ABSTRACT
Green tides, dominated by Ulva, are on the rise worldwide, and invasive Ulva spp. tend to bloom in new habitats. Over the last decade, green tides have caused serious problems in all marine regions of China, especially the Yellow Sea, but there has been no study emphasizing alien species or new records of Ulva in China. In this study, based on a molecular investigation spanning over 10 years, we found six new records of Ulva in China and analysed their distribution patterns. Three northern temperate species – Ulva simplex, U. splitiana and U. partita – were also distributed in temperate marine areas in China. Meanwhile, three subtropical species – U. meridionalis, U. tepida and U. chaugulii – were found to be dominant in the green tides in the South China Sea, and have also successfully exploited temperate areas, especially U. meridionalis which has a wide distribution in China. U. meridionalis has an unusual morphology distinct from the other three types reported in Ulva: along the middle of the longitudinal axis, both sides of the thallus are connected, forming a thin sheet in the middle with bilateral tubular structures, like a bow-tie in transverse view. We found a rapid Ulva bioinvasion and we provide fundamental new data for the future monitoring and risk assessment of green tides in China.
Introduction
The green macroalgal genus Ulva Linnaeus (Ulvophyceae, Chlorophyta) has a cosmopolitan distribution in marine, estuarine and freshwater environments worldwide (Reed & Russell, Citation1979; Martins et al., Citation1999; Maggs et al., Citation2007; Shimada et al., Citation2008; Ichihara et al., Citation2009). A total of 130 species have been identified to date (Guiry & Guiry, Citation2019). Because of its rapid growth, diverse reproductive patterns and adaptability to wide-ranging environmental conditions, Ulva species are often the dominant taxa in harmful blooms called green tides (Fletcher, Citation1996; Taylor et al., Citation2001; Lin et al., Citation2008). During the bloom, masses of floating seaweed may block sunlight, preventing benthic algae from photosynthesizing, or the bloom may release toxic substances such as hydrogen sulphide into the sediments and atmosphere, altering the local community structure and damaging the marine ecosystem (Yabe et al., Citation2009; Teichberg et al., Citation2010; Lyons et al., Citation2014). Green tides are occurring at an accelerated rate worldwide because of increased eutrophication (Smetacek & Zingone, Citation2013). In China, which has the most severe blooms globally in terms of biomass, area and drifting distance, green tides have occurred annually in the Yellow Sea since 2007 (Keesing et al., Citation2011; Zhao et al., Citation2015). These blooms have caused heavy economic losses and acute environmental problems (Ye et al., Citation2011; Liu et al., Citation2013a).
To efficiently monitor and forecast green tides, it is first necessary to determine the regional distribution of Ulva species. In China, seaweed diversity, including species belonging to Ulva, has been investigated extensively based on morphological features (Tseng, Citation1962, Citation2009; Dong, Citation1963; Tseng & Chang, Citation1963; Ding, Citation2013; Ding et al., Citation2015; Titlyanov et al., Citation2015). Over 2000 Ulva specimens have been deposited in the herbarium of the Institute of Oceanology, Chinese Academy of Sciences (IOCAS) since the 1940s. However, the morphological identification of Ulva is notoriously complex because of the lack of defining characters (Bliding, Citation1963; Tanner, Citation1979; Koeman & van den Hoek, Citation1981; Phillips, Citation1988; Hayden et al., Citation2003). These limited morpho-anatomical features vary widely depending on environmental factors (Tan et al., Citation1999) such as salinity (Reed & Russell, Citation1978; Blomster et al., Citation1998), temperature (Tanner, Citation1986; Blomster et al., Citation2002), or interactions with associated bacteria (Weiss et al., Citation2017; Kessler et al., Citation2018). In some cases, species may exhibit two distinct morphologies: monostromatic tubes or distromatic sheets (Bonneau, Citation1977; Provasoli & Pintner, Citation1980). For this reason, molecular identification techniques have been developed and used in regional investigations of Ulva around the world, including but not limited to Japan (Shimada et al., Citation2008; Ogawa et al., Citation2013; Masakiyo & Shimada, Citation2014), Korea (Kang et al., Citation2019), Hawaiʻi (O’Kelly et al., Citation2010; Spalding et al., Citation2016), the North-east Pacific (Hayden & Waaland, Citation2004; Saunders & Kucera, Citation2010), New Zealand (Heesch et al., Citation2009), Australia (Kraft et al., Citation2010; Kirkendale et al., Citation2013; Phillips et al., Citation2016), the Mediterranean (Wolf et al., Citation2012; Krupnik et al., Citation2018), and western Europe (Malta et al., Citation1999; Loughnane et al., Citation2008).
Through molecular surveys, recent variations have been detected in the species composition of Ulva (Israel & Einav, Citation2017), and many new or cryptic species have been identified (Shimada et al., Citation2008; Kraft et al., Citation2010; Masakiyo & Shimada, Citation2014). Additionally, alien or new Ulva species have been recorded in Ireland (Loughnane et al., Citation2008), the Mediterranean (Flagella et al., Citation2010; Israel & Einav, Citation2017; Krupnik et al., Citation2018; Miladi et al., Citation2018), Japan (Shimada et al., Citation2008; Ogawa et al., Citation2013), New Zealand (Heesch et al., Citation2009), Australia (Kirkendale et al., Citation2013; Hanyuda & Kawai, Citation2018), Hawaii (O’Kelly et al., Citation2010) and the Gulf of Mexico (Melton et al., Citation2016). Compared with red or brown seaweeds, the spread of green seaweeds is usually more rapid: bioinvasion of Ulva species might occur via ballast water, with mariculture, the seafood trade, or attachment on ship hulls (Mineur et al., Citation2008; Heesch et al., Citation2009; Wolf et al., Citation2012; Melton et al., Citation2016).
Alien Ulva species may cause harmful algal blooms in new habitats. For example, U. tepida Masakiyo & S. Shimada was found as an alien species forming green tides on the western Indian coast (Bast et al., Citation2014). In addition, both U. ohnoi M. Hiraoka & S. Shimada and U. tepida were found to bloom once introduced into Australia (Lawton et al., Citation2013; Phillips et al., Citation2016). In the Yellow Sea, the dominant U. prolifera O.F. Müller was revealed to be a unique ecotype that was genetically distinct from all attached populations, implying that this ecotype has a potential exogenous origin (Zhao et al., Citation2015). Moreover, blooming Ulva can produce large numbers of propagules, increasing the probability for the further occurrence and diffusion of green tides (Zhao et al., Citation2018). To effectively manage the risks of green tides within a region of interest, the monitoring of all Ulva species, including those newly described or reported, must be improved.
Although regional molecular surveys of attached Ulva species have been conducted in China (Jiang et al., Citation2008; Duan et al., Citation2012; Han et al., Citation2013; Liu et al., Citation2013b; Zhao et al., Citation2013), no report on alien species or new records has been published. Based on a molecular investigation of Ulva spp. along the coast of China over the past decade, we selected possible new records for further analyses, including molecular identification and distribution patterns in China and the rest of the world. We also present a detailed description of the unusual morphology and anatomy, as well as the morphogenetic process, for one green tide-forming species.
Materials and methods
Seaweed collection and culture
From August 2007 to November 2018, Ulva samples were collected in the intertidal zone along the coast of China including the Bohai Sea, the Yellow Sea, the East China Sea and the South China Sea (). All specimens were transported to the laboratory in an ice-cooled container for further processing. Using sterilized seawater, specimens were washed several times to remove any attached impurities or epiphytes. A total of 195 Ulva specimens were selected for this study based on a preliminary molecular investigation (Supplementary table 1). Some specimens were pressed on herbarium sheets for vouchers, and others were cultured in Von Stosch’s Enriched (VSE) medium, renewed once a week, at 20°C with a 12 h: 12 h L:D photoperiod and a photosynthetic irradiance of about 70–100 µmol photons m–2 s–1. All voucher specimens (with assigned numbers MBM 286543–286549) were deposited in the Marine Biological Museum of Chinese Academy of Sciences (MBMCAS) at the Institute of Oceanology, Chinese Academy of Sciences, Qingdao, China.
