ABSTRACT
In the past, various species of the unicellular algal genus Micrasterias (Zygnematophyceae) have been used for research in the fields of cell biology and physiology. Relatively large cell size, highly differentiated cell shape and a remarkable evolutionary position make Micrasterias interesting, especially for cytomorphogenetic studies. However, within this genus a model organism enabling molecular research has not yet been established. Micrasterias radians var. evoluta (W.B.Turner) Krieger allows easy culturing under axenic conditions and performs its whole life cycle in vitro thus fulfilling two important prerequisites for a model organism. In this work resources allowing transient expression of transgenes were developed. First, axenic lines were obtained by the treatment of zygospores with a cocktail of antibiotics followed by germination and regeneration. In order to allow transgene expression we isolated the 5′ -flanking region of the M. radians var. evoluta Actin1 gene (MrACT1) and fused it to the green fluorescent protein (GFP). A higher promoter activity compared with various heterologous promoters regarding GFP expression was observed. Transient transgene expression was achieved by polyethylene glycol (PEG)-mediated protoplast transformation, yielding a rate of 3.9% transformed cells per surviving protoplasts. Transgene expression was also achieved by particle bombardment of vegetative cells. Employing the established protocol, a Lifeact-GFP fusion protein for labelling F-actin was expressed, allowing visualization of the actin cytoskeleton in M. radians var. evoluta.
Introduction
Since it has been confirmed that algae of the class Zygnematophyceae represent the group most closely related to land plants, these organisms have attracted increasing attention for research in cell biology, developmental biology, physiology and phylogenomics. A deeper understanding of Zygnematophyceae will help in elucidating evolutionary mechanisms in the water-to-land transition (Delwiche & Cooper, Citation2015; Domozych et al., Citation2016). Desmidiaceae represents the most species-rich family among the Zygnematophyceae, comprising unicellular as well as filamentous forms. In phylogenetic trees Desmidiaceae appear as a derived clade (e.g. Gontcharov & Melkonian, Citation2011).
As typical members of the unicellular Desmidiaceae, algae of the genus Micrasterias, which possess an extremely complex and ornamented cellular architecture, have previously been considered as models for studying cell biology and physiology (Meindl, Citation1993; Lutz-Meindl, Citation2016). Vegetative cells of Micrasterias consist of two bilaterally symmetrical semi-cells that are characterized by a central polar lobe and flanking lateral lobes that vary in numbers across species (Škaloud et al., Citation2011). During the process of asexual reproduction, the semi-cells are separated by a septum formed soon after mitotic division of the nucleus. Mitosis is followed by a series of cytomorphogenetic processes in which the daughter semi-cells establish the morphology of the parental semi-cells (Kiermayer, Citation1968; Lacalli, Citation1975; Vannerum et al., Citation2011; Zhou & von Schwartzenberg, Citation2020). This peculiar growth pattern of Micrasterias made it attractive for research on revealing cell shaping and regulation in the process of cytomorphogenesis (Lutz-Meindl, Citation2016).
Algae from the genus Micrasterias, like many Desmidiaceae, predominantly inhabit peatlands at different altitudes and climate zones. This genus has been shown to be adapted to a variety of abiotic stress factors such as UV radiation, drought, varying temperatures, as well as pollutants such as heavy metals (Meindl & Lütz, Citation1996; Affenzeller et al., Citation2009; Volland et al., Citation2014). Understanding the adaptive mechanisms of these freshwater algae that enable them to cope with different stress factors will be helpful for studies of evolutionary processes related to the land plant transition. In this context the establishment of new and robust models becomes more and more important (Rensing, Citation2017), as can be inferred from work on comparative genomics/transcriptomics of charophytes (e.g. Hori et al., Citation2014; Nishiyama et al., Citation2018; Cheng et al., Citation2019; Jiao et al., Citation2020). For the Desmidiaceae, the most species-rich taxonomy group in Charophyta, the lack of suitable models is a limiting factor for this kind of evolutionary research.
