ABSTRACT
We confirm an amnesic shellfish poisoning risk associated with domoic acid production by the species Pseudo-nitzschia plurisecta and determine the toxin profile, which includes three domoic acid isomers. Although the species was reported once in European waters, along the Spanish Atlantic coast, here we describe and characterize P. plurisecta collected during the first occurrence in French Atlantic waters. Three strains (IFR-PPL-15.1, 15.2 and 15.3) were isolated from French coastal water samples in June 2015. These strains were identified and characterized by microscopic observation, molecular (ITS region sequencing and phylogenetic analysis) and metabolite (toxins and pigments) analyses. The cell shape is lanceolate and the valve contains a central interspace with uniseriate stria of oval-square poroids, each divided into 3–10 sectors. The ITS gene sequence is homologous to the original sequence available for the Spanish strain P. plurisecta. The pigment profile contains chl a, c3, c2, fucoxanthin, diatoxanthin, diadinoxanthin and traces of β,β-carotene. Results on morphology, phylogeny and pigments led to the identification of P. plurisecta and confirmed its first occurrence in French waters.
Introduction
Several species of the marine diatom genus Pseudo-nitzschia are toxic due to their ability to produce the phycotoxin domoic acid (DA) (Bates et al., Citation1989, Citation2018; Caruana & Amzil, Citation2018). These diatoms bloom along coasts worldwide and the DA they produce may accumulate in shellfish, causing amnesic shellfish poisoning (ASP) in human consumers (Lelong et al., Citation2012; Grattan et al., Citation2016; Ajani et al., Citation2018; Wang et al., Citation2018). Along French coasts, several toxigenic Pseudo-nitzschia species have been reported since the first identification in 1998 of Pseudo-nitzschia species associated with the detection of DA (Amzil et al., Citation2001). These potentially toxic species include P. australis Frenguelli, P. multiseries (Hasle) Hasle, P. pseudodelicatissima (Hasle) Hasle, P. pungens (Grunow ex Cleve) G.R. Hasle, P. fraudulenta (Cleve) Hasle and, less frequently, P. calliantha Lundholm, Moestrup & Hasle (Amzil et al., Citation2001; Nézan et al., Citation2006; Quiroga, Citation2006). Pseudo-nitzschia populations generally develop from March to September with maximum abundance in May–June. They proliferate along the entire French coast including the Atlantic, English Channel and Mediterranean seafronts. However, DA contamination events are mainly reported to occur in the Atlantic areas of southern and western Brittany, where there are scallop fisheries (Husson et al., Citation2016). The risk of ASP is limited by screening and identification of toxic Pseudo-nitzschia species. Regular screening for the neurotoxin DA is done for shellfish flesh, and if DA contamination is detected shellfish harvesting areas are closed. This prevents contaminated seafood from coming on to the market. DA contamination causes huge economic losses to French scallop (Pecten maximus) fisheries due to frequent and long-term accumulations of DA, which can last from several months to a year (Blanco et al., Citation2002; Belin & Amzil, Citation2010). Moreover, DA may also contaminate other shellfish species such as those belonging to the genera Mytilus, Crassostrea, Donax, Glycymeris, Polititapes, Venerupis, Callista and Cerastoderma (Belin & Amzil, Citation2010). For the benefit of global public health, it is necessary to survey, report and update the occurrence and diversity of Pseudo-nitzschia species and their toxic potential worldwide.
The number of known Pseudo-nitzschia species has increased over the last decade. In 2012, only 37 species of Pseudo-nitzschia were reported, of which 14 were toxigenic (Lelong et al., Citation2012). Today, the genus Pseudo-nitzschia comprises 52 species (Bates et al., Citation2018; Guiry & Guiry, Citation2018) including 26 toxigenic species (Caruana & Amzil, Citation2018; Lundholm, Citation2019). This rise in the number of toxic species described is a result of increasing frequency and abundance of Pseudo-nitzschia blooms (Lundholm et al., Citation2010; Hinder et al., Citation2012; Hernández-Fariñas et al., Citation2014; Bazzoni et al., Citation2015) and technological advances. Indeed, there have been recent improvements in coastal monitoring effort (Lelong et al., Citation2012), development of laboratory tools for species identification (electron microscopy and molecular analyses) and high-sensitivity toxin detection, all of which have become more accessible in terms of costs, facilities and trained personnel. Available methods for toxin detection include the analytical chemistry method LC-MS/MS; the more affordable and widespread screening using the ELISA assay, which nevertheless requires confirmation by chemical analysis; and the recent but more costly multitoxin Surface Plasmon Resonance (SPR biosensor) (De la Iglesia et al., Citation2013; McNamee et al., Citation2016). As a consequence of bloom frequency and scientific progress over recent years, novel Pseudo-nitzschia species have been identified and their toxicity demonstrated, e.g. P. simulans Li, Huang & Xu, P. batesiana Lim, Teng, Leaw & Lim, P. kodamae Teng, Lim, Leaw & Lim and P. fukuyoi Lim, Teng, Leaw & Lim (Lim et al., Citation2013; Teng et al., Citation2014, Citation2016; Dao et al., Citation2015; Li et al., Citation2017). Furthermore, some species have been shown to be toxic several years after their first taxonomic identification, when the opportunity arose to measure DA in monospecific blooms or isolated and cultivated algal cells. For instance, DA was detected in cells of P. cuspidata (Hasle) Hasle, P. brasiliana Lundholm, Hasle & Fryxell, P. caciantha Lundholm, Moestrup & Hasle, P. subpacifica (Hasle) Hasle and P. obtusa (Hasle) Hasle & Lundholm between 2009 and 2015 (Trainer et al., Citation2009; Sahraoui et al., Citation2011; Dao et al., Citation2014; Fernandes et al., Citation2014; Haroardottir et al., Citation2015), although these species had all already been identified 10–50 years earlier (Hasle, Citation1965; Lundholm et al., Citation2002, Citation2003). The toxic status of some species still needs to be confirmed, such as in the case of P. hasleana Lundholm, for which there is only one report of DA production (Sakka Hlaili et al., Citation2016). In the case of P. plurisecta Orive & Pérez-Aicua, the species was described by Orive et al. (Citation2013) after being isolated in an estuary on the Spanish Atlantic coast. Prior to its description, an unnamed American species belonging to the same clade was isolated from the Gulf of Maine and shown to produce DA (Fernandes et al., Citation2014). Based on the genetic affinities of this American strain with P. plurisecta, the diatom species was later listed among DA-producing species, but with an ambiguous reference due to the association of the toxin data with an anonymous strain (Zabaglo et al., Citation2016). A more recent paper has since reported DA in two strains of P. plurisecta from Namibian waters (Gai et al., Citation2018).
