ABSTRACT
The reproductive structures, early development and carotenoid composition of Umbraulva kuaweuweu, which is a deep-water ulvacean species recently reported from Hawaii, are reported for the first time using the specimens collected by dredging at ~30 m depth from Ogasawara Islands and Mageshima in southern Japan. Although Umbraulva kuaweuweu had siphonaxanthin as in U. japonica, a characteristic carotenoid of Umbraulva, U. kuaweuweu lacked α-carotene and β-carotene. U. kuaweuweu differed markedly from U. japonica in thallus morphology and reproductive structures: cell shape, presence/absence of inner rhizoidal filaments in the upper parts of thalli, solid/partly saccate thalli, and developmental processes of zoids. Multigene concatenated DNA sequences of chloroplast rbcL and tufA genes, and nuclear 18S, 5.8S, 28S rDNA and rDNA ITS1 and ITS2 regions indicated separation of U. kuaweuweu from the clade comprised of the generitype U. japonica, U. amamiensis, U. dangeardii and U. kaloakulau. In conclusion, based on the distinctive morphology, life history, carotenoid composition and molecular phylogeny, we propose the establishment of a new genus Ryuguphycus gen. nov. to accommodate U. kuaweuweu, as R. kuaweuweu comb. nov.
Introduction
The genus Umbraulva E.H.Bae & I.K.Lee was distinguished from Ulva based on the occurrence of the characteristic carotenoid siphonaxanthin, imparting a yellowish colour to this species, and its sister relationship suggested by molecular phylogeny (Bae & Lee, Citation2001). Three species, the generitype Umbraulva japonica (Holmes) E.H.Bae & I.K.Lee, Umbraulva amamiensis (T.Tanaka) E.H.Bae & I.K.Lee and Umbraulva olivascens (P.J.L.Dangeard) G.Furnari were included within the genus Umbraulva in Bae & Lee (Citation2001). Later, Wynne & Furnari (Citation2014) proposed a new name U. dangeardii M.J.Wynne & G.Furnari for U. olivascens because the type was not designated in Dangeard (Citation1961). In their genetic analyses, the specimens representing U. amamiensis (type locality: Amami-Oshima, Japan) and U. olivascens (type locality: Roscoff, France) were from Seoguimpo, Korea and Ireland respectively, which were somewhat distant from their type localities. Later, two additional species U. kaloakulau H.L.Spalding & A.R.Sherwood and U. kuaweuweu H.L.Spalding & A.R.Sherwood, were described from deep habitats in Hawaii (Spalding et al., Citation2016).
Species of Umbraulva are more common in deep habitats and the carotenoid siphonaxanthin was conjectured to have an important role as an accessory photosynthetic pigment for adaptation to the light quality in deep habitats (Kageyama et al., Citation1977; Yokohama, Citation1981). As to the occurrence of siphonaxanthin in other species of Umbraulva, Bae & Lee (Citation2001) cited Yokohama (Citation1981) for U. amamiensis and U. japonica, and Levavasseur (Citation1989) for U. olivascens. U. kaloakulau and U. kuaweuweu were assigned to Umbraulva based on their close phylogenetic relationships with U. dangeardii, and the dark green olive colour of their thalli suggesting occurrence of siphonaxanthin However, their pigment compositions have not been examined, and the generitype U. japonica was not included in their genetic analyses (Spalding et al., Citation2016).
Recent dredge samples from sea bottoms of ~30–60 m at several localities in southern Japan (Ogasawara Islands, Mageshima and Amami-Oshima) contained ulvoid specimens genetically referable to Umbraulva kuaweuweu and Ulva iliohaha H.L.Spalding & S.R.Sherwood, which have not been reported from the north-western Pacific. We also collected fresh mature specimens of Umbraulva japonica from central Pacific Honshu for morphological and molecular studies. In the present study, we re-examined the morphology, life history and the accessory photosynthetic pigments of U. kuaweuweu, and compared them with and re-examined the taxonomy of the generitype U. japonica.
Materials and methods
Sample collection
For morphological and molecular phylogenetic studies, specimens of Umbraulva kuaweuweu were collected by dredging from the sea bottom at ~60m depth near Ogasawara Islands (27°9.40′N, 142°12.16′E) on 12 July and 17 July 2017, and at ~30 m depth near Mageshima, Kagoshima, Japan (30°41.72′N, 130°51.04′E) on 27 September 2017 (, Supplementary table S1). A specimen referable to Ulva iliohaha by DNA sequence was also collected from Mageshima on 26 May 2018. For comparison, specimens of U. japonica were collected by snorkelling at 4 m depth at Oishizaki, Awajishima, Japan (34°16.23′N, 134°57.16′E) on 9 May 2011, 26 December 2016 and 20 September 2017. The voucher specimen of Ulva olivascens (= Umbraulva dangeardii) collected by F. Magne at Roscoff, France on 26 January 1958 and housed in the herbarium of the Roscoff Marine Biological Station was used for genetic analysis. Silica gel-dried specimens of the newly collected material used for the molecular analyses were deposited in the Kobe University Research Center for Inland Seas (Supplementary table S1). Unialgal cultures of U. kuaweuweu isolated from Ogasawara Islands were housed in the Kobe University Macroalgal Culture Collection (KU-MACC; KU-3336).
