, 22, 23 and 24°C ± 0.1°C for 13 days. One set of beakers was left at 12°C (control: approx. optimum temperature for growth). Fourteen sporophytes were added to each beaker at the beginning of the experiment, but only six sporophytes were present by the end due to sporophyte sampling during the course of the experiment for future transcriptomic studies. Culture medium was changed every 3–4 days. Since the microscopic sporophytes of L. pallida and both reciprocal interspecific crosses had survived 23°C in experiment 2, a 24°C treatment was added. The sporophytes of both reciprocal interspecific crosses also survived at 24°C, thus an additional experiment was performed at 25 and 26°C for 13 days. Tolerance to experimental temperatures was determined by quantifying survival and relative growth rate of sporophytes.
Relative growth rate: Sporophyte area and fresh weight (FW) of all crosses were quantified on day 0 and 13 and relative growth rates (RGR) of both parameters were estimated according to the following formula:
Digital photos (Canon EOS 550D digital camera, Canon, Tokyo, Japan) were processed with ImageJ software (Schneider et al., Citation2012). The average area of the 14 sporophytes was used to calculate the RGR in each replicate. To minimize potential dehydration stress during FW measurements, all sporophytes per beaker were pooled, and weighed at the same time after blotting. Individual weights were estimated by dividing by the number of sporophytes.
Estimation of heterosis level: The level of heterosis for temperature tolerance based on area- and FW-RGR was estimated for both reciprocal interspecific hybrid sporophytes by calculating mid-parent heterosis (MPH) and better-parent heterosis (BPH) (Hochholdinger & Hoeckerm, Citation2007). MPH is a measure of the degree by which the hybrids exceed expectations based on the average performance of both single species, while BPH compares hybrid trait values to the best performing single species. Absolute MPH was calculated as (hybrid – mp) and BPH as (hybrid – bp), where hybrid is the RGR value in each interspecific cross, mp is the mid-parent values (mean of the RGR values of the two parental species) and bp is the better-parent values.
Statistics
Percentage abundance of sporophytes after 21 days (experiment 1b), sporophyte density after 33 days (experiment 1b), the microscopic sporophyte survival after 13 days (experiment 2), RGR of the macroscopic sporophytes after 13 days of thermal treatment (experiment 3b), mid-parent and better-parent heterosis (experiment 3b) all failed the assumptions of normality and homoscedasticity. Therefore, they were analysed with PERMANOVA software (v.1.6; Department of Statistics, University of Auckland, New Zealand), which assumes neither normality nor homoscedasticity (Anderson, Citation2001; McArdle & Anderson, Citation2001). Data were analysed under a two-factor design, with temperature and cross as fixed factors. Post-hoc pairwise t-test comparisons were performed whenever a significant difference between treatments was found. As 24°C was lethal for macroscopic sporophytes of L. digitata, this temperature was excluded from the respective analysis (temperature × cross interaction) of relative growth rate based on area. However, the data from the remaining crosses at 24°C were analysed with cross as a fixed factor. Analyses were performed with Euclidian distances and 9999 permutations. Differences were considered significant at p < 0.05.
Results
Gametophyte reproductive success
The relative (day 21) and absolute (day 33) reproductive successes showed significant temperature × cross interactions (, ; PERMANOVA, F = 25.9, p = 0.0001 for relative reproductive success, F = 9.7, p = 0.0001 for absolute reproductive success of normal sporophytes). The highest reproductive success in intraspecific crosses occurred at 12°C and 15°C in L. digitata and at 12°C in L. pallida (Supplementary fig. S1, ). Reproductive success was significantly greater in L. pallida (~81% sporophytes) than in L. digitata (55%). Numbers of sporophytes per female gametophyte were greater at 8–12°C in L. pallida (), and 1.8-fold higher than in L. digitata. Malformed partheno-sporophytes only developed at low rates in female parental gametophyte cultures of both species and the rate was almost null at 15°C ().
Table 1. Experiment 1. PERMANOVA for the effects of temperature on the presence of juvenile Laminaria sporophytes in: female gametophyte cultures (L. digitata ♀; L. pallida ♀), intraspecific (L. digitata ♀ × ♂; L. pallida ♀ × ♂) and reciprocal interspecific crosses (L. digitata ♀ × L. pallida ♂; L. pallida ♀ × L. digitata ♂) after 21 days.
Table 2. Experiment 1. PERMANOVA for the effects of temperature (8, 12 and 15°C) on the absolute number of (a) normal sporophytes per female gametophytes, (b) partheno-sporophytes per female gametophytes and (c) total sporophytes per female gametophytes developed from Laminaria: female gametophyte cultures (L. digitata ♀; L. pallida ♀), intraspecific (L. digitata ♀ × ♂; L. pallida ♀ × ♂) and reciprocal interspecific crosses (L. digitata ♀ × L. pallida ♂; L. pallida ♀ × L. digitata ♂) after 33 days.
Fig. 1. Experiment 1. Effect of temperature on the formation of juvenile sporophytes in female gametophyte cultures (L. digitata ♀; L. pallida ♀), intraspecific (L. digitata ♀ × ♂; L. pallida ♀ × ♂) and reciprocal interspecific crosses (L. digitata ♀ × L. pallida ♂; L. pallida ♀ × L. digitata ♂). (A) Percentage of female gametophytes with juvenile sporophytes after 21 days. (B) Absolute number of normal sporophytes per female gametophytes after 33 days. (C) Absolute number of loose lying and/or abnormal sporophytes considered to represent partheno-sporophytes per female gametophytes after 33 days. (D) Absolute number of normal sporophytes and partheno-sporophytes together per female gametophytes after 33 days. Box plots with median, boxes for 25th and 75th percentiles and whiskers indicating min and max values (n = 5). At each temperature, different lower case letters above boxplot bars indicate significant differences between all crosses (p < 0.05). Within each cross, different upper case letters indicate differences between temperatures (p < 0.05). See and for statistics.
