ABSTRACT
The red seaweeds Porphyra umbilicalis and Grateloupia turuturu are often used for human consumption. Nevertheless, there are knowledge gaps in studies focusing on their potential for genoprotection and in vivo studies assessing effects of whole algae intake. Thus, P. umbilicalis and G. turuturu were evaluated as genome integrity- and longevity-promoters, using a somatic mutation and recombination test (SMART) and longevity assay, respectively, in Drosophila melanogaster. For the longevity assay, alga supplementation (1.25, 2.5, 5, 10 and 20% (w/w)) occurred until eclosion (emergence as adults). Flies were counted once a week until death. For SMART, the diet was supplemented with 5 and 10% P. umbilicalis and 10 and 20% G. turuturu until adults’ eye observation. Streptonigrin (SN) was added as genotoxic insult. Supplementations with 10% P. umbilicalis and 20% G. turuturu promoted longevity increases, with G. turuturu achieving the highest potential. Basal DNA damage (without SN-challenge) was reduced in flies supplemented with 10% P. umbilicalis and 10 and 20% G. turuturu. All seaweed concentrations demonstrated antigenotoxic potential against SN-induced genotoxicity. At the 10% supplementation level, a higher antigenotoxicity was displayed by G. turuturu. Overall, the best promoter of genome integrity was 20% G. turuturu. The seaweeds proved to be promoters of a safe and healthy nutrition as functional foods.
Introduction
Seaweeds have been increasingly explored within different contexts (FAO, Citation2016), as they are associated with beneficial biological activities (as a whole or as individual components/extracts) that have supported their application in several fields (e.g. agriculture (Baweja et al., Citation2016), biofiltering (Silva et al., Citation2013), biofuel (Kraan, Citation2016), the textile industry (Dawes, Citation2016), as cosmetics and hygiene products (Rupérez et al., Citation2002; Couteau & Coiffard, Citation2016), in thalassotherapy (Baweja et al., Citation2016), medicine and the pharmaceutical industry (Holdt & Kraan, Citation2011; Levine, Citation2016), biotechnology and microbiology (Holdt & Kraan, Citation2011), as well as in the animal (Pereira, Citation2015) and human food industries (Holdt & Kraan, Citation2011)). Food production is a major application, which can be divided into three main areas: food additives, dietary supplements and edible seaweeds (full intake) (Bixler & Porse, Citation2011). Moreover, seaweeds have been demonstrated to provide specific health benefits beyond basic nutrition, i.e. they have potential as functional foods (Holdt & Kraan, Citation2011; Mendis & Kim, Citation2011). Red seaweeds (phylum Rhodophyta) are of particular interest due to their unique constitution in bioactive compounds (such as R-phycoerythrin, agar and carrageenan) (Baweja et al., Citation2016) and beneficial effects (Mohamed et al., Citation2012), as well as their greater uses in food industry (Bixler & Porse, Citation2011).
In previous studies, specimens of Porphyra spp. and Grateloupia turuturu collected from the Atlantic European coast were found to be rich in proteins (Marsham et al., Citation2007; Munier et al., Citation2013), carbohydrates (Morrissey et al., Citation2001; Denis et al., Citation2010), minerals (Marsham et al., Citation2007; Rodrigues et al., Citation2015a), vitamins (Morrissey et al., Citation2001; Kendel et al., Citation2013), ω-3 polyunsaturated fatty acids (Sánchez-Machado et al., Citation2004; Kendel et al., Citation2013), glycolipids, phospholipids (Fleurence et al., Citation1994; Kendel et al., Citation2013) and phenols (Rupérez & Saura-Calixto, Citation2001; Rodrigues et al., Citation2015a). Studies of the beneficial properties of P. umbilicalis have shown reinforced skin barrier function, cell membrane structure restoration, increased epidermal hydration (Pereira, Citation2015), and antifungal (Corato et al., Citation2017), photoprotective (Guinea et al., Citation2012) and antioxidant (Cofrades et al., Citation2010) properties. G. turuturu has demonstrated antibacterial (Pang et al., Citation2006; García-Bueno et al., Citation2014), antifungal (Plouguerné et al., Citation2008), antiviral (Hudson et al., Citation1999), anticoagulant (Shanmugam & Mody, Citation2000) and antioxidant (Liu & Pang, Citation2010) actions. The availability of the P. umbilicalis genome (Brawley et al. (Citation2017) also makes it possible to better understand the biology (and evolution) of this algae group.
Studies regarding the antioxidant-promoting properties (by scavenging of oxygen free radicals) of P. umbilicalis have been exclusively focused on algal extracts assayed in vitro. These properties were associated with specimens collected from the European Atlantic (Jiménez-Escrig et al., Citation2001; Cofrades et al., Citation2010), American Atlantic (Sampath-Wiley et al., Citation2008) and Mediterranean (Oucif et al., Citation2017) coasts. To the best of our knowledge, a single study (in vitro) has addressed the antioxidant actions of G. turuturu (from the Chinese coast), with an extract showing high antioxidant enzymatic activities (Liu & Pang, Citation2010). In addition, in vivo studies evaluating full intake of P. umbilicalis and G. turuturu are lacking. Despite the antioxidant properties displayed by both seaweeds, there is a knowledge gap regarding specific actions promoting genome integrity, a critical condition for disease prevention (McKinnon, Citation2017) and, ultimately, for survival and longevity (Edifizi & Schumacher, Citation2015).
Longevity is a complex trait which depends on the interrelationships among genetic, epigenetic, environment and lifestyle factors (Govindaraju et al., Citation2015). Understanding the mechanisms that determine the ageing process remains a major scientific challenge (Edifizi & Schumacher, Citation2015). According to free radical and mitochondrial theories of ageing, oxidative damage is believed to be a major contributor to the functional decline associated with ageing (Pan et al., Citation2012; Genova & Lenaz, Citation2015). Consequently, supplementing the diet with health-promoting bioactive compounds (antioxidants) can promote the delay of the ageing process and increase longevity through modulation of enzymatic and direct antioxidant scavenging actions (Zhao et al., Citation2008; Pan et al., Citation2012). In East Asia particularly (as advocated by traditional Chinese medicine), dietary intake of seaweeds is thought to slow the ageing process and enhance longevity (Zhao et al., Citation2007; Qin, Citation2018), although scientific evidence is still sparse. An in vivo study has shown that supplementation with sulphated polysaccharides (porphyrans) from Porphyra haintenensis increased the longevity of Drosophila melanogaster (Zhao et al., Citation2008). However, there are no studies that address the effects on ageing or longevity of P. umbilicalis and G. turuturu, in any organism.
D. melanogaster is currently one of the preferred organisms for toxicological research. Recent studies emphasize the high level of conservation between D. melanogaster and humans, not only in individual domains and proteins, but also in entire complexes and multistep pathways (Ong et al., Citation2014). In particular, metabolic pathways responsible for dietary input are conserved between humans and D. melanogaster (Lemaitre & Miguel-Aliaga, Citation2013; Ong et al., Citation2014). Moreover, genotoxicological and longevity assays with D. melanogaster are commonly used for understanding the relationship between food metabolism and development of cancer and ageing. In particular, the somatic mutation and recombination test (SMART) has proven to be a suitable tool for detecting, rapidly and inexpensively, a broad range of genetic alterations (Stamenković-Radak & Andjelković, Citation2016; Mishra et al., Citation2017).