Molecular phylogenetic analysis
Field-collected specimens desiccated in silica gel, the unialgal culture (KU-3336) housed in KU-MACC and fragments taken from the voucher specimens were used for DNA extractions (Supplementary table S1). Genomic DNA was extracted using a DNeasy Plant Mini Kit (Qiagen, Hilden, Germany) or GenCheck DNA Extraction Reagent (FASMAC, Atsugi, Japan) following each manufacturer’s instructions. Polymerase chain reaction (PCR) amplifications of the plastid rbcL and tufA genes and nuclear 18S, 5.8S, 28S rDNA and rDNA ITS1 and ITS2 regions were carried out using a KOD FX (Takara Bio, Kusatsu, Japan) and a TaKaRa PCR Thermal Cycler Dice (Takara Shuzo, Otsu, Japan). Primers used for PCR and sequencing are listed in Supplementary table S2. The profile of the PCR followed Kawai et al. (Citation2015) and Kojima et al. (Citation2015). After PEG purification (Lis, Citation1980), PCR products were Sanger sequenced using a CE DTCS Quick Start Kit (Beckman Coulter, Fullerton, CA, USA) and a CEQ8000 DNA analysis system (Beckman Coulter) according to the manufacturer’s instructions, or sequenced by a DNA sequencing service (FASMAC). Published and newly determined sequence data of the Percursaria, Ulva, Ulvaria and Umbraulva specimens were used for molecular phylogenetic analyses (Supplementary table S1). Alignments were performed using MAFFT v.6 (Katoh & Toh, Citation2008) and then manually adjusted. The ambiguous regions of the alignments (rDNA ITS1 and ITS2 regions) were removed. Samples with identical nucleotide sequences were treated as a single operational taxonomic unit (OTU). Percursaria percursa (C.Agardh) Rosenvinge and Ulvaria splendens (Ruprecht) K.L.Vinogradova were selected as the outgroup for the analyses, because these two genera are regarded as closely related to Ulva and Umbraulva (Heesch et al., Citation2009, Ichihara et al., Citation2009). Molecular phylogenetic trees for each data set (data set 1: 20 OTUs, rbcL (1329 bp), tufA (868 bp), 18S rDNA (1693 bp), 28S rDNA (1,149 bp), and ITS1+5.8S+ITS2 rDNA region (512 bp), total 5551 bp; data set 2: 39 OTUs, rbcL gene, 1327 bp) were constructed by Maximum likelihood (ML) and Bayesian (BI) analyses. For ML analysis, rapid bootstrap analysis (10 000 replicates) and search for a best-scoring ML tree (RAxML option ‘-f a’) were performed using RAxML GUI v.1.31 (Silvestro & Michalak, Citation2012). The GTR+G model was implemented with partitioning by each codon position of each protein coding gene (rbcL and tufA) or other gene and region for the analyses. Bayesian analyses were performed using MrBayes v.3.2.2 (Ronquist et al., Citation2012). With the help of the Kakusan4 program (Tanabe, Citation2011), the best-fit evolutionary model for each codon position of each protein coding gene or other gene and region was determined by comparing different evolutionary models via the corrected Bayesian Information Criterion (Schwarz, Citation1978). The Bayesian analyses were initiated with a random starting tree and four chains of Markov chain Monte Carlo iterations run simultaneously for 10 000 000 (data set 1) or 50 000 000 (data set 2) generations, keeping one tree every 100 generations. The first 25% of trees sampled were discarded as burn-in, based on the stationarity of ln L as assessed using Tracer v.1.7 (Rambaut et al., Citation2018). A consensus topology and posterior probability values were calculated from the remaining trees.
Morphological observations and culture experiments
Unialgal cultures of U. kuaweuweu were obtained from a crude culture of the vegetative thallus collected on 12 July 2016 at Ogasawara Islands following Kawai et al. (Citation2005). The culture conditions used were: 5°C SD (short day; 8:16 h Light:Dark), 5°C LD (long day; 16:8 h Light:Dark), 10°C SD, 10°C LD, 15°C SD, 15°C LD, 20°C SD, 20°C LD, 25°C LD and 25°C SD under daylight-type white fluorescent or LED illumination of ~50 µmol photons m−2s−1 using a temperature gradient culture chamber (TG-100AD, TG-200AD, Nippon Medical and Chemical Instruments, Osaka, Japan).
For anatomical observations, cross-sections of thalli were made by hand using a razor blade. Photomicrographs were taken using a VB–7010 Digital Camera (Keyence, Tokyo, Japan) attached to an Axioplan microscope (Zeiss, Oberkochen, Germany).