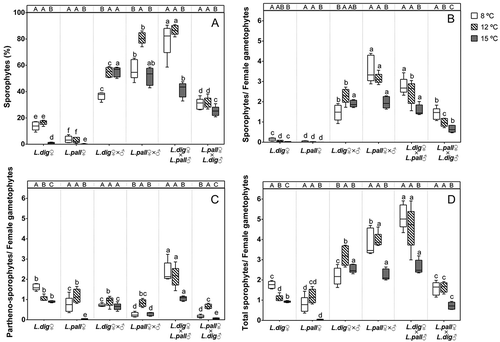
Reproductive success of reciprocal interspecific crosses was greatest at 8–12°C but rates differed. The cross L. digitata ♀ × L. pallida ♂ had a significantly higher reproductive success than L. pallida ♀ × L. digitata ♂ in relative and absolute measures (Supplementary fig. S1, , ). Interestingly, the number of malformed sporophytes, which are interpreted as unfertilized partheno-sporophytes, was also significantly higher in L. digitata ♀ × L. pallida ♂ than in any other cross, thereby contributing to the high overall rate (, ).
Comparing reproductive success of intraspecific and interspecific crosses we observed that the presence of L. pallida males significantly increased the total production of sporophytes at 8 and 12°C () while the presence of L. digitata males significantly decreased the total sporophyte production at 12 and 15°C () in the respective intraspecific compared with interspecific crosses with the same female parent. Overall, our results suggest that the origin of the male gametophyte strain has a strong effect on the reproductive success of the crosses, with L. pallida males inducing a higher success rate than L. digitata males.
Thermal tolerance of microscopic sporophytes
Sporophyte densities (normal and malformed morphologies estimated separately) were determined after six and 13 days exposure to experimental temperatures, and after six days recovery (). Statistical analysis was performed at a single time point (day 13) and indicated a significant cross × temperature interaction (; PERMANOVA, F = 10.9, p = 0.0001 for normal sporophytes, F = 3.6, p = 0.0011 for malformed sporophytes).
Table 3. Experiment 2. PERMANOVA for the effects of temperature (12, 21, 22 and 23°C) on the normalized density of (a) normal microscopic sporophytes, and (b) malformed microscopic sporophytes of intraspecific (L. digitata ♀ × ♂; L. pallida ♀ × ♂) and reciprocal interspecific crosses (L. digitata ♀ × L. pallida ♂; L. pallida ♀ × L. digitata ♂) after 13 days.
Fig. 2. Experiment 2. Effect of temperature on the density of normal microscopic sporophytes (A, B and C) and malformed sporophytes (D, E and F) of intraspecific (L. digitata ♂ × ♀; L. pallida ♂ × ♀) and reciprocal interspecific crosses (L. digitata ♀ × L. pallida ♂; L. pallida ♀ × L. digitata ♂) after 6 and 13 days of temperature treatment and 6 days of recovery at 12°C. Box plots with median, boxes for 25th and 75th percentiles and whiskers indicating min and max values (n = 4). Density values were normalized to the respective initial value for each cross (adjusted mean = 1). Only data from day 13 were statistically analysed. At each temperature, lower case letters above boxplot bars indicate differences between all crosses (p < 0.05). Within each cross, different upper case letters indicate differences between temperatures (p < 0.05). See for statistics.
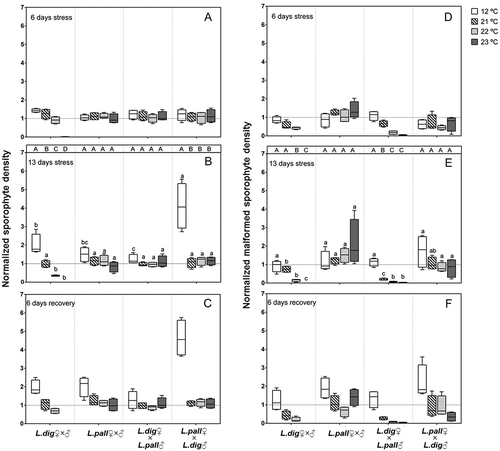
In L. digitata normalized sporophyte densities significantly declined at 22–23°C, while this was not the case for L. pallida or reciprocal interspecific crosses. Thus, over this range of sublethal temperatures, the presence of the warmer-adapted L. pallida genome in crosses (with either male or female origin) clearly conferred L. pallida-like thermal tolerance on hybrids containing the L. digitata genome. No sporophytes survived in L. digitata at 23°C and severe die-back was already evident after six days at 23°C (, ). In all crosses sporophyte densities increased at 12°C, with different rates, after 13 days indicating continuous formation of sporophytes. This was particularly pronounced in the cross L. pallida ♀ × L. digitata ♂ (, ). At the higher temperatures (21–23°C), further maturation seems to be prevented, and sporophyte densities reflect survival rather than additional development.
In contrast to the normal sporophytes from the interspecific cross L. digitata ♀ × L. pallida ♂ (–), the malformed sporophytes from the same cross (probably L. digitata ♀ partheno-sporophytes) showed the lowest thermal tolerance characteristic of L. digitata (–).
Parentage analysis of macroscopic sporophytes
Both intraspecific and reciprocal interspecific crosses produced healthy and morphologically normal sporophytes, while both ‘female unisexual’ cultures produced low numbers of malformed partheno-sporophytes with stunted and irregular morphologies often lacking holdfast development ().
Fig. 3. Morphology of macroscopic sporophytes derived from female gametophyte cultures resulting in malformed partheno-sporophytes (L. digitata ♀; L. pallida ♀), and of intraspecific (L. digitata ♂ × ♀; L. pallida ♂ × ♀) and reciprocal interspecific crosses (L. digitata ♀ × L. pallida ♂; L. pallida ♀ × L. digitata ♂) with normal shape. All sporophytes were grown at 12°C for 14 weeks. Scale bar = 1 cm (applies to all images).