Molecular identification and phylogenetic analysis
A piece of each specimen, about 5 mm2, was removed for DNA extraction. Total genomic DNA was extracted by using a Plant Genomic DNA Extraction Kit (Tiangen Biotech Co., Ltd, Beijing, China) following the provided instructions. The polymerase chain reaction (PCR) mixture contained 12.5 µl of 2× Taq polymerase (Novoprotein Scientific Inc., Suzhou, China), 0.5 µl of reverse primer (10 µmol l–1), 0.5 µl of forward primer (10 µmol l–1), 2 µl of genomic DNA, and 9.5 µl of ultrapure water, for a total volume of 25 µl. PCR amplifications were carried out in a Bioer Thermal Cycler TC-XP-D (Hangzhou Bioer Technology Co. Ltd, Hangzhou, China). Three pairs of primers – ITS-a and ITS-d for internal transcribed spacer (ITS, including ITS1, 5.8S and ITS2) (Leskinen & Pamilo, Citation1997), rbcL-RH1 and rbcL-1385r for rbcL (Manhart, Citation1994), and tufGF4 and tufAR for tufA (Saunders & Kucera, Citation2010) – were used in this study. The PCR profile for amplifying ITS was an initial denaturation at 94°C for 10 min, 35 cycles of 94°C for 1 min, 54°C annealing for 50 s, 72°C extension for 1 min, and a final extension at 72°C for 10 min; for rbcL it was an initial denaturation step at 94°C for 4 min, 35 cycles of 94°C for 1 min, 54°C for 50 s, 72°C for 1 min, and a final step at 72°C for 10 min; for tufA it was an initial 4 min denaturation at 94°C, 38 cycles of 1 min at 94°C, 30 s at 45°C, 1 min at 72°C, and a final 10 min at 72°C for an extra extension. PCR products were visualized using gel electrophoresis in a 2.0% agarose gel stained with Super GelRed (US Everbright Inc., Suzhou, China). All PCR products were purified using a Gel Extraction Kit (200) (OMEGA Bio-Tek, Georgia, USA) according to the manufacturer’s instructions, and were sent for commercial sequencing (Ruibo BioTech Co. Ltd, Qingdao,China), by Genetic Analyzer (ABI3730XL, USA), based on the Sanger method.
Phylogenetic analysis was performed with DNA sequences from all samples and those downloaded from GenBank, plus sequences from Ulvaria or Blidingia as outgroups. Since DNA sequences from type material are the most reliable (Hanyuda & Kawai, Citation2018; Hughey et al., Citation2018, Citation2019), when we chose reference sequences we preferentially selected sequence data derived from the type material or topotype material. If neither of these was available we chose other published references. After sequence alignment by ClustalX (1.83) (Thompson et al., Citation1997), maximum likelihood (ML) phylogenetic trees were constructed with MEGA 6.0 using a GTR+G model (Tamura et al., Citation2013). Statistical support for individual branches was estimated by 1000 bootstrap replicates. To analyse the global biogeographic distribution patterns for each potential new record in China, all highly similar sequences (identity ≥98%) were downloaded from GenBank. After subsequent phylogenetic analysis and reassessment of species identity, the sampling location information for each selected sequence was extracted. All biogeographic distribution information for these species, from AlgaeBase, was included for reference (Guiry & Guiry, Citation2019).
Morphological studies
Because the Ulva meridionalis R. Horimoto & S. Shimada (Citation2011) samples collected in this study had an unusual shape that differed from that of the haplotype in Japan, the morphological and anatomical characteristics of Ulva meridionalis were observed and recorded in detail. All photographs of gross morphological features, such as the size and branching pattern of thalli, were taken with a Canon PC2152 digital camera (Canon Inc., Tokyo, Japan). Anatomical observations – the shape, size and arrangement of cells; the pyrenoid number per cell in the upper, mid- and basal region of thalli; and the position of chloroplasts in cells (Blomster et al., Citation1998) – were carried out under a BH2 light microscope (Olympus Corp., Tokyo, Japan). Lugol’s iodine, consisting of 6% potassium iodide and 4% iodine crystals, was used to stain the pyrenoids in cells or the zoids of reproductive cells. Anatomical features in transverse section of different parts of thalli, including cell size, shape and chloroplast position in cells, as well as the traits of the rhizoidal cells, were also examined. All images of anatomical features were taken with a CCD camera (Scope Tek MDC200, Mingshi, Ningbo, China) mounted on the microscope.
Reproduction and morphogenesis
To record the entire life history of Ulva meridionalis, the punching method was used to establish a unialgal culture from zoids (Hiraoka & Enomoto, Citation1998); small fragments (1–5 mm long) of the middle or upper parts of healthy thalli were excised to induce zoid formation. The freshly released zoids were transferred into new Petri dishes filled with 15 ml of VSE medium and cultured under the same conditions mentioned above. The phototaxis of zoids was recorded, and key morphological features such as the number of zoids in each sporangium or gametangium, as well as the size, eyespot and number of flagellates for each zoid, were examined at 600× magnification under the light microscope. Furthermore, the entire period was recorded from the germination of zoids to formation of adult thalli and from the cell division pattern at the initial developmental stage of zoids, the formation time of rhizoidal cells, and the morphological development of thalli. To clearly understand the morphogenesis of tubular thalli, frozen sections of thalli at different developmental stages were prepared with a cryostat microtome (Microm HM505E Cryostat, Walldorf, Germany).
Results
Molecular identification and phylogenetic analysis
A total of 220 nucleotide sequences of ITS (about 700 bp), including 186 from identified samples (nine of our 195 samples failed to sequence) and 34 from GenBank as references, were combined for the construction of an ML phylogenetic tree. All samples were clearly resolved into six clades, clades 1 to 6, with high bootstrap values (). Within each clade, the maximum sequence divergences were small, varying from 0.4% to 2.4%. These values fall into the general range for ITS intraspecific sequence divergence in Ulva (0–2.3%; Blomster et al., Citation1999), suggesting that all samples within each clade belonged to one species. The sequence divergences among the six clades varied from 6.6% to 11.3%, within the general range for interspecific ITS sequence divergence in Ulva (7.5–20.3%; Blomster et al., Citation1999), indicating that the clades represented six distinct species. The two other ML phylogenetic trees based on either rbcL or tufA showed similar topological structures to that of the ITS-ML tree (Supplementary figs 1, 2).
Fig. 2. ML phylogenetic tree based on ITS DNA sequences. Numbers at the nodes indicate bootstrap values. GenBank accession numbers and localities for all reference sequences are provided. Asterisks indicate the sequences generated from the holotype specimens. Sequences with a ‘#’ symbol are from lectotype materials or topotype materials, or matched to the sequences from holotype materials or topotype materials. Numbers in parentheses following the sample no. represent the total number of highly similar sequences (identity ≥ 99.5%)
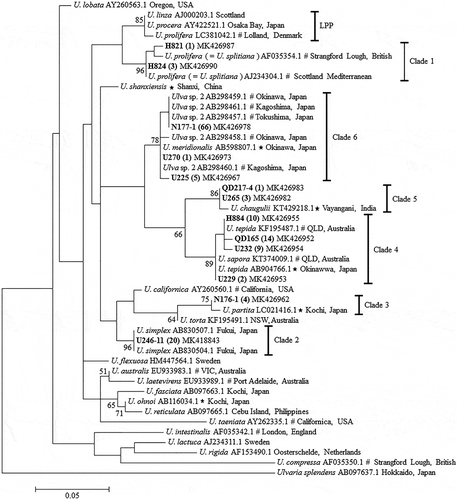
None of the six clades clustered with any of the 12 recorded Ulva species in China, U. prolifera, U. linza Linnaeus 1753, U. compressa Linnaeus 1753, U. intestinalis Linnaeus 1753, U. australis Areschoug 1854 (= U. pertusa Kjellman 1897), U. lactuca Linnaeus 1753, U. fasciata Delile 1813, U. flexuosa Wulfen 1803, U. rigida C. Agardh 1823 (Tseng, Citation2009; Ding, Citation2013), the freshwater species U. shanxiensis L. Chen, J. Feng & S.L. Xie 2014 (Chen et al., Citation2015), U. laetevirens Areschoug 1854 and U. ohnoi. Instead, the six clades were tightly clustered with sequences of, respectively, U. meridionalis, U. tepida (= U. sapora J.A. Phillips, R.J. Lawton & C. Carl 2016), U. chaugulii M.G. Kavale & M.A. Kazi 2016; U. simplex (K.L. Vinogradova) H.S. Hayden, Blomster, Maggs, P.C. Silva, Stanhope & Waaland (first described as Enteromorpha simplex by R.P.T. Koeman & Hoek in 1982); U. partita Ichihara 2015; and U. splitiana Alongi, Cormaci & Furnari (first described as Enteromorpha jugoslavica by Bliding in 1960). The reference sequences of U. meridionalis, U. tepida, U. chaugulii and U. partita were all derived from holotype specimens (Horimoto et al., Citation2011; Ogawa et al., Citation2013; Ichihara et al., Citation2015; Kazi et al., Citation2016). For another two species, U. splitiana and U. simplex, there were no sequences from their holotype specimens and sequences from topotype materials were not uploaded to GenBank (Cui et al., Citation2018). So for U. splitiana we selected two sequences of U. prolifera in clade 1 which had been identified as U. splitiana by crossing experiments and had been matched to the sequences from topotype materials (Cui et al., Citation2018). For U. simplex, the sequences were from Ogawa et al. (Citation2013), in which the samples were identified based on morphological characteristics and ecological properties. In addition, U. sapora was specified as a synonym of U. tepida in clade 4 (Masakiyo & Shimada, Citation2014; Phillips et al., Citation2016) and Ulva sp. 2 in clade 6 was confirmed as U. meridionalis (Shimada et al., Citation2008; Horimoto et al., Citation2011). These six species were thus identified as new records in China. For the first time, the dominant species forming green tides at several locations in the South China Sea was identified as U. meridionalis.