For epifluorescence microscopy of DNA, thalli were fixed in filtered seawater (FSW) containing 1% glutaraldehyde at room temperature for 1 h, washed with FSW three times, stained in FSW containing 5 µg ml−1 SYTO-13 (Thermo Fisher Scientific, Tokyo, Japan) for 30 min, washed with FSW three times, mounted in Vectashield (Vector Laboratories, Burlingame, CA, USA) and observed with an epifluorescence microscope (BX-51, Olympus, Tokyo, Japan) using NIBA excitation.
For transmission electron microscopy (TEM), thalli were pre-fixed in 0.1 M sodium cacodylate buffer (pH 7.2) containing 2% NaCl and 3% glutaraldehyde for 2 h at 4°C, washed with the buffer containing 2% NaCl, post-fixed in the buffer containing 2% NaCl and 1% osmium tetroxide for 2 h at 4°C, washed with the buffer, dehydrated in acetone, and embedded in Spurr’s resin (Spurr, Citation1969). Thin sections were cut using a UCT ultramicrotome (Leica Microsystems, Wetzlar, Germany) with a diamond knife, stained with uranyl acetate and lead citrate, and observed with a JEM-1400 transmission electron microscope (JEOL, Tokyo, Japan).
Pigment analysis
For pigment analyses, field-collected specimens of U. japonica, cultures of U. kuaweuweu (see above), and field-collected Ulva australis Areschoug (Oishizaki, Awajishima, Japan) were frozen in liquid nitrogen and stored at −75°C. The pigments were extracted with acetone:methanol (7:2, v/v) using an ultrasonicator, followed by evaporation of the solvents. The pigments dissolved in hexane were loaded on a column of DEAE-Toyopearl 650 M (Tosoh, Tokyo, Japan), and carotenoids were eluted with hexane, whereas chlorophylls a and b remained on the column. Carotenoids were identified from their absorption spectra and specific retention times on a HPLC equipped with a µBondapak C18 column (8 × 100 mm, RCM type; Waters, USA), eluted with a liner gradient from methanol:water (9:1, v/v) to 100% methanol for 20 min and then 100% methanol (1.8 ml min−1) (Takaichi et al., Citation2006). Absorbance at 460 nm was analysed with a photodiode-array detector (SPD-M10A, Shimadzu, Kyoto, Japan) attached to the HPLC apparatus.
Results
Molecular phylogenetic analyses
In the molecular phylogeny based on concatenated DNA sequences of chloroplast rbcL and tufA genes, and nuclear 18S, 5.8S, 28S rDNA and rDNA ITS regions (total 5551 bp), specimens of Umbraulva kuaweuweu from Mageshima and Ogasawara, Japan and Hawaii formed a fully supported clade (). U. japonica, U. amamiensis, U. kalaokulau and U. dangeardii formed a clade with low supports (ML: <70%, BI: <0.90). Monophyly of Umbraulva spp. was also supported by high values (ML: 97%, BI: 1.00), and the clade was sister to Ulva spp. The Bayesian consensus tree indicated the same tree topology as the ML tree except for the position of U. dangeardii (data not shown, U. dangeardii was sister to the clade of U. kuaweuweu).
Fig. 2. Maximum likelihood tree based on the DNA sequences of chloroplast rbcL and tufA gene, and nuclear 18S, 5.8S, 28S rDNA and ITS regions (total 5551 bp). Numbers on the branches indicate the bootstrap values (BP, right) and Bayesian posterior probabilities (PP, left). Only the BP (≥70%) and PP (≥0.90) are shown
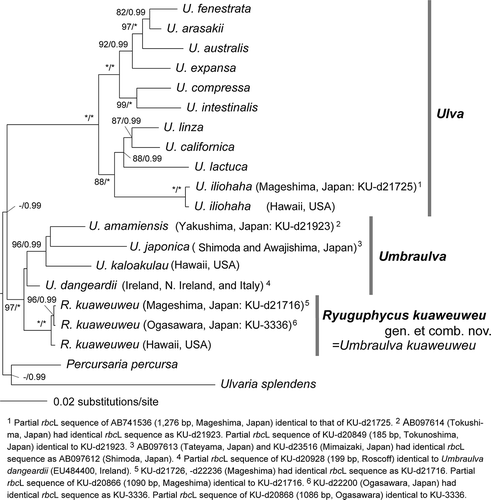
In the molecular phylogeny based on rbcL sequence (1327 bp), Ulva spp. formed a fully supported clade, whereas Umbraulva spp. formed a clade with only low statistical support (). Within the Umbraulva clade, U. japonica, U. amamiensis, U. dangeardii, U. kaloakulau and Umbraulva. sp. from Auckland (New Zealand) formed a clade although the supports were low. U. kuaweuweu from Hawaii, Japan and Kermadec (New Zealand) formed a clade with medium support, and Umbraulva. sp. from Northland (New Zealand) was sister to U. kuaweuweu although the support was low. The rbcL sequence divergences within U. kuaweuweu and between U. kuaweuweu and the other Umbraulva species were 0–0.5% and 1.0–2.4%, respectively. The Bayesian consensus tree indicated a tree topology similar to the ML tree, and two clades of U. kuaweuweu and U. japonica, U. amamiensis, U. dangeardii, U. kaloakulau and Umbraulva. sp. (Auckland) were recognized (data not shown).