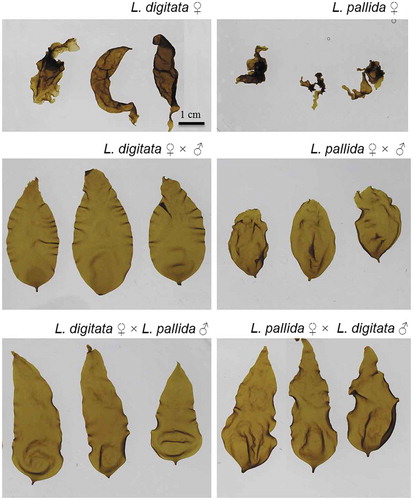
Genomic DNA from partheno-sporophytes and sporophytes of L. digitata generated a single band of smaller size, and those of L. pallida produced a single band of larger size (). The presence of the genomes of both species in the hybrids L. digitata ♀ × L. pallida ♂ and L. pallida ♀ × L. digitata ♂ was confirmed by the presence of two bands of the expected sizes from each species in all 18 sporophytes sampled per cross (see for three examples per cross). This indicated that they were true hybrid sporophytes of L. digitata and L. pallida.
Fig. 4. Electrophoresis pattern of microsatellite marker LolVVIV-23 products amplified in sporophytes derived from female gametophyte cultures (L. digitata ♀; L. pallida ♀), intraspecific (L. digitata ♂ × ♀; L. pallida ♂ × ♀) and reciprocal interspecific crosses (L. digitata ♀ × L. pallida ♂; L. pallida ♀ × L. digitata ♂). Eighteen sporophytes per treatment were used to amplify the microsatellite marker, however only three individuals each are shown as examples.
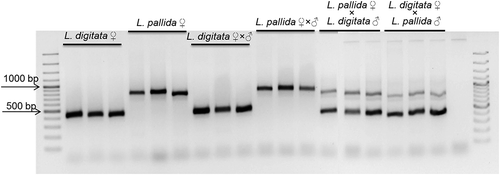
Thermal tolerance of macroscopic sporophytes
Relative growth rates (RGR) showed significant temperature × cross interactions (, ; PERMANOVA, F = 20.1, p = 0.0001 for area, F = 10.7, p = 0.0011 for FW). Area and FW-RGR were negatively affected in all crosses at 21, 22 and 23°C compared with controls at 12°C (, ).
Fig. 5. Experiment 3. Effect of temperature on the relative growth rate (RGR) based on area (A) or fresh weight (B) of macroscopic sporophytes derived from intraspecific (L. digitata ♂ × ♀; L. pallida ♂ × ♀) and reciprocal interspecific crosses (L. digitata ♀ × L. pallida ♂; L. pallida ♀ × L. digitata ♂) after 13 days. Box plots with median, boxes for 25th and 75th percentiles and whiskers indicating min and max values (n = 4). At each temperature, different lower case letters above boxplot bars indicate differences between all crosses (p < 0.05). Within each cross, different upper case letters indicate differences between temperatures (p < 0.05). Area-RGR at 24°C was excluded from the statistical analysis (temperature × cross interaction), since it was lethal for L. digitata sporophytes. The data at 24°C from the remaining crosses was analysed with cross as a fixed factor. See for statistics.
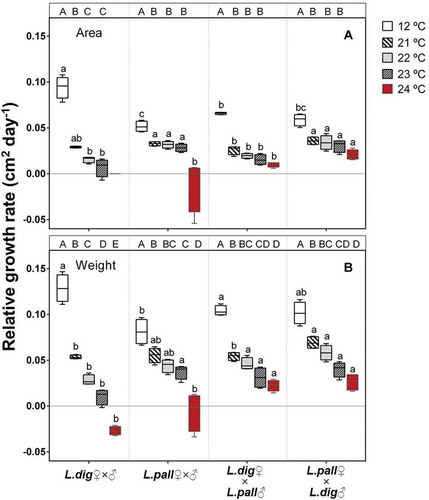
Table 4. Experiment 3. PERMANOVA for the effects of temperature (12, 21, 22, 23 and 24°C) on the area- and FW-RGR of macroscopic sporophytes of intraspecific (L. digitata ♀ × ♂; L. pallida ♀ × ♂) and reciprocal interspecific crosses (L. digitata ♀ × L. pallida ♂; L. pallida ♀ × L. digitata ♂) after 13 days.
Area and FW-RGR revealed two major results: (1) L. pallida has a significantly lower growth rate at 12°C but greater thermal tolerance than L. digitata. Neither species survived at 24°C (, ; ), but L. pallida maintained significantly higher area-RGR at 22–23°C, and FW-RGR at 23°C compared with L. digitata. (2) Reciprocal interspecific crosses showed clear evidence of heterosis in thermal tolerance, as both hybrid sporophytes grew at 24°C but significantly less than at 21–23°C (, ; ). Both hybrid sporophytes also survived 25°C and only died at 26°C, thereby surpassing the thermal tolerance of the intraspecific crosses by 2–3°C ().
Fig. 6. Experiment 3. Visual appearance of sporophytes from intraspecific (L. digitata ♂ × ♀; L. pallida ♂ × ♀) and reciprocal interspecific crosses (L. digitata ♀ × L. pallida ♂; L. pallida ♀ × L. digitata ♂) after 13 days of temperature treatment at 12, 21, 22, 23, 24, 25 and 26°C. Scale bar = 1 cm (applies to all images).
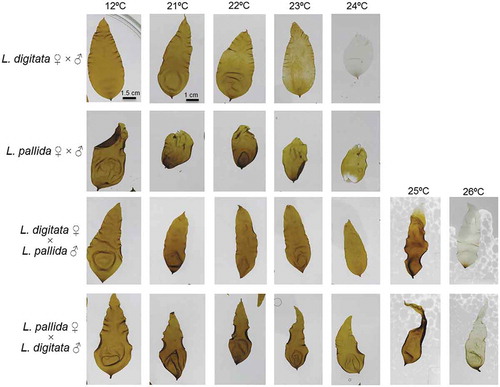
Fig. 7. Absolute mid-parent (A, B) and best-parent heterosis (C, D) values for the relative growth rate based on area or fresh weight of macroscopic sporophytes derived from reciprocal interspecific crosses (L. digitata ♀ × L. pallida ♂; L. pallida ♀ × L. digitata ♂) grown for 13 days at different temperatures. Box plots with median, boxes for 25th and 75th percentiles and whiskers indicating min and max values (n = 4). At each temperature, * above boxplot bars indicates differences between crosses (p < 0.05). Within each cross, different upper case letters indicate differences between temperatures (p < 0.05). See for statistics.