The principles of animal welfare (3Rs) have been considered in scientific research since their development over 50 years ago. In this framework, the current tendency is to replace living organisms by in vitro methods (replacement), making suitably designed animal trials that are robust and reproducible (reduction), as well as minimizing animal suffering (refinement) (Holmes et al., Citation2010). The use of D. melanogaster as a model organism respects this course of action, since, in view of the current scientific knowledge, this does not present any ethical issues (Mishra et al., Citation2017). Moreover, considering the similarities with humans described previously, D. melanogaster is a suitable replacement for higher animals (partial replacement) and, contrasting with in vitro approaches, has the advantage of enabling a more solid extrapolation at the organism level (Holmes et al., Citation2010; Mishra et al., Citation2017).
In the present work, D. melanogaster larval diet was supplemented with the red seaweeds P. umbilicalis and G. turuturu in order to evaluate their potential to promote genome integrity (as antigenotoxic agents) and lifespan extension, using SMART and a longevity assay, respectively. The ultimate goal was to elucidate the value of seaweeds as food sources to promote safe and healthy nutrition, demonstrating their potential as functional foods.
Materials and methods
Chemicals
Formula 4-24® Instant Drosophila Medium (henceforward described as instant medium) was purchased from Carolina Biological Supply Company, Burlington, North Carolina, USA. Streptonigrin (CAS no. 3930-19-6) was obtained from Santa Cruz Biotechnology, Inc., Dallas, Texas, USA. All the other chemicals were obtained from Sigma-Aldrich Chemical Company, Madrid, Spain.
Seaweeds harvesting and preparation
P. umbilicalis Kützing and G. turuturu Yamada were harvested from Mindelo beach (41°18’36.8”N, 8°44’25.9”W), Vila do Conde, and from Aguda beach (41°02’53.7”N, 8°39’14.5”W), Gaia, respectively, in the western Portuguese coast, between September/October 2015.
Seaweeds were taken to the ALGAplus company, Ílhavo, Portugal, where they were dried for 24 h in a controlled temperature chamber (25°C), to 10–12% humidity. Then, each seaweed batch was ground in a coffee mill for 1 min, obtaining particles < 2 mm, and stored in ziplock bags in a dark dry place until use for culture medium supplementation.
Drosophila melanogaster strain, phenotypes and maintenance conditions
D. melanogaster Oregon-K (OK) strain was chosen, since a study from Gaivão & Comendador (Citation1996) showed the potential of this strain for genotoxicity testing. The OK strain has high susceptibility to reactive oxygen species (ROS), mainly due to a low activity of antioxidant enzymes, and is therefore more sensitive to increase its antioxidant status upon intake of dietary antioxidants. Two distinct alleles for the sex-linked white (w) gene were involved: wild-type with red eyes (w+) and mutant with white eyes (w). The OK strain with the two different phenotypes for eye colour was kindly provided by Prof. María Sierra (University of Oviedo, Spain).
Culture medium (or standard medium) was prepared at high temperature (85–90°C), containing 100 g sucrose, 100 g yeast, 12 g agar, 5.4 g sodium chloride and 5 ml propionic acid per 1000 ml of distilled water (dH2O). Fruit fly cultures were kept in 200 ml bottles with 20 ml standard medium at 24 ± 1ºC and 60% relative humidity (RH). Thirty pairs were crossed per bottle, for egg laying, and transferred to new bottles once a week to avoid generations blending.
Handling of fruit flies was preceded by anaesthetic administration (diethyl ether) when necessary.
Longevity assay
Instant medium, dH2O and ground seaweeds were used for media preparation. Supplementation levels were chosen based on Japanese seaweed consumption, i.e. 10–25% relative to total food intake (Peinado et al., Citation2014; Collins et al., Citation2016). Thus, five concentrations of P. umbilicalis and G. turuturu were tested: 1.25, 2.5, 5, 10 and 20% (relative to medium dry weight; w/w). Experimental groups were identified by abbreviations (e.g. 1.25P, 1.25G) composed of the percentage of algal supplementation and P for P. umbilicalis and G for G. turuturu. Vials with 18 ml capacity were used, containing 2.5 ml (instant medium + seaweed) and 2.8 ml dH2O. As independent assays were carried out, negative controls were considered for each seaweed trial supplementation. For replication purposes, duplicates were performed for all conditions. Medium hydration was only carried out on the day of transferring fruit flies to the experimental vials (all conditions including controls).
Three to four days old culture flies (separated after eclosion) were etherized, separated by sex and placed into vials with standard medium (10 couples per vial). OK wild-type and OK w mutant were randomly mixed, and kept at 24 ± 1°C and 60% RH for 48 h. In the pre-crossing step (), females laid their first eggs in the standard media (in small amounts) during the 48 h, ensuring that the egg laying in the experimental media (next step) was maximum, providing a greater offspring in the shortest time possible.
Fig. 1. Experimental design of the longevity assay: (a) pre-crossing, (b) flies transfer, crossing and disposal, (c) F1 offspring development and transfer, and (d) weekly media renewal. RH, relative humidity.
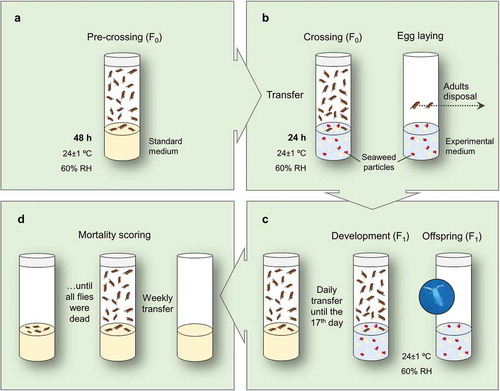
After pre-crossing, couples were transferred to vials with experimental media (hydrated just before receiving flies), incorporating seaweed particles, for crossing. Subsequently, females laid their eggs. Adults (F0) were then eliminated after 24 h of the transfer (). The offspring (F1) was chronically exposed to the experimental media, i.e. from egg up to adult eclosion. After the eclosion, the number of individuals in each experimental medium was counted and the passage to vials with standard medium was carried out daily (anaesthesia needed), until the 17th day after egg laying (). Thereafter, once a week, flies were transferred to vials with new standard medium. These passages were performed using anaesthesia, with sexing and counting of flies in order to register the differential mortality of males and females. The procedure was repeated until all individuals were dead (). The final results were expressed in days (as mean, median and maximum longevities).
Eye-spot SMART
Among the two types of SMART currently used, eye-spot test (Vogel & Zijlstra, Citation1987) is faster (compared to wing-spot test; Graf et al., Citation1984) in detecting loss of heterozygosity by deletions, point mutations, mitotic recombination and nondisjunction, with high sensitivity, specificity and accuracy (Gaivão et al., Citation1999; Marcos et al., Citation2014). Following the methodology described by Vogel & Nivard (Citation1993) for eye-spot SMART, when wild-type eyed females (w+/w+) are crossed with white eyed males (w/Y), a heterozygous offspring is developed for females (w+/w). In the offspring’s development period, genotoxic agents trigger mutagenic events that can cause the formation of white phenotype spots in the wild-type eyes. A spot is characterized by the presence of one or more white phenotype ommatidia (eye units) in a red eye.
