ABSTRACT
Marine species of the oomycete genus Olpidiopsis that infect cultivated red macroalgae, most notably Pyropia spp., are one of the main causes of economic loss in the Asian seaweed industry. We recently described novel Olpidiopsis species infecting red algae in Scotland, and thus hypothesized that this genus is more abundant and widespread than previously recognized.
Here, we show that the eukaryotic microbiome of macroscopically healthy Porphyra umbilicalis thalli frequently contains marker genes closely related to Olpidiopsis. Thanks to a custom pipeline that allows for de novo OTU and biogeography discovery, and the recovery of precomputed OTUs from large-scale metabarcoding campaigns, we unveil more than 20 unknown Olpidiopsis taxa with a worldwide distribution. Additionally, laboratory-controlled cross-infection experiments show that a Scottish variety of O. porphyrae is virulent on the most commonly cultivated Pyropia yezoensis cultivar in Korea and that conversely, a Korean strain of O. porphyrae successfully infects wild Bangia sp. strains isolated from Scotland. These results provide proof-of-concept that a native Olpidiopsis pathogen may threaten an introduced crop or that an Olpidiopsis pathogen potentially introduced alongside a non-native crop might cross-infect a native European alga.
Thus, we draw parallels with several current biosecurity crises, where major risks to native floras and faunas, as well as crops, are caused by the inadvertent introduction of poorly known pathogens through the agricultural and horticultural trades. Therefore, we express concern that the rapid growth of algal cultivation worldwide, linked to international movement of seaweed seed and the absence of biosecurity monitoring or reulation pertaining to this trade, potentially lays the ground for grave ecological and economic crises in the marine environment.
Introduction
In only 10 years, the global production of cultivated macroalgae has more than doubled, increasing from 13.5 Mt to 29.4 Mt between 2005 and 2015 (Ferdouse et al., Citation2018). Since 2010, the production of red algae has overtaken in quantity the production of brown algae, which previously dominated the market. Production of laver (Pyropia spp., formerly known as Porphyra) has almost doubled, mostly due to a rapid expansion in China and Korea. Over the same period, the production of seaweeds grown for their gelling properties (carageenophytes and agarophytes, including eucheumatoids and Gracilaria) has increased more than 10-fold and now exceeds 14 Mt. While Asia dominates the sector, macroalgal cultivation is rapidly expanding across several continents, including Africa, South America and Europe. The number of algal species subjected to cultivation trials is increasing steadily, with over 220 species now recognized as having some commercial value (Ferdouse et al., Citation2018).
This rapid development of the macroalgal aquaculture sector is followed by a similarly increasing economic burden of disease outbreaks (Cottier-Cook et al., Citation2016). A pioneering epidemiological study conducted in Korea showed that alongside the intensification of production, disease management is a growing concern for Pyropia farmers, and now contributes up to half the running cost of a farm (Kim et al., Citation2014); in the last four years, several new species of fungal, viral and oomycete pathogens infecting Pyropia crops have been described (Kim et al., Citation2016; Klochkova et al., Citation2016; Mo et al., Citation2016).
This trend is well known in agriculture and animal aquaculture, whereby novel pathogens are discovered when newly domesticated species are first cultivated on a large scale and crop yields are affected by disease outbreaks. This has been the case, for example, following the rapid development of invertebrate aquaculture (e.g. shrimp) since the 1990s (Stentiford & Lightner, Citation2011; Stentiford et al., Citation2017). Additionally, the propagation of pathogens outside their native range via infected crops, or via the trade of (infected) agricultural products, has been identified as a major threat to native animal and plant biodiversity worldwide (Brasier, Citation2008; Stentiford et al., Citation2017). Therefore, the identification and characterization of pathogens infecting algal crops are becoming a research priority to underpin the sustainable development of the industry (Cottier-Cook et al., Citation2016). Conversely, the potential of algal crops to act as reservoirs of pathogens threatening neighbouring wild stocks also needs to be considered (e.g. presence of pathogen reservoirs and wild crop cross-contamination; Loureiro et al., Citation2015; Valero et al., Citation2017).
The oomycetes Olpidiopsis porphyrae and O. pyropiae are responsible for destructive disease outbreaks in Asia (Arasaki, Citation1947, Citation1960; Park et al., Citation2001; Ding & Ma, Citation2005; Sekimoto et al., Citation2008; Kim et al., Citation2014; Klochkova et al., Citation2016; Kwak et al., Citation2017). Based on a modest sampling campaign of wild and farmed Scottish algae, we recently described two novel Olpidiopsis species, and recorded another that was previously known only in Japan (Badis et al., Citation2018). A few more molecular records are available for this genus: O. bostrychiae infecting Bostrychia moritziana, a small red alga of West Indian Ocean mangroves (West et al., Citation2006; Sekimoto et al., Citation2009); O. feldmanni infecting Asparagopsis sp. in the Adriatic sea (Fletcher et al., Citation2015); and O. heterosiphoniae infecting Heterosiphonia japonica (Klochkova et al., Citation2017). These organisms belong to the basal oomycetes, together with the Anisolpidiales, Haliphthorales and several newly identified clades of diatom pathogens (Gachon et al., Citation2017; Garvetto et al., Citation2018, Citation2019; Hasset et al., Citation2019). The clade commonly recognized as the order Olpidiopsidales (sensu Beakes et al., Citation2014), contains three highly supported subclades of pathogens of red algae referred to as the ‘pyropiae’, the ‘bostrychiae’ and the ‘porphyrae’ lineages (Badis et al., Citation2018; Garvetto et al., Citation2018). The recent epitypification of the water mould O. saprolegniae, the type species of the genus Olpidiopsis, confirmed that these parasites of red algae, and two other pathogens of diatoms assigned to Olpidiopsis (Buaya et al., Citation2017, Citation2019a), do not belong to Olpidiopsis sensu stricto (Buaya et al., Citation2019b). In the same study, the authors also defined an epitype for the parasite of red algae Pontisma lagenioides, and tentatively reassigned all other ‘Olpidiopsis’ parasites of red algae to the genus Pontisma despite the lack of strong phylogenetic evidence (see Garvetto et al., Citation2019). Additional intracellular holocarpic oomycete pathogens of red algae have been described morphologically, and tentatively assigned to the genera Eurychasma, Eurychasmidium, Pontisma and Petersenia (Sparrow, Citation1960; Van der Meer & Pueschel, Citation1985; Dick, Citation2001). Some have a reported impact on cultivated red algae (e.g. Petersenia pollagaster on Chondrus crispus; Craigie & Shacklock, Citation1995), adding weight to our hypothesis that more oomycete taxa of relevance to red algal cultivation remain either to be described or better characterized.