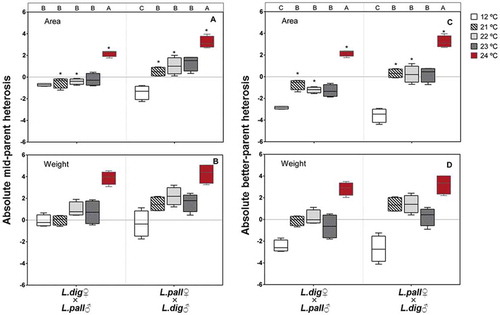
The area-RGR of reciprocal hybrid crosses over the 21–24°C range was significantly higher for L. pallida ♀ × L. digitata ♂ than for L. digitata ♀ × L. pallida ♂ sporophytes and the same as for intraspecific L. pallida. While this observation was only confirmed at 21°C for FW-RGR, this result suggests the presence of a female-specific component in thermal tolerance.
Mid-parent and better-parent heterosis (MPH and BPH) showed significant temperature × cross interactions when assessed for area-RGR (, ; PERMANOVA, F = 6.0, p = 0.0013 for mid-parent, F = 6.0, p = 0.0012 for better-parent). On the other hand, MPH and BPH differed significantly with crosses (; F = 8.5, p = 0.0072 for mid-parent, F = 8.5, p = 0.0079 for better-parent) and temperature (; F = 31.0, p = 0.0001 for mid-parent, F = 49.1, p = 0.0001 for better-parent) for FW-RGR. Both reciprocal hybrids displayed positive heterosis (MPH and BPH) for area- and FW-RGR at 24°C (). Additionally, the hybrid L. pallida ♀ × L. digitata ♂ also showed positive heterosis (MPH and BPH) for area-RGR and FW-RGR over the 21–23°C range. Overall, both heterosis values were higher in the hybrid L. pallida ♀ × L. digitata ♂ than in L. digitata ♀ × L. pallida ♂ at 21, 22 and 24°C for area-RGR and regardless of the temperature for FW-RGR. For both hybrids, the highest MPH and BPH values were observed at 24°C. MPH and BPH could not be calculated for 25 and 26°C as we only measured survival and not RGR in the additional experiment.
Table 5. Experiment 3. PERMANOVA for the absolute mid-parent (MPH) and best-parent heterosis (BPH) assessed on area- and FW-RGR of the reciprocal interspecific crosses (L. digitata ♀ × L. pallida ♂; L. pallida ♀ × L. digitata ♂) at different temperatures (12, 21, 22, 23 and 24°C) for 13 days.
Discussion
Four key findings can be taken from this study: (1) the reciprocal interspecific hybrids between the kelp species, L. digitata and L. pallida showed heterosis for upper thermal tolerance limits, surviving 2–3°C higher than the inbred single species. (2) The phenotypic response of hybrids under thermal stress may be sex-dependent, as hybrid area-RGR under thermal stress was more correlated with the genome of the female than the male parental species. (3) Male gametophytes influence the rate of gametogenesis and reproductive success in female gametophytes, indicating the presence of chemical signals arising from males that are detected by females. (4) Maintenance of clonal cultures over > 25 years in constant laboratory conditions only slightly changed the general response to temperature in the case of L. pallida (upper survival temperature increased 1°C).
In agreement with an earlier study (tom Dieck & de Oliveira, Citation1993), we were able to successfully hybridize Northern Atlantic L. digitata and Southern Atlantic L. pallida, as confirmed by parentage analysis using a microsatellite marker. Hybridization between congeneric kelp species is not uncommon under laboratory conditions (Alaria: Kraan & Guiry, Citation2000; Kraan et al., Citation2001; Saccharina: Bolton et al., Citation1983; Druehl et al., Citation2005; Li et al., Citation2007; Lessonia: Müller et al., Citation2018), and even occurs between genera of the same family (Macrocystis × Pelagophycus: Lewis & Neushul, Citation1995; Alaria marginata × Lessoniopsis littoralis: Liptack & Druehl, Citation2000). A recent phylogeographic study placed L. pallida and L. abyssalis from the South Atlantic, L. ochroleuca (North Atlantic) and the Mediterranean endemic L. rodriguezii in a common clade, while the Northern Atlantic species L. digitata and L. hyperborea formed a separate sister clade (Rothman et al., Citation2017). Although L. digitata is phylogenetically closest to L. hyperborea and both species occur in sympatry they do not hybridize (tom Dieck, Citation1992). On the other hand, successful hybridization to F1-sporophytes was observed between the allopatric species L. digitata and L. pallida and between L. digitata and L. abyssalis (this study; tom Dieck & de Oliveira, Citation1993). Allopatry may prevent reinforcement (selection on reproductively isolating mechanisms), while in the sympatric species L. digitata and L. hyperborea stronger reinforcement might be expected.
In L. digitata and L. pallida, and in both reciprocal crosses, the most rapid gametogenesis was observed at 12°C, which is in accordance with earlier studies showing that the optimum temperature range for gametogenesis of L. pallida is between 11°C and 17°C (tom Dieck & de Oliveira, Citation1993) and for L. digitata between 5°C and 15°C (tom Dieck, Citation1992; Martins et al., Citation2017).