Instant medium, ground seaweeds and phosphate-buffered saline (PBS; 0.2 M and pH 6.8) were used for experimental media preparation. PBS was used for media hydration because streptonigrin (SN) is more stable in PBS that in dH2O. SN (20 μM) was added as standard genotoxicant, allowing evaluation of the seaweeds’ actions against a genotoxic insult, as well as to distinguish the protection against basal DNA damages (DNA damages that occur, in the absence of exogenous challenges, from replication errors and other biological processes). The effects of SN in D. melanogaster were first demonstrated by Gaivão et al. (Citation1999) in eye-spot SMART, where it showed the greatest genotoxic activity at 20 μM (with the lowest lethal toxicity; unpublished work).
Two concentrations of each seaweed were chosen following the best antitoxic results from a preliminary toxicological screening (data not shown): 5% and 10% of P. umbilicalis, and 10% and 20% of G. turuturu. Briefly, the preliminary toxicological screening consisted of measuring developmental time (time from egg to adult eclosion), survival (total number of eclosions) and sex ratio in flies exposed to different seaweed species (at different concentrations). Decreased developmental times and increased survival were verified for flies supplemented with 5 and 10% of P. umbilicalis, and 10 and 20% of G. turuturu. As before, experimental groups were identified by abbreviations (5P, 10P, 10G and 20G) for the percentage of alga supplementation and species (P for P. umbilicalis and G for G. turuturu), adding ‘+SN’ to denote SN incorporation in the medium. Ten conditions were delineated (in triplicate bottles): one with 20 ml instant medium + 20 ml PBS = control – C; four with 20 ml instant medium + seaweed + 20 ml PBS = 5P, 10P, 10G and 20G; one with 20 ml instant medium + 20 ml SN (20 µM in PBS) = SN; and four with 20 ml (instant medium + seaweed) + 20 ml SN (20 µM in PBS) = 5P+SN, 10P+SN, 10G+SN and 20G+SN ().
Fig. 2. Schematic representation of the experimental design of the SMART. The first step was the pre-crossing of 20 couples (F0) in each bottle with standard medium for 2 days, followed by the crossing (F0 couples) and the subsequent egg laying (3 days) in the experimental media. F1 developed (feeding larvae and pupa) until eclosion, and the females’ eyes were observed at the stereoscopic microscope for spots scoring. Two major groups were tested, one unchallenged (boxes with black outline) and another challenged with streptonigrin (SN). For each group, five conditions were tested: no alga supplementation (C – control for unchallenged groups; SN – SN exposure), two conditions with Porphyra umbilicalis supplementation (5 and 10%), without (5P, 10P) and with SN exposure (5P+SN, 10P+SN), and two conditions with Grateloupia turuturu supplementation (10 and 20%), without (10G, 20G) and with SN exposure (10G+SN, 20G+SN). The white boxes represent no algal supplementation, while the lighter and darker boxes represent, respectively, the lower and the higher supplementation level tested for each seaweed.
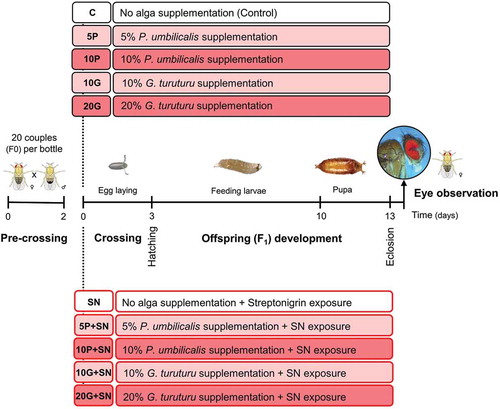
Then, w+/w+ virgin females and w/Y males (1–2 days old) were added to bottles with standard medium. Twenty couples were set per bottle at 24 ± 1°C and 60% RH for 48 h (pre-crossing). Thereafter, the experimental media (all conditions including controls) were hydrated and the fruit flies were transferred. Flies were crossed in the experimental media for 72 h and after this time they were discarded ().
As for the longevity assay, only offspring (F1) larvae were chronically exposed to the experimental media (). After eclosion, females were separated for eye observation at the stereoscopic microscope (Leica Wild M3Z stereo microscope, with Hund Wetzlar halogen optic fibre light source; 40× magnification) (). Two solutions were prepared in order to avoid light reflection in the eye observation, according to Marcos et al. (Citation2014): solution 1 with 90 ml of 96% ethanol, 9 ml of dH2O and 1 ml of TritonTM X-100; solution 2 with 10 ml of solution 1 plus 90 ml of dH2O. Eye observation consisted of counting the total number of spots per eye. The procedure was performed until 400 eyes were analysed per condition (200 females). The different spot sizes were assumed as a single class. The inhibition percentage (IP) was calculated as the proportion of decrease for spots frequency in the different conditions with seaweed supplementation (without insult) when compared with control (non-supplemented; C), as described by Abraham (Citation1994), adopting the following formula: [(control − condition with seaweed)/control] × 100.
Conditions with SN exposure were analysed in the same way.
Data evaluation and statistical analysis
Statistical analyses of the longevity data were performed using online application for survival analysis (OASIS) (Yang et al., Citation2011) and online application for survival analysis 2 (OASIS 2) (Han et al., Citation2016). Using Kaplan–Meier survival analysis mean, median and maximum values for longevity were obtained for both genders independently (Kaplan & Meier, Citation1958). Mantel–Cox tests were used to assess the statistical significance of differences in mean longevity (Mantel, Citation1966) and Wang–Allison tests to assess the statistical significance of differences in median and maximum longevity data (Wang et al., Citation2004). The replicates in each condition (in terms of survivors per day) were summed separately for males and for females. Differences were considered significant for Mantel–Cox when χ2 value(1) > 3.84, p < 0.05; and for Wang–Allison when p < 0.05.
Microsoft Office Excel 2016 (Microsoft Corporation, USA) was used to perform the statistical analyses for SMART data. χ2 goodness of fit tests (Franke et al., Citation2012) were performed between the replicates for each condition. The replicates were summed in terms of spots, giving rise to spots per 400 eyes for each condition. The procedure used by Frei & Würgler (Citation1988) was followed to compare these values. Considering the formulation of an H0, which states that the number of spots is equal between two conditions tested, and an H1, stating that the spots number of a certain condition is m (multiplication factor) times the mutant spots of another condition. This allowed four diagnostics to be obtained through a multiple-decision procedure: positive, weak positive, negative or inconclusive (Selby & Olson, Citation1981). To test the hypotheses, a χ2 test of homogeneity for proportions was applied (α = 0.05). The m = 0.5 was used when conditions with or without SN challenge were compared within themselves, and m = 5 when the comparisons concerned conditions with versus without SN challenge. An inconclusive result was analysed with the one-tailed Mann–Whitney U test (Mann & Whitney, Citation1947). U test differences were significant when z < −1.645, p < 0.05.