Distribution and habitat
For each of the six new records in China mentioned above, the global distribution pattern was analysed by mining sequence data and their corresponding sampling information from GenBank. For instance, among 26 ITS sequences sharing high identity (≥ 98%) with U. meridionalis, 23 were confirmed by phylogenetic analysis to be conspecific (Supplementary fig. 3). According to its geographic information (Supplementary table 2), U. meridionalis seems to be distributed mainly in East Asia and Australia. Interestingly, it was recorded in rivers or brackish lakes in southern Japan (Shimada et al., Citation2008; Horimoto et al., Citation2011), but according to our samples it was mostly in marine environments along China’s coastline, including the Bohai Sea, Yellow Sea, East China Sea and South China Sea. In Australia, U. meridionalis was common in the intertidal zones of Queensland (Phillips et al., Citation2016). The distribution of U. simplex was limited in East Asia, along the coast of China (34 ITS sequences from our samples) and in the central region of Japan (four ITS and one rbcL sequences from GenBank) (Supplementary fig. 4, Supplementary table 3); it also existed in the Baltic Sea according to morphological taxonomy from AlgaeBase (Guiry & Guiry, Citation2019). Additionally, based on 21 and 48 highly similar sequences from GenBank respectively, U. chaugulii shared a similar distribution pattern with U. tepida (Supplementary figs 5, 6, Supplementary tables 4, 5). Both were found along the coast of the Yellow Sea and the South China Sea in China, as well as the west coast of India (Bast et al., Citation2014; Kazi et al., Citation2016), Mediterranean coast of Israel (Krupnik et al., Citation2018), Persian Gulf in Iran (Pirian et al., Citation2016) and coast of Queensland in Australia (Lawton et al., Citation2013; Phillips et al., Citation2016). U. tepida was also found in Okinawa and Kanagawa in Japan. Among the six species, U. partita was the least collected; it was only recorded in Hokkaido, Fukui, and Kochi in Japan (eight sequences from GenBank), and one location in China (Supplementary fig. 7, Supplementary table 6). U. splitiana (24 sequences from GenBank) had the widest range: Europe (Denmark, Greenland, Iceland, Britain and the Netherlands), East Asia (China) and even North America (the Atlantic and Arctic coasts of the USA and Canada) (Supplementary fig. 8, Supplementary table 7). Also, in the AlgaeBase database it was found on the coasts of France and Spain (Guiry & Guiry, Citation2019).
Figs 3–9. Morphology of U. meridionalis. Fig. 3. Voucher specimen collected from Huiquan Bay, Qingdao, China (MBM286543). Figs 4–6. Axis showing central longitudinal fusion of cells along the tube, forming a double tubular thallus. Figs 7–9. Transverse constrictions of thallus. Scale bar: 1 cm in Fig. 3; 3 mm in Figs 4–9
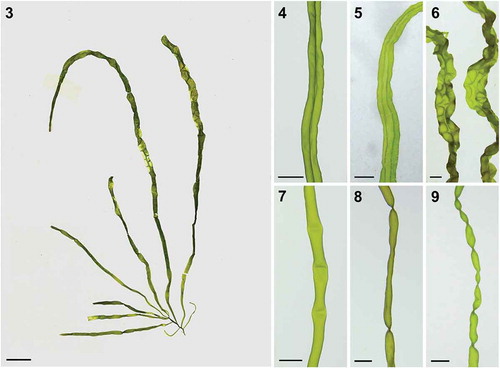
Morphology and anatomy
Ulva meridionalis grew mainly in tufts on gravel or reefs in the intertidal zone. Well-developed thalli were usually light green, fragile with a smooth surface, and measured up to 25 cm in height and 0.5 cm in width (n = 20) (). Based on its tubular thalli, U. meridionalis appeared to be an Enteromorpha-like species. However, the axes were always connected in part longitudinally, forming a thin middle sheet between a pair of tubes (–). The thalli had an obvious main branch, which decreased in width towards the base. Lateral branches always occurred in the basal region of the thalli and rarely in the mid- or upper regions. Second-order branching was not observed among our samples (). Usually, there were many transverse constrictions 2–15 mm (n = 20) apart (–).
In surface view under the microscope, most cells except those in the basal region were triangular, rectangular or polygonal with rounded corners. They were irregularly arranged throughout the thallus, but there were small areas with about 6–28 cells aligned in rows (–). Cells in the upper basal region of the thalli averaged 19 ± 3.3 µm in length × 14.6 µm ± 2.0 in width (, ). In the middle region, cells were 15.7 µm ± 0.3 × 11.3 µm ± 3.0 (), and in the upper region, were 17.3 µm ± 2.6 × 12.8 µm ± 2.4 (n = 50) (). Each cell contained a single chloroplast, typically filling the whole cell () but occasionally towards one side wall (). Chloroplasts had 1–11 (3.67 ± 1.16) pyrenoids in the upper basal region of the thalli (), 4.29 ± 1.35 in the mid-thallus (), and 3.64 ± 1.14 in the upper region (n = 200) (). The branch apices were usually rounded and multiseriate (), except in a few cases with a uniseriate row one to three cells in length. Cells in the basal region were up to 114 µm in length and contained many starch granules (), with lots of translucent filamentous extensions inside the monostromatic cell layer towards the substrate (). These hyaline thread-like structures also occurred in the basal region of branches adjacent to the rhizoidal cells, which was not mentioned in Horimoto et al. (Citation2011).
Figs 10–22. Surface and transverse sectional (TS) views of U. meridionalis. Figs 10, 11. Upper basal region. Fig. 12. Middle region. Fig. 13. Upper region. Fig. 14. Pyrenoids in upper basal region. Fig. 15. Pyrenoids in middle region. Fig. 16. Pyrenoids in upper region. Fig. 17. Apex of branch. Fig. 18. Basal region. Fig. 19. Rhizoidal cells with translucent filamentous extensions. Fig. 20. TS of upper region. Fig. 21. TS of middle region. Fig. 22. TS of upper basal region. Black arrow indicates trabeculae inside tubular thallus. Scale bar: 10 μm
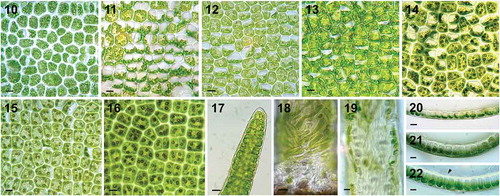
In transverse section (TS), the tubes consisted of a single layer of cells, and the chloroplasts were positioned towards the outside of the tube (–). Both monostromatic and distromatic thallus parts were seen in TS, consistent with the surface-view observation. The pair of monolayered tubes were formed of quadrangular cells with blunt corners, 22.6 µm ± 1.3 thick in the upper thalli (), 32.6 µm ± 1.4 in the middle region (), and 45.9 µm ± 1.9 in the upper basal region where there were trabeculae inside the tubular thalli (). In contrast, the median bilayered thallus was composed of rectangular cells with rounded corners 60.8 µm ± 3.3 thick (n = 35).
The main morphological characteristics, including gross morphology and pyrenoid number, of the five other samples (i.e. excluding U. meridionalis) – U. tepida (), U. partita (), U. simplex (), U. splitiana () and U. chaugulii () – matched those described in the literature (Ogawa et al., Citation2013; Ichihara et al., Citation2015; Kazi et al., Citation2016; Phillips et al., Citation2016; Cui et al., Citation2018). Interestingly, two types of thallus morphology were detected in U. chaugulii. The samples collected in Qingdao had flattened, banded and distromatic blades (), while those collected in Zhanjiang were tubular as described by Kazi et al. (Citation2016) ().