Sexual reproduction in kelps is facilitated by the universal pheromone lamoxirene, secreted by eggs from the female gametophyte. Lamoxirene triggers spermatozoid release from antheridia and their attraction to the eggs (Lüning & Müller, Citation1978), improving mating efficiency by synchronizing sexual events leading up to syngamy. No pheromone production or chemical signalling by male gametophytes has hitherto been described in kelps. Surprisingly, in the current investigation gametogenesis in both species was enhanced when male gametophytes were cultivated together with females. We must therefore assume that bidirectional chemical communication occurs between both sexes. More rapid and higher fertility was observed in both species and their reciprocal crosses compared to the ‘female unisexual’ cultures. This provides a strong hint that the presence of male gametophytes, which are protandric (Hsiao & Druehl, Citation1971; Bartsch pers. comm.), positively influences female gametophyte reproductive success. Additionally, the signalling efficiency of male gametophytes was asymmetric between L. digitata and L. pallida. Male gametophytes of L. pallida promoted faster development of female gametophytes in intraspecific (L. pallida ♀ × ♂) and interspecific (L. digitata ♀ × L. pallida ♂) crosses than male gametophytes of L. digitata. This may be a result of intrinsic differences between the species (composition or concentration of signalling substances), differences in maturation time, or reduced vigour of the male L. digitata strain compared with the L. pallida strain used in these experiments.
The microscopic and macroscopic sporophytes of L. digitata and L. pallida showed similar upper thermal tolerances. The microscopic sporophytes of L. digitata died at 23°C, and only 35% survived at 22°C, while the macroscopic sporophytes survived at 22°C but with slight visible bleaching of the blade (). This upper survival temperature of 21–22°C is consistent with previous work from the same location (tom Dieck, Citation1992). Microscopic and macroscopic sporophytes of L. pallida survived at 23°C, but not at 24°C () which is 1°C higher than observed in an earlier study performed > 25 years earlier with the same clonal strains (tom Dieck & de Oliveira, Citation1993). Long-term maintenance of these gametophyte cultures thereby did not induce major changes in the general response of the resulting sporophytic material to temperature survival. The differences observed may also reflect methodological differences in the current study and the studies of tom Dieck. The temperature tolerance of juvenile sporophytes was used as proxy for mature sporophytes as large mesocosm systems were not available.
Parthenogenesis, development of an unfertilized egg, may occur in the absence of males or may be stimulated by a male (pseudogamy) (Beukeboom & Vrijenhoek, Citation1998). In our study, even in the presence of males, we detected a small percentages of microscopic sporophytes with deformations similar to those in ‘female unisexual’ cultures in all crosses. It was not possible to ascertain whether these sporophytes were a result of fertilization or not, but we assumed they were partheno-sporophytes (sensu tom Dieck Citation1992). The malformed sporophytes developing in L. pallida and the hybrid cross L. pallida ♀ × L. digitata ♂ survived 23°C, while those from L. digitata and the hybrid cross L. digitata ♀ × L. pallida ♂ died at 22°C (), suggesting their parthenogenetic origin. Previous studies showed that partheno-sporophytes of several Laminaria species exhibited 1–2°C lower upper temperature tolerance than the single species sporophytes (tom Dieck, Citation1992; tom Dieck & de Oliveira, Citation1993). However, in the current study, the microscopic partheno-sporophytes of L. digitata had the same thermal tolerance as the sporophytes of the parental species.
Because species of the order Laminariales are able to produce partheno-sporophytes in culture (Motomura & Sakai, Citation1981; Lüning et al., Citation1978; tom Dieck, Citation1992), crossing experiments within Laminariales require careful controls (Druehl et al., Citation2005). Most partheno-sporophytes of Laminaria spp. and Atlantic Saccharina spp. show a high mortality rate, and those that survive have an irregular-shaped morphology, no clear polar development and often fail to form rhizoids (Bolton et al., Citation1983; tom Dieck, Citation1992). However, in some kelp species adult fertile partheno-sporophytes with normal morphology develop (Fang et al., Citation1978; Shan et al., Citation2013). In this study, intraspecific and both reciprocal interspecific crosses of L. digitata and L. pallida mostly produced morphologically normal macroscopic sporophytes. These were different to partheno-sporophytes developing in female-only cultures, which had a stunted and irregular appearance and lacked holdfasts (). Although sex-specific markers have been developed for kelp species including L. digitata (Lipinska et al., Citation2015) to genetically discriminate between diploid sporophytes generated from sexual reproduction and female partheno-sporophytes, no amplification of sex-specific bands was possible in either L. digitata or L. pallida sporophytes using these PCR-based markers. However, the parentage study with a microsatellite marker successfully confirmed that sporophytes from both reciprocal crosses between L. digitata and L. pallida were true hybrids containing the genomes of both species.
For the first time in Laminaria, we show significant best parent heterosis (BPH) for heat tolerance in hybrids of reciprocal crosses between L. digitata and L. pallida. The upper survival temperature was 22°C for sporophytes of L. digitata and 23°C for L. pallida, while for both reciprocal hybrids it was 25°C showing an increase of 2–3°C in the thermal tolerance compared with the pure species. Heterosis breeding strategies have been applied with success in Laminariales mariculture in China to obtain hybrids tolerant to high temperatures, using intraspecific crossings between S. japonica cultivars (Li et al., Citation2016) and interspecific Saccharina crosses (S. japonica and S. longissima: Li et al., Citation2007; S. japonica and hybrid S. japonica × S. latissima: Zhang et al., Citation2011). Hybrids between the two South Atlantic species L. pallida and L. abyssalis also displayed a better temperature tolerance than the less tolerant single species (mid-parent heterosis: superior performance of the F1 hybrid in comparison with the mid-parent average) but no BPH (tom Dieck & de Oliveira, Citation1993). Best parent heterosis is one major target for aquaculture production due to its potential economic value (Gjedrem & Baranski, Citation2009). In the current investigation, we only worked with one pair of gametophytes for each species. Hybrid offspring generated from gametophytes spanning diverse locations across the species distribution range may lead to a diversity of heterosis responses (e.g. Westermeier et al., Citation2010).
Genetic distance between species or strains is an indicator of heterosis strength, with a positive correlation between genetic distance of the parental lines and the superior hybrid performance (Li et al., Citation2008b). The superior thermal tolerance of hybrids between L. pallida and L. digitata (our study) compared with those between L. pallida and L. abyssalis (tom Dieck & de Oliveira, Citation1993) could be attributed to the genetic distance between the species involved. L. pallida and L. abyssalis belong to the same phylogenetic clade while L. digitata belongs to a sister clade (Rothman et al., Citation2017).