Metabarcoding has the potential to accelerate species discovery (de Vargas et al., Citation2015), and an increasing number of continental-scale surveillance programmes, including the Ocean Sampling Day (Kopf et al., Citation2015) and the Australian Marine Dataset (AMD; Brown et al., Citation2018), now provide invaluable resources to study the biodiversity and biogeography of marine microbes.
In this study, we used metabarcoding and data-mining to assess the extent of the undescribed diversity amongst marine Olpidiopsis endoparasites, as well as their occurrence worldwide. We first investigated the presence of Olpidiopsis in the eukaryotic microbiome of the North Atlantic species Porphyra umbilicalis using an 18S rRNA metabarcoding dataset. Additionally, we screened publicly available data obtained during large-scale seawater barcoding campaigns (Tara Oceans, AMD); we also developed a dedicated pipeline, hereafter named MOULINETTE, that couples automatic data mining, de novo OTU calling and retrieval of GPS coordinates in metadata of the NCBI Sequence Read Archive (SRA). This novel approach allowed us to screen all publicly available marine shotgun and metabarcoding datasets, revealing substantial unknown diversity and shedding light on the biogeography of the marine Olpidiopsis pathogens at a global scale. Finally, we performed cross-inoculation experiments using O. porphyrae strains isolated in Korea and Scotland from cultivated and wild algae (respectively), in order to investigate the possibility of pathogen-mediated cross-talk between wild and cultivated red algae.
Materials and methods
Metabarcoding of Porphyra umbilicalis blades
As part of a metabarcoding effort run alongside the Porphyra umbilicalis genome project (Brawley et al., Citation2017), nine individual P. umbilicalis specimens were collected from January to February 2015 from Bantry Bay (Ireland, one blade), Oban (Scotland, one blade), Sidmouth (England, three blades), Porto (Portugal, one blade) and Isle Au Haut (Maine, USA, three blades). Briefly, samples were washed three times with sterile filtered seawater and flash frozen in liquid nitrogen. For each specimen, total DNA was extracted using the Qiagen DNeasy kit after grinding algal tissue. Eukaryotic 18S v4 RNA barcodes were amplified (Stoeck et al., Citation2010), sequenced and analysed at the US DOE Joint Genome Institute (JGI) using the iTagger pipeline v1.1 (Tremblay et al., Citation2015). The complete library preparation, QC and sequencing procedure can be found on the iTag protocol section of the JGI website (https://jgi.doe.gov/user-programs/pmo-overview/protocols-sample-preparation-information/). To avoid potential artefacts arising from multiplexed samples in sequencing machines, we only considered the occurrence of an Olpidiopsis OTU if a sample contained more than 0.1% of the total OTU read count. Selected OTUs were manually blasted to ascertain their phylogenetic position.
MOULINETTE: a custom pipeline for automated screen of the SRA database
In order to automatically retrieve sequence and GPS information from metabarcoding datasets, we designed MOULINETTE, a sequence search tool that screens raw reads of environmental sequences deposited in the SRA database (Leinonen et al., Citation2011). Its structure is detailed in Supplementary notes S1 together with the original custom bash script, and the full strategy is discussed in Supplementary notes S2. Briefly, based on a list of query sequences and a list of SRA Run Ids, the pipeline extracts raw sequencing reads matching the queries (if any) and corresponding GPS coordinates of each positive dataset. After screening all input SRA Runs, all paired reads identified are processed in the Usearch OTU clustering pipeline (Edgar, Citation2010). For each query sequence, the pipeline outputs a list of reads, GPS coordinates and OTUs (hereafter called SRA-OTUs) that can be manually inspected and plotted on a world map using QGIS (2.18.0 ‘Las Palmas’ http://www.qgis.org/). To extract the biogeography of each SRA-OTU, the GPS coordinates of the SRA runs containing reads with over 97% identity to any SRA-OTUs are used to plot the biogeographical maps. For the screen of the Tara Oceans and AMD metabarcoding campaigns, local blast databases were built using all precomputed OTUs, and candidate OTUs were selected on the basis of sequence homology using the blast algorithm. OTUs were further filtered on the basis of reciprocal best blast hit with known Olpidiopsis sequences in the nr database (excluding uncultured sequences), and subsequent phylogenetic anchoring with known Olpidiopsis sequences. A compilation of all Olpidiopsis records gathered here is provided (Supplementary table S1) as well as a fasta file containing the final list of Olpidiopsis OTUs (Supplementary notes S3).
Phylogenetic placement of representative OTUs
The OTUs selected as described above were aligned to all reference 18S sequences of known Olpidiopsis (Badis et al., Citation2018) in GENEIOUS v6 using the MAFFT algorithm. Alignments were manually corrected prior to processing in MEGA7 (Kumar et al., Citation2016). Maximum likelihood (ML) algorithm and settings used previously (Badis et al., Citation2018) were modified to account for the short (less than 400 bp) length of OTUs, using the partial deletion parameter to accept 30% of missing data for each position. Although we manually checked that this parameter did not alter the tree topology, this approach is solely used to anchor OTUs to their respective reference Olpidiopsis sequences, and readers are referred to Sekimoto et al. (Citation2009) and Garvetto et al. (Citation2018) for an up to date phylogenetic placement of marine Olpidiopsis within oomycetes.
Cross-inoculation and microscopy
Olpidiopsis porphyrae var. scotiae (Badis et al., Citation2018) and O. porphyrae var. koreanae (Kwak et al., Citation2017) were maintained on their respective Scottish and Korean algal hosts. All cultures were maintained at 10°C with a 12h:12 h photoperiod under 10 μmol photons m–2 s–1 fluorescent lighting and using sterilized half-strength Provasoli-enriched seawater as culture medium. The Scottish Bangia sp. was inoculated with O. porphyrae var. koreanae in Scotland, and the Korean Pyropia cultivar was inoculated with O. porphyrae var. scotiae in Korea. Cross-inoculation experiments were performed by trained personnel in Class 2 facilities using appropriate measures for handling and disposal of biological material. Inoculation procedures were adapted from Strittmatter et al. (Citation2013). Briefly, 40-μm mesh cell strainers containing the inoculum were added to healthy algal cultures in 50 mm diameter, 20 mm-deep Petri dishes. Observations of infected Scottish Porphyra sp. and Bangia sp. were performed using a Zeiss Axioskop 2, coupled to an Axiocam. Where applicable, Calcofluor White staining of parasite cell walls was performed as previously described (Gachon et al., Citation2017). Observations of the infected Korean Pyropia cultivar were performed according to Klochkova et al. (Citation2017).