Reproduction and morphogenesis ofUlva meridionalis
Two to five days after induction, zoids were formed and released from the thalli, which had turned light brown. Each sporangium or gametangium contained eight spores or 16 gametes, respectively (, ). After the swimming zoids were completely released, the remaining cells were empty with one round hole on the surface of the cell wall (). The quadriflagellate zoospore was ovate, 11.1 µm ± 0.9 × 5.5 µm ± 0.6 (n = 50) with a reddish eyespot and negative phototaxis (). Meanwhile, the biflagellate gametes exhibited positive phototaxis, and were 7.1 µm ± 0.4 × 4.1 µm ± 0.6 (n = 50) in size, also with a reddish eyespot ().
Figs 29–46. Reproduction and morphogenesis of U. meridionalis. Fig. 29. Sporangia. Fig. 30. Gametangia. Fig. 31. Exit pores of sporangia. Fig. 32. Quadriflagellate zoospores. Fig. 33. Biflagellate gametes. Fig. 34. Settled zoospore. Fig. 35. Two-cell stage. Fig. 36. Uniseriate germling. Fig. 37. Multiseriate filamentous thallus. Fig. 38. Thallus with branches arising from basal region. Figs 39–42. Surface view of formation of double-tubular structure in chronological order. Figs 43–46. Transverse views of same stages in formation of double-tubular structure in chronological order. Scale bars: 10 μm in Figs 29–36, 100 μm in Fig. 37, 1 cm in Fig. 38, 2 mm in Figs 39–42, and 500 μm in Figs 43–46
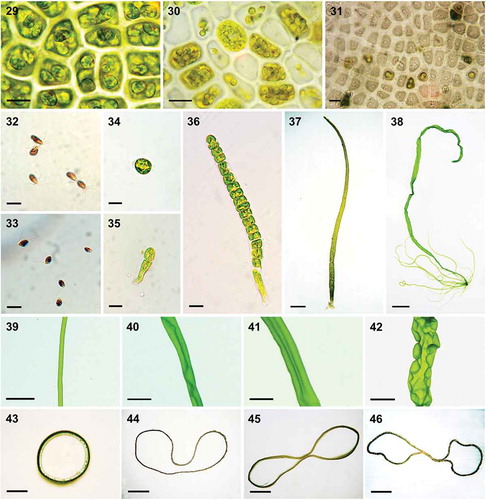
We found that both the spores and gametes of U. meridionalis could develop and grow into adult thalli. Here only the usual germination of the spore is described in detail. Upon release from the sporangium and a period of swimming, the quadriflagellate zoospores began attaching to the substratum, and became bigger and rounded, with the reddish eyespot and flagella disappearing (). The first cell division occurred after 2–3 days, at the same time that the rhizoidal cell started to proliferate, forming filamentous extensions (). Then the germinated spores developed into uniseriate filaments by dividing longitudinally (), while the transverse division did not start until there were more than 20 cells, when the filamentous extensions from the rhizoidal cell had grown to about 170 µm. Then, the multiseriate thallus generated a tube-like frond () with branches arising from the basal region (). As growth continued, transverse folds or constrictions formed on the branches at intervals of 2 to 12 mm (n = 20), as in wild samples. Meanwhile, the monolayered tubular thalli became relatively flattened. The tube started connecting along the longitudinal axis, while the bilateral parts remained tubular (–). In general, this distinct structure – a thin distromatic sheet in the middle and wide monostromatic tubes at the margins, which resembles a bow in transverse view – was initially found in the main branch. We found that this bow-shaped structure, which was present in both wild and regenerated U. meridionalis samples, was quite stable, without any alternation between generations or different geographic populations.
Discussion
To our knowledge, none of the six Ulva species described in this study have been formally recorded in China, whether based on morpho-anatomical (Tseng, Citation1962, Citation2009; Dong, Citation1963; Ding, Citation2013; Ding et al., Citation2015; Titlyanov et al., Citation2015) or DNA sequence data (Jiang et al., Citation2008; Duan et al., Citation2012; Han et al., Citation2013; Liu et al., Citation2013b; Zhao et al., Citation2013). According to the phylogenetic trees (Supplementary figs. 3–8) and the geographic information from GenBank (Supplementary tables 2–7), some DNA sequences, matching two of these six new records, were from samples collected in China by other researchers; however, none was correctly identified. For instance, a total of 10 ITS sequences, identical to that of U. meridionalis, were generated from the samples collected along the Chinese coast (Supplementary fig. 3, Supplementary table 2). Five had been annotated as Ulva sp. and five as U. prolifera. Because numerous molecular phylogenetic analyses have shown that U. prolifera belongs to the LPP (linza-prolifera-procera) cluster (Jiang et al., Citation2008; Shimada et al., Citation2008; Cui et al., Citation2018), which was separated from U. meridionalis, these could be confirmed as incorrect species identifications. Similarly, two ITS sequences from China, which were identical to those of U. tepida, should be considered to have been incorrectly attributed to U. intestinalis, since U. tepida did not cluster with U. intestinalis (Supplementary fig. 6, Supplementary table 5). In contrast, for U. simplex (Supplementary fig. 4, Supplementary table 3), U. chaugulii (Supplementary fig. 5, Supplementary table 4), U. partita (Supplementary fig. 7, Supplementary table 6) and U. splitiana (Supplementary fig. 8, Supplementary table 7), no identical ITS sequence was mined from samples collected in China.
Previous morphological investigations in China identified 11 Ulva species (Tseng, Citation1962, Citation2009; Dong, Citation1963). This was followed by a systematic revision in which 15 Ulva species were recorded, but still based on morphological methods (Ding, Citation2013; Ding et al., Citation2015). Two new records, U. laetevirens (unpublished paper) and U. ohnoi (unpublished paper), and one new species, U. shanxiensis, were added to this list (Chen et al., Citation2015), based on molecular analyses. These 18 species consist of nine blade-forming members, including U. lactuca, U. australis (= U. pertusa), U. fasciata, U. rigida, U. laetevirens, U. ohnoi, U. conglobata Kjellman 1897, U. reticulata Forsskål 1775, U. spinulosa Okamura & Segawa 1936, and nine Enteromorpha-like tubular members, which are U. prolifera, U. linza, U. flexuosa, U. compressa, U. intestinalis, U. shanxiensis, U. clathrata (Roth) C. Agardh 1811, U. paradoxa C. Agardh 1817 (= U. crinita J.D. Hooker & Harvey in J.D. Hooker 1847) and U. ralfsii (Harvey) Le Jolis 1863. DNA sequence data are still lacking for U. conglobata, U. reticulata, U. spinulosa, U. clathrata, U. paradoxa and U. ralfsii to confirm their presence in China. In terms of morphology, for three Enteromorpha-like species, U. clathrata has many fine uniseriate branches, U. paradoxa has slender tubes 2–5 mm wide and U. ralfsii consists of extremely thin filaments less than 80 µm in diameter (Ding, Citation2013). All are distinct from the six species recorded in this study.
Among these six Ulva species, the U. meridionalis samples in this study were morphologically distinct from the samples collected in Japan’s Okinawa Prefecture (Horimoto et al., Citation2011). Since this species is distributed widely along China’s coast and dominates green tides in the South China Sea, its morphological and anatomical characteristics were compared in detail with those from Japan, with the following differences observed. First, the distinct bow-shaped structure was visible in transverse view for almost all the Chinese U. meridionalis samples, and there were also many transverse folds. In contrast, the tubular specimens described by Horimoto et al. (Citation2011) have either wrinkled or smooth thalli. Second, second-order branching in U. meridionalis was reported by Horimoto et al. (Citation2011), but only primary branches were observed in our samples. Several studies have shown that environmental factors, such as photon irradiance or salinity, could change the branching pattern in Ulva (De Silva & Burrows, Citation1973; Reed & Russell, Citation1978). In particular, low salinity may induce a significant increase of branching in U. intestinalis (Leskinen et al., Citation2004) and U. prolifera (Gao et al., Citation2016). The Japanese U. meridionalis samples were collected from freshwater or brackish environments (Shimada et al., Citation2008; Horimoto et al., Citation2011) and ours were from higher salinity waters (> 20 psu); thus, the differences in branching pattern between Chinese and Japanese samples might be a result of the different salinities. Finally, the number of pyrenoids differed greatly. Horimoto et al. (Citation2011) reported that each cell contained 1–4 pyrenoids, with most having 1–2. In contrast, there were up to 11 pyrenoids in our samples; cells containing 3–5 pyrenoids made up the majority, while those with only 1–2 were rare. Although pyrenoid number per cell was previously considered to be an important trait in the discrimination of Ulva species (Bliding, Citation1968; Koeman & van den Hoek, Citation1981; Coat et al., Citation1998), it has also been shown to be too variable for accurate species delimitation (Tanner, Citation1986; Phillips, Citation1988). For instance, pyrenoid number per cell fluctuates seasonally in U. lactuca and U. scandinavica (Malta et al., Citation1999). Our study suggests that pyrenoid number in different U. meridionalis populations is highly variable, and that it might not be a reliable trait for identifying this species.