Results
Longevity assay
Supplementation with Porphyra umbilicalis
Regarding males that ingested P. umbilicalis, statistically significant longevity increases were found for 10P (mean, χ2 value(1) = 4.69, p = 0.030; median, p = 0.039) and 20P (median, p = 0.045) when compared with the control (non-supplemented group) (). Mean longevities increased by 17.95% (9.55 days) and 14.15% (7.53 days) in 10P and 20P, respectively, in relation to the control, and median longevities increased 12.50% (7 days) for both 10P and 20P relative to the control. There were no significant differences for mean, median or maximum longevities for the remaining comparisons ().
Table 1. Mean, median and maximum longevity values obtained for Drosophila melanogaster males and females following Porphyra umbilicalis supplementation, performing Kaplan–Meier survival analysis (Kaplan & Meier, Citation1958).
Of the females that ingested P. umbilicalis, the survivorship percentage per day for 10P stood out, as statistically significant longevity increases were found (mean, χ2 value(1) > 3.84, p < 0.05; median, p < 0.05) when compared with the control, 1.25P and 5P (). The mean longevity of 10P increased by 17.35% (9.52 days) and median 11.11% (7 days) in relation to the control. When compared with 20P, 10P displayed a significant increase in median longevity (p = 0.031). The comparison of 5P vs 20P also showed a significant increase in longevity for 20P (median, p = 0.016). For the remaining comparisons, there were no significant differences ().
The comparisons of longevity between males and females of the control and of groups supplemented with P. umbilicalis displayed no significant differences.
Supplementation with Grateloupia turuturu
The males supplemented with G. turuturu 20G displayed significant longevity increases (mean, χ2 value(1) > 3.84, p < 0.05; median, p < 0.05) relative to the control, 1.25G and 10G (). Mean longevity increased 27.09% (14.41 days), and median longevity 25% (14 days), for 20G relative to the control. There were no significant differences for the remaining comparisons ().
Table 2. Mean, median and maximum longevity values obtained for Drosophila melanogaster males and females following Grateloupia turuturu supplementation, performing Kaplan–Meier survival analysis (Kaplan & Meier, Citation1958).
Similarly to males, the females that had 20G added to their diet showed significant increases for mean (χ2 value(1) > 3.84, p < 0.05) and median (p < 0.05) longevities when compared with the control, 1.25G and 10G, and for median longevity only in comparison to 2.5G (p = 0.008) (). For mean longevity, there was an increase of 22.05% (12.10 days) for 20G with respect to the control. Regarding median longevity, the increase was 11.11% (7 days) from control to 20G. In the comparison of 1.25G vs 10G, significant increases occurred for 10G in relation to median longevity (p = 0.045) and for 1.25G in relation to maximum longevity (p = 0.045). For the remaining comparisons, there were no significant differences of longevity ().
As for P. umbilicalis supplementation, the comparisons between the males and females supplemented with G. turuturu demonstrated no significant differences.
SMART
Concerning unchallenged groups, the spots frequency significantly decreased (m = 0.5, i.e. by half) in 10P, 10G and 20G relative to the control group (C; non-supplemented). Regarding C versus 5P, the multiple-decision was inconclusive and the Mann–Whitney U test demonstrated a non-significant difference (z = −1.528, p = 0.063) ().
Fig. 3. Spots frequency (spots per 400 eyes) observed in the Drosophila melanogaster eyes (SMART). The table shows the statistical diagnosis for the comparisons of the spots frequency (only comparable conditions are shown; +, positive result; w+, weak positive result; -, negative result; i, inconclusive result), according to Frei & Würgler (Citation1988). Tested groups are identified by abbreviations (5P, 10P, 10G and 20G; percentage of alga supplementation and P for Porphyra umbilicalis and G for Grateloupia turuturu); ‘+SN’ denotes streptonigrin incorporation in the medium; C (no alga supplementation) and SN (no alga supplementation plus SN exposure) were used for comparison purposes. Bars and cells (in the table) with black outline correspond to groups unchallenged and bars and cells without black outline correspond to SN-challenged groups. White bars and cells represent no alga supplementation, the lighter bars and cells represent the lower concentration tested and the darker bars and cells the higher concentration tested for each seaweed; m = multiplication factor.
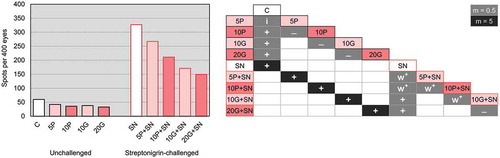
All the conditions challenged with SN significantly increased (m = 5, i.e. five times) the number of spots relative to the unchallenged conditions (). In addition, the spots frequency in all groups challenged with SN and supplemented with algae (5P+SN, 10P+SN, 10G+SN and 20G+SN) significantly decreased relative to the non-supplemented group (SN) (). The multiple-decision showed the reduction of spots by half in 10G+SN and 20G+SN in relation to SN. For 5P+SN and 10P+SN, the reductions were less impressive (weak positive result). In the comparisons of 5P+SN versus 10P+SN and 10P+SN versus 10G+SN, weak positive results were shown as well ().
To better appraise the effect of seaweed supplementation, the inhibition percentage (IP) was calculated for both challenged and unchallenged conditions (). Increasing IPs were verified for all unchallenged conditions as the seaweed concentration increased: 5P (29.93%), 10P (40.07%), 10G (36.60%) and 20G (46.93%). Comparing the same supplementation level of both seaweeds, the IP was slightly higher (3.47%) in 10P relative to 10G ().
Fig. 4. Inhibition percentages of the SMART conditions. Tested groups are identified by abbreviations (5P, 10P, 10G and 20G) composed by the percentage of alga supplementation and a capital letter identifying the species (P for Porphyra umbilicalis and G for Grateloupia turuturu), adding ‘+SN’ to denote the streptonigrin incorporation in the medium. The lighter bars represents the lower concentration tested and the darker bars the higher concentration tested for each seaweed.
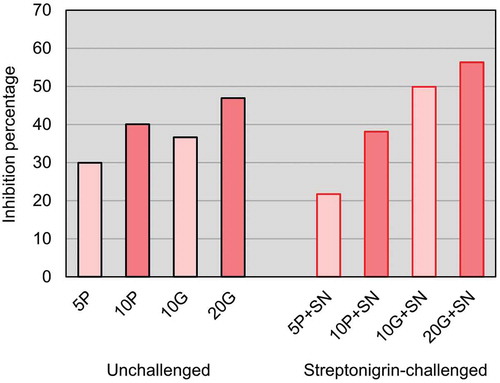
Concerning the SN challenged conditions, IPs also increased with the increasing concentration of seaweed tested (). For P. umbilicalis supplementation the IPs were: 5P+SN (21.72%) and 10P+SN (38.12%); for G. turuturu supplementation they were: 10G+SN (49.89%) and 20G+SN (56.33%). Thus, 20G+SN demonstrated the highest inhibition (for both SN challenged and unchallenged conditions) and, comparing the same concentration for both seaweeds in challenged conditions, a higher IP occurred for G. turuturu (increase of 11.77% more) ().