Morphology
Umbraulva japonica
Erect thalli are up to ~20 cm high, elliptical to cuneate, attached to rocks by a small discoidal holdfast, dark green olive in colour, ellipsoidal to flabellately expanded, later often split radially (, Supplementary table S3). Inner parts of the thallus near the base are filled with dense rhizoidal filaments, which form a protuberance of the cell walls extending inwards (). Rhizoidal filaments often included green cytoplasmic contents (). In cross section, the thallus is distromatic and solid. Vegetative cells contain a single chloroplast with one or two prominent pyrenoid(s) (). Fine rhizoidal filaments, perhaps issuing from the inner cell wall as in the basal part, are present throughout the thalli in rows from the base to the distal portion (). When mature, cell contents become condensed, giving a diffuse appearance, and by repeated cytoplasmic divisions form zoids (). Cytoplasm and mature zoids are densely packed in the cells ().
Figs 4–14. Morphology of Umbraulva japonica. Fig. 4. Habit of thallus (at ~5 m depth; 7 cm high) at Oishizaki, Awajishima, Japan. Figs 5, 6. Longitudinal section near the base. Note dense rhizoidal filaments (arrow) inside the thallus. Fig. 7. Longitudinal section of upper part of thallus with inner rhizoidal filaments (arrow). Fig. 8. Surface view of thallus showing roundish, quadrate and polygonal cells. Fig. 9. Edge of torn thallus showing fine inner rhizoidal filaments (arrow). Fig. 10. View of interior side of surface cells exposed by tearing the thallus, showing fine rhizoidal filaments running parallel from base to distal parts of thallus (arrows). Fig. 11. Longitudinal section of thallus with different developmental stages of zoidangia. Arrow, premature. Arrowhead, condensation of cytoplasm. Double arrowhead, cytokinesis in developing zoidangia. Figs 12, 13. Developing zoidangia showing different developmental stages. Fig. 14. Mature and emptied zoidangia showing rhizoidal filaments (arrow) and mature zoid (arrowhead)
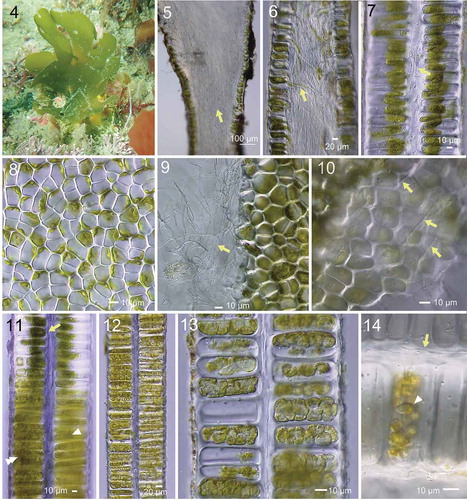
Umbraulva kuaweuweu
Erect thalli of Japanese U. kuaweuweu were foliose, olive-green to olive in colour, flabellately expanded with cuneate lobes and a few perforations (, Supplementary Fig S1, Supplementary table S3). They measured up to 20 cm long and 15 cm wide. Young thalli had a narrow basal part with internal rhizoids (). Thalli had entire margins (). Cells in surface view were quadrate to polygonal and were irregularly arranged (). Thalli were distromatic, and different parts were solid or saccate (). Inner rhizoidal filaments were present only in the basal part of the thallus (). Each cell contained a single parietal chloroplast with a prominent pyrenoid ().
Figs 15–20. Morphology of Ryuguphycus kuaweuweu gen. et comb. nov. in crude culture. Fig. 15. Habit of young thallus with basal terete portion. Fig. 16. Lower terete part of thallus. Fig. 17. Inner rhizoidal filaments near the basal part of thallus (arrowheads). Fig. 18. Surface view of upper part of thallus. Each cell contains a parietal chloroplast with prominent pyrenoid (arrowhead). Fig. 19. Cross section of marginal portion of middle thallus with inner cavity. Fig. 20. Cross section of solid middle thallus
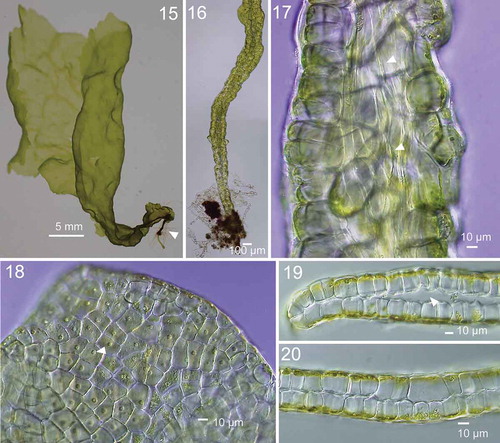
In culture, the thalli did not grow and died at 5°C within a couple of months, grew slowly at 10°C, and grew well at 15–25°C. The thalli remained vegetative at 15°C, but started gametogenesis at 20°C and fully matured within 2–3 weeks and released bi-flagellated zoids (putative gametes). There were no clear differences in the responses between long-day and short-day conditions. The germlings derived from zoids developed into prostrate branched filaments () and developed into parenchymatous, partly hollow upright thalli (). In old cultures, cells of upright thalli tended to become swollen (). When mature, cytoplasm became condensed and formed zoids by repeated cytoplasmic divisions. During zoid maturation, the distal part of the cell protruded to form a release pore (). Condensed cytoplasm and developed zoids were localized in the centre of the cells, and mature zoids started to swim within the cells (). Zoids that failed to be released from the zoidangia germinated in situ and started to develop, forming cell walls (). Emptied zoidangia had a release pore in the middle of the cell surface ().