U. meridionalis exhibited a novel pattern of morphogenesis that differed from the other three patterns seen in Ulva. In general, there are two typical morphologies in Ulva species: monostromatic tubes and distromatic sheets. Both types share the same early developmental process, in which an attached zoid initially divides into one apical cell and one rhizoidal cell, and then grows into a uniseriate filament and subsequently produces a multiseriate and tubular sporeling. Afterwards, one type maintains the tubular form () while the other turns into a completely distromatic frond () as the walls of hollow sporelings start to coalesce (Phillips, Citation1988). For the third pattern, seen in U. linza, coalescence is incomplete, generating a distromatic thallus with bilateral thin tubular margins (Kapraun & Flynn, Citation1973), forming a dumbbell structure in transverse section (). By contrast, the coalescence in U. meridionalis stopped soon after the walls of the tubular thallus reached the longitudinal axis, thus producing a distinct bow-shaped structure in transverse sectional view (). Unlike in U. linza, the middle distromatic part in U. meridionalis was only 0.5–3.0 mm in width, and the monostromatic tubular sacs to both sides were clearly visible. Notably, this bow-shaped structure in U. meridionalis was quite stable in both wild and regenerated samples, regardless of the fact that they were from different geographic populations. Molecular phylogenetic analyses have shown that monostromatic tubes and distromatic sheets are dispersed throughout the phylogeny of Ulva and do not form separate monophyletic clades. Therefore, these morphologies have evolved multiple times, and the two patterns may be controlled by a molecular switch. Thus, this divergence might occur independently many times in evolution (Tan et al., Citation1999). This switch could be easily triggered even during the individual developmental process, for both the tubular (Tan et al., Citation1999) and sheet-like Ulva species (Bonneau, Citation1977). We found blade-like or tubular forms of U. chaugulii collected from different sites (, ). Moreover, thalli of U. meridionalis were tubular in freshwater or brackish environments in Japan and bow-patterned in marine environments in China. Each Ulva species may have multiple potential developmental patterns, which are influenced by a combination of environmental factors. Overall, the blade or tubular patterns are displayed in most Ulva species. The other two patterns, the dumbbell in U. linza and the bow in U. meridionalis, have only been found in a few Ulva species, suggesting that they may be controlled by more complicated switches.
Figs 47–50. Four morphogenesis patterns in Ulva. Fig. 47. Tubular type. Fig. 48. Sheet type. Fig. 49. Dumbbell type. Fig. 50. Double-tubular type

As we found up to six newly recorded Ulva species in China, the possibility for serious bioinvasion and the subsequent risk of green tides along the coastline of China deserves special attention. In China, the diversity and flora of Ulva have been investigated extensively (Tseng, Citation1962, Citation2009; Dong, Citation1963; Ding, Citation2013; Ding et al., Citation2015; Titlyanov et al., Citation2015). Over 2000 Ulva specimens have been deposited in the herbarium of IOCAS since the 1940s. In this study, we have shown that U. meridionalis has a distinct and stable morphology, making it readily distinguishable from other recorded Ulva species. However, there are no previous records or descriptions of this species in China, suggesting it may have recently invaded the region. Originally, U. meridionalis was recorded in north-eastern Australia (Phillips et al., Citation2016), Okinawa, southern Kyushu, and the east and west coastline of Honshu in Japan (Shimada et al., Citation2008; Horimoto et al., Citation2011), which indicates that it is a typical subtropical species. However, along the coast of China, it bloomed in subtropical areas as a dominant species in spring and autumn and even emerged in a temperate area in summer, suggesting that it has a strong capacity to spread northward, perhaps driven by the global warming (Ma et al., Citation2009; Liu et al., Citation2012). Similarly, U. tepida and U. chaugulii were categorized as subtropical species, as they were primarily recorded in the Indo-Pacific region including north-eastern Australia (Lawton et al., Citation2013; Phillips et al., Citation2016), the west coast of India (Bast et al., Citation2014; Kazi et al., Citation2016), the Persian Gulf (Bast et al., Citation2014; Kazi et al., Citation2016) and the eastern Mediterranean where U. chaugulii is considered invasive (Krupnik et al., Citation2018). In China, they were mainly found in the subtropical region, but both spread northward to the temperate area. Because the records for U. chaugulii in China are sparse, its invasion is probably recent. By contrast, global distribution records for U. simplex, U. splitiana and U. partita show that they are all temperate species in the northern hemisphere (Ogawa et al., Citation2013; Ichihara et al., Citation2015; Cui et al., Citation2018). Similar to U. chaugulii, U. splitiana has been rarely recorded in China and U. partita was recorded only once, indicating that they are probably new invaders as well, and this was similarly supported by the non-indigenous species (NIS) analyses (Supplementary table 8).
Globally, occurrences of green tides dominated by Ulva spp. are becoming more common (Smetacek & Zingone, Citation2013). As is now typical in China, floating Ulva spp. began blooming throughout the main sea areas in the past decade (Wang et al., Citation2015; Zhang et al., Citation2015), and the largest green tides in the world have been successively occurring in the Yellow Sea for more than 10 years (Zhao et al., Citation2018). The eutrophic conditions present along China’s coast are favourable for the occurrence of green tides (Li et al., Citation2015; Wei et al., Citation2015). Therefore, the ecological risks caused by invasive Ulva spp. require immediate attention. It was reported that U. meridionalis may dominate Ulva blooms in Japan (Tsubaki et al., Citation2017) and, under culture conditions, its daily growth rate could exceed 100% (Hiraoka, Citation2012). Likewise, U. tepida formed serious green tides on the west coast of India (Bast et al., Citation2014). In the South China Sea, U. meridionalis dominated the green tides in November 2018 and it co-occurred with U. tepida and U. chaugulii in April 2018. These three subtropical species are all probably invasive, and they have succeeded in exploiting their new temperate habitats, especially U. meridionalis with its wide distribution. Thus, improved monitoring of the distribution, biomass and green tide events in China is urgently needed.
Supplementary information
The following supplementary material is accessible via the Supplementary Content tab on the article’s online page at accssed https://doi.org/10.1080/09670262.2020.1740946
Supplementary table 1. List of specimens, with final taxonomic diagnosis, sample number, collection information, and GenBank accessions.
Supplementary table 2. Information of all highly similar sequences (identity ≥ 98%) downloaded from NCBI and identified as from U. meridionalis based on phylogenetic analysis.
Supplementary table 3. Information of all highly similar sequences (identity ≥98%) downloaded from NCBI and identified as from U. simplex based on phylogenetic analysis.
Supplementary table 4. Information of all highly similar sequences (identity ≥98%) downloaded from NCBI and identified as from U. chaugulii based on phylogenetic analysis.
Supplementary table 5. Information of all highly similar sequences (identity ≥98%) downloaded from NCBI and identified as from U. tepida based on phylogenetic analysis.
Supplementary table 6. Information of all highly similar sequences (identity ≥98%) downloaded from NCBI and identified as from U. partita based on phylogenetic analysis.
Supplementary table 7. Information of all highly similar sequences (identity ≥98%) downloaded from NCBI and identified as from U. splitiana based on phylogenetic analysis.
Supplementary fig. 1. ML phylogenetic tree based on DNA sequences of rbcL.
Supplementary fig. 2. ML phylogenetic tree based on DNA sequences of tufA.
Supplementary fig. 3. ML phylogenetic tree based on DNA sequences of ITS, with special reference to U. meridionalis.
Supplementary fig. 4. ML phylogenetic tree based on DNA sequences of ITS, with special reference to U. simplex.
Supplementary fig. 5. ML phylogenetic tree based on DNA sequences of ITS (A) and rbcL (B), with special reference to U. chaugulii.
Supplementary fig. 6. ML phylogenetic tree based on DNA sequences of ITS, with special reference to U. tepida.
Supplementary fig. 7. ML phylogenetic tree based on DNA sequences of ITS, with special reference to U. partita.
Supplementary fig. 8. ML phylogenetic tree based on DNA sequences of ITS, with special reference to U. splitiana.