Higher IPs were identified in 5P compared with 5P+SN and in 10P relative to 10P+SN. However, for 10G, the IP obtained was lower than for 10G+SN, and the same occurred in 20G in comparison to 20G+SN ().
Discussion
For both male and female D. melanogaster, supplementation with 10P and 20G during larval development led to increased mean and median longevities. Accordingly, G. turuturu demonstrated a greater longevity-promoting potential than P. umbilicalis. The mean longevity increases were not statistically significant for 20P, which may illustrate that a specific concentration of this seaweed is required for the longevity-enhancing actions. Hence, it would be pertinent to test intermediate concentrations of both seaweeds to clarify this point.
Despite a lack of studies directly evaluating longevity effects of dietary supplementation with the seaweed species used, some have focused on compounds commonly present in the algae, such as porphyrans (MacArtain et al., Citation2007), fucoxanthin, β-carotene, lutein (Ferraces-Casais et al., Citation2012) and vitamin E (Ferraces-Casais et al., Citation2012; Kendel et al., Citation2013). Porphyrans (from P. haitanensis) increased mean longevity of flies, indicating their free radical scavenging action (Zhao et al., Citation2008). Mean longevity was also enhanced when two carotenoids, fucoxanthin and β-carotene, were administered (individually) to fruit flies (Ekaterina et al., Citation2015). Supplementation with another pigment, lutein, prolonged mean and median longevities, which was consistent with an increase of antioxidant enzymes activity (Zhang et al., Citation2014). According to Driver & Georgeou (Citation2003), D. melanogaster mean longevity increased on a diet supplemented with vitamin E. However, the longevity increases were not as high as for supplementation with 10P and 20G. These differences may have occurred owing to the use of different D. melanogaster strains, the fact that supplementation was performed during adult life stages in these studies (the impact of these compounds could be life cycle stage-dependent; see Soh et al. Citation2013) and/or the combined effect of several compounds present in the whole seaweed in comparison with isolated compounds.
Additionally, according to Rodrigues et al. (Citation2015b), when feeding provides a low protein to carbohydrate ratio, D. melanogaster longevity significantly increases. Taking into account the protein (15–37%; w/w) and carbohydrate (50–76%; w/w) contents of P. umbilicalis (Morrissey et al., Citation2001), and G. turuturu (22.9%; w/w protein), (60.4%; w/w carbohydrate) (Denis et al., Citation2010), it can be seen that there is a lower percentage of proteins in both seaweeds, resulting in a low ratio of protein to carbohydrate which could lead to the longevity increases verified for the flies fed with 10P and 20G.
Considering basal DNA damages, 5P was not able to significantly reduce DNA damage, which appears to demonstrate that this concentration is not sufficient to promote genome protection. The significant spots reductions for all SN-challenged conditions supplemented with seaweed (compared with the non-supplemented one – SN), showed the potential of the seaweeds in counteracting basal DNA damage, as well as the genotoxicity developed by SN. The supplementation with 5% of P. umbilicalis demonstrated genome protection when a higher genotoxic insult was considered (SN insult), even though it had the lowest protection.
SN genotoxic effects include the formation of DNA adducts, which consist of SN-metal-DNA complexes (Donohoe et al., Citation2013). The prolonged SN linkage and ROS continuous production can inhibit the synthesis of DNA and RNA, induce unscheduled DNA synthesis and promote strand breaks. Additionally, SN inhibits topoisomerase II by stabilizing cleavable complexes (Deepa et al., Citation2011). These actions create genomic instability in cells and, consequently, may develop into carcinogenic events (Bolzán & Bianchi, Citation2001). As SN imposes oxidative stress in cells, high levels of antioxidants can suppress SN activity (Bolzán & Bianchi, Citation2001; Troxell et al., Citation2012). Therefore, P. umbilicalis and G. turuturu may display their antigenotoxic potential by increasing/improving the fruit flies’ antioxidant mechanisms, acting as dietary antioxidants and protecting flies from SN damage to the genome.
Common compounds present in the tested seaweed species, including boron (Rodrigues et al., Citation2015a), selenium (Cofrades et al., Citation2010), β-carotene, chlorophyll a (Ferraces-Casais et al., Citation2012) and vitamin C (Ferraces-Casais et al., Citation2012), have shown antigenotoxicity in SMART: boron was able to prevent ethyl methanesulphonate genotoxicity (Sarıkaya et al., Citation2016); the antigenotoxic effects of sodium selenite, a selenium compound, were demonstrated in the co-treatment with potassium dichromat (Rizki et al., Citation2001); β-carotene demonstrated antigenotoxicity against doxorubicin (Dias et al., Citation2009); chlorophyll a and vitamin C demonstrated their antigenotoxicity against acrolein and malondialdehyde (Demir et al., Citation2013); and vitamin C inhibited the genotoxicity displayed by methyl urea plus sodium nitrite (Graf et al., Citation1998). No compound was able to show protection against basal DNA damages only (without an exogenous genotoxic insult), which contrasts with the results for 10P, 10G and 20G. This may indicate greater genome protective actions of whole seaweeds (our work) relative to each of their compounds tested individually (previous studies).
Oxidative damage to the genome is believed to be a major cause of mutations occurring in genes regulating the cell cycle, resulting in cancerous cells (Panieri & Santoro, Citation2016), as well as being the main contributor to the functional decline associated with ageing (Genova & Lenaz, Citation2015). Thus, antioxidant mechanisms are required for cancer prevention and delaying the ageing process. By supplementing the regular diet with antioxidants, a greater antioxidant protection can be obtained (Pan et al., Citation2012; Panieri & Santoro, Citation2016). It is possible therefore that the antigenotoxic and longevity-promoting potential displayed by P. umbilicalis and G. turuturu are connected to the most common antioxidant mechanisms seen in other dietary antioxidants. P. umbilicalis and G. turuturu may exhibit their antigenotoxicity and longevity-promotion by scavenging ROS directly, donating electrons and/or protons to enzymatic and/or endogenous non-enzymatic antioxidants for ROS conversion, and/or chelating metal ions responsible of producing hydroxyl radicals through Fenton reaction (Fernández-Bedmar et al., Citation2011; Panieri & Santoro, Citation2016).
For future research, it would be pertinent to carry out chemical characterizations of the species used, together with screenings of their bioactive compounds, in order to identify the source(s) of the antigenotoxic and longevity-promoting actions. However, as the fruit flies’ diet was supplemented with whole seaweeds (not individual compounds), the identification of the bioactive compound(s) linked to the protective actions displayed can be difficult, since a complex net of interactions is likely to occur.
The findings from this study contribute to understanding the action mechanisms and potentials of P. umbilicalis and G. turuturu as promoters of safe and healthy nutrition as functional foods. Hereafter, it is intended to boost in vivo studies with edible seaweeds to further explore the harvest (promoting socioeconomic development) and intake (promoting health and well-being) of these natural resources.