Figs 21–31. Development of thallus of Ryuguphycus kuaweuweu gen. et comb. nov. in unialgal culture. Figs 21, 22. Germlings. Figs 23, 24. Young biseriate erect thallus. Figs 25, 26. Young parenchymatous erect thalli. Fig. 27. Cross section of upper part of thallus showing hollow portion (arrowheads). Fig. 28. Cross section of mature zoidangia. Arrowheads show developing release pores. Fig. 29. Surface view of mature zoidangia in surface view. Note the remarkable size difference between large (arrowheads) and normal (arrows) zoids. Fig. 30. Mature zoidangia (arrowhead) and in situ germination of zoids (arrow) in surface view. Fig. 31. Surface view showing release pore (arrowheads) of zoidangia
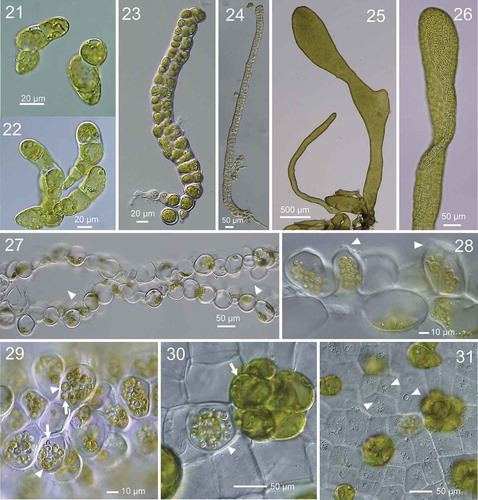
Morphological changes in the nucleus and cytoplasm during zoidangial development were further investigated by epifluorescence microscopy for DNA (). In the vegetative cells, a single parietal chloroplast was located at the cell periphery along the thallus surface, and a nucleus was located at the opposite side of the cell (). During zoidangial development, after an initial series of nuclear divisions, cytoplasm was not completely divided but became branched into several small lobes in which dividing nuclei were frequently observed (), and which finally divided into zoids with a single, condensed nucleus (). Observations of thin sections of mature zoidangia by TEM showed that the zoids with flagella (arrowhead in ), basal bodies (arrowhead in ) and chloroplast with eyespot (arrow in ) were arranged in a centripetal direction, i.e., the cell tip with basal bodies was oriented toward the centre of the zoidangium (). Released zoids were 3.3–4.4 × 1.6–2.2 µm and had two isokont flagella ().
Figs 32–37. Development of zoidangia in Ryuguphycus kuaweuweu gen. et comb. nov. Figs 32–34. DNA epifluorescence microscopy. Figs 32a, 33a and 34a: Brightfield. Figs 32b, 33b and 33b: Epifluorescence. Fig. 32. Cross section of vegetative thallus. Note parietal chloroplasts located in the cell periphery along the thallus surface (Fig. 32a) and nuclei located on opposite sides of the cell (arrowheads in Fig. 32b). Fig. 33. Surface view of thallus during zoidangial development. Note nuclei at late anaphase (arrowhead) in small lobes of cytoplasm. Fig. 34. Surface view of mature zoidangia. Note a number of zoids with condensed nucleus within zoidangium (arrowhead). Figs 35, 36. Transmission electron micrograph of thin section of mature zoidangium. Fig. 35. Zoidangium with zoids with centripetal arrangement. Arrowhead indicates a flagellum of a zoid. Fig. 36. Higher-magnification view of a zoid in mature zoidangium showing a basal body (arrowhead) and an eyespot (arrow). Fig. 37. Light micrograph of released zoids with flagella (arrowheads)
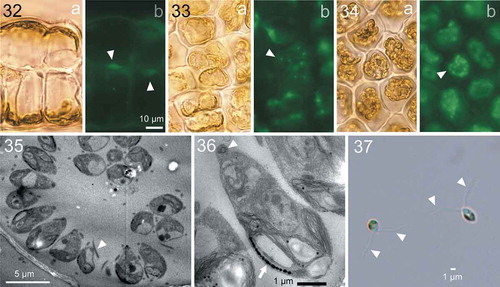
Pigment analysis
HPLC analysis indicated the presence of the major carotenoids neoxanthin, violaxanthin, lutein, α- and β-carotene in Ulva australis; siphonaxanthin, neoxanthin, violaxanthin, α- and β-carotene in Umbraulva japonica; and siphonaxanthin, neoxanthin and violaxanthin in U. kuaweuweu ().