Results
Firstly, we assessed if Olpidiopsis barcodes could be detected in microbiomes associated with macroscopically healthy P. umbilicalis collected on both sides of the North Atlantic Ocean. Secondly, based on the hypothesis that seawater may contain free-living oomycete stages (e.g. zoospores), we set out to screen all seawater marine metabarcoding and metagenomic datasets available on the Sequence Read Archive using our custom MOULINETTE pipeline. Finally, we expanded this screen to additional large-scale marine metabarcoding campaigns (i.e. Tara Oceans and AMD) using conventional blastn retrieval of OTUs. A summary of all marine datasets generated or investigated in this study is presented in Supplementary table S2.
The Porphyra umbilicalis microbiome frequently contains Olpidiopsis spp
The diversity of potential interactions between P. umbilicalis and its eukaryotic microbiome was explored: most OTUs retrieved (named Pumb-OTUn, see list in Supplementary table S3) reflected associations with epiphytic diatoms and other red or brown algae. Six oomycete Pumb-OTUs were detected, with five of them being attributed to the Olpidiopsidales by the iTagger pipeline. Phylogenetic anchoring () revealed four genuine Olpidiopsis (Pumb-OTUs 5, 150, 187, 225) and one sequence (Pumb-OTU 81) clustering with the pathogens of brown algae Anisolpidium ectocarpii and A. rosenvingei (Gachon et al., Citation2017). Pumb-OTU 5 was nearly identical to O. palmariae (99.7% Identity on 388 residues). It was the fifth most abundant OTU in this dataset and was detected in four out of the eight P. umbilicalis individuals sampled. Pumb-OTUs 150, 187 and 225 were each detected once in different P. umbilicalis blades, at low abundance. The short v4 (under 400 nt) barcodes did not allow separation of those three OTUs from O. feldmanni, O. bostrychiae and O. muelleri, with which they formed a single clade. Interestingly, up to three different OTUs were observed on the same individual blade (Pumb-OTUs 5, 150, and 225) collected in Bantry Bay. Overall, these four Pumb-OTUs (red circles on ) illustrate the widespread occurrence of Olpidiopsis associated with wild populations of P. umbilicalis, both on the western and eastern North Atlantic shores.
Fig. 1. Hidden diversity and host range in the marine genus Olpidiopsis. Phylogenetic anchoring of all V4 18S rDNA Operational Taxonomic units (OTUs) identified in this study with reference Olpidiopsis sequences, as well as four additional environmental 18S sequences (KT815133, KT810401, AY426928, AY789783) identified by similarity search in GenBank. Grey brackets define the three Olpidiopsis lineages named after their first described species. Olpidiopsis species initially isolated on Bangiophycean hosts are indicated in red. Purple, green and blue OTUs correspond to sequences obtained from P. umbilicalis metabarcoding, the Australian Marine Dataset (AMD) and our dedicated SRA screening pipeline, respectively. The right panel summarizes the host range of Olpidiopsis spp. based on previous laboratory experiments, where hosts can be infected (+), not infected (−), not tested (NT), or probably infected based on metabarcoding evidence (*). Dashed circles highlight naturally occurring hosts.
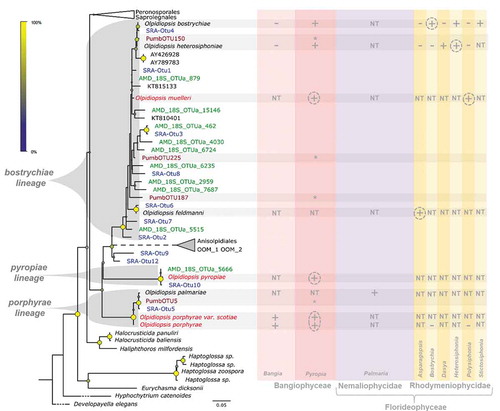
Fig. 2. Overview of biogeographic evidence in the marine genus Olpidiopsis. Worldwide evidence of the occurrence of marine Olpidiopsis parasites, including the GPS coordinates of all molecular data () collected in this study, additional morphological reports of marine Olpidiopsis and a selection of red algal pathogens of doubtful taxonomic position (inland points represent missing locations), summarized in Supplementary table S1. Stars: Genuine Olpidiopsis isolates; Squares: GenBank environmental sequences; Triangles: morphological reports; Dots: Operational Taxonomic Units gathered from the Sequence Read Archive (yellow), Tara Oceans (purple), the Australian Marine Dataset (green) and P. umbilicalis microbiome (red). Individual maps of each SRA-OTUs are provided in Supplementary figs S2–S13.