Author contributions
W.F. Xie: collection and cultivation of the algae, DNA isolation, PCR, molecular phylogenetic analyses, manuscript preparation; C.H. Wu: sample collection; J. Zhao: sample collection; X.Y. Lin: sample collection; P. Jiang: original concept, sample collection, manuscript drafting; All authors: manuscript editing.
TEJP-2019-0066-File009.pdf
Download PDF (2.5 MB)Acknowledgements
The authors are very grateful to Prof. Chen W.Z. from Shantou Univ., Dr Pan X.J. from Ocean University of China, Dr Lin K.X. from the Chinese Research Academy of Environmental Sciences, and Prof. Cao X.H., Dr Guo Y., Sun Z.M., Wang J.F., Liu Z.Y., Feng D.W. from IOCAS for assistance with sample collection.
Disclosure statement
No potential conflict of interest was reported by the authors.
Supplemental Material
The following supplementary material is accessible via the Supplementary Content tab on the article’s online page at https://doi.org/10.1080/09670262.2020.1740946.
Additional information
Funding
References
- Bast, F., John, A.A. & Bhushan, S. (2014). Strong endemism of bloom-forming tubular Ulva in Indian west coast, with description of Ulva paschima sp. nov. (Ulvales, Chlorophyta). PLoS ONE, 9: e109295.
- Bliding, C. (1963). A critical survey of European taxa in Ulvales, Part I. Capsosiphon, Percursaria, Blidingia, Enteromorpha. Opera Botanica, 8: 1–160.
- Bliding, C. (1968). A critical survey of European taxa in Ulvales. Part II. Ulva, Ulvaria, Monostroma, Kornmannia. Botaniska Notiser, 121: 535–629.
- Blomster, J., Maggs, C.A. & Stanhope, M.J. (1998). Molecular and morphological analysis of Enteromorpha intestinalis and E. compressa (Chlorophytta) in the British Isles. Journal of Phycology, 34: 319–400.
- Blomster, J., Maggs, C.A. & Stanhope, M.J. (1999). Extensive intraspecific morphological variation in Enteromorpha muscoides (Chlorophyta) revealed by molecular analysis. Journal of Phycology, 35: 575–586.
- Blomster, J., Bäck, S., Fewer, D.P., Kiirikki, M., Lehvo, A., Maggs, C.A. & Stanhope, M. (2002). Novel morphology in Enteromorpha (Ulvophyceae) forming green tides. American Journal of Botany, 89: 1756–1763.
- Bonneau, E.R. (1977). Polymorphic behavior of Ulva lactuca (Chlorophyta) in axenic culture. I. Occurrence of Enteromorpha-like plants in haploid clones. Journal of Phycology, 13: 133–140.
- Chen, L., Feng, J. & Xie, S.L. (2015). Ulva shanxiensis (Ulvaceae), a new species from Shanxi, China. Novon, 23: 397–405.
- Coat, G., Dion, P., Noailles, M.C., de Reviers, B., Fontaine, J.M., Berger, P.Y. & de Goér, L.S. (1998). Ulva armoricana (Ulvales, Chlorophyta) from the coasts of Brittany (France). II. Nuclear rDNA ITS sequence analysis. European Journal of Phycology, 33: 81–86.
- Cui, J.J., Monotilla, A.P., Zhu, W.R., Takano, Y., Shimada, S., Ichihara, K., Matsui, T., He, P.M. & Hiraoka, M. (2018). Taxonomic reassessment of Ulva prolifera (Ulvophyceae, Chlorophyta) based on specimens from the type locality and Yellow Sea green tides. Phycologia, 57: 692–704.
- De Silva, M.W.R.N. & Burrows, E.M. (1973). An experimental assessment of the status of the species Enteromorpha intestinalis (L.) Link and Enteromorpha compressa (L.). Grev. Journal of the Marine Biological Association of the United Kingdom, 53: 895–904.
- Ding, L.P. (2013). Flora Algarum Marinarum Sinicarum, Tomus IV: Chlorophyta, No. 1: Ulotrichales, Chaetophorales, Phaeophilales, Ulvales, Prasiolales, Cladophorles, Acrosiphoniales. Science Press, Beijing.
- Ding, L.P., Huang, B.X. & Luan, R.X. (2015). New classification system of marine green algae of China. Guangxi Sciences, 22: 201–210.
- Dong, M.L. (1963). A preliminary phytogeographical studies on Chinese species of Enteromorpha. Oceanologia et Limnologia Sinica, 5: 46–51.
- Duan, W.J., Guo, L.X., Sun, D., Zhu, S.F., Chen, X.F., Zhu, W.R., Xu, T. & Chen, C.F. (2012). Morphological and molecular characterization of free-floating and attached green macroalgae Ulva spp. in the Yellow Sea of China. Journal of Applied Phycology, 24: 97–108.
- Flagella, M.M., Andreakis, N., Hiraoka, M., Verlaque, M. & Buia, M.C. (2010). Identification of cryptic Ulva species (Chlorophyta, Ulvales) transported in ballast water. Journal of Biological Research–Thessaloniki, 13: 47–57.
- Fletcher, R.L. (1996). The occurrence of “Green tides” – a review. In Marine Benthic Vegetation: Recent Changes and the Effects of Eutrophication (Schramm, W. & Nienhuis, P. H., editors), 7–43. Springer, Berlin.
- Gao, G., Zhong, Z., Zhou, X. & Xu, J. (2016). Changes in morphological plasticity of Ulva prolifera under different environmental conditions: a laboratory experiment. Harmful Algae, 59: 51–58.
- Guiry, M.D. & Guiry, G.M. (2019). AlgaeBase. World-wide electronic publication, National University of Ireland, Galway. http://www.algaebase.org.
- Han, W., Chen, L.P., Zhang, J.H., Tian, X.L., Hua, L., He, Q., Huo, Y.Z., Yu, K.F., Shi, D.J., Ma, J.H. & He, P.M. (2013). Seasonal variation of dominant free-floating and attached Ulva species in Rudong coastal area, China. Harmful Algae, 28: 46–54.
- Hanyuda, T. & Kawai, H. (2018). Genetic examination of the type specimen of Ulva australis suggests that it was introduced to Australia. Phycological Research, 66: 238–241.
- Hayden, H.S. & Waaland, J.R. (2004). A molecular systematic study of Ulva (Ulvaceae, Ulvales) from the northeast Pacific. Phycologia, 43: 364–382.
- Hayden, H.S., Blomster, J., Maggs, C.A., Silva, P.C., Stanhope, M.J. & Waaland, J.R. (2003). Linnaeus was right all along: Ulva and Enteromorpha are not distinct genera. European Journal of Phycology, 38: 277–294.
- Heesch, S., Broom, J.E.S., Neill, K.F., Farr, T.J., Dalen, J.L. & Nelson, W.A. (2009). Ulva, Umbraulva and Gemina: genetic survey of New Zealand taxa reveals diversity and introduced species. European Journal of Phycology, 44: 143–154.
- Hiraoka, M. (2012). Intensive biomass production by green seaweed Ulva. Journal of the Japan Institute of Energy, 91: 1154–1160.
- Hiraoka, M. & Enomoto, S. (1998). The induction of reproductive cell formation of Ulva pertusa Kjellman (Ulvales, Ulvophyceae). Phycological Research, 46: 199–203.
- Horimoto, R., Masakiyo, Y., Ichihara, K. & Shimada, S. (2011). Enteromorpha-like Ulva (Ulvophyceae, Chlorophyta) growing in the Todoroki River, Ishigaki Island, Japan, with special reference to Ulva meridionalis Horimoto et Shimada, sp. nov. Bulletin of the National Museum of Nature and Science, Series B, Botany, 37: 155–167.
- Hughey, J.R., Millerb, K.A. & Gabrielson, P.W. (2018). Mitogenome analysis of a green tide forming Ulva from California, USA confirms its identity as Ulva expansa (Ulvaceae, Chlorophyta). Mitochondrial DNA Part B, 3: 1302–1303.
- Hughey, J.R., Maggs, C.A., Mineur, F., Jarvis, C., Miller, K.A. Shabaka, S.H. & Gabrielson, P.W. (2019). Genetic analysis of the Linnaean Ulva lactuca (Ulvales, Chlorophyta) holotype and related type specimens reveals name misapplications, unexpected origins, and new synonymies. Journal of Phycology, 55: 503–508.
- Ichihara, K., Arai. S., Uchimura, M., Fay, J.E., Ebata, H., Hiraoka, M. & Shimada, S. (2009). New species of freshwater Ulva, Ulva limnetica (Ulvales, Ulvophyceae) from the Ryukyu Islands, Japan. Phycological Research, 57: 94–103.