Fig. 38. HPLC chromatographs comparing accessory photosynthetic pigments among Ulva australis, Umbraulva japonica and Ryuguphycus kuaweuweu gen. et comb. nov. (= U. kuaweuweu). Note that the carotenoid siphonaxanthin is present in U. japonica and R. kuaweuweu but absent in Ulva australis. In contrast, R. kuaweuweu lacks α- and β-carotene, which are present in Ulva australis and Umbraulva kuaweuweu
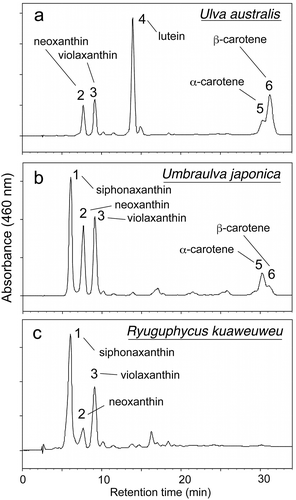
Discussion
Umbraulva japonica and U. kuaweuweu had considerably different morphologies in the vegetative and reproductive structures (, Supplementary table 3). U. japonica had fine inner rhizoidal filaments through the solid thallus, whereas U. kuaweuweu lacked such rhizoidal filaments in the middle to upper part of the thallus, and was partly hollow as in some Ulva species. Perhaps because of the thick cell walls and the inner rhizoidal filaments, Umbraulva japonica has a rather tough thallus which tends to form radial splits along the directions of rhizoidal filaments. In contrast, U. kuaweuweu easily tears at any part of the thallus. Cells of U. kuaweuweu are larger and wider, thin-walled, and the bonding between cells is not as tight as in U. japonica and Ulva spp., resulting in a fragile thallus.
Table 1. Comparison of habitat and selected taxonomic features between Ulva spp., Umbraulva represented by generitype U. japonica, and Ryuguphycus kuaweuweu gen. et comb. nov. (= Umbraulva kuaweuweu)
We presume that Umbraulva kuaweuweu has an isomorphic life history as in Ulva spp. and Umbraulva japonica. As to the reproductive structures, currently we have only confirmed the formation of bi-flagellated zoids in the culture we obtained, which we presume to be a gametophyte. The developmental processes of zoids were considerably different between U. japonica and U. kuaweuweu. In U. japonica, the septum of the first and second cell division in gametogenesis was almost parallel to the cell surface, whereas the direction of the plane was rather irregular in U. kuaweuweu. Mature zoids were tightly packed in U. japonica, but rather loose in U. kuaweuweu and tended to leave empty spaces within the zoidangia, and mature zoids often started to swim within them before release. At least in culture, irregularly large (swollen) zoids were often observed. Often in situ germination of unreleased zoids were observed, which may act as propagules after the breakdown of the thallus. The early development of the thallus is also distinctive in U. kuaweuweu, forming large, irregularly shaped cells.
In U. kuaweuweu, the whole cytoplasm of the zoidangium is not completely divided during the first several nuclear divisions of zoidangial development, resulting in a cytoplasm composed of several small lobes where further nuclear divisions occur. This is unlike the zoid formation in Ulva, where 2- and 4-cell stages of the development are obviously distinguished in both gametogenesis and sporogenesis (Nordby, Citation1974). From the present light microscopy observations, the cytoplasm of the zoidangia of Umbraulva japonica was also divided completely, even in early stages of zoidangial development (), like Ulva. In addition, the spatial arrangement of mature zoids in zoidangia was centripetal in R. kuaweuweu () whereas zoids are irregularly arranged in Ulva ( in Vesty et al., Citation2015) and Umbraulva japonica ( in the present study).
The carotenoid composition of U. kuaweuweu resembled U. japonica in having siphonaxanthin, but was unique in lacking α- and β-carotene, which are present in Ulva spp. and U. japonica (Kageyama et al., Citation1977; Yokohama, Citation1981; Levavasseur, Citation1989).
In the molecular phylogeny, monophyly of U. kuaweuweu from Hawaii (KT932986: Spalding et al., Citation2016), Japan (KU-3336, -d20866, -d20868, -d21716, -d21726, -d22200, -d22236), New Zealand (EF110288: Heesch et al. Citation2009) and Australia (JN029249: Kirkendale et al., Citation2013) was confirmed. Reflecting its broad distributional range, the rbcL sequence divergence was relatively high (up to 0.50% within U. kuaweuweu in this study, up to 0.23% within Ulva fenestrata and up to 0.30% within Ulva lactuca based on the data in Hughey et al., Citation2019). However, Umbraulva kuaweuweu was genetically distinct from the Umbraulva clade comprised of U. amamiensis, U. dangeardii, U. japonica and U. kalaokulau.