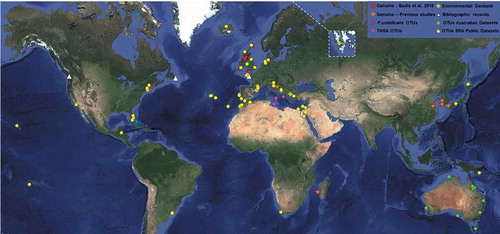
Marine metabarcoding unveils unknown diversity and worldwide distribution of marine Olpidiopsis
Using the MOULINETTE pipeline with a query dataset of all Olpidiopsis 18S sequences and Pumb-OTUs, we screened all marine 18S metabarcoding and metagenome datasets available on the SRA (1127 SRA runs in total as per April 2017, see Supplementary table S4). To harvest all possible Olpidiopsis-related reads, we used MOULINETTE with relaxed stringency parameters (97% blast identity, e-value under 1e-90). To obtain genuine Olpidiopsis barcodes, we extracted all reads matching Olpidiopsis sequences in GenBank nr (i.e. best reciprocal blast hit), resulting in 1426 putative Olpidiopsis reads (165 single reads; 1261 read pairs). 1080 filtered and merged read pairs were clustered in 12 SRA-OTUs (11 v4 OTUs displayed in and 1 v9 OTU displayed in Supplementary fig. S1). Finally, 1283 reads were successfully mapped back to their best matching SRA-OTU using a 97% identity threshold (Supplementary table S5). This final list of Olpidiopsis reads was used to count reads matching each SRA-OTU (Supplementary table S6), and extrapolate their biogeography based on the GPS coordinates of the 131 source SRA Runs (Supplementary table S7). SRA-OTU10 was nearly identical to O. pyropiae (99.7%), and SRA-OTU5 was almost identical to O. palmariae (99.5% identical). With the exception of the potentially spurious SRA-OTUs 9 and 12 (discussed in Supplementary notes S2(j)), all other SRA-OTUs clustered well with the three Olpidiopsis lineages, including the most represented sequences (SRA-OTUs 1, 2, 3, 4). Most identified SRA runs contained between 1 and 31 putative Olpidiopsis reads, but runs ERR867714, ERR867766 and ERR867821 contained 144, 156 and 320 such reads, respectively. Strikingly, up to five Olpidiopsis OTUs were simultaneously detected in run ERR867821.
Finally, we also screened the AMD v4 OTUs (www.bioplatforms.com/marine-microbes/) and Tara Oceans V9 OTUs (https://doi.pangaea.de/10.1594/PANGAEA.843022) using conventional blastn. Based on their best reciprocal blast hit, and anchoring with known Olpidiopsis sequences in our 18S phylogenetic tree, 10 AMD-OTUs and three Tara-OTUs were retrieved (, named AMD_18S_OTUa_n; Tara-OTUs represented on Supplementary fig. S1). AMD_18S_OTUa_5666 was nearly identical (99.5%) to O. pyropiae while the other nine were anchored in the ‘bostrychiae’ lineage. Although shorter than the v4 region, alignment and phylogenetic inference over the 100pb of the 18S v9 region grouped Tara-OTU 236817 with O. pyropiae (95% identity), and Tara-OTUs 632184 and 239197 with O. bostrychiae (98 and 94%, respectively; Supplementary fig. S1). The three v9 OTUs were detected in two distinct TARA stations (Supplementary table S1), with TARA stations TARA_S400007202 and TARA_A100000032 containing two OTUs.
Overall, our comprehensive screen of metabarcoding datasets highlights the existence of at least 20 unknown taxonomic entities falling within the marine Olpidiopsis clades.
Marine Olpidiopsis taxa occur worldwide
The GPS coordinates associated with all Olpidiopsis sequences identified in this study were plotted on maps, together with those associated with published Olpidiopsis reports (see overview in and individual maps of each SRA-OTUs in Supplementary figs S2–S13). This revealed a truly cosmopolitan occurrence of the Olpidiopsis porphyrae, pyropiae and bostrychiae lineages, including the shores of all continents sampled (Asia, Africa, America, Europe and Australia), all oceans, and archipelagos such as Hawaii, Madeira and French Polynesia. Most importantly, our screen demonstrates the worldwide distribution of destructive pathogens previously thought to be restricted to Asia: sequences nearly identical and most similar to the Korean pathogen O. pyropiae correspond to SRA-OTU10 and were detected in the UK (ERR867914, ERR855788, ERR867821), Madeira (ERR867942), the USA (ERR867745), Egypt (ERR867716) and Israel (ERR867890). O. pyropiae was also detected in Australia with AMD_18S_Otua_5666. Note that although we recently reported the presence of O. porphyrae in Scotland (Badis et al., Citation2018), no OTU related to this species was detected in metabarcoding datasets, as the presence of introns in the 18S probably interferes with barcoding. However, the closely related O. palmariae (bearing no introns) was successfully detected in the UK via SRA-OTU5 (99.5% identical to O. palmariae) detected in Essex (ERR867821, England), and Pumb-OTU5 (99.7% identical to O. palmariae) detected in Bantry Bay (Ireland) and Sidmouth (England).
Cross-infection experiments illustrate the potential transfer of non-native diseases between wild and farmed algal stocks
On the basis that O. porphyrae was initially described in Japan and recently reported in Korea, we assessed whether a Scottish isolate of this species could infect Korean commercial cultivars of Pyropia yezoensis (, ). Incubating infected Scottish Porphyra material with healthy P. yezoensis blades led to similar symptoms within 24–48 h (, arrow). While infections observed on wild Scottish Porphyra were mostly located on blade margins, most cells in the Korean cultivar were infected after 4–5 days (); blades could ultimately not regenerate and fell apart into debris. Conversely, the cross-inoculation of O. porphyrae var. koreanae on a clonal isolate of Bangia sp. collected in Scotland also led to successful infection (–).
Figs 3–8. Non-native Olpidiopsis parasites could infect wild and farmed red algae. Cross-inoculations of Korean and Scottish algae with non-native Olpidiopsis parasites. Figs 3–4. O. porphyrae var. scotiae infecting the major Korean commercial cultivar of Pyropia yezoensis. Fig. 3. Early developing parasite thallus (arrow) 48 hours after infection. Fig. 4. Densely infected blade of the same Pyropia cultivar 5 days after infection, where only 5 host cells are left alive (white arrowheads). Figs 5–8. Young (5–6) and mature (7–8) thalli of the Korean parasite Olpidiopsis porphyrae var. koreanae (arrow) infecting Bangia sp. from Scotland. As revealed by Calcofluor White staining, the early stage of thallus development is unwalled (6) while a clear cell wall is detected in mature thallus (7). Scale bars: 20 μm.