Here, we report further evidence of DA production by P. plurisecta as well as its first detection from the French Atlantic coast, supported by a detailed biological description and toxin profile.
Materials and methods
Algal sampling, isolation and culture
A subsurface seawater sample (<1 m) was collected (8 June 2015) in a Niskin bottle from the ‘Ouest Loscolo’ station (GPS coordinates 47.457596, –2.538268) by the French phytoplankton and phycotoxin monitoring network REPHY (). The water temperature was 15.7°C and salinity 33. Pseudo-nitzschia chains were isolated from fresh seawater samples under the microscope using an elongated Pasteur pipette and 4-well plates (Nunc, Thermo Fisher Scientific, Waltham, USA) filled with L1 medium for the rinsing steps of individual cells. Each isolated cell led to a clonal Pseudo-nitzschia strain that was then maintained in Erlenmeyer flasks for a few months by subculturing each week. Several strains, including three we named as IFR-PPL-15.1, IFR-PPL-15.2 and IFR-PPL-15.3, were cultivated in batch cultures under 120 µmol photons m–2 s–1 light intensity. Cell density was enumerated by direct counting on a culture aliquot fixed with acidified Lugol’s iodine and placed in a Nageotte counting chamber under a microscope. Two culture samples were used so that a mean could be calculated. Counts were made on 400 cells to limit deviation to 10% (Lund et al., Citation1958).
Figure 1. Map of the French Phytoplankton Monitoring Network sampling sites in the part of the Atlantic Ocean where Pseudo-nitzschia plurisecta was observed. In the bottom left-hand corner, the black square shows the site at the scale of the whole France. On the large-scale map, the site ‘Ouest Loscolo’ close to the Vilaine Estuary is indicated by the bold black star. The other sites ‘Concarneau Large’ and ‘Men er Roué’ where P. plurisecta was found in 2009 and 2016, respectively, are indicated by small black stars.
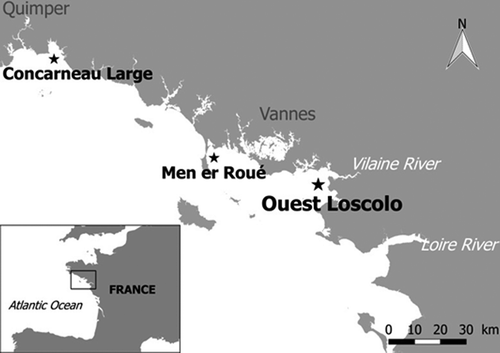
Taxonomic identification
Taxonomic identification of Pseudo-nitzschia species was performed by coupling morphological and molecular identification results. Transmission electron microscopy (TEM; JEOL JEM-12-30 Tokyo, Japan) was carried out on the strain IFR-PPL-15.2 to study the detailed morphology of the frustules and confirm that the morphological characters agreed with the molecular identification (described below). Frustules were cleaned with acid, rinsed in distilled water and then mounted on Formvar grids according to the method described by Hasle (Citation1978).
Molecular sequencing of rDNA ITS regions (ITS1, 5.8S, ITS2) was used to identify the Pseudo-nitzschia strains maintained in laboratory cultures. PCRs were performed using the oligonucleotide primers and protocols described in Nézan et al. (Citation2014). The sequences obtained were aligned together with 49 sequences of Pseudo-nitzschia retrieved from GenBank using MAFFT v.7 software with the E-ins-I strategy (Katoh & Standley, Citation2013). Unaligned parts of the matrix were removed using Gblocks 0.91b (Castresana, Citation2000). The dataset comprising 52 sequences and 603 characters was analysed by two methods of phylogenetic reconstruction: Maximum likelihood (ML), using PhyML v.3.0 (Guindon et al., Citation2010) and Bayesian inference (BI) using MrBayes v.3.2.6 (Ronquist & Huelsenbeck, Citation2003). jModeltest v.2.1.10 5 (Darriba et al., Citation2012) was first used to select the most suitable substitution model. The General Time-Reversible model (GTR) with invariant sites (I) and gamma distribution with four categories (G) was chosen, as indicated by Hierarchical Likelihood Ratio Tests (hLRTs), Akaike Information Criterion 1 (AIC1), Akaike Information Criterion 2 (AIC2) and Bayesian Information Criterion (BIC) tests, implemented in jModeltest 2. Bootstrap values of trees (indicating branch support) were obtained after 1000 iterations in ML. For Bayesian inference, four Markov chains were run simultaneously for 2 × 106 generations with sampling every 100 generations. Of the 2 × 104 trees obtained, the first 2000 were discarded (burn-in) and a consensus tree was constructed from the remaining trees. The posterior probabilities, corresponding to the frequency at which a node is present in preserved trees, were calculated using a coupled Metropolis–Monte Carlo Markov Chain (MCMC) approach.
Domoic acid analyses
Algal cell extracts of the three cultivated strains were analysed for intracellular toxins. First, cell pellets were obtained by centrifugation (3600 × g, 5 min) of 10 ml culture samples. The pellet was extracted in 1 ml methanol/water (50/50 v/v) and stored at –80°C until the subsequent steps were carried out. Defrosted cells were sonicated (20 min of intermittent 15 s pulses at 5 s intervals) with ice and ultracentrifuged (8000 × g, 0.2 µm, 15 min, Nanosep MF, Pall Corporation, East Hills, New York, USA) to obtain the supernatant. Samples were stored at −20°C until analyses. Ten µl of sample were injected into an Ultra Fast Liquid Chromatography (UFLC) system (Shimadzu, Marne-La-Vallée, France) coupled to 4000 QTrap tandem mass spectrometer (ABSciex, Les Ulis, France). The LC system was equipped with a Kinetex C18 precolumn (2.6 μm, 2 × 2.1 mm, Phenomenex, Le Pecq, France) and column (2.6 μm, 150 × 2.1 mm, Phenomenex). Flow rate was 0.2 ml min–1 and column temperature was 35°C. The mobile phase was composed of water as eluent A and 95% acetonitrile and 5% water as eluent B, both eluents containing 0.1% formic acid. The eluting gradient varied from 5 to 30% of eluent B in 14 min, then changed to 90% of B in 0.1 min and was maintained for 2.5 min before returning to initial conditions.