Author contributions
J. Ferreira: practical work, data analysis, manuscript writing; A. Marques: seaweeds harvesting, practical work, manuscript editing; H. Abreu, R. Pereira and A. Rego: seaweeds harvesting, identification and dehydration; M. Pacheco and I. Gaivão: original concept, manuscript editing.
Disclosure statement
No potential conflict of interest was reported by the authors.
Additional information
Funding
References
- Abraham, S.K. (1994). Antigenotoxicity of coffee in the Drosophila assay for somatic mutation and recombination. Mutagenesis, 9: 383–386.
- Baweja, P., Kumar, S., Sahoo, D. & Levine, I. (2016). Biology of seaweeds. In Seaweed in Health and Disease Prevention (Fleurence, J. & Levine, I., editors), 41–106. Academic Press, London.
- Bixler, H.J. & Porse, H. (2011). A decade of change in the seaweed hydrocolloids industry. Journal of Applied Phycology, 23: 321–335.
- Bolzán, A.D. & Bianchi, M.S. (2001). Genotoxicity of streptonigrin: a review. Mutation Research, 488: 25–37.
- Brawley, S.H., Blouin, N.A., Ficko-Blean, E., Wheeler, G., Lohr, M., Goodson, H.V., Jenkins, J., Blaby-Haas, C.E., Helliwell, K., Chan, C.X., Marriage, T., Bhattacharya, D., Klein, A., Badis, Y., Brodie, J., Cao, Y., Collen, J., Dittami, S., Gachon, C., Green, B., Karpowicz, S., Kim, J., Lin, S., Michel, G., Mittag, M., Olson, B., Pangilinan, J., Peng, Y., Qiu, H., Riek, S.Z., Shu, S.Q., Singer, J., Smith, A., Sprecher, B., Wagner, V., Wang, W., Wang, Z., Yan, Z., Yarish, C., Zhuang, Y.Y., Zou, Y., Lindquist, E., Grimwood, J., Barry, K., RoksharD., Schumtz, J., Gantt, E., Stiller, J., Grossman, A., Prochnik, S. (2017). Insights into the red algae and eukaryotic evolution from the genome of Porphyra umbilicalis (Bangiophyceae, Rhodophyta). Proceedings of the National Academy of Sciences USA, 114: E6361–E6370.
- Cofrades, S., López-López, I., Bravo, L., Ruiz-Capillas, C., Bastida, S., Larrea, M.T. & Jiménez-Colmenero, F. (2010). Nutritional and antioxidant properties of different brown and red Spanish edible seaweeds. Food Science and Technology International, 16: 361–370.
- Collins, K.G., Fitzgerald, G.F., Stanton, C. & Ross, R.P. (2016). Looking beyond the terrestrial: the potential of seaweed derived bioactives to treat non-communicable diseases. Marine Drugs, 14: 1–31.
- Corato, U.D., Salimbeni, R., Pretis, A.D., Avella, N. & Patruno, G. (2017). Antifungal activity of crude extracts from brown and red seaweeds by a supercritical carbon dioxide technique against fruit postharvest fungal diseases. Postharvest Biology and Technology, 131: 16–30.
- Couteau, C. & Coiffard, L. (2016). Seaweed application in cosmetics. In Seaweed in Health and Disease Prevention (Fleurence, J. & Levine, I., editors), 423–441. Academic Press, London.
- Dawes, C. (2016). Macroalgae systematics. In Seaweed in Health and Disease Prevention (Fleurence, J. & Levine, I., editors), 107–148. Academic Press, London.
- Deepa, P.V., Akshaya, A.S. & Solomon, F.D.P. (2011). Anthracycline (epirubicin) induced mutation studies in Drosophila melanogaster. Drosophila Information Service, 94: 53–61.
- Demir, E., Kaya, B. & Cenkci, S.K. (2013). Antigenotoxic activities of ascorbic acid, chlorophyll a, and chlorophyll b in acrolein and malondialdehyde-induced genotoxicity in Drosophila melanogaster. Ekoloji, 22: 36–42.
- Denis, C., Morançais, M., Li, M., Deniaud, E., Gaudin, P., Wielgosz-Collin, G., Barnathan, G., Jaouen, P. & Fleurence, J. (2010). Study of the chemical composition of edible red macroalgae Grateloupia turuturu from Brittany (France). Food Chemistry, 119: 913–917.
- Dias, C.D., Araújo, B.C., Dutra, E.S. & Nepomuceno, J.C. (2009). Protective effects of β-carotene against the genotoxicity of doxorubicin in somatic cells of Drosophila melanogaster. Genetics and Molecular Research, 8: 1367–1375.
- Donohoe, T.J., Jones, C.R., Kornahrens, A.F., Barbosa, L.C.A., Walport, L.J., Tatton, M.R., O’Hagan, M., Rathi, A.H. & Baker, D.B. (2013). Total synthesis of the antitumor antibiotic (±)-streptonigrin: first- and second-generation routes for de novo pyridine formation using ring-closing metathesis. Journal of Organic Chemistry, 78: 12338–12350.
- Driver, C. & Georgeou, A. (2003). Variable effects of vitamin E on Drosophila longevity. Biogerontology, 4: 91–95.
- Edifizi, D. & Schumacher, B. (2015). Genome instability in development and aging: insights from nucleotide excision repair in humans, mice, and worms. Biomolecules, 5: 1855–1869.
- Ekaterina, L., Ekaterina, P., Svetlana, Z., Oksana, S., Elena, M., Sergey, L., Alex, M., Alex, Z. & Alexey, M. (2015). Fucoxanthin increases lifespan of Drosophila melanogaster and Caenorhabditis elegans. Pharmacological Research, 100: 228–241.
- FAO (2016). The State of World Fisheries and Aquaculture 2016. FAO, Rome.
- Fernández-Bedmar, Z., Anter, J., Cruz-Ares, S.L., Muñoz-Serrano, A., Alonso-Moraga, Á. & Pérez-Guisado, J. (2011). Role of citrus juices and distinctive components in the modulation of degenerative processes: genotoxicity, antigenotoxicity, cytotoxicity, and longevity in Drosophila. Journal of Toxicology and Environmental Health, Part A, 74: 1052–1066.
- Ferraces-Casais, P., Lage-Yusty, M.A., Quirós, A.R.-B.d., & López-Hernández, J. (2012). Evaluation of bioactive compounds in fresh edible seaweeds. Food Analytical Methods, 5: 828–834.
- Fleurence, J., Gutbier, G., Mabeau, S. & Leray, C. (1994). Fatty acids from 11 marine macroalgae of the French Brittany coast. Journal of Applied Phycology, 6: 527–532.
- Franke, T.M., Ho, T. & Christie, C.A. (2012). The chi-square test: often used and more often misinterpreted. American Journal of Evaluation, 33: 448–458.
- Frei, H. & Würgler, F.E. (1988). Statistical methods to decide whether mutagenicity test data from Drosophila assays indicate a positive, negative, or inconclusive result. Mutation Research, 203: 297–308.
- Gaivão, I. & Comendador, M.A. (1996). The w/w+ somatic mutation and recombination test (SMART) of Drosophila melanogaster for detecting reactive oxygen species: characterization of 6 strains. Mutation Research, 360: 145–151.