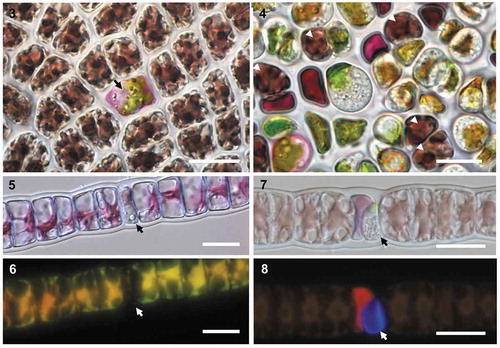
Discussion
Hidden diversity in marine Olpidiopsis lineages
Here, metabarcoding highlights that: (i) OTUs closely related to known Olpidiopsis pathogens of red algae are commonly found in the eukaryotic microbiome of Porphyra umbilicalis; and (ii) at least 20 OTUs corresponding to unknown taxa remain to be characterized amongst the ‘pyropiae’, ‘porphyrae’ and ‘bostrychiae’ lineages. The most parsimonious interpretation of these data, both in terms of conservation of host range and pathogenic lifestyle, is therefore that all novel OTUs identified within the three marine Olpidiopsis lineages probably represent taxa of holocarpic, intracellular parasites of red algae. Interestingly, the majority of OTUs clustered within the ‘bostrychiae’ lineage, the only one to include parasites of the Rhodymeniophycidae (). It is thus worth noting that several known taxa of pathogens of red algae awaiting molecular characterization (e.g. Eurychasmidium, Petersenia or Eurychasma) have been reported with a similar host range (Supplementary table S1). Altogether, our data demonstrate the existence of a rich diversity of mostly undescribed taxa amongst the marine Olpidiopsis lineages, and highlight the necessity to overhaul the taxonomy of Olpidiopsis and other genera of marine oomycetes.
Some marine Olpidiopsis species have a cosmopolitan biogeography and broad host spectrum
The host range of most marine Olpidiopsis pathogens is poorly known. Thus far however, all available data point to Olpidiopsis not only having the capacity to infect commercially valuable algae such as Palmaria palmata, Pyropia yezoensis or Porphyra sp., but also surrounding wild stocks, especially Ceramiales, in the Western Indian Ocean (West et al., Citation2006), Yellow Sea (Klochkova et al., Citation2016) and Scotland (i.e. O. muelleri var. polysiphoniae). The confirmed presence in Scotland of O. porphyrae var. scotiae shows that this economically devastating species has a far broader biogeographic range than previously thought (Kwak et al., Citation2017). Similarly, although we acknowledge the limitations of barcoding for species delimitation and biogeography, the broad geographic range that we retrieved for many OTUs (Supplementary figs S2–S13) suggests that some other marine Olpidiopsis taxa may have the ability to persist and propagate in contrasting environmental conditions, including if they were introduced outside their native range. This hazard is further suggested by the ability of O. porphyrae var. scotiae to infect a major Korean cultivar of P. yezoensis in laboratory conditions.
Marine Olpidiopsis species represent a potential hazard to red algal cultivation and conservation worldwide.
The destructiveness of O. pyropiae and O. porphyrae in Asia demonstrates that these oomycete pathogens represent a hazard for the red seaweed industry (Ding & Ma, Citation2005; Kim et al., Citation2014; Kwak et al., Citation2017). Accordingly, disease management has become an integral part of farm design and operation in Asia; for example, insurance schemes have recently been set up to protect farmers against worsening crop losses (Cottier-Cook et al., Citation2016). The Korean Pyropia cultivar tested in this study is deployed each year in the Jindo and Wando area, accounting for over 70% of the Korean Pyropia production. The susceptibility of this cultivar to a Scottish Olpidiopsis therefore exemplifies the potential impact of introducing non-native pathogens, especially in the context of uncontrolled germplasm movement for aquaculture purposes. Likewise, our repeated observations of O. palmariae in cultivation facilities in Scotland (Badis et al., Citation2018) highlight the distinct possibility of yield-limiting epidemic outbreaks in the nascent Western aquaculture industry, and echo repeated reports of Petersenia diseases in Canadian Chondrus production facilities (Craigie & Correa, Citation1996 and references therein).
Time and again in the history of land agriculture and animal aquaculture, the spread of pathogens, often previously unknown, through seed movement and international trade has damaged wild stocks. Despite the existence of a well-established international biosecurity framework and the relative ease of deploying eradication measures on land compared to the sea, inadvertent introductions of plant pathogens happens at a large scale, with 234 species discovered in the UK between 1970 and 2004 (Jones & Baker, Citation2007). For example, the ubiquitous prevalence of highly diverse Phytophthora species in European nurseries is fuelling a large-scale crisis that puts many natural and horticultural ecosystems at risk (Jung et al., Citation2016). Compounding factors are the paucity of knowledge on their native host range and difficulty of detection, combined with their capacity to infect a wide range of hosts in their non-indigenous range. Similarly worrying concerns have been arising in all food-producing sectors, for a similar set of reasons: in the shrimp industry, four pandemics caused by previously unknown viral pathogens have devastated the industry and now threaten over 100 wild crustacean species (Small & Pagenkopp, Citation2011; Stentiford et al., Citation2017); and in fish farms, over 30 marine diseases are deemed of economic significance (Lafferty et al., Citation2015), and potential disease exchanges between farmed and wild fish are being increasingly documented, with a recent first report of escaped farmed salmon infected with two of the most prevalent viruses in Norwegian aquaculture (Madhun et al., Citation2015). Across the board, there is rising awareness that farm-borne pathogens threaten wild species, sometimes down to extinction, and calls are unanimous to adapt biosecurity measures to the intensifying, increasingly globalized, agricultural trade.
Our experimental data lead us to conclude that similarly, the extensive unknown diversity of marine Olpidiopsis pathogens, their poorly known – yet potentially broad – host range, and their widespread distribution represent a potentially grave threat not only to red algal cultivation, but also to marine ecosystems worldwide. This potential threat might be compounded both by unregulated seed movement in many seaweed-producing countries, sometimes without any quarantine (Hayashi et al., Citation2010), and the general difficulty of implementing any containment measure in the open sea. Already, the accidental introduction of economically detrimental seaweed pathogens has been suggested in Indonesia (Vairappan, Citation2006) and Korea (Park et al., Citation2003). Thus, it is urgent to underpin the rapid expansion and globalization of the algal cultivation industry with a biosecurity framework paralleling those in place for animals and plants in order to limit economic losses and potential impacts on marine ecosystems.
Supplementary information
The following supplementary material is accessible via the Supplementary Content tab on the article’s online page at https://doi.org/10.1080/09670262.2019.1664769
Supplementary notes S1. MOULINETTE Pipeline – usage and script
Supplementary notes S2. MOULINETTE Pipeline – commentary
Supplementary notes S3. Fasta sequences of all Olpidiopsis OTUs gathered in this study.
Supplementary table S1. Detailed information for Olpidiopsis sequences and bibliographic records.
Supplementary table S2. Summary table of all metabarcoding datasets/campaigns processed in this study.