The LC-MS/MS system was used in MRM in positive mode. The following parameters were applied to the electrospray ionization source: curtain gas 20 psi, temperature 550°C, gas1 50 psi, gas2 60 psi, ion spray voltage 5500 V. The following m/z transitions 312.1→266.1; 312.1→248.0; 312.1→220.0; 312.1→193.1; and 312.1→161.1 were used for identifying DA and some isomers. For detecting these fragments, the declustering potential was 60 V and the collision energies were 23, 25, 29, 27 and 33 eV for transitions 1 to 5, respectively. A calibration curve (0.5–250 ng ml–1) based on a certified DA standard (CNRC, Halifax, Canada) prepared in methanol/water made it possible to perform quantification based on the most intense transition 312.1→266.1 (LD and LQ were respectively 0.1 and 0.25 ng ml–1, respectively, corresponding to 1 and 2.5 pg DA on column). The commercial DA standard contains DA and traces of isodomoic acid-A, isodomoic acid-D and epi-DA among the nine existing isomers. Data were analysed with Analyst 1.5.1, ABSciex software and the cell toxin profile and toxin concentrations are given in fg per cell.
Pigment analyses
Pigment analyses were performed on the P. plurisecta strains. Fifty ml of culture were filtered on a 25 mm GF/F filter (Whatman, Maidstone, UK). The filter was snap-frozen in liquid N2 and stored at −80°C until extraction. Pigments were extracted from cells by adding 1 ml of cold (4°C) 100% acetone. An internal standard (trans-β-apo-8’-carotenal, Sigma-Aldrich, Saint-Quentin-Fallavier, France) was also added at this stage to allow final corrections according to the extraction yield. Cell lyses were stimulated using an ultrasonic bath filled with ice (10 min, twice) and the sample was stored for 6–24 hours at −20°C in darkness. Debris was removed by filtration of the supernatant on a PTFE 0.2-µm membrane. Sample aliquots of 30 µl were supplemented with 45 µl of ultrapure water and analysed by Liquid Chromatography with Diode Array Detection (LC-DAD). The 1200 LC-DAD (Agilent Technologies, Massy, France) apparatus was set up with a precolumn (XSelect HSS T3, 3.5 µm, 20 × 3 mm, Waters) and a C18 column (XSelect HSS T3, 3.5 µm, 150 × 3 mm, Waters).
The LC-DAD method was adapted from Zapata et al. (Citation2012) and used the same eluents as that previous study, but gradients were modified to improve peak separation. Hence, four eluents were used: A: methanol, B: acetonitrile, C: pyridine (25 mM, pH 5) and D: acetone (Sigma-Aldrich). The initial mobile phase was composed of A:B:C:D=45:38:17:0 (v/v/v/v) from t0 to t3, with time (t) expressed in minutes. The gradient of elution then evolved to 35:50:15:0 between t3 and t20 and to 20:60:0:20 between t20 and t25. It then evolved to 0:60:0:40 between t25 and t35 and remained at these proportions until t43 before finally returning to the initial mobile phase at t45. Flow rate was 0.6 ml min–1 and column temperature was 30°C. Data were collected with the OpenLab CDS Chemstation C.01.07 program.
Pigments were identified by their retention time and comparison of the absorbance spectra between 350 and 700 nm with those obtained with commercial standards. Pigment quantification was done at two wavelengths according to the maximum absorbance wavelengths of the pigments: 436 nm for chl a and 450 nm for the others. Commercial standards (DHI Water Environment Health, Hørsholm, Denmark) for each pigment were used with calibration curves, for identification and quantification of the pigments in the algal cell extracts.
Algal cells from each strain in triplicate batch cultures were sampled several times during culture growth (at days 3, 7 and 10 for strain IFR-PPL-15.1, at days 2, 5, 6, 9 and 10 for strain IFR-PPL-15.2 and at days 6 and 9 for strain IFR-PPL-15.3). Range data and means are given to cover potential growth variation. Data are displayed in several units: fg per cell, mol per mol of chl a, µg per l of culture and as a percentage of total pigments. Sample extractions and analyses were performed under weak light conditions.
Results
Strain identification
The Pseudo-nitzschia strains IFR-PPL-15.1, 15.2 and 15.3 isolated from the ‘Ouest Loscolo’ station on the French Atlantic coast () in 2015 were identified as Pseudo-nitzschia plurisecta according to their morphological characters (–) and the molecular analyses (). Based on the ITS region, the three strains were genetically identical. Morphologically, frustules of the culture IFR-PPL-15.2 were lanceolate, 23.3–29.7 µm long (mean 26.0 ± 1.4 µm, n = 30) and 1.9–2.5 µm wide (mean 2.2 ± 0.2 µm, n = 30). A central interspace () was present on the valves. The number of striae in 10 µm was 37–41 while the number of fibulae in 10 µm was 22–25. Each stria was uniseriate and contained one row of oval-square poroids, with 5–7 poroids in 1 µm. Each poroid was split into 3–10 sectors, with a few possessing a central sector (), as previously shown for P. plurisecta (Orive et al., Citation2013). As in P. australis (Amato et al., Citation2005), when cultivated in artificial medium, P. plurisecta rapidly showed cell deformation as illustrated by several humps on each side of the frustule ().
Figures 2–8. TEM photographs of Pseudo-nitzschia plurisecta frustules. Fig. 2. Whole valve; Fig. 3. Detail of the central area; Figs 4, 5. Extremities; Fig. 6. Detail of the central interspace and arrangement of poroids, arrowheads point to poroids with a central sector, in uniseriate striae; Fig. 7. Detail of sectors within poroids; Fig. 8. Detail of poroids in a cingular band. Scale: Fig. 2 = 5 µm; Fig. 3 = 1 µm, Figs 4–6 = 200 nm; Figs 7–8 = 100 nm.