In the past, it has been difficult to identify potential model organism candidates that exhibit fast growth under axenic conditions and can be easily genetically manipulated (Zhou & von Schwartzenberg, Citation2020). Despite considerable DNA sequence resources, no high coverage transcriptome and genome dataset has been reported for Micrasterias, only fragmented sequences are accessible for several species. Transgene expression was first achieved for Micrasterias denticulata using the construct pSA405A containing a heterologous promoter from Closterium (Closteriaceae), transferred via particle bombardment, but this yielded relatively low transformation rates (Vannerum et al., Citation2010, Citation2011, Citation2012). Increased transformation efficiencies can be obtained when using homologous promoters as reported for Closterium peracerosum-strigosum-littorale complex (C. psl complex) (Abe et al., Citation2008) and Mougeotia scalaris (Regensdorff et al., Citation2018). Stable transformation of Zygnematophyceae algae was so far reported for C. psl complex (Abe et al., Citation2011; Hirano et al., Citation2015; Kanda et al., Citation2017) and Penium margaritaceum (Sørensen et al., Citation2014). However, versatile genetic transformation protocols which are easily applicable and enable efficient subcellular localization, as well as genomic manipulation, are still lacking for Desmidiaceae.
In this paper we describe a procedure where an axenic culture of Micrasterias radians var. evoluta (MZCH 672), preselected from the Microalgae and Zygnematophyceae Collection Hamburg (MZCH), was established. With this axenic strain, we established a highly efficient transient genetic transformation system via different approaches (PEG mediated protoplast transfection and particle bombardment) utilizing the green fluorescent protein (GFP) as a reporter. The endogenous Actin1 promoter (pMrACT1) was isolated and enabled higher expression efficiency compared with heterologous promoters. We demonstrate that the established protocol is useful for in vivo gene expression and subcellular localization studies. As a proof of concept, we expressed the fluorescent tag Lifeact (Riedl et al., Citation2008; Regensdorff et al., Citation2018) for labelling the cellular actin network. The proposed resources established for M. radians var. evoluta make this organism a candidate model facilitating molecular and cell biological research for Desmidiaceae.
Material and methods
Algal cultures
The algal strain employed in this study was Micrasterias radians var. evoluta (W.B.Turner) Krieger, which was selected from the Microalgae and Zygnematophyceae Collection Hamburg (MZCH, strain No. 518) (von Schwartzenberg et al., Citation2013). The alga was originally collected in 2001 by L. Krienitz from Lake Ol Bolossat, Kenya; the variety ‘evoluta’ was determined by Škaloud et al. (Citation2011). An axenic derivative of strain MZCH 518 is listed under the number 672 in MZCH. Cultures were maintained in 100 ml Erlenmeyer flasks in the modified C-medium (Ichimura, Citation1971; Supplementary table 1) under the standard growth condition (23°C, 30 µmol photons m–2 s–1 with 16 h light: 8 h dark cycle). The working cultures were cultivated in 500 ml Schott flasks with aeration (sterile air ~500 ml min–1) under standard conditions except that the light intensity was increased to 80 µmol photons m–2 s–1. The culture medium was refreshed weekly to ensure rapid growth. For the purposes of cultivation on solid C-medium, 1.5% plant agar (Duchefa, Haarlem, the Netherlands) was supplemented.
Purification of Micrasterias radians var. evoluta
For the purification of M. radians var. evoluta, mature and dry zygospores were suspended in C-medium and treated with serial dilution of two antibiotic mixtures modified from Droop (Citation1967). Mix I contained 10 mg ml–1 ampicillin, 1.6 mg ml–1 streptomycin, 1.6 mg ml–1 cycloheximide (actidione) and 0.8 mg ml–1 chloramphenicol while Mix II contained 16 mg ml–1 ampicillin, 4 mg ml–1 streptomycin and 0.016 mg ml–1 chloramphenicol. The treated zygospores were incubated under standard growth conditions (23°C, 30 µmol photons m–2 s–1 with 16 h light: 8 h dark cycle) until germination and growing up to culture suspensions. The absence of microbial contaminants was tested by cultivation of the culture aliquots on LB-agar for bacteria and PDA-agar for fungi.