Supplementary table S3. OTUs calling and abundance in all sampled P. umbilicalis blade (Pumb iTag V4 Data).
Supplementary table S4. MOULINETTE – Run Information for each of the 1127 SRA runs processed.
Supplementary table S5. MOULINETTE - Per-read blast scores for best matching SRA-OTUs and reciprocal best hit in the nr Database.
Supplementary table S6. MOULINETTE - SRA-OTUs retrieved by the pipeline, with corresponding reads and SRA run numbers.
Supplementary table S7. MOULINETTE - Biogeography data for each SRA-OTUs.
Supplementary fig. S1. 18S Phylogenetic tree featuring v9 barcodes gathered in this study.
Supplementary figs S2–S13. Biogeography of individual OTUs detected by the MOULINETTE pipeline.
Author contributions
C.M.M. Gachon, G.H. Kim, M. Ostrowski, Y. Badis and T.A. Klochkova designed the experiments; C.M.M. Gachon and G.H. Kim supervised the research; Y. Badis, C.M.M. Gachon and J. Brakel conducted the fieldwork; Y. Badis, T.A. Klochkova, J. Brakel and P. Arce conducted the laboratory work; S.G. Tringe designed and conducted the metabarcoding of P. umbilicalis; Y. Badis designed code, conducted data analysis and drafted the initial manuscript; Y. Badis and C.M.M. Gachon finalized the manuscript with contributions from all co-authors. All authors gave final approval for publication.
TEJP-2019-0015-File009.pdf
Download PDF (1.7 MB)TEJP-2019-0015-File008.xlsx
Download MS Excel (630.7 KB)TEJP-2019-0015-File007.fasta
Download (9.6 KB)TEJP-2019-0015-File006.docx
Download MS Word (727.6 KB)TEJP-2019-0015-File005.docx
Download MS Word (337.4 KB)Acknowledgements
We are grateful to Juliet Brodie (National History Museum, London, UK), Helena Abreu (ALGAPlus, Ílhavo, Portugal) and Susan Brawley (University of Maine, Orono, USA) for collection of wild P. umbilicalis blades. We would like to acknowledge the contribution of the Marine Microbes consortium in the generation of data used in this publication.
Disclosure statement
No potential conflict of interest was reported by the authors.
Additional information
Funding
References
- Arasaki, S. (1947). Studies on the rot of Porphyra tenera by a Pythium. Bulletin of the Japanese Society of Scientific Fisheries, 13: 74–90.
- Arasaki, S. (1960). A chytridean parasite on the Porphyra. Bulletin of the Japanese Society of Scientific Fisheries, 26: 543–548.
- Badis, Y., Klochkova, T.A., Strittmatter, M., Garvetto, A., Murúa, P., Sanderson, J.C., Kim, G.H & Gachon, C.M.M. (2018). Novel species of the oomycete Olpidiopsis potentially threaten European red algal cultivation. Journal of Applied Phycology, 31: 1239–1250.
- Beakes, G.W., Honda, D. & Thines, M. (2014). Systematics of the Straminipila: Labyrinthulomycota, Hyphochytriomycota, and Oomycota. In The Mycota: Systematics and Evolution (7A) (McLaughlin, D.J. & Spatafora, J.W., editors), 39–97. Springer, Heidelberg.
- Brasier, C.M. (2008). The biosecurity threat to the UK and global environment from international trade in plants. Plant Pathology, 57: 792–808.
- Brawley, S.H., Blouin, N.A., Ficko-Blean, E., Wheeler, G., Lohr, M., Goodson, H.V., Jenkins, J., Blaby-Haas, C.E., Helliwell, K., Chan, C.X., Marriage, T., Bhattacharya, D., Klein, A., Badis, Y., Brodie, J., Cao, Y., Collen, J., Dittami, S., Gachon, C., Green, B., Karpowicz, S., Kim, J., Lin, S., Michel, G., Mittag, M., Olson, B., Pangilinan, J., Peng, Y., Qiu, H., Riek, S.Z., Shu, S.Q., Singer, J., Smith, A., Sprecher, B., Wagner, V., Wang, W., Wang, Z., Yan, Z., Yarish, C., Zhuang, Y.Y., Zou, Y., Lindquist, E., Grimwood, J., Barry, K., Rokshar, D., Schumtz, J., Gantt, E., Stiller, J., Grossman, A. & Prochnik, S. (2017). Insights into the red algae and eukaryotic evolution from the genome of Porphyra umbilicalis (Bangiophyceae, Rhodophyta). Proceedings of the National Academy of Sciences USA, 114: E6361–E6370.
- Brown, M.V., van de Kamp, J., Ostrowski, M., Seymour, J.R., Ingleton, T., Messer, L.F., Jeffries, T., Siboni, N., Laverock, B., Bibiloni-Isaksson, J., Nelson, T.M., Coman, F., Davies, C.H., Frampton, D., Rayner, M., Goosen, K., Robert, S., Holmes, B., Abell, G.C.J., Craw, P., Kahlke, T., Sow, S.L.S., McAllister, K., Windsor, J., Skuza, M., Crossing, R., Patten, N., Malthouse, P., Van Ruth, P.D., Paulsen, I., Fuhrman, J.A., Richardson, A., Koval, J., Bissett, A., Fitzgerald, A., Moltmann, T. & Bodrossy, L. (2018). Systematic, continental scale temporal monitoring of marine pelagic microbiota by the Australian Marine Microbial Biodiversity Initiative. Nature Scientific Data, 5: 180130.
- Buaya, A.T., Ploch, S., Hanic, L., Nam, B., Nigrelli, L., Kraberg, A. & Thines, M. (2017). Phylogeny of Miracula helgolandica gen. et sp. nov. and Olpidiopsis drebesii sp. nov., two basal oomycete parasitoids of marine diatoms, with notes on the taxonomy of Ectrogella-like species. Mycological Progress, 16: 1041–1050.
- Buaya, A.T., Ploch, S. & Thines, M. (2019a). Rediscovery and phylogenetic placement of Olpidiopsis gillii (de Wildeman) Friedmann, a holocarpic oomycete parasitoid of freshwater diatoms. Mycoscience, 60: 141–146.
- Buaya, A.T., Ploch, S., Inaba, S. & Thines, M. (2019b). Holocarpic oomycete parasitoid of red algae are not Olpidiopsis. Fungal Systematics and Evolution, 4: 21–31.