Although both reciprocal hybrid crosses exhibited a similar heterosis for upper thermal tolerance limits, our results also provide intriguing evidence that the transmission of thermal traits may be partially sex-dependent, with female parents being more important in determining the thermal-response phenotype of hybrids than male parents. Pure L. pallida sporophytes had greater upper thermal limits and higher area-RGR at sub-lethal temperatures than L. digitata. The superior performance at sub-lethal temperatures of hybrid crosses was transmitted through the female genome, as L. pallida ♀ × L. digitata ♂ sporophytes grew faster at 21–24°C than those from the reciprocal cross. Qualitatively, the L. pallida ♀ × L. digitata ♂ cross also displayed the best performance at 25°C, where all siblings appeared healthy, while the hybrid L. digitata ♀ × L. pallida ♂ showed the onset of bleaching in the blade. The high temperature tolerant and high-yield hybrid ‘Rongfu’ was obtained through the cross between a male parent (hybrid S. japonica × S. latissima) from high-yield sporophytes and a female parent (S. japonica) with high temperature tolerance, supporting the idea that the thermal tolerance may be preferentially inherited from the female parent (Zhang et al., Citation2011). Similarly, hybrids between L. pallida and L. abyssalis inherited the sensitivity against low temperature from the female parent (tom Dieck & de Oliveira, Citation1993).
This study provides valuable insights regarding thermal tolerance inheritance in kelps that will help to integrate and improve heat-tolerance characteristics through breeding selection and thus benefit the kelp aquaculture industry in the face of a changing climate.
Supplementary information
The following supplementary material is accessible via the Supplementary Content tab on the article’s online page at https://doi.org/10.1080/09670262.2018.1613571
Supplementary Table S1. Overview of parental crosses, cultivation conditions during experiments and time course of experiments and parameter estimation. All cultures were maintained in a 16:8 h L:D regime and in half-strength Provasoli medium under LED light. For methodological details see text.
Supplementary Fig. S1. Experiment 1. Effect of temperature on gametogenesis stages of female gametophyte cultures (L. digitata ♀; L. pallida ♀), intraspecific (L. digitata ♀ × ♂; L. pallida ♀ × ♂) and reciprocal interspecific crosses (L. digitata ♀ × L. pallida ♂; L. pallida ♀ × L. digitata ♂) over time (7, 14, 21 days; mean values, n = 5). SE values are omitted for clarity.
Author contributions
N. Martins: original concept, experiments and data analysis, drafting and editing manuscript; G.A. Pearson: data analysis assistance, drafting and editing manuscript; L. Gouveia and A.I. Tavares: parentage analysis work; E.A. Serrão: editing manuscript; I. Bartsch: original concept, work supervision, editing manuscript.
TEJP-2018-0107-File010.tif
Download TIFF Image (448.2 KB)TEJP-2018-0107-File009.docx
Download MS Word (15.6 KB)Acknowledgements
We thank A. Wagner for maintaining the algal material and for help with laboratory facilities.
Disclosure statement
No potential conflict of interest was reported by the authors.
Additional information
Funding
References
- Anderson, M.J. (2001). A new method for non-parametric multivariate analysis of variance. Austral Ecology, 26: 32–46.
- Barton, N.H. & Hewitt, G.M. (1985). Analysis of hybrid zones. Annual Review of Ecology and Systematics, 16: 113–148.
- Bartsch, I., Vogt, J., Pehlke, C. & Hanelt, D. (2013). Prevailing sea surface temperatures inhibit summer reproduction of the kelp Laminaria digitata at Helgoland (North Sea). Journal of Phycology, 49: 1061–1073.
- Bennett, S., Wernberg, T., Connell, S.D., Hobday, A.J., Johnson, C.R. & Poloczanska, E.S. (2016). The ‘Great Southern Reef’: social, ecological and economic value of Australia’s neglected kelp forests. Marine and Freshwater Research, 67: 47–56.
- Beukeboom, L.W. & Vrijenhoek, R.C. (1998). Evolutionary genetics and ecology of sperm-dependent parthenogenesis. Journal of Evolutionary Biology, 11: 755–782.
- Bolton, J.J., Germann, I. & Lüning, K. (1983). Hybridization between Atlantic and Pacific representatives of the Simplices section of Laminaria (Phaeophyta). Phycologia, 22: 133–140.
- Buchholz, C.M., Krause, G. & Buck, B.H. (2012). Seaweed and man. In Seaweed Biology: New Insights into Ecophysiology, Ecology and Utilization (Wiencke. C. & Bischof, K., editors), 471–493. Springer, Berlin.
- Buschmann, A.H., Camus, C., Infante, J., Neori, A., Israel, A., Hernández-González, M.C., Pereda, S.V., Gomez-Pinchetti, J.L., Golberg, A., Tadmor-Shalev, N. & Critchley, A.T. (2017). Seaweed production: overview of the global state of exploitation, farming and emerging research activity. European Journal of Phycology, 52: 391–406.
- Charrier, B., Abreu, M.H., Araujo, R., Bruhn, A., Coates, J.C., De Clerck, O., Katsaros, C., Robaina, R.R. & Wichard, T. (2017). Furthering knowledge of seaweed growth and development to facilitate sustainable aquaculture. New Phytologist, 216: 967–975.
- Coelho, N.C., Serrão, E.A. & Alberto, F. (2014). Characterization of fifteen microsatellite markers for the Laminaria ochroleuca and cross species amplification within the genus. Conservation Genetics Resources, 6: 949–950.
- Dieckmann, G.S. (1980). Aspects of the ecology of Laminaria pallida (Grev.) J. Ag. off the Cape Peninsula (South Africa). Botanica Marina, 23: 579–585.