In culture, maturation of the thalli was clearly controlled by temperature. In the deep habitat of R. kuaweuweu, water is relatively still and the temperature is rather low and stable. Therefore, it is interesting that the small temperature rise would cause synchronous maturation of the reproductive cells, which may increase the opportunity for mating of gametes in this still habitat. It is unclear how the geographic range of R. kuaweuweu came to extend in the deep habitat for more than several thousand kilometres.
In conclusion, based on the distinctive morphology, life history, carotenoid composition and molecular phylogeny, we propose the establishment of a new genus Ryuguphycus gen. nov. to accommodate U. kuaweuweu, as R. kuaweuweu comb. nov. Further morphological studies are needed for U. amamiensis, U. dangeardii and U. kalaokulau to confirm whether the anatomical feature of U. japonica having fine rhizoidal filaments throughout the thallus is characteristic in the clade.
Description and diagnosis
Ryuguphycus gen. nov. H.Kawai, T.Hanyuda & T.Kitayama
Thallus foliose, distromatic, obovate to cuneate, with perforations, with or without stipe, attached by a holdfast composed of rhizoidal filaments, olive green to olive in colour and slightly lubricous and stiff in texture. Thallus solid or hollow. Inner rhizoidal filaments abundant near the base, but absent in middle to distal portions of thallus. Cells in surface view angular to polygonal, arranged irregularly. Zoidangia transformed from surface cells without forming obvious sori, and zoids not densely packed in mature zoidangia. Siphonaxanthin, neoxanthin and violaxanthin are included as carotenoids. This genus resembles Ulva and Umbraulva but differs from Ulva in having siphonaxanthin and differs from Umbraulva in lacking inner rhizoidal filaments in middle to distal portions of thallus. Nucleotide sequences of chloroplast rbcL and tufA genes, and nuclear 18S, 5.8S, 28S rDNA and rDNA ITS region are also distinctive.
Ryuguphycus kuaweuweu (H.L.Spalding & A.R.Sherwood) H.Kawai, T.Hanyuda & T.Kitayama comb. nov.
Basionym: Umbraulva kuaweuweu H.L.Spalding & A.R.Sherwood. Spalding, H. L., Conklin, K. Y., Smith, C. M., O’Kelly, C. J. & Sherwood, A. R. (Citation2016). Journal of Phycology, 52: p. 45, .
Holotype: BISH 759425. Collected at Penguin Bank, west Molokai, Hawaii, USA on the 28th of November, 2006.
Etymology: The genus name originates from an undersea palace in Japanese folklore, referencing the deep habitat of the taxon.
Author contributions
H. Kawai: original concept, collection of specimens, morphological observations, culture studies, taxonomy, drafting and editing manuscript; T. Hanyuda: molecular phylogeny; I. Mine: DNA fluorescene microscopy and TEM study; S. Takaichi: pigment analysis; R. Terada; collection of specimens; T. Kitayama: collection of specimens.
Supplemental Material
Download TIFF Image (9.1 MB)TEJP-2019-0133-File010.docx
Download MS Word (49.5 KB)Acknowledgements
We are grateful to Dr Eric Henry for critically reading and improving the manuscript, the herbarium of the Roscoff Marine Station for examination of voucher specimen of Ulva olivascens, crew members of T/S Nansei-maru, Faculty of Fisheries, Kagoshima University and Dr Masahiro Suzuki for their help in collecting specimens. Part of the study was supported by a JSPS Grant-in-Aid for Scientific Research (No. 16H04832) to H.K.
Supplementary Information
The following supplementary material is accessible via the Supplementary Content tab on the article’s online page at https://doi.org/10.1080/09670262.2020.1753815
Supplementary table S1. Origin of specimens and sequence data used for molecular phylogenetic analysis, including their database accession numbers.
Supplementary table S2. List of primers used for polymerase chain reaction (PCR) and sequencing.
Supplementary table S3. Comparisons of selected morphological features between Umbraulva spp. and Ryuguphycus kuaweuweu.
Supplementary fig. S1. Voucher of field-collected thalli of Ryuguphycus kuaweuweu gen. et comb. nov. collected on 12 July, 2016 at Ototojima, Ogasawara Islands, Japan. Scale in mm.
References
- Bae, H.B. & Lee, I.K. (2001). Umbraulva, a new genus based on Ulva japonica (Holmes) Papenfuss (Ulvaceae, Chlorophyta). Algae, 16: 217–231.
- Dangeard, P.J.L. (1961). Le problème de l’espèce avec référence au groupe des Ulvacées. Le Botaniste, 44: 21–36.
- Heesch, S., Broom, J.E.S., Neill, K.F., Farr, T.J., Dalen, J.L. & Nelson, W.A. (2009). Ulva, Umbraulva and Gemina: genetic survey of New Zealand taxa reveals diversity and introduced species. European Journal of Phycology, 44: 143–154.
- Hughey, J.R., Maggs, C.A., Mineur, F., Jarvis, C., Miller, K.A., Shabaka, S.H. & Gabrielson, P.W. (2019). Genetic analysis of the Linnaean Ulva lactuca (Ulvales, Chlorophyta) holotype and related type specimens reveals name misapplications, unexpected origins, and new synonymies. Journal of Phycology, 55: 503–508.