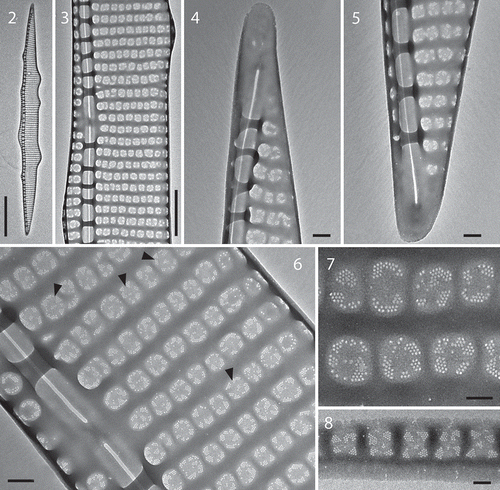
Figure 9. Maximum likelihood (ML) phylogenetic tree based on ITS rDNA sequences (52 sequences/603 characters). Bootstraps include x/y value where x is the ML statistic value and y is the Bayesian probability.
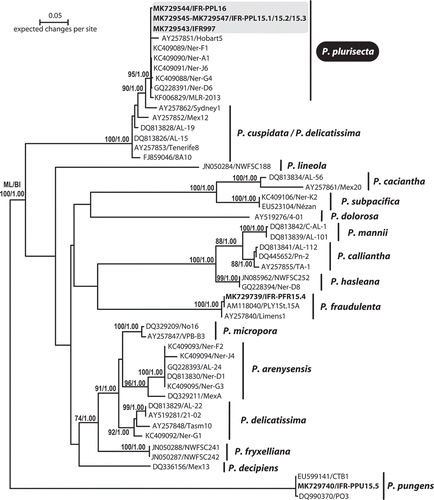
The phylogenetic analysis revealed that the sequences of strains IFR-PPL-15.1, 15.2 and 15.3 were identical to sequence KC409089 of strain Ner-F1, isolated from Bilbao outer estuary and considered as the type strain for P. plurisecta (Orive et al., Citation2013). Notably, a sequence (IFR997) previously acquired from a single-chain isolated in the Bay of Concarneau in 2009 (; GPS coordinates 47.833406, –3.949922), clustered with and corresponds to P. plurisecta (). Likewise, a 2016 isolate (IFR-PPL-16) from the REPHY ‘Men er Roué’ Atlantic sampling station (GPS coordinates of 47.534765, –3.093748) is now genetically identified as P. plurisecta.
Strain toxicity
For the three P. plurisecta strains IFR-PPL-15.1, 15.2 and 15.3, toxin analyses of cell extracts revealed similar DA chromatographic profiles compared with the standard profile for DA. The DA peak appeared at a retention time of 8.1 min with five transition ions: 312.1 → 266.1, 248.0, 220.0, 193.1 and 161.1. For clarity, only the most and least intense transitions (312.1 →266.1 and 161.1) are shown on –. Several peaks of DA isomers were observed. Based on the comparison of their retention time with those of the DA standard, these isomers were identified as possibly being isodomoic acid-A and epi-DA in the three P. plurisecta strains and isodomoic acid-D in the strain IFR-PPL-15.3. The three strains contain DA at 3.1, 3.9 and 58.3 fg cell–1, respectively ().
Table 1. Toxin profiles of three Pseudo-nitzschia plurisecta strains including DA and proposed DA isomers according to the available but incomplete standard profile.
Figures 10–13. Chromatogram of domoic acid (DA) and isomers obtained after LC-MS/MS analyses of standard and Pseudo-nitzschia plurisecta cell extracts. Fig. 10. Domoic acid standard; Fig. 11. P. plurisecta strain IFR-PPL-15.1; Fig. 12. Strain IFR-PPL-15.2; Fig. 13. Strain IFR-PPL-15.3. Figs 10 and 11 include magnified views to show the isomer peaks. The highest peak represents the most abundant m/z transition 312.1 → 266.1 and the peak beneath is the less intense fragment 312.1 → 161.1.
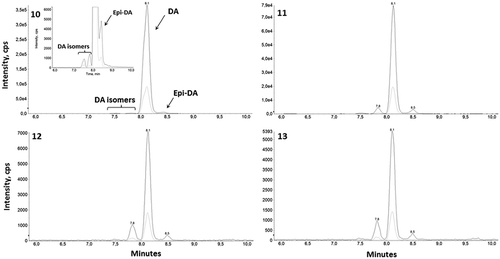
Pigment analyses
Pigment analyses () confirmed the presence of chlorophylls a, c3 and c2, the carotenoids fucoxanthin, diatoxanthin, diadinoxanthin and traces of β,β-carotene in the three strains of P. plurisecta. Although the pigment ratio could vary during growth in batch culture, chl a and fucoxanthin were the major pigments, each accounting for between a third and half of the total pigments. Apart from fucoxanthin, chl c3 was the other main accessory pigment (reaching a maximum of 10%). Chl c2, diadinoxanthin and diatoxanthin were minor pigments (<7% for each). β,β-carotene was present at trace level (<2%). The ratios of major accessory pigments to chl a are in the ranges of 0.834–1.439, 0.164–0.209 and 0.194–0.325 for fucoxanthin, chl c2 and chl c3, respectively.
Table 2. Pigment profiles of Pseudo-nitzschia plurisecta cells observed in the three strains IFR-PPL-15.1, 15.2 and 15.3 cultivated in batch cultures for between 2 to 10 days.
Discussion
The morpho-molecular analyses performed in the present study unambiguously confirm that strains IFR-PPL-15.1, 15.2 and 15.3 correspond to Pseudo-nitzschia plurisecta. However, their morphology was analysed on aged cultures and the cell dimensions (mean length 26.0 µm) were not in the same range (56–60 µm) as those given in the original description of the Spanish strain (Orive et al. Citation2013). Nevertheless, the detailed characters of striae, fibulae and poroid arrangement were the same as given by the previous authors, allowing us to confirm the species identification. Based on molecular data, our study demonstrates that this species was already present in June 2009 in the northern part of the Bay of Biscay (France), before its description by Orive et al. (Citation2013), and was observed again in 2016, confirming its recurrence in the phytoplankton community.