Isolation of Actin1 promoter from Micrasterias radians var. evoluta and cloning to GFP expression vector
Due to the lack of sequence data for M. radians var. evoluta, degenerate primers (Act-Fwd 01, Act-Fwd 02, Act-Rv 01 and Act-Rv 02) were designed to clasp the coding region of Actin1 gene (MrACT1) based on an alignment of homologues derived from eight plant species (M. denticulata (DQ987611.1), M. rotata (HG805276.1), Penium margaritaceum (Seq. Nb.: 8436), Mougeotia scalaris (Seq. Nb.: 17221), Spirogyra pratensis (Seq. Nb.: 24,003), Klebsormidium nitens (Seq. Nb.: 21459), Mesostigma viride (Seq. Nb.: 64,879) (Delwiche & Cooper, Citation2015) and Arabidopsis thaliana (U42007.1). Total genomic DNA was isolated from M. radians var. evoluta by the cetyltrimethyl ammonium bromide (CTAB) method (Murray & Thompson, Citation1980) and was used as a template for the following PCRs. PCR was performed with the DreamTaq™ polymerase kit (Thermo Fisher Scientific, Bremen, Germany). The gene-specific TAILB primers were nested reverse primers designed according to the clasped coding sequence of MrACT1 gene and used for isolation of the promoter regions via Thermal Asymmetric Interlaced (TAIL) PCR following the protocol described by Liu & Whittier (Citation1995) and Liu et al. (Citation1995). High-efficiency TAIL (Hi-TAIL) PCR was performed according to Liu & Chen (Citation2007) with the gene-specific TAILC primers, which were nested reverse primers binding to the promoter region isolated via TAIL-PCR. The forward arbitrary primers AD1-4 and LAD1-4 used for both TAIL and Hi-TAIL were the same as the ones used by Liu et al. (Citation1995) and Liu & Chen (Citation2007). The PCR products were checked via agarose gels and afterwards sequenced by commercial Sanger sequencing. Rapid amplification of 5′ cDNA ends (5′-RACE) was performed with a ligation dependent method according to Dallmeier & Neyts (Citation2013). The total RNA was isolated according to Oñate-Sánchez & Vicente-Carbajosa (Citation2008). The cDNA used for RACE-PCR was synthesized by using the RevertAid Reverse Transcriptase (Thermo Scientific) with the specific 5′-phosphorylated primer cDNAsy. Ligation of cDNA was performed with the T4 RNA Ligase (Thermo Scientific). The circularized cDNA was amplified by inverted PCR with the specific forward (RACEFW2) and reverse (TAILB1) primers.
The GFP expression vector pSA405A (Abe et al., Citation2008) served as backbone for the construction of the transformation vector, in which the endogenous MrACT1 promoter was used to drive cGFP expression. The 1158 bp MrACT1 promoter sequence was amplified from the genomic DNA with the Q5 high-fidelity DNA polymerase (New England Biolabs, Frankfurt/Main, Germany) using the primer pair FwProm and RvProm, which contains the restriction enzyme EcoRI and SpeI recognition sites in the 5′ end respectively. After restriction digestion, the PCR product was inserted into the corresponding sites of pSA405A. The sequence information of the primers used for all PCRs is listed in Supplementary table 2. The MrACT1 promoter sequence and the partial coding sequence were submitted to GenBank (MN844879).
Protoplast isolation
Protoplast isolation and transfection of M. radians var. evoluta with the plasmid pAW001 via polyethylene glycol (PEG) was performed using a modified method published for Physcomitrella patens by Schaefer et al. (Citation1990). Protoplasts were generated from M. radians var. evoluta by enzymatic digestion with combination of 1% driselase (Sigma-Aldrich, Munich, Germany) and 1% cellulase (Sigma-Aldrich, Munich, Germany) in the digestion buffer (pH 5.6) containing 0.25 M mannitol, 10 mM CaCl2, 3 mM MgSO4 and 10 mM 2-(N-morpholino)ethanesulfonic acid (MES). After overnight incubation, undigested cells and cell debris in the supernatant were carefully removed by gentle pipetting. The isolated protoplasts were transferred to a sterile round bottom tube (13 ml) and sedimented by centrifugation (100 g, 5 min). The protoplasts were re-suspended in digestion buffer and the washing step was repeated twice. The density of the protoplasts was determined microscopically by a Neubauer haemocytometer.
PEG mediated protoplast transfection of Micrasterias radians var. evoluta
The isolated protoplasts were suspended in 3M medium (0.25 M mannitol, 15 mM MgCl2, 0.1% MES, pH 5.6) and adjusted to a density of 1.0 × 106 protoplasts ml–1. Transfection of M. radians var. evoluta was performed by mixing 200 µl of protoplast suspension with 20 µg plasmid DNA (~1 µg µl–1) and 200 µl PEG solution (40% PEG 4000 and 0.1 M Ca(NO3)2 in 3M medium with 2-fold dilution). The mixture was subsequently incubated at room temperature for different durations followed by heat shock as indicated in the Results section. Afterwards the assay mixture was stepwise diluted by addition of 3M medium to a final volume of 7 ml. For cultivation of the treated protoplasts, the regeneration (REG) buffer (pH 5.8) was prepared from the C-medium supplemented with 0.1% CaCl2, 2.5% glucose and 2.5% mannitol. GFP signal was microscopically observed after protoplast incubation for 2 days (1 day in dark and 1 day with 30 µmol photons m–2 s–1 irradiation under standard growth condition) using a Zeiss Axio Imager A2 (Carl Zeiss Microscopy, Jena, Germany).