- Dillehay, T.D., Ramırez, C., Pino, M., Collins, M.B., Rossen, J. & Pino‐Navarro, J.D. (2008). Monte Verde: seaweed, food, medicine, and the peopling of South America. Science, 320: 784–786.
- Driedonks, N., Rieu, I. & Vriezen, W.H. (2016). Breeding for plant heat tolerance at vegetative and reproductive stages. Plant Reproduction, 29: 67–79.
- Druehl, L.D., Collins, J.D., Lane, C.E. & Saunders, G.W. (2005). An evaluation of methods used to assess intergeneric hybridization in kelp using Pacific Laminariales (Phaeophyceae). Journal of Phycology, 41: 250–262.
- Duarte, C.M., Holmer, M., Olsen, Y., Soto, D., Marbà, N., Guiu, J., Black, K. & Karakassis, I. (2009). Will the oceans help feed humanity? BioScience, 59: 967–976.
- Fang, T.C., Tai, J.H., Ou, Y.L., Tui, C.C. & Chen, T.C. (1978). Some genetic observations on the monoploid breeding of Laminaria japonica. Scientia Sinica, 21: 401–408.
- FAO (2016). Fisheries & Aquaculture – Fisheries and Aquaculture Fact Sheets. http://www.fao.org/fishery/factsheets/en [accessed 2 April 2018].
- Farooq, J., Khaliq, I., Ali, M.A., Kashif, M., Rehman, A., Naveed, M., Ali, Q., Nazeer, W. & Farooq, A. (2011). Inheritance pattern of yield attributes in spring wheat at grain filling stage under different temperature regimes. Australian Journal of Crop Science, 5: 1745–1753.
- Fu, D., Xiao, M., Hayward, A., Fu, Y., Liu, G., Jiang, G. & Zhang, H. (2014). Utilization of crop heterosis: a review. Euphytica, 197: 161–173.
- Gazala, P., Kuchnur, P.H., Zaidi, P.H., Arunkumar, B., Patil, A., Seetharam K. & Vinayan M.T. (2017). Combining ability and heterosis for heat stress tolerance in maize (Zea mays L.). Journal of Farm Sciences, 30: 326–333.
- Gjedrem, T. & Baranski, M. (2009). Selective Breeding in Aquaculture: an Introduction. Dordrecht, Springer.
- Hochholdinger, F. & Hoeckerm, N. (2007). Towards the molecular basis of heterosis. Trends in Plant Science 12: 427–432.
- Hsiao, S.I.C. & Druehl, L.D. (1971). Environmental control of gametogenesis in Laminaria saccharina. I. The effects of light and culture media. Canadian Journal of Botany, 49: 1503–1508.
- Koscielny, C.B., Garner, S.W. & Duncan, R.W. (2018). Impact of high temperature on heterosis and general combining ability in spring canola (Brassica napus L.). Field Crops Research, 221: 61–70.
- Kraan, S. & Guiry, M.D. (2000). Molecular and morphological character inheritance in hybrids of Alaria esculenta and A. praelonga (Alariaceae, Phaeoohyceae). Phycologia, 39: 554–559.
- Kraan, S., Rueness, J. & Guiry, M.D. (2001). Are North Atlantic Alaria esculenta and A. grandifolia (Alariaceae, Phaeophyceae) conspecific? European Journal of Phycology, 36: 35–42.
- Lewis, R.J. & Neushul, M. (1995). Intergeneric hybridization among five general of the family Lessoniaceae (Phaeophyceae) and evidence for polyploidy in a fertile Pelagophycus × Macrocystis hybrid. Journal of Phycology, 31: 1012–1017.
- Li, X.J., Cong, Y.Z., Yang, G.P., Shi, Y.Y., Qu, S.C., Li, Z.L., Wang, G.W., Zhang, Z.Z., Luo, S.J., Dai, H.L., Xie, J.Z., Jiang, G.L., Liu, J.L. & Wang, T.Y. (2007). Trait evaluation and trial cultivation of Dongfang no. 2, the hybrid of a male gametophyte clone of Laminaria longissima (Laminariales, Phaeophyta) and a female one of L. japonica. Journal of Applied Phycology, 19: 139–151.
- Li, X.J., Liu, J.L., Cong, Y.Z., Qu, S.C., Zhang, Z.Z., Dai, H.L., Luo, S.J., Han, X.B., Huang, S.S., Wang, Q.Y., Liang, G.J., Sun, J., Jin, Y., Wang, D.Q. & Yang, G.P. (2008a). Breeding and trial cultivation of Dongfang no. 3, a hybrid of Laminaria gametophyte clones with a more than intraspecific less than interspecific relationship. Aquaculture, 280: 76–80.
- Li, X., Yang, G., Shi, Y., Cong, Y., Che, S., Qu, S. & Li, Z. (2008b). Prediction of the heterosis of Laminaria hybrids with the genetic distance between their parental gametophyte clones. Journal of Applied Phycology, 20: 1097–1102.
- Li, X., Zhang, Z., Qu, S., Liang, G., Zhao, N., Sun, J., Song, S., Cao, Z., Li, X., Pan, J., Luo, S., Zhang, L., Cui, C., Peng, J., Li, Y., Wu, R., Zhao, J., Qian, R., Wang, L., Sai, S. & Yang, G. (2016). Breeding of an intraspecific kelp hybrid Dongfang no. 6 (Saccharina japonica, Phaeophyceae, Laminariales) for suitable processing products and evaluation of its culture performance. Journal of Applied Phycology, 28: 439–447.
- Lipinska, A.P., Ahmed, S., Peters, A., Faugeron, S., Cock, M. & Coelho, S. (2015). Development of PCR-based markers to determine the sex of kelps. PLoS ONE 10: e0140535.
- Lippman, Z.B. & Zamir, D. (2007). Heterosis: revisiting the magic. Trends in Genetics, 23: 60–66.
- Liptack, M.K. & Druehl, L.D. (2000). Molecular evidence for an interfamilial laminarialean cross. European Journal of Phycology, 35: 135–142.