- Ichihara, K., Suzuki, R., Yamazaki, T., Ota, S., Mogi, Y., Kagami, Y., Kuwano K. & Kawano, S. (2015). Ulva partita sp. nov., a novel Enteromorpha-like Ulva species from Japanese coastal areas. Cytologia, 80: 261–270.
- Israel, A. & Einav, R. (2017). Alien seaweeds from the Levant basin (Eastern Mediterranean Sea), with emphasis to the Israeli shores. Israel Journal of Plant Sciences, 64: 99–110.
- Jiang, P., Wang, J.F., Cui, Y.L., Li, Y.X., Lin, H.Z. & Qin, S. (2008). Molecular phylogenetic analysis of attached Ulvaceae species and free-floating Enteromorpha from Qingdao coasts in 2007. Chinese Journal of Oceanology and Limnology, 26: 276–279.
- Kang, J.H., Jang, J.E., Kim, J.H., Byeon, S.Y., Kim, S., Choi, S.K., Kang, Y.H., Park, S.R. & Lee, H.J. (2019). Species composition, diversity, and distribution of the genus Ulva along the coast of Jeju Island, Korea based on molecular phylogenetic analysis. PLoS ONE, 14: e0219958.
- Kapraun, D.F. & Flynn, E.H. (1973). Culture studies of Enteromorpha linza (L.) I.Ag. and Vivaria oxysperma (Kutzing) Bliding (Chlorophyceae, Ulvales) from Central America. Phycologia, 12: 145–152.
- Kazi, M.A., Kavale, M.G. & Singh, V.V. (2016). Morphological and molecular characterization of Ulva chaugulii sp. nov., U. lactuca and U. ohnoi (Ulvophyceae, Chlorophyta) from India. Phycologia, 55: 45–54.
- Keesing, J.K., Liu, D.Y., Fearns, P. & Garcia, R. (2011). Inter- and intra-annual patterns of Ulva prolifera green tides in the Yellow Sea during 2007–2009, their origin and relationship to the expansion of coastal seaweed aquaculture in China. Marine Pollution Bulletin, 62: 1169–1182.
- Kessler, R.W., Weiss, A., Kuegler, S., Hermes, C. & Wichard, T. (2018). Macroalgal-bacterial interactions: role of dimethylsulfoniopropionate in microbial gardening by Ulva (Chlorophyta). Molecular Ecology, 27: 1808–1819.
- Kirkendale, L., Saunders, G.W. & Winberg, P. (2013). A molecular survey of Ulva (Chlorophyta) in temperate Australia reveals enhanced levels of cosmopolitanism. Journal of Phycology, 49: 69–81.
- Koeman, R.P.T. & van den Hoek, C. (1981). The taxonomy of Ulva (Chlorophyceae) in the Netherlands. British Phycological Journal, 16: 9–53.
- Kraft, L.G., Kraft, G.T. & Waller, R.F. (2010). Investigations into Southern Australian Ulva (Ulvophyceae, Chlorophyta) taxonomy and molecular phylogeny indicate both cosmopolitanism and endemic cryptic species. Journal of Phycology, 46: 1257–1277.
- Krupnik, N., Paz, G., Douek, J., Lewinsohn, E., Israel, A., Mineur, F. & Maggs, C. (2018). Native, invasive and cryptogenic Ulva species from the Israeli Mediterranean Sea: risk and potential. Mediterranean Marine Science, 19: 132–146.
- Lawton, R.J., Mata, L., Nys, R.D. & Paul, N.A. (2013). Algal bioremediation of waste waters from land-based aquaculture using Ulva: selecting target species and strains. PLoS ONE, 8: e77344.
- Leskinen, E. & Pamilo, P. (1997). Evolution of the ITS sequences of ribosomal DNA in Enteromorpha (Chlorophyceae). Hereditas, 126: 17–23.
- Leskinen, E., Alström-Rapaport, C. & Pamilo, P. (2004). Phylogeographical structure, distribution and genetic variation of the green algae Ulva intestinalis and U. compressa (Chlorophyta) in the Baltic Sea area. Molecular Ecology, 13: 2257–2265.
- Li, H.M., Zhang, C.S., Han, X.R. & Shi, X.Y. (2015). Changes in concentrations of oxygen, dissolved nitrogen, phosphate, and silicate in the southern Yellow Sea, 1980–2012: sources and seaward gradients. Estuarine, Coastal and Shelf Science, 163: 44–55.
- Lin, A.P., Shen, S.D., Wang, J.W. & Yan, B.L. (2008). Reproduction diversity of Enteromorpha prolifera. Journal of Integrative Plant Biology, 50: 622–629.
- Liu, D.Y., Jiang, J.J., Wang, Y., Zhang, Y. & Di, B.P. (2012). Large scale northward expansion of warm water species Skeletonema tropicum (Bacillariophyceae) in China seas. Chinese Journal of Oceanology and Limnology, 30: 519–527.
- Liu, D.Y., Keesing, J.K., He, P.M., Wang, Z.L., Shi, Y.J. & Wang, Y.J. (2013a). The world’s largest macroalgal bloom in the Yellow Sea, China: formation and implications. Estuarine, Coastal and Shelf Science, 129: 2–10.
- Liu, H.Y., Liu, Z.Y., Wang, Y.C., Zhao, Y.S. & Qin, S. (2013b). Molecular diversity of Enteromorpha from the coast of Yantai: a dual-marker assessment. Chinese Journal of Oceanology and Limnology, 31: 1210–1215.
- Loughnane, C.J., McIvor, L.M., Rindi, F., Stengel, D.B. & Guiry, M.D. (2008). Morphology, rbcL phylogeny and distribution of distromatic Ulva (Ulvophyceae, Chlorophyta) in Ireland and southern Britain. Phycologia, 47: 416–429.
- Lyons, D.A., Arvanitidis, C., Blight, A.J., Chatzinikolaou, E., Guy-Haim, T. & Kotta, J. (2014). Macroalgal blooms alter community structure and primary productivity in marine ecosystems. Global Change Biology, 20: 2712–2724.
- Ma, Z.L., Xu, Z.L. & Zhou, J. (2009). Effect of global warming on the distribution of Lucifer intermedius and L. hanseni (Decapoda) in the Changjiang estuary. Progress in Natural Science, 19: 1389–1395.
- Maggs, C.A., Blomster, J., Mineur, F. & Kelly, J. (2007). Ulva. In Green Seaweeds of Britain and Ireland (Brodie, J., Maggs, C.A. & John, D.M., editors), 80–103. Natural History Museum Publications, London.
- Malta, E.J., Draisma, S. & Kamermans, P. (1999). Free-floating Ulva in the southwest Netherlands: species or morphotypes? A morphological, molecular and ecological comparison. European Journal of Phycology, 34: 443–454.
- Manhart, J.R. (1994). Phylogenetic analysis of green plant rbcL sequences. Molecular Phylogenetics and Evolution, 3: 114–127.
- Martins, I., Oliveria, J.M., Findt, M.R. & Marques, J.C. (1999). The effect of salinity on the growth rate of the macroalgae Enteromorpha intestinalis (Chlorophyta) in the Mondego estuary (west Portugal). Acta Oecologica, 20: 259–265.
- Masakiyo, Y. & Shimada, S. (2014). Species diversity of the genus Ulva (Ulvophyceae, Chlorophyta) in Japanese waters, with special reference to Ulva tepida Masakiyo et S. Shimada sp. nov. Bulletin of the National Museum of Nature and Science, Series B, Botany, 40: 1–13.
- Melton, J.T., Collado, V.L. & Lopez, B.J.M. (2016). Molecular identification and nutrient analysis of the green tide species Ulva ohnoi M. Hiraoka & S. Shimada, 2004 (Ulvophyceae, Chlorophyta), a new report and likely nonnative species in the Gulf of Mexico and Atlantic Florida, USA. Aquatic Invasions, 11: 225–237.
- Miladi, R., Manghisi, A., Minicante, S.A., Genovese, G., Abdelkafi, S. & Morabito, M. (2018). A DNA barcoding survey of Ulva (Chlorophyta) in Tunisia and Italy reveals the presence of the overlooked alien U. ohnoi. Cryptogamie Algologie, 39: 85–107.
- Mineur, F., Johnson, M.P. & Maggs, C.A. (2008). Macroalgal introductions by hull fouling on recreational vessels: seaweeds and sailors. Environmental Management, 42: 667–676.
- Ogawa, T., Ohki, K. & Kamiya, M. (2013). Differences of spatial distribution and seasonal succession among Ulva species (Ulvophyceae) across salinity gradients. Phycologia, 52: 637–651.