In terms of toxin production, P. plurisecta is confirmed to produce DA and is rightfully categorized as a toxic species. P. plurisecta is thus an additional toxigenic species that may be present in Pseudo-nitzschia populations in French coastal waters, contributing to ASP risk. The DA content of P. plurisecta varies among strains in laboratory cultures, potentially due to diversity of cell sizes, growth phases or phenotypes. The French strains show a 17-fold variation in DA concentration (3–59 fg cell–1), while American and Namibian strains contain a 6-fold higher DA concentration (19–385 fg cell–1; Fernandes et al., Citation2014; Gai et al., Citation2018). The lower DA content of French P. plurisecta strains may result from the smaller cell size (23–30 µm length in comparison with 30–54 µm for the American strain). Indeed, DA content generally decreases with culture ageing and smaller cell size in Pseudo-nitzschia (Mafra et al., Citation2009). P. plurisecta appears to produce a much lower concentration of DA than the most toxigenic species, P. australis or P. multiseries that also proliferate along the French coasts and other parts of the world, producing up to 78 and 67 pg DA cell–1 respectively (Lelong et al., Citation2012; Trainer et al., Citation2012). However, P. plurisecta produces an equivalent or higher DA concentration than most other toxigenic Pseudo-nitzschia species (reviewed in Lelong et al., Citation2012 and Trainer et al., Citation2012).
The toxin profile of P. plurisecta is composed of DA and three DA isomers whose identities, based on an incomplete panel of standards, remain uncertain and would require further RMN analyses. So far, isodomoic acid-A, -B and -C have been identified in some Pseudo-nitzschia spp. and traces of isodomoic acid-D, -E and epi-DA have been identified in Nitzschia navis-varingica (Holland et al., Citation2005; Hansen et al., Citation2011; Romero et al., Citation2011). According to published chromatographic data (Romero et al., Citation2011), DA isomers in P. plurisecta cells are most likely to be isodomoic acid-D and -A. Here, it is also proposed that epi-DA is present in P. plurisecta cells, and a possible contribution of isodomoic acid-B to the epi-DA peak cannot be excluded. P. plurisecta may represent a significant source of DA contamination for countries with coasts along the Atlantic Ocean. Further ecophysiological studies on this species are needed to precisely determine its DA producing capacity in relation to growth phases, environmental conditions and strain variability and to confirm the DA isomer identities.
The pigment composition of P. plurisecta observed here is similar to that described by Orive et al. (Citation2013). P. plurisecta appears to have the most common pigment type of Pseudo-nitzschia species: type 3 as described by Zapata et al. (Citation2011), like the toxic Pseudo-nitzschia species P. calliantha, P. cuspidata, P. delicatissima, P. galaxiae and P. pseudodelicatissima, while some other toxic Pseudo-nitzschia species belong to pigment types 1 or 2. The pigment composition of P. plurisecta shows some differences from other Pseudo-nitzschia species. Although chl a and fucoxanthin are found to be the major pigments here as in other Pseudo-nitzschia spp. (Quijano-Scheggia et al., Citation2008; Zapata et al., Citation2011), chl c1, chl c2-like, MgDVP, cis-fucoxanthin and chlorophyllide were not detected in our P. plurisecta cultures or in the previously reported occurrence (Orive et al., Citation2013). Chl c3 is the second most important accessory pigment, as in P. delicatissima (Quijano-Scheggia et al., Citation2008), while chl c2 is generally found as the dominant chl c-type pigment in other Pseudo-nitzschia species. The most toxic species, P. australis and P. multiseries, contain chl c2 but not chl c3. Zapata et al. (Citation2011) observed that the ratios of accessory pigments to chl a (fuco : chl a or chl c2 : chl a) are conserved among 49 Pseudo-nitzschia strains belonging to 17 species (0.448–1.130, 0.150–0.424, respectively) while the chl c3 to chl a ratio is naturally more variable (0–0.313). In P. plurisecta cells, apart from the slightly higher ratio of fuco : chl a, the ratios chl c2 : chl a and chl c3 : chl a are within similar ranges to those described by Zapata et al. (Citation2011).
P. plurisecta is distributed on both sides of the North Atlantic Ocean, including along the European coast (Orive et al., Citation2013), Gulf of Maine (Fernandes et al., Citation2014); it is also found in Namibian waters in the South Atlantic Ocean (Gai et al., Citation2018). This distribution appears limited due to the recent description of this species and might expand in the future. Along the European coast, the occurrence of this species seems to be favoured by estuarine influences as observed in the Bilbao Estuary in Spain and the Vilaine Estuary in France. Along the French coast, the proliferation of Pseudo-nitzschia populations appears to be facilitated by nutrient-rich waters in confined or semi-enclosed bays enriched by river input and benthic sediment mixing (Husson et al., Citation2016). Moreover, the increasing abundance of Pseudo-nitzschia populations along the French coast, e.g. between 1992 and 2011 reported by Hernández-Fariñas et al. (Citation2015), may lead to the observation of new or rare Pseudo-nitzschia species. Consequently, eutrophic waters potentially represent a nursery for new Pseudo-nitzschia species, suggesting that eutrophication may not only influence the abundance but also the richness in toxic species.
Regarding shellfish farming management, further investigations are required to understand what favours the occurrence of toxic Pseudo-nitzschia species compared with non-toxic ones, and their possible relationship with other phytoplankton species. For instance, more data on species assemblages and succession would be helpful to investigate whether there is a link between changes in species assemblages and the observed rise in toxic species occurrence. In the present study, P. plurisecta was isolated in association with non-toxic strains of P. fraudulenta and P. pungens (SEM photographs in supplementary files). In Namibian waters, P. plurisecta was found with P. bucculenta or P. pungens and P. australis (Gai et al., Citation2018). In a study along the English Channel, Klein et al. (Citation2010) observed the succession of several Pseudo-nitzschia species in relation, although not exclusively, to peak concentrations of NO3 or NH4. Interestingly, the authors found that P. australis occurred under high NH4 and low NO3 concentrations, while P. delicatissima, P. fraudulenta and P. multistriata preferred high NO3 concentrations. However, other environmental factors may also influence Pseudo-nitzschia population dynamics: such as irradiance and temperature conditions triggering the bloom initiation; and, over a decadal timescale, changes in salinity, temperature and the Atlantic multidecadal oscillation index that may become favourable for the development of Pseudo-nitzschia populations (Hernández-Fariñas et al., Citation2014; Husson et al., Citation2016).
Our study confirms DA production by P. plurisecta and provides a detailed description of the species. P. plurisecta cells were shown to contain DA and three isomers that are potentially isodomoic acid-A, -D and epi-DA. Because P. plurisecta can pose a severe risk of amnesic shellfish poisoning to humans, and is known to be present in Atlantic coastal and estuarine areas, it is important to list P. plurisecta as a toxic Pseudo-nitzschia species (Lundholm, Citation2019). The increase in Pseudo-nitzschia populations means that further ecological and ecophysiological investigations should be encouraged to enhance our knowledge of the ecological niche and DA production capacity of P. plurisecta.