- Longin, C.F.H., Mühleisen, J., Maurer, H.P., Zhang, H., Gowda, M. & Reif, J.C. (2012). Hybrid breeding in autogamous cereals. Theoretical and Applied Genetics, 125: 1087–1096.
- Lüning, K. (1990). Seaweeds: Their environment, Biogeography and Ecophysiology. John Wiley and Sons, New York.
- Lüning, K. & Müller, D.G. (1978). Chemical interaction in sexual reproduction of several Laminariales (Phaeophyceae) release and attraction of spermatozoids. Zeitschrift für Pflanzenphysiologie, 89: 333–341.
- Lüning, K., Chapman A.R.O. & Mann, K.H. (1978). Crossing experiments in the non-digitate complex of Laminaria from both sides of the Atlantic. Phycologia, 17: 293–298.
- Lunt, T., Jones, A.W., Mulhern, W.S., Lezaks, D.P.M. & Jahn, M.M. (2016). Vulnerabilities to agricultural production shocks: an extreme, plausible scenario for assessment of risk for the insurance sector. Climate Risk Management, 13: 1–9.
- Martins, N., Tanttu, H., Pearson, G.A., Serrão, E.A. & Bartsch, I. (2017). Interactions of daylength, temperature and nutrients affect thresholds for life stage transitions in the kelp Laminaria digitata (Phaeophyceae). Botanica Marina, 60: 109–121.
- Mazarrasa, I., Olsen, Y.S., Mayol, E., Marbà, N. & Duarte, C.M. (2014). Global unbalance in seaweed production, research effort and biotechnology markets. Biotechnology Advances, 32: 1028–1036.
- McArdle, B.H. & Anderson, M.J. (2001). Fitting multivariate models to community data: a comment on distance-based redundancy analysis. Ecology, 82: 290–297.
- Motomura, T. & Sakai, Y. (1981). Effect of chelated iron in culture media on oogenesis in Laminaria angustata. Bulletin of the Japanese Society for the Science of Fish, 47: 1535–1540.
- Müller, D.G., Murúa, P. & Westermeier, R. (2018). Reproductive strategies of Lessonia berteroana (Laminariales, Phaeophyceae) gametophytes from Chile: apogamy, parthenogenesis and cross-fertility with L. spicata. Journal of Applied Phycology, 2: 1475–1481.
- Provasoli, L. (1968). Media and prospects for the cultivation of marine algae. In Cultures and Collections of Algae (Watanabe, A. & Hattori, A., editors), 63–75. Proceedings of the US Japan Conference, Hakone 1966. Japanese Society of Plant Physiology, Tokyo.
- Rothman, M.D., Mattio, L., Anderson, R.J. & Bolton, J.J. (2017). A phylogeographic investigation of the kelp genus Laminaria (Laminariales, Phaeophyceae), with emphasis on the South Atlantic Ocean. Journal of Phycology, 53: 778–789.
- Schneider, C.A., Rasband, W.S. & Eliceiri, K.W. (2012). NIH Image to ImageJ: 25 years of image analysis. Nature Methods, 9: 671–675.
- Shan, T.F., Pang, S.J. & Gao, S.Q. (2013). Novel means for variety breeding an sporeling production in the brown seaweed Undaria pinnatifida (Phaeophyceae): crossing female gametophytes from parthenosporophytes with male gametophyte clone. Phycological Research, 61: 154–161.
- Shull, G.H. (1908). The composition of field of maize. American Breeders Association Report, 4: 296–301.
- Song, G., Wang, M., Zeng, B., Zhang, J., Jiang, C., Hu, Q., Geng, G. & Tang, C. (2015). Anther response to high-temperature stress during development and pollen thermotolerance heterosis as revealed by pollen tube growth and in vitro pollen vigor analysis in upland cotton. Planta, 241: 1271–1285.
- Stegenga, H., Bolton, J.J. & Anderson, R.J. (1997). Seaweeds of the South African West Coast. Cape Town: Bolus Herbarium, University of Cape Town.
- Steneck, R.S., Graham, M.H., Bourque, B.J., Corbett, D., Erlandson, J.M., Estes, J.A. & Tegner, M.J. (2002). Kelp forest ecosystems: biodiversity, stability, resilience and future. Environmental Conservation, 29: 436–459.
- tom Dieck, I. (1992). North Pacific and North Atlantic digitate Laminaria species (Phaeophyta): hybridization experiments and temperature responses. Phycologia, 31: 147–163.
- tom Dieck, I. & de Oliveira, E.C. (1993). The section Digitatae of the genus Laminaria (Phaeophyta) in the northern and southern Atlantic: crossing experiments and temperature responses. Marine Biology, 115: 151–160.
- Wernberg, T., Smale, D.A., Tuya, F., Thomsen, M.S., Langlois, T.J., de Bettignies, T., Bennett, S. & Rousseaux, C.S.G. (2013). An extreme climatic event alters marine ecosystem structure in a global biodiversity hotspot. Nature Climate Change, 3: 78–82.
- Westermeier, R., Patiño, D., Müller, H. & Müller, D.G. (2010). Towards domestication of giant kelp (Macrocystis pyrifera) in Chile: selection of haploid parent genotypes, outbreeding, and heterosis. Journal of Applied Phycology, 22: 357–361.
- Zhang, J., Liu, Y., Yu, D., Song, H., Cui, J. & Liu, T. (2011). Study on high-temperature-resistant and high-yield Laminaria variety “Rongfu”. Journal of Applied Phycology, 19: 303–311.
- Zhang, Q.S., Tang, X.X., Cong, Y.Z., Qu, S.C., Luo, S.J. & Yang, G.P. (2007). Breeding of an elite Laminaria variety 90-1 through interspecific gametophyte crossing. Journal of Applied Phycology, 19: 303–311.
- Zhao, X.B., Pang, F S.J. Liu, F., Shan, T.F., Li, J., Gao, S.Q. & Kim, H.G. (2016). Intraspecific crossing of Saccharina japonica using distantly related unialgal gametophytes benefits kelp farming by improving blade quality and productivity at Sanggou Bay, China. Journal of Applied Phycology, 28: 449–455.