- O’Kelly, C.J., Kurihara, A., Shipley, T.C. & Sherwood, A.R. (2010). Molecular assessment of Ulva spp. (Ulvophyceae, Chlorophyta) in the Hawaiian islands. Journal of Phycology, 46: 728–735.
- Phillips, J.A. (1988). Field, anatomical and developmental studies on Southern Australian species of Ulva (Ulvaceae, Chlorophyta). Australian Systematic Botany, 1: 411–456.
- Phillips, J.A., Lawton, R.J., Denys, R., Paul, N.A. & Carl, C. (2016). Ulva sapora sp. nov., an abundant tubular species of Ulva (Ulvales) from the tropical Pacific Ocean. Phycologia, 55: 55–64.
- Pirian, K., Piri, K., Sohrabipour, J., Jahromi, T.S. & Blomster, J. (2016). Molecular and morphological characterisation of Ulva chaugulii, U. paschima and U. ohnoi (Ulvophyceae) from the Persian Gulf, Iran. Botanica Marina, 59: 147–158.
- Provasoli, L. & Pintner, I.J. (1980). Bacteria induced polymorphism in an axenic laboratory strain of Ulva lactuca (Chlorophyceae). Journal of Phycology, 16: 196–201.
- Reed, R.H. & Russell, G. (1978). Salinity fluctuations and their influence on “bottle brush” morphogenesis in Enteromorpha intestinalis (L.) Link. British Phycological Journal, 13: 149–153.
- Reed, R.H. & Russell, G. (1979). Adaptation to salinity stress in populations of Enteromorpha intestinalis (L.) Link. Estuarine and Coastal Marine Science, 8: 251–258.
- Saunders, G.W. & Kucera, H. (2010). An evaluation of rbcL, tufA, UPA, LSU and ITS as DNA barcode markers for the marine green macroalgae. Cryptogamie Algologie, 31: 487–528.
- Shimada, S., Yokoyama, N., Arai, S. & Hiraoka, M. (2008). Phylogeography of the genus Ulva (Ulvophyceae, Chlorophyta), with special reference to the Japanese freshwater and brackish taxa. Journal of Applied Phycology, 20: 979–989.
- Smetacek, V. & Zingone, A. (2013). Green and golden seaweed tides on the rise. Nature, 504: 84–88.
- Spalding, H.L, Conklin, K.Y., Smith, C.M., O’Kelly, C.J. & Sherwood, A.R. (2016). New Ulvaceae (Ulvophyceae, Chlorophyta) from mesophotic ecosystems across the Hawaiian Archipelago. Journal of Phycology, 52: 40–53.
- Tamura, K., Stecher, G., Peterson, D., Filipski, A. & Kumar, S. (2013). MEGA6: Molecular Evolutionary Genetics Analysis Version 6.0. Molecular Biology and Evolution, 30: 2725–2729.
- Tan, I.H., Blomster, J., Hansen, G., Leskinen, E., Maggs, C.A., Mann, D.G., Sluimam, H.J. & Stanhope, M.J. (1999). Molecular phylogenetic evidence for a reversible morphogenetic switch controlling the gross morphology of two common genera of green seaweeds, Ulva and Enteromorpha. Molecular Biology and Evolution, 16: 1011–1018.
- Tanner, C.E. (1979). The taxonomy and morphological variation of distromatic Ulvaceous algae (Chlorophyta) from the northeast Pacific. Ph.D. dissertation. University of British Columbia, Vancouver, BC, Canada.
- Tanner, C.E. (1986). Investigations of the taxonomy and morphological variation of Ulva (Chlorophyta): Ulva californica. Phycologia, 25: 510–520.
- Taylor, R., Fletcher, R.L. & Raven, J.A. (2001). Preliminary studies on the growth of selected ‘Green tide’ algae in laboratory culture: effects of irradiance, temperature, salinity and nutrients on growth rate. Botanica Marina, 44: 327–336.
- Teichberg, E., Fox, S.E., Olsen, Y.S., Valiela, I., Martinetto, P., Iribarne, O., Muto, E.Y., Petti, M.A.V., Corbisier, T.N., Sotojimenez, M.F., Paezosuna, F., Castro, P., Freitas, H., Zitelli, A., Cardinaletti, M. & Tagliapietra, D. (2010). Eutrophication and macroalgal blooms in temperate and tropical coastal waters: nutrient enrichment experiments with Ulva spp. Global Change Biology, 16: 2624–2637.
- Thompson, J.D., Gibson, T.J., Plewniak, F., Jeanmougin, F. & Higgins, D.G. (1997). The CLUSTAL_X windows interface: flexible strategies for multiple sequence alignment aided by quality analysis tools. Nucleic Acids Research, 25: 4876–4882.
- Titlyanov, E.A., Titlyanova, T.V., Li, X.B., Kalita, T. & Huang, H. (2015). Recent (2008–2012) seaweed flora of Hainan Island, South China Sea. Marine Biology Research, 11: 540–550.
- Tseng, C.K. (1962). Economic Marine Algae Flora of China. Science Press, Beijing.
- Tseng, C.K. (2009). Seaweeds in Yellow Sea and Bohai Sea of China. Science Press, Beijing.
- Tseng, C.K. & Chang, C.F. (1963). A preliminary analytical study of the Chinese marine algal flora. Oceanologia et Limnologia Sinica, 8: 245–253.
- Tsubaki, S., Wada, Y., Onda, A., Ueda, T., Hiraoka, M. & Fujii, S. (2017). Hydrothermal processing of seaweed biomass. In Hydrothermal Processing in Biorefineries (Ruiz, H.A., Thomsen, H.M. & Tranjano H.L., editors), 443–445. Springer International Publishing AG, Cham.
- Wang, Z.L., Xiao, J., Fan, S.L., Li, Y., Liu, X.Q. & Liu, D.Y. (2015). Who made the world’s largest green tide in China? An integrated study on the initiation and early development of the green tide in Yellow Sea. Limnology and Oceanography, 60: 1105–1117.
- Wei, Q.S., Yao, Q.Z., Wang, B.D., Wang, H.W. & Yu, Z.G. (2015). Long-term variation of nutrients in the southern Yellow Sea. Continental Shelf Research, 111: 184–196.
- Weiss, A., Costa, R. & Wichard, T. (2017). Morphogenesis of Ulva mutabilis (Chlorophyta) induced by Maribacter species (Bacteroidetes, Flavobacteriaceae). Botanica Marina, 60: 197–206.
- Wolf, M.A., Sciuto, K., Andreoli, C. & Moro, I. (2012). Ulva (Chlorophyta, Ulvales) biodiversity in the North Adriatic Sea (Mediterranean, Italy): cryptic species and new introductions. Journal of Phycology, 48: 1510–1521.
- Yabe, T., Ishii, Y., Amano, Y., Koga, T., Hayashi, S., Nohara, S. & Tatsumoto, H. (2009). Green tide formed by free-floating Ulva spp. at Yatsu tidal flat, Japan. Limnology, 10: 239–245.
- Ye, N.H., Zhang, X.W., Mao, Y.Z., Liang, C.W., Xu, D., Zou, J., Zhuang, Z.M. & Wang, Q.Y. (2011). Green tides are overwhelming the coastline of our blue planet: taking the world’s largest example. Ecological Research, 26: 477–485.
- Zhang, J.H., Liu, C.C., Yang, L.L., Gao, S., Ji, X., Huo, Y.Z., Yu, K.F., Xu, R. & He, P.M. (2015). The source of the Ulva blooms in the East China Sea by the combination of morphological, molecular and numerical analysis. Estuarine, Coastal and Shelf Science, 164: 418–424.
- Zhao, J., Jiang, P., Liu, Z.Y., Wei, W., Lin, H.Z., Li, F.C., Wang, J.F. & Qin, S. (2013). The Yellow Sea green tides were dominated by one species, Ulva (Enteromorpha) prolifera, from 2007 to 2011. Chinese Science Bulletin, 58: 2298–2302.
- Zhao, J., Jiang, P., Qin, S., Liu, X.J., Liu, Z.Y., Lin, H.Z., Li, F.C., Chen, H.X. & Wu, C.H. (2015). Genetic analyses of floating Ulva prolifera in the Yellow Sea suggest a unique ecotype. Estuarine, Coastal and Shelf Science, 163: 96–102.
- Zhao, J., Jiang, P., Qiu, R., Ma, Y.Y., Wu, C.H. & Fu, H.H. (2018). The Yellow Sea green tide: a risk of macroalgae invasion. Harmful Algae, 77: 11–17.