Author Contributions
A.M.N. Caruana: original concept, drafting and editing manuscript; N. Ayache: cultures and pigment analyses; V. Raimbault: algal isolation; M. Rétho: field sampling of microalgae; F. Hervé: metabolite analyses; G. Bilien: molecular analyses; Z. Amzil: editing manuscript; N. Chomérat: taxonomic identification, editing manuscript.
Supplementary Information
The following supplementary material is accessible via the Supplementary Content tab on the article’s online page at https://doi.org/10.1080/09670262.2019.1620342
Supplementary fig. S1. Photographs of Pseudo-nitzschia fraudulenta IFR-PFR-15.4 acquired by scanning electron microscopy.
Supplementary fig. S2. Photographs of Pseudo-nitzschia pungens IFR-PPU-15.5 acquired by scanning electron microscopy.
TEJP-2017-0108-File007.docx
Download MS Word (1.3 MB)TEJP-2017-0108-File006.docx
Download MS Word (1.4 MB)Acknowledgements
This work was supported by IFREMER through the internal funding of the ECOPSEUDO project on Pseudo-nitzschia. We are also grateful to Cédric Gaillard of the BIBS Platform at INRA Nantes for allowing us to produce the TEM photographs.
Disclosure statement
No potential conflict of interest was reported by the authors.
References
- Ajani, P.A., Verma, A., Lassudrie, M., Doblin, M.A. & Murray, S.A. (2018). A new diatom species P. hallegraeffii sp. nov. belonging to the toxic genus Pseudo-nitzschia (Bacillariophyceae) from the East Australian Current. PLoS ONE, 13: 20.
- Amato, A., Orsini, L., D’alelio, D. & Montresor, M. (2005). Life cycle, size reduction patterns, and ultrastructure of the pennate planktonic diatom Pseudo-nitzschia delicatissima (Bacillariophyceae). Journal of Phycology, 41: 542–556.
- Amzil, Z., Fresnel, J., Le Gal, D. & Billard, C. (2001). Domoic acid accumulation in French shellfish in relation to toxic species of Pseudo-nitzschia multiseries and P. pseudodelicatissima. Toxicon, 39: 1245–1251.
- Bates, S.S., Bird, C.J., Defreitas, A.S.W., Foxall, R., Gilgan, M., Hanic, L.A., Johnson, G.R., Mcculloch, A.W., Odense, P., Pocklington, R., Quilliam, M.A., Sim, P.G., Smith, J.C., Rao, D.V.S., Todd, E.C.D., Walter, J.A. & Wright, J.L.C. (1989). Pennate diatom Nitzschia pungens as the primary source of domoic acid, a toxin in shellfish from eastern Prince Edward Island, Canada. Canadian Journal of Fisheries and Aquatic Sciences, 46: 1203–1215.
- Bates, S.S., Hubbard, K.A., Lundholm, N., Montresor, M. & Leaw, C.P. (2018). Pseudo-nitzschia, Nitzschia, and domoic acid: new research since 2011. Harmful Algae, 79: 3–43.
- Bazzoni, A.M., Caddeo, T., Pulina, S., Padedda, B.M., Satta, C.T., Sechi, N. & Luglie, A. (2015). Spatial distribution and multiannual trends of potentially toxic microalgae in shellfish farms along the Sardinian coast (NW Mediterranean Sea). Environmental Monitoring and Assessment, 187.
- Belin, C. & Amzil, Z. (2010). Phycotoxin monitoring in France: risk-based strategy and main results (2006–2008). In Proceedings of the 7th International Conference on Molluscan Shellfish Safety (Lassus, P., editor), 149–156. Ifremer, Nantes.
- Blanco, J., Acosta, C.P., De La Puente, M.B. & Salgado, C. (2002). Depuration and anatomical distribution of the amnesic shellfish poisoning (ASP) toxin domoic acid in the king scallop Pecten maximus. Aquatic Toxicology, 60: 111–121.
- Caruana, A.M.N. & Amzil, Z. (2018). Microalgae and toxins. In Microalgae in Health and Disease Prevention ( Levine, I.A. & Fleurence, J., editors), 263–305. Academic Press, London.
- Castresana, J. (2000). Selection of conserved blocks from multiple alignments for their use in phylogenetic analysis. Molecular Biology and Evolution, 17: 540–552.
- Dao, H.V., Lim, P.T., Ky, P.X., Takata, Y., Teng, S.T., Omura, T., Fukuyo, Y. & Kodama, M. (2014). Diatom Pseudo-nitzschia cf. caciantha (Bacillariophyceae), the most likely source of domoic acid contamination in the thorny oyster Spondylus versicolor Schreibers 1793 in Nha Phu Bay, Khanh Hoa Province, Vietnam. Asian Fisheries Science, 27: 16–29.
- Dao, H.V., Phan, V.B., Teng, S.T., Uchida, H., Leaw, C.P., Lim, P.T., Suzuki, T. & Pham, K.X. (2015). Pseudo-nitzschia fukuyoi (Bacillariophyceae), a domoic acid-producing species from Nha Phu Bay, Khanh Hoa Province, Vietnam. Fisheries Science, 81: 533–539.
- Darriba, D., Taboada, G.L., Doallo, R. & Posada, D. (2012). jModelTest 2: more models, new heuristics and parallel computing. Nature Methods, 9: 772.
- De la Iglesia, P., Fernandez-Tejedor, M., Trobajo, R. & Diogene, J. (2013). An analytical perspective on detection, screening, and confirmation in phycology, with particular reference to toxins and toxin-producing species. Journal of Phycology, 49: 1056–1060.
- Fernandes, L.F., Hubbard, K.A., Richlen, M.L., Smith, J., Bates, S.S., Ehrman, J., Leger, C., Mafra, L.L., Kulis, D., Quilliam, M., Libera, K., McCauley, L. & Anderson, D.M. (2014). Diversity and toxicity of the diatom Pseudo-nitzschia Peragallo in the Gulf of Maine, Northwestern Atlantic Ocean. Deep-Sea Research Part II- Topical Studies in Oceanography, 103: 139–162.