- Gaivão, I., Sierra, L.M. & Comendador, M.A. (1999). The w/w+ SMART assay of Drosophila melanogaster detects the genotoxic effects of reactive oxygen species inducing compounds. Mutation Research, 440: 139–145.
- García-Bueno, N., Decottignies, P., Turpin, V., Dumay, J., Paillard, C., Stiger-Pouvreau, V., Kervarec, N., Pouchus, Y.-F., Marín-Atucha, A.A. & Fleurence, J. (2014). Seasonal variation in the antivibrio activity of two organic extracts from two red seaweed: Palmaria palmata and the introduced Grateloupia turuturu against the abalone pathogen Vibrio harveyi. Aquatic Living Resources, 27: 83–89.
- Genova, M.L. & Lenaz, G. (2015). The interplay between respiratory supercomplexes and ROS in aging. Antioxidants & Redox Signaling, 23: 208–238.
- Govindaraju, D., Atzmon, G. & Barzilai, N. (2015). Genetics, lifestyle and longevity: lessons from centenarians. Applied and Translational Genomics, 4: 23–32.
- Graf, U., Würgler, F.E., Katz, A.J., Frei, H., Juon, H., Hall, C.B., & Kale, P.G. (1984). Somatic mutation and recombination test in Drosophila melanogaster. Environmental Mutagenesis, 6: 153–188.
- Graf, U., Abraham, S.K., Guzman-Rincon, J. & Würgler, F.E. (1998). Antigenotoxicity studies in Drosophila melanogaster. Mutation Research, 402: 203–209.
- Guinea, M., Franco, V., Araujo-Bazán, L., Rodríguez-Martín, I. & González, S. (2012). In vivo UVB-photoprotective activity of extracts from commercial marine macroalgae. Food and Chemical Toxicology, 50: 1109–1117.
- Han, S.K., Lee, D., Lee, H., Kim, D., Son, H.G., Yang, J.-S., Lee, S.-J.V. & Kim, S. (2016). OASIS 2: online application for survival analysis 2 with features for the analysis of maximal lifespan and healthspan in aging research. Oncotarget, 7: 56147–56152.
- Holdt, S.L. & Kraan, S. (2011). Bioactive compounds in seaweed: functional food applications and legislation. Journal of Applied Phycology, 23: 543–597.
- Holmes, A.M., Creton, S. & Chapman, K. (2010). Working in partnership to advance the 3Rs in toxicity testing. Toxicology, 267: 14–19.
- Hudson, J.B., Kim, J.H., Lee, M.K., DeWreede, R.E. & Hong, Y.K. (1999). Antiviral compounds in extracts of Korean seaweeds: evidence for multiple activities. Journal of Applied Phycology, 10: 427–434.
- Jiménez-Escrig, A., Jiménez-Jiménez, I., Pulido, R. & Saura-Calixto, F. (2001). Antioxidant activity of fresh and processed edible seaweeds. Journal of the Science of Food and Agriculture, 81: 530–534.
- Kaplan, E.L. & Meier, P. (1958). Nonparametric estimation from incomplete observations. Journal of the American Statistical Association, 53: 457–481.
- Kendel, M., Couzinet-Mossion, A., Viau, M., Fleurence, J., Barnathan, G. & Wielgosz-Collin, G. (2013). Seasonal composition of lipids, fatty acids, and sterols in the edible red alga Grateloupia turuturu. Journal of Applied Phycology, 25: 425–432.
- Kraan, S. (2016). Seaweed and alcohol: biofuel or booze? In Seaweed in Health and Disease Prevention (Fleurence, J. & Levine, I., editors), 169–184. Academic Press, London.
- Lemaitre, B. & Miguel-Aliaga, I. (2013). The digestive tract of Drosophila melanogaster. Annual Review of Genetics, 47: 377–404.
- Levine, I. (2016). Algae: a way of life and health. In Seaweed in Health and Disease Prevention (Fleurence, J. & Levine, I., editors), 1–5. Academic Press, London.
- Liu, F. & Pang, S.J. (2010). Stress tolerance and antioxidant enzymatic activities in the metabolisms of the reactive oxygen species in two intertidal red algae Grateloupia turuturu and Palmaria palmata. Journal of Experimental Marine Biology and Ecology, 382: 82–87.
- MacArtain, P., Gill, C.I.R., Brooks, M., Campbell, R. & Rowland, I.R. (2007). Nutritional value of edible seaweeds. Nutrition Reviews, 65: 535–543.
- Mann, H.B. & Whitney, D.R. (1947). On a test of whether one of two random variables is stochastically larger than the other. Annals of Mathematical Statistics, 18: 50–60.
- Mantel, N. (1966). Evaluation of survival data and two new rank order statistics arising in its consideration. Cancer Chemotherapy Reports, 50: 163–170.
- Marcos, R., Sierra, L.M. & Gaivão, I. (2014). The SMART assays of Drosophila: wings and eyes as target tissues. In Genotoxicity and DNA Repair: A Practical Approach (Sierra, L.M. & Gaivão, I., editors), 283–295. Humana Press, New York.
- Marsham, S., Scott, G.W. & Tobin, M.L. (2007). Comparison of nutritive chemistry of a range of temperate seaweeds. Food Chemistry, 100: 1331–1336.
- McKinnon, P.J. (2017). Genome integrity and disease prevention in the nervous system. Genes and Development, 31: 1180–1194.
- Mendis, E. & Kim, S.-K. (2011). Present and future prospects of seaweeds in developing functional foods. Advances in Food and Nutrition Research, 64: 1–15.
- Mishra, N., Srivastava, R., Agrawal, U.R. & Tewari, R.R. (2017). An insight into the genotoxicity assessment studies in dipterans. Mutation Research, 773: 220–229.
- Mohamed, S., Hashim, S.N. & Rahman, H.A. (2012). Seaweeds: a sustainable functional food for complementary and alternative therapy. Trends in Food Science and Technology, 23: 83–96.
- Morrissey, J., Kraan, S. & Guiry, M.D. (2001). A Guide to Commercially Important Seaweeds on the Irish Coast. Bord Iascaigh Mhara, Dublin.
- Munier, M., Dumay, J., Morançais, M., Jaouen, P. & Fleurence, J. (2013). Variation in the biochemical composition of the edible seaweed Grateloupia turuturu Yamada harvested from two sampling sites on the Brittany Coast (France): the influence of storage method on the extraction of the seaweed pigment R-phycoerythrin. Journal of Chemistry, 2013: 1–8.
- Ong, C., Yung, L.-Y.L., Cai, Y., Bay, B.-H. & Baeg, G.-H. (2014). Drosophila melanogaster as a model organism to study nanotoxicity. Nanotoxicology, 9: 396–403.
- Oucif, H., Adjout, R., Sebahi, R., Boukortt, F.O., Ali-Mehidi, S. & Abi-Ayad, S.-M.E.-A. (2017). Comparison of in vitro antioxidant activity of some selected seaweeds from Algerian West Coast. African Journal of Biotechnology, 16: 1474–1480.