- Cottier-Cook, E.J., Nagabhatla, N., Badis, Y., Campbell, M., Chopin, T., Dai, W., Fang, J., He, P., Hewitt, C., Kim, G.H., Huo, Y., Jiang, Z., Kema, G., Li, X., Liu, F., Liu, H., Liu, Y., Lu, Q., Luo, Q., Mao, Y., Msuya, F.E., Rebours, C., Shen, H., Stentiford, G.D., Yarish, C., Wu, H., Yang, X., Zhang, J., Zhou, Y. & Gachon, C.M.M. (2016). Safeguarding the future of the global seaweed aquaculture industry. United Nations University (INWEH) and Scottish Association for Marine Science Policy Brief. ISBN 978-92-808-6080-1.
- Craigie, J.S. & Shacklock, P.F. (1995). Culture of Irish moss. In Cold-water Aquaculture in Atlantic Canada (Boghen, A.D., editor), 243–270. University of Moncton, Moncton.
- Craigie, J.S. & Correa, J.A. (1996). Etiology of infectious diseases in cultivated Chondrus crispus (Gigartinales, Rhodophyta). Hydrobiologia, 326: 97–104.
- de Vargas, C., Audic, S., Henry, N., Decelle, J., Mahé, F., Logares, R., Lara, E., Berney, C., Le Bescot, N., Probert, I., Carmichael, M., Poulain, J., Romac, S., Colin, S., Aury, J.-M., Bittner, L., Chaffron, S., Dunthorn, M., Engelen, S., Flegontova, O., Guidi, L., Horák, A., Jaillon, O., Lima-Mendez, G., Lukeš, J., Malviya, S., Morard, R., Mulot, M., Scalco, E., Siano, R., Vincent, F., Zingone, A., Dimier, C., Picheral, M., Searson, S., Kandels-Lewis, S., Acinas, S.G., Bork, P., Bowler, C., Gorsky, G., Grimsley, N., Hingamp, P., Iudicone, D., Not, F., Ogata, H., Pesant, S., Raes, J., Sieracki, M.E., Speich, S., Stemmann, L., Sunagawa, S., Weissenbach, J., Wincker, P. & Karsenti, E. (2015). Eukaryotic plankton diversity in the sunlit ocean. Science, 348: 1261605.
- Dick, M.W. (2001). Straminipilous fungi: systematics of the peronosporomycetes, including accounts of the marine straminipilous protists, the plasmodiophorids, and similar organisms. Kluwer Academic Publishers, Dordrecht.
- Ding, H. & Ma, J. (2005). Simultaneous infection by red rot and chytrid diseases in Porphyra yezoensis Ueda. Journal of Applied Phycology, 17: 51–56.
- Edgar, R.C. (2010). Search and clustering orders of magnitude faster than BLAST. Bioinformatics, 26: 2460–1.
- Ferdouse, F., Yang, Z., Holdt, S.L., Murúa, P. & Smith, R. (2018). FAO 2018. The global status of seaweed production, trade and utilization. FAO Globefish Research Program, 124: 1–100.
- Fletcher, K., Uljevic, A., Tsirigoti, A., Antolic, B., Katsaros, C., Nikolic, V., van West, P. & Kuepper, F.C. (2015). New record and phylogenetic affinities of the oomycete Olpidiopsis feldmanni infecting Asparagopsis sp. (Rhodophyta). Diseases of Aquatic Organisms, 117: 45–57.
- Gachon, C.M.M., Strittmatter, M., Badis, Y., Fletcher, K.I., Van West, P. & Müller, D.G. (2017). Pathogens of brown algae: culture studies of Anisolpidium ectocarpii and A. rosenvingei reveal that the Anisolpidiales are uniflagellated oomycetes. European Journal of Phycology, 52: 133–148.
- Garvetto, A., Nézan, E., Badis, Y., Bilien, G., Arce, P., Bresnan, E., Gachon, C.M.M. & Siano, R. (2018). Novel widespread marine oomycetes parasitising diatoms, including the toxic genus pseudo-nitzschia: genetic, morphological, and ecological characterisation. Frontiers in Microbiology, 9: 2918.
- Garvetto, A., Perrineau, M.M., Dressler-Allame, M., Bresnan, E. & Gachon, C.M.M. (2019). “Ectrogella” parasitoids of the diatom Licmophora sp. are polyphyletic. Journal of Eukaryotic Microbiology, doi:10.1111/jeu.12750.
- Hasset, B.T., Thines, M., Buaya, A., Ploch, S. & Gradinger, R. (2019). A glimpse into the biogeography, seasonality, and ecological functions of arctic marine Oomycota. IMA Fungus, 10: 6.
- Hayashi, L., Hurtado, A.Q., Msuya, F.E., Bleicher-Lhonneur, G. & Critchley, A.T. (2010). A review of Kappaphycus farming: prospects and constraints. In Seaweeds and their Role in Globally Changing Environments (Seckbach, J., Einav, R. & Israel, A., editors). Springer Netherlands, Dordrecht.
- Jones, D.R. & Baker, R.H.A. (2007). Introductions of non-native plant pathogens into Great Britain, 1970–2004. Plant Pathology, 56: 891–910.
- Jung, T., Orlikowski, L., Henricot, B., Abad-Campos, P., Aday, A.G., Aguín Casal, O. & Perez-Sierra, A. (2016). Widespread Phytophthora infestations in European nurseries put forest, semi-natural and horticultural ecosystems at high risk of Phytophthora diseases. Forest Pathology, 46: 134–163.
- Kim, G.H., Moon, K.-H., Kim, J.-Y., Shim, J. & Klochkova, T.A. (2014). A revaluation of algal diseases in Korean Pyropia (Porphyra) sea farms and their economic impact. ALGAE, 29: 249–265.
- Kim, G.H., Klochkova, T.A., Lee, D.J. & Im, S.H. (2016). Chloroplast virus causes green-spot disease in cultivated Pyropia of Korea. Algal Research, 17: 293–299.
- Klochkova, T.A., Shin, Y.J., Moon, K.-H., Motomura, T. & Kim, G.H. (2016). New species of unicellular obligate parasite, Olpidiopsis pyropiae sp. nov., that plagues Pyropia sea farms in Korea. Journal of Applied Phycology, 28: 73–83.