- Gai, F.F., Hedemand, C.K., Louw, D.C., Grobler, K., Krock, B., Moestrup, O. & Lundholm, N. (2018). Morphological, molecular and toxigenic characteristics of Namibian Pseudo-nitzschia species – including Pseudo-nitzschia bucculenta sp nov. Harmful Algae, 76: 80–95.
- Grattan, L.M., Holobaugh, S. & Morris, J.G. (2016). Harmful algal blooms and public health. Harmful Algae, 57: 2–8.
- Guindon, S., Dufayard, J.F., Lefort, V., Anisimova, M., Hordijk, W. & Gascuel, O. (2010). New algorithms and methods to estimate Maximum-Likelihood phylogenies: assessing the performance of PhyML 3.0. Systematic Biology, 59: 307–321.
- Guiry, M.D. & Guiry, G.M. (2018). AlgaeBase. World-wide electronic publication, National University of Ireland, Galway. http://www.algaebase.org.
- Hansen, L.R., Soylu, S.I., Kotaki, Y., Moestrup, O. & Lundholm, N. (2011). Toxin production and temperature-induced morphological variation of the diatom Pseudo-nitzschia seriata from the Arctic. Harmful Algae, 10: 689–696.
- Haroardottir, S., Pancic, M., Tammilehto, A., Krock, B., Moller, E.F., Nielsen, T.G. & Lundholm, N. (2015). Dangerous relations in the Arctic marine food web: interactions between toxin producing Pseudo-nitzschia diatoms and Calanus copepodites. Marine Drugs, 13: 3809–3835.
- Hasle, G.R. (1965). Nitzschia and Fragilariopsis species studied in the light and electron microscopes. II. The group Pseudo-nitzschia. Skrifter utgitt av Det Norske Videnskaps-Akademii Oslo. I. Matematisk-Naturvidenskapelig Klasse. Series 18: 1–45.
- Hasle, G.R. (1978). Some specific preparations: diatoms. In Phytoplankton Manual (Sournia, A., editor), 136–142. UNESCO, Paris.
- Hernández-Fariñas, T. (2015). Analyse et modélisation des évolutions à long terme de la biodiversité phytoplanctonique dans les zones côtières sous l’effet des pressions environnementales et anthropiques. PhD dissertation. University of Nantes, France, 352 pp.
- Hernández-Fariñas, T., Soudant, D., Barille, L., Belin, C., Lefebvre, A. & Bacher, C. (2014). Temporal changes in the phytoplankton community along the French coast of the eastern English Channel and the southern Bight of the North Sea. ICES Journal of Marine Science, 71: 821–833.
- Hinder, S.L., Hays, G.C., Edwards, M., Roberts, E.C., Walne, A.W. & Gravenor, M.B. (2012). Changes in marine dinoflagellate and diatom abundance under climate change. Nature Climate Change, 2: 271–275.
- Holland, P.T., Selwood, A.I., Mountfort, D.O., Wilkins, A.L., Mcnabb, P., Rhodes, L.L., Doucette, G.J., Mikulski, C.M. & King, K.L. (2005). Isodomoic acid C, an unusual amnesic shellfish poisoning toxin from Pseudo-nitzschia australis. Chemical Research in Toxicology, 18: 814–816.
- Husson, B., Hernandez-Farinas, T., Le Gendre, R., Schapira, M. & Chapelle, A. (2016). Two decades of Pseudo-nitzschia spp. blooms and king scallop (Pecten maximus) contamination by domoic acid along the French Atlantic and English Channel coasts: Seasonal dynamics, spatial heterogeneity and interannual variability. Harmful Algae, 51: 26–39.
- Katoh, K. & Standley, D.M. (2013). MAFFT multiple sequence alignment software version 7: improvements in performance and usability. Molecular Biology and Evolution, 30: 772–780.
- Klein, C., Claquin, P., Bouchart, V., Le Roy, B. & Veron, B. (2010). Dynamics of Pseudo-nitzschia spp. and domoic acid production in a macrotidal ecosystem of the Eastern English Channel (Normandy, France). Harmful Algae, 9: 218–226.
- Lelong, A., Hegaret, H., Soudant, P. & Bates, S.S. (2012). Pseudo-nitzschia (Bacillariophyceae) species, domoic acid and amnesic shellfish poisoning: revisiting previous paradigms. Phycologia, 51: 168–216.
- Li, Y., Huang, C.X., Xu, G.S., Lundholm, N., Teng, S.T., Wu, H. & Tan, Z. (2017). Pseudo-nitzschia simulans sp. nov. (Bacillariophyceae), the first domoic acid producer from Chinese waters. Harmful Algae, 67: 119–130.
- Lim, H.C., Teng, S.T., Leaw, C.P., & Lim, P.T. (2013). Three novel species in the Pseudo-nitzschia pseudodelicatissima complex: P. batesiana sp. nov., P. lundholmiae sp. nov., and P. fukuyoi sp. nov. (Bacillariophyceae) from the strait of Malacca, Malaysia. Journal of Phycology, 49: 902–916.
- Lund, J.W.G., Kipling, C. & Lecren, E.D. (1958). The inverted microscope method of estimating algal numbers and the statistical basis of estimations of counting. Hydrobiologia, 11: 143–170.
- Lundholm, N. (ed.) (2019). Bacillariophyceae. In IOC-UNESCO Taxonomic Reference List of Harmful Micro Algae. Available at http://www.marinespecies.org/hab.
- Lundholm, N., Clarke, A. & Ellegaard, M. (2010). A 100-year record of changing Pseudo-nitzschia species in a sill-fjord in Denmark related to nitrogen loading and temperature. Harmful Algae, 9: 449–457.
- Lundholm, N., Hasle, G.R., Fryxell, G.A. & Hargraves, P.E. (2002). Morphology, phylogeny and taxonomy of species within the Pseudo-nitzschia americana complex (Bacillariophyceae) with descriptions of two new species, Pseudo-nitzschia brasiliana and Pseudo-nitzschia linea. Phycologia, 41: 480–497.
- Lundholm, N., Moestrup, O., Hasle, G.R. & Hoef-Emden, K. (2003). A study of the Pseudo-nitzschia pseudodelicatissima/cuspidata complex (Bacillariophyceae): What is P. pseudodelicatissima? Journal of Phycology, 39: 797–813.
- Mafra, L.L., Bricelj, V.M., Ouellette, C., Leger, C. & Bates, S.S. (2009). Mechanisms contributing to low domoic acid uptake by oysters feeding on Pseudo-nitzschia cells. I. Filtration and pseudofeces production. Aquatic Biology, 6: 201–212.