- Ichihara, K., Arai, S., Uchimura, M., Fay, E.J., Ebata, H., Hiraoka, M. & Shimada, S. (2009). New species of freshwater Ulva, Ulva limnetica (Ulvales, Ulvophyceae) from the Ryukyu Islands, Japan. Phycological Research, 57: 94–103.
- Kageyama, A., Yokohama, Y., Shimura, S. & Ikawa, T. (1977). An efficient excitation energy transfer from a carotenoid, siphonaxanthin to chlorophyll a observed in a deep water species of chlorophycean seaweed. Plant & Cell Physiology, 18: 477–480.
- Katoh, K. & Toh, H. (2008). Recent developments in the MAFFT multiple sequence alignment program. Briefings in Bioinformatics, 9: 286–298.
- Kawai, H., Motomura, T. & Okuda, K. (2005). Isolation and purification techniques for macroalgae. In Algal Culturing Techniques (Andersen, R.A., editor), 133–143. Elsevier Academic Press, Burlington, MA.
- Kawai, H., Hanyuda, T., Yamagishi, T., Kai, A., Lane, C.E., McDevit, D., Küpper, F.C. & Saunders, G.W. (2015). Reproductive morphology and DNA sequences of the brown alga Platysiphon verticillatus support the new combination Platysiphon glacialis. Journal of Phycology, 51: 910–917.
- Kirkendale, L., Saunders, G.W., & Winberg, P. (2013). A molecular survey of Ulva (Chlorophyta) in temperate Australia reveals enhanced levels of cosmopolitanism. Journal of Phycology, 49: 69–81. doi:10.1111/jpy.2013.49.issue-1
- Kojima, R., Hanyuda, T. & Kawai, H. (2015). Taxonomic re-examination of Japanese Halimeda species using genetic markers, and proposal of a new species Halimeda ryukyuensis (Bryopsidales, Chlorophyta). Phycological Research, 63: 178–188.
- Levavasseur, G. (1989). Analyse comparée des complexes pigment-protéines de chlorophycophytes marines benthiques. Phycologia, 28: 1–14.
- Lis, J.T. (1980). Fractionation of DNA fragments by polyethylene glycol induced precipitation. Methods in Enzymology, 65: 347–353.
- Nordby, Ø. 1974. Light microscopy of meiotic zoosporogenesis and mitotic gametogenesis in Ulva mutabilis Føyn. Journal of Cell Science, 15: 443–445.
- Rambaut, A., Drummond, A.J., Xie, D., Baele, G. & Suchard, M.A. (2018). Posterior summarisation in Bayesian phylogenetics using Tracer 1.7. Systematic Biology, 67: 901–904.
- Ronquist, F., Teslenko, M., van der Mark, P., Ayres, D.L., Darling, A., Hohna, S., Larget, B., Liu, L., Suchard, M.A. & Huelsenbeck, J.P. (2012). MrBayes 3.2: Efficient Bayesian phylogenetic inference and model choice across a large model space. Systematic Biology, 61: 539–542.
- Schwarz, G. (1978). Estimating the dimension of a model. Annals of Statistics, 6: 461–464.
- Silvestro, D. & Michalak, I. (2012). raxmlGUI: a graphical front-end for RAxML. Organisms Diversity & Evolution, 12: 335–337.
- Spalding, H.L., Conklin, K.Y., Smith, C.M., O’Kelly, C.J. & Sherwood, A.R. (2016). New Ulvaceae (Ulvophyceae, Chlorophyta) from mesophotic ecosystems across the Hawaiian Archipelago. Journal of Phycology, 52: 40–53.
- Spurr, A.R. (1969). A low viscosity epoxy resin embedding medium for electron microscopy. Journal of Ultrastructure Research, 26: 31–42.
- Takaichi, S., Mochimaru, M. & Maoka, T. (2006). Presence of free myxol and 4-hydroxymyxol and absence of myxol glycosides in Anabaena variabilis ATCC 29413, and proposal of biosynthetic pathway of carotenoids. Plant & Cell Physiology, 47: 211–216.
- Tanabe, A.S. (2011). Kakusan4 and Aminosan: two programs for comparing nonpartitioned, proportional and separate models for combined molecular phylogenetic analyses of multilocus sequence data. Molecular Ecology Resources, 11: 914–921.
- Vesty, E.F., Kessler, R.W., Wichard, T. and Coates, J.C. (2015). Regulation of gametogenesis and zoosporogenesis in Ulva linza (Chlorophyta): comparison with Ulva mutabilis and potential for laboratory culture. Frontiers in Plant Science B, 15. 10.3389/fpls.2015.00015.
- Wynne, M.J. & Furnari, G. (2014). A census of J.P.L. [sic] Dangeard’s invalid taxa with proposals to resolve the nomenclatural problems of some of them. Nova Hedwigia, 98: 515–517.
- Yokohama, Y. (1981). Green light-absorbing pigments in marine green algae, their ecological and systematic significance. Japanese Journal of Phycology, 29: 209–222. (In Japanese with English summary)