Transformation of Micrasterias radians var. evoluta by particle bombardment
Micrasterias radians var. evoluta grown in liquid C-medium under standard conditions for 5–7 days was harvested by centrifugation (200 g, 5 min) and washed with fresh C-medium. Approximately 5 × 104 cells were transferred to the centre of 90 mm diameter Petri dishes containing C-medium with 1.5% agar and spread in an area with a diameter of ~5 cm. The samples were dried until no obvious liquid was visible and subsequently cultivated for 6 days under standard conditions (23°C, 30 µmol photons m–2 s–1 with 16 h light: 8 h dark cycle). The preparation of plasmid DNA coated gold particles was followed according to the protocol described by Regensdorff et al. (Citation2018). The bombardment of the M. radians var. evoluta was performed using the PDS-1000/He Biolistic Particle Delivery System (Bio-Rad Laboratories, Feldkirchen, Germany) according to the manufacturer’s instructions. The following parameters were applied: the pressure capacity of rupture discs was 1100, 1350 or 1550 psi. The distance from the stopping screen to cell targets was 6.0 cm or 9.0 cm. The size of gold particles used in this study was 0.6 µm or 1.0 µm (Bio-Rad). After bombardment, the plates were incubated at 23°C in dim light (15 µmol photons m–2 s–1). After culturing for 3 days, cells were observed for GFP expression with a fluorescence microscope (Zeiss Axio Imager A2, Carl Zeiss Microscopy, Jena, Germany) and/or laser scanning confocal microscope (LSCM, Leica Microsystem, Wetzlar, Germany) equipped with a VisiScope live cell imaging system (Visitron Systems GmbH, Puchheim, Germany).
Results
Preparation of axenic cultures Micrasterias radians var. evoluta
?Axenization of M. radians var. evoluta was achieved by treatment of zygospores with dilution series of two different antibiotics mixtures: Mix I containing ampicillin, streptomycin, actidione and chloramphenicol and Mix II containing ampicillin, streptomycin and chloramphenicol. The zygospores treated with dilution series of Mix II yielded either exclusively dead cells or surviving algae cells with microorganisms still being present. However, an axenic culture was obtained in the treated samples with 1:4 dilution of Mix I, which inactivated the bacteria and fungi but did not affect the viability of zygospores. The axenic status of the obtained strain was confirmed by growth tests on LB and PDA agar plates. The new strain, entered into the MZCH collection under the accession No. 672, was used in all further experiments.
Isolation of the Micrasterias radians var. evoluta Actin1 promoter and construction of GFP expression vector
With the aim to highly express genes of interest in M. radians var. evoluta, the endogenous Actin1 gene (MrACT1) was chosen for promoter isolation. Since no sequence information was available for this Micrasterias species, the MrACT1 promoter was isolated in three steps as follows. Firstly, a 740 bp genomic fragment of the MrACT1 coding region was amplified with degenerate primers (Act-Fwd 02 and Act-Rv 02). Relying on the sequence of the coding region, the Thermal Asymmetric Interlaced (TAIL) PCR was performed to extend the 5’-flanking region by ~550 bp. With the High-efficiency TAIL (Hi-TAIL) PCR, another ~1500 bp were obtained. In total, 1838 bp of genomic sequence upstream and 937 bp downstream from the putative start codon of MrACT1 were identified (; accession no. MN844879). The sequence obtained via the ligation based 5′ RACE-PCR was about 300 bp. It was aligned with the identified genomic sequence of MrACT1 gene (Supplementary fig. 1) and the result revealed that the transcriptional start is located 175 bp upstream of the start codon ( and Supplementary fig. 1). The sequence also contained a 32 bp sequence from the coding region due to the methodology. By comparison of the gDNA and cDNA sequences of the coding region of Actin genes from different organisms (Supplementary figs 1 and 2), one intron was identified in the 5′ UTR (nucleotides −121 to −18) and a further three were localized in the known coding sequence (nucleotides 59 to 195, 363 to 452 and 681 to 770). The putative TATA-Box is positioned 29 bp upstream from the transcriptional start and 204