- Klochkova, T.A., Kwak, M.S. & Kim, G.H. (2017). A new endoparasite Olpidiopsis heterosiphoniae sp. nov. that infects red algae in Korea. Algal Research, 28: 264–269.
- Kopf, A. et al. (2015). The ocean sampling day consortium. GigaScience, 4: 27.
- Kumar, S., Stecher, G. & Tamura, K. (2016). MEGA7: Molecular Evolutionary Genetics Analysis Version 7.0 for bigger datasets. Molecular Biology and Evolution, 33: 1870–4.
- Kwak, M.S., Klochkova, T.A., Jeong, S. & Kim, G.H. (2017). Olpidiopsis porphyrae var. koreanae, an endemic endoparasite infecting cultivated Pyropia yezoensis in Korea. Journal of Applied Phycology, 29: 2003–2012.
- Lafferty, K.D., Harvell, C.D., Conrad, J.M., Friedman, C.S., Kent, M.L., Kuris, A.M., Powell, E.N., Rondeau, D. & Saksida, S.M (2015). Infectious diseases affect marine fisheries and aquaculture economics. Annual Review of Marine Science, 7: 471–496.
- Leinonen, R., Sugawara, H. & Shumway, M. (2011). The sequence read archive. Nucleic Acids Research, 39: D19–21.
- Loureiro, R., Gachon, C.M. & Rebours, C. (2015). Seaweed cultivation: potential and challenges of crop domestication at an unprecedented pace. The New Phytologist, 206: 489–92.
- Madhun, A.S., Karlsbakk, E., Isachsen, C.H., Omdal, L.M., Eide Sørvik, A.G., Skaala, Ø., Barlaup, B.T. & Glover, K.A. (2015). Potential disease interaction reinforced: double-virus-infected escaped farmed Atlantic salmon, Salmo salar L., recaptured in a nearby river. Journal of Fish Diseases, 38: 209–219.
- Mo, Z., Li, S., Kong, F., Tang, X. & Mao, Y. (2016). Characterization of a novel fungal disease that infects the gametophyte of Pyropia yezoensis (Bangiales, Rhodophyta). Journal of Applied Phycology, 28: 395–404.
- Park, C.S., Kakinuma, M. & Amano, H. (2001). Detection and quantitative analysis of zoospores of Pythium porphyrae, causative organism of red rot disease in Porphyra, by competitive PCR. Journal of Applied Phycology, 13: 433–441.
- Park, C.S., Kakinuma, M., Sakaguchi, K. & Amano, H. (2003). Genetic variation detected with random amplified polymorphic DNA markers among isolates of the red rot disease fungus Pythium porphyrae isolated from Porphyra yezoensis from Korea and Japan. Fisheries Science, 69: 361–368.
- Sekimoto, S., Yokoo, K., Kawamura, Y. & Honda, D. (2008). Taxonomy, molecular phylogeny, and ultrastructural morphology of Olpidiopsis porphyrae sp. nov. (Oomycetes, Stramenipiles), a unicellular obligate endoparasite of Bangia and Porphyra spp. (Bangiales, Rhodophyta). Mycological Research, 112: 361–374.
- Sekimoto, S., Klochkova, T.A., West, J.A., Beakes, G.W. & Honda, D. (2009). Olpidiopsis bostrychiae sp. nov.: an endoparasitic oomycete that infects Bostrychia and other red algae (Rhodophyta). Phycologia, 48: 460–472.
- Small, H.J. & Pagenkopp, K.M. (2011). Reservoirs and alternate hosts for pathogens of commercially important crustaceans: a review. Journal of Invertebrate Pathology, 106: 153–64.
- Sparrow, F.K. (1960). Aquatic Phycomycetes. University of Michigan Press, Ann Arbor.
- Stentiford, G.D. & Lightner, D.V. (2011). Cases of White Spot Disease (WSD) in European shrimp farms. Aquaculture, 319: 302–306.
- Stentiford, G.D., Sritunyalucksana, K., Flegel, T.W., Williams, B.A.P., Withyachumnarnkul, B., Itsathitphaisarn, O. & Bass, D. (2017). New paradigms to help solve the global aquaculture disease crisis. PLoS Pathogens, 13: e1006160.
- Stoeck, T., Bass, D., Nebel, M., Christen, R., Jones, M.D.M., Breiner. H.-W. & Richards, T.A. (2010). Multiple marker parallel tag environmental DNA sequencing reveals a highly complex eukaryotic community in marine anoxic water. Molecular Ecology, 19 (Suppl. 1): 21–31.
- Strittmatter, M., Gachon, C.M.M., Muller, D.G., Kleinteich, J., Heesch, S., Tsirigoti, A., Katsaros, C., Kostopoulou, M. & Kupper, F.C. (2013). Intracellular eukaryotic pathogens in brown macroalgae in the Eastern Mediterranean, including LSU rRNA data for the oomycete Eurychasma dicksonii. Diseases of Aquatic Organisms, 104: 1–11.
- Tremblay, J., Singh, K., Fern, A., Kirton, E., He, S., Woyke, T., Lee, J., Chen, F., Dangl, J.L. & Tringe, S.G. (2015). Primer and platform effects on 16S rRNA tag sequencing. Frontiers in Microbiology, 6: 711.
- Vairappan, C.S. (2006). Seasonal occurrences of epiphytic algae on the commercially cultivated red alga Kappaphycus alvarezii (Solieriaceae, Gigartinales, Rhodophyta). Journal of Applied Phycology, 18: 611–617.
- Valero, M., Guillemin, M.-L., Destombe, C., Jacquemin, B., Gachon, C.M.M., Badis, Y., Buschmann, A.H., Camus, C. & Faugeron, S. (2017). Perspectives on domestication research for sustainable seaweed aquaculture. Perspectives in Phycology, 4: 33–46.
- Van der Meer, J.P. & Pueschel, C.M. (1985). Petersenia palmariae n. sp. (Oomycetes): a pathogenic parasite of the red alga Palmaria mollis (Rhodophyceae). Canadian Journal of Botany, 63: 404–408.
- West, J.A., Klochkova, T.A., Kim, G.H. & Loiseaux-de Goër, S. (2006). Olpidiopsis sp., an oomycete from Madagascar that infects Bostrychia and other red algae: host species susceptibility. Phycological Research, 54: 72–85.