- Pan, M.-H., Lai, C.-S., Tsai, M.-L., Wu, J.-C. & Ho, C.-T. (2012). Molecular mechanisms for anti-aging by natural dietary compounds. Molecular Nutrition and Food Research, 56: 88–115.
- Pang, S.J., Xiao, T., Shan, T.F., Wang, Z.F. & Gao, S.Q. (2006). Evidences of the intertidal red alga Grateloupia turuturu in turning Vibrio parahaemolyticus into non-culturable state in the presence of light. Aquaculture, 260: 369–374.
- Panieri, E. & Santoro, M.M. (2016). ROS homeostasis and metabolism: a dangerous liason in cancer cells. Cell Death and Disease, 7: e2253.
- Peinado, I., Girón, J., Koutsidis, G. & Ames, J.M. (2014). Chemical composition, antioxidant activity and sensory evaluation of five different species of brown edible seaweeds. Food Research International, 66: 36–44.
- Pereira, L. (2015). Seaweed flora of the European North Atlantic and Mediterranean. In Handbook of Marine Biotechnology (Kim, S.-K., editor), 65–178. Springer, Berlin.
- Plouguerné, E., Hellio, C., Deslandes, E., Véron, B. & Stiger-Pouvreau, V. (2008). Anti-microfouling activities in extracts of two invasive algae: Grateloupia turuturu and Sargassum muticum. Botanica Marina, 51: 202–208.
- Qin, Y. (2018). Applications of bioactive seaweed substances in functional food products. In Bioactive Seaweeds for Food Applications: Natural Ingredients for Healthy Diets (Qin, Y., editor), 111–132. Academic Press, London.
- Rizki, M., Amrani, S., Creus, A., Xamena, N. & Marcos, R. (2001). Antigenotoxic properties of selenium: studies in the wing spot test in Drosophila. Environmental and Molecular Mutagenesis, 37: 70–75.
- Rodrigues, D., Freitas, A.C., Pereira, L., Rocha-Santos, T.A.P., Vasconcelos, M.W., Roriz, M., Rodríguez-Alcalá, L.M., Gomes, A.M.P. & Duarte, A.C. (2015a). Chemical composition of red, brown and green macroalgae from Buarcos bay in Central West Coast of Portugal. Food Chemistry, 183: 197–207.
- Rodrigues, M.A., Martins, N.E., Balancé, L.F., Brooma, L.N., Dias, A.J.S., Fernandes, A.S.D., Rodrigues, F., Sucena, É. & Mirth, C.K. (2015b). Drosophila melanogaster larvae make nutritional choices that minimize developmental time. Journal of Insect Physiology, 81: 69–80.
- Rupérez, P. & Saura-Calixto, F. (2001). Dietary fibre and physicochemical properties of edible Spanish seaweeds. European Food Research and Technology, 212: 349–354.
- Rupérez, P., Ahrazem, O. & Leal, J.A. (2002). Potential antioxidant capacity of sulfated polysaccharides from the edible marine brown seaweed Fucus vesiculosus. Journal of Agricultural and Food Chemistry, 50: 840–845.
- Sampath-Wiley, P., Neefus, C.D. & Jahnke, L.S. (2008). Seasonal effects of sun exposure and emersion on intertidal seaweed physiology: fluctuations in antioxidant contents, photosynthetic pigments and photosynthetic efficiency in the red alga Porphyra umbilicalis Kützing (Rhodophyta, Bangiales). Journal of Experimental Marine Biology and Ecology, 361: 83–91.
- Sánchez-Machado, D.I., López-Cervantes, J., López-Hernández, J. & Paseiro-Losada, P. (2004). Fatty acids, total lipid, protein and ash contents of processed edible seaweeds. Food Chemistry, 85: 439–444.
- Sarıkaya, R., Erciyas, K., Kara, M.I., Sezer, U., Erciyas, A.F. & Ay, S. (2016). Evaluation of genotoxic and antigenotoxic effects of boron by the somatic mutation and recombination test (SMART) on Drosophila. Drug and Chemical Toxicology, 39: 400–406.
- Selby, P.B. & Olson, W.H. (1981). Methods and criteria for deciding whether specific-locus mutation-rate data in mice indicate a positive, negative or inconclusive result. Mutation Research, 83: 403–418.
- Shanmugam, M. & Mody, K.H. (2000). Heparinoid-active sulphated polysaccharides from marine algae as potential blood anticoagulant agents. Current Science, 79: 1672–1683.
- Silva, M., Vieira, L., Almeida, A.P. & Kijjoa, A. (2013). The marine macroalgae of the genus Ulva: chemistry, biological activities and potential applications. Oceanography, 1: 1–6.
- Soh, J.-W., Marowsky, N., Nichols, T.J., Rahman, A.M., Miah, T., Sarao, P., Khasawneh, R., Unnikrishnan, A., Heydari, A.R., Silver, R.B. & Arking, R. (2013). Curcumin is an early-acting stage-specific inducer of extended functional longevity in Drosophila. Experimental Gerontology, 48: 229–239.
- Stamenković-Radak, M. & Andjelković, M. (2016). Studying genotoxic and antimutagenic effects of plant extracts in Drosophila test systems. Botanica Serbica, 40: 21–28.
- Troxell, B., Xu, H. & Yang, X.F. (2012). Borrelia burgdorferi, a pathogen that lacks iron, encodes manganese-dependent superoxide dismutase essential for resistance to streptonigrin. Journal of Biological Chemistry, 287: 19284–19293.
- Vogel, E.W. & Nivard, M.J.M. (1993). Performance of 181 chemicals in a Drosophila assay predominantly monitoring interchromosomal mitotic recombination. Mutagenesis, 8: 57–81.
- Vogel, E.W. & Zijlstra, J.A. (1987). Mechanistic and methodological aspects of chemically induced somatic mutation and recombination in Drosophila melanogaster. Mutation Research, 182: 243–264.
- Wang, C., Li, Q., Redden, D.T., Weindruch, R. & Allison, D.B. (2004). Statistical methods for testing effects on “maximum lifespan”. Mechanisms of Ageing and Development, 125: 629–632.
- Yang, J.-S., Nam, H.-J., Seo, M., Han, S.K., Choi, Y., Nam, H.G., Lee, S.-J. & Kim, S. (2011). OASIS: online application for the survival analysis of lifespan assays performed in aging research. PLoS ONE, 6: e23525.
- Zhang, Z., Han, S., Wang, H. & Wang, T. (2014). Lutein extends the lifespan of Drosophila melanogaster. Archives of Gerontology and Geriatrics, 58: 153–159.
- Zhao, T., Zhang, Q., Qi, H. & Li, Z. (2007). Positive effect of porphyrans on the lifespan and vitality of Drosophila melanogaster. Chinese Journal of Oceanology and Limnology, 25: 373–377.
- Zhao, T., Zhanga, Q., Qic, H., Liud, X. & Li, Z. (2008). Extension of life span and improvement of vitality of Drosophila melanogaster by long-term supplementation with different molecular weight polysaccharides from Porphyra haitanensis. Pharmacological Research, 57: 67–72.