- McNamee, S.E., Medlin, L.K., Kegel, J., Mccoy, G.R., Raine, R., Barra, L., Ruggiero, M.V., Kooistra, W., Montresor, M., Hagstrom, J., Blanco, E.P., Graneli, E., Rodriguez, F., Escalera, L., Reguera, B., Dittami, S., Edvardsen, B., Taylor, J., Lewis, J.M., Pazos, Y., Elliott, C.T. & Campbell, K. (2016). Distribution, occurrence and biotoxin composition of the main shellfish toxin producing microalgae within European waters: a comparison of methods of analysis. Harmful Algae, 55: 112–120.
- Nézan, E., Antoine, E., Fiant, L., Amzil, Z. & Billard, C. (2006). Identification of Pseudonitzschia australis and P. multiseries in the Bay of Seine. Was there a relation to presence of domoic acid in king scallops in autumn 2004? Harmful Algae News, 31: 1–3.
- Nézan, E., Siano, R., Boulben, S., Six, C., Bilien, G., Chèze, K., Duval, A., Le Panse, S., Quéré, J. & Chomérat, N. (2014). Genetic diversity of the harmful family Kareniaceae (Gymnodiniales, Dinophyceae) in France, with the description of Karlodinium gentienii sp nov.: a new potentially toxic dinoflagellate. Harmful Algae, 40: 75–91.
- Orive, E., Perez-Aicua, L., David, H., Garcia-Etxebarria, K., Laza-Martinez, A., Seoane, S. & Miguel, I. (2013). The genus Pseudo-nitzschia (Bacillariophyceae) in a temperate estuary with description of two new species: Pseudo-nitzschia plurisecta sp nov and Pseudo-nitzschia abrensis sp nov. Journal of Phycology, 49: 1192–1206.
- Quijano-Scheggia, S., Garces, E., Sampedro, N., Van Lenning, K., Flo, E., Andree, K., Fortuno, J.M. & Camp, J. (2008). Identification and characterisation of the dominant Pseudo-nitzschia species (Bacillariophyceae) along the NE Spanish coast (Catalonia, NW Mediterranean). Scientia Marina, 72: 343–359.
- Quiroga, I. (2006). Pseudo-nitzschia blooms in the Bay of Banyuls-sur-Mer, northwestern Mediterranean Sea. Diatom Research, 21: 91–104.
- Romero, M.L.J., Kotaki, Y., Lundholm, N., Thoha, H., Ogawa, H., Relox, J.R., Terada, R., Takeda, S., Takata, Y., Haraguchi, K., Endo, T., Lim, P.-T., Kodama, M. & Fukuyo, Y. (2011). Unique amnesic shellfish toxin composition found in the South East Asian diatom Nitzschia navis-varingica. Harmful Algae, 10: 456–462.
- Ronquist, F. & Huelsenbeck, J.P. (2003). MrBayes 3: Bayesian phylogenetic inference under mixed models. Bioinformatics, 19: 1572–1574.
- Sahraoui, I., Bates, S.S., Bouchouicha, D., Mabrouk, H.H. & Hlaili, A.S. (2011). Toxicity of Pseudo-nitzschia populations from Bizerte Lagoon, Tunisia, southwest Mediterranean, and first report of domoic acid production by P. brasiliana. Diatom Research, 26: 293–303.
- Sakka Hlaili, A., Sahraoui Khalifa, I., Bouchouicha-Smida, D., Melliti Garali, S., Ksouri, J., Chalghaf, M., Bates, S.S., Lundholm, N., Kooistra, W.H.C.F., De La Iglesia, P. & Diogène, J. (2016). Toxic and potentially toxic diatom blooms in Tunisian (SW Mediterranean) waters: review of ten years of investigations. In Advances in Environmental Research (48) (Daniels, J.A., editor), 51–69. Nova Science Publishers, Hauppage, NY.
- Teng, S.T., Lim, H.C., Lim, P.T., Dao, V.H., Bates, S.S., & Leaw, C.P. (2014). Pseudo-nitzschia kodamae sp. nov. (Bacillariophyceae), a toxigenic species from the Strait of Malacca, Malaysia. Harmful Algae, 34: 17–28.
- Teng, S.T., Tan, S.N., Lim, H.C., Dao, V.H., Bates, S.S. & Leaw, C.P. (2016). High diversity of Pseudo-nitzschia along the northern coast of Sarawak (Malaysian Borneo), with descriptions of P. bipertita sp. nov. and P. limii sp. nov. (Bacillariophyceae). Journal of Phycology, 52: 973–989.
- Trainer, V.L., Wells, M.L., Cochlan, W.P., Trick, C.G., Bill, B.D., Baugh, K.A., Beall, B.F., Herndon, J. & Lundholm, N. (2009). An ecological study of a massive bloom of toxigenic Pseudo-nitzschia cuspidata off the Washington State coast. Limnology and Oceanography, 54: 1461–1474.
- Trainer, V.L., Bates, S.S., Lundholm, N., Thessen, A.E., Cochlan, W.P., Adams, N.G. & Trick, C.G. (2012). Pseudo-nitzschia physiological ecology, phylogeny, toxicity, monitoring and impacts on ecosystem health. Harmful Algae, 14: 271–300.
- Wang, Y., Xiang, P., Kang, J.H., Ye, Y.Y., Lin, G.M., Yang, Q.L., & Lin, M. (2018). Microphytoplankton community structure in the western Arctic Ocean: surface layer variability of geographic and temporal considerations in summer. Hydrobiologia, 811: 295–312.
- Zabaglo, K., Chrapusta, E., Bober, B., Kaminski, A., Adamski, M. & Bialczyk, J. (2016). Environmental roles and biological activity of domoic acid: a review. Algal Research – Biomass, Biofuels and Bioproducts, 13: 94–101.
- Zapata, M., Rodriguez, F., Fraga, S., Barra, L. & Ruggiero, M.V. (2011). Chlorophyll c pigment patterns in 18 species (51 strains) of the genus Pseudo-nitzschia (Bacillariophyceae). Journal of Phycology, 47: 1274–1280.
- Zapata, M., Fraga, S., Rodriguez, F. & Garrido, J.L. (2012). Pigment-based chloroplast types in dinoflagellates. Marine Ecology Progress Series, 465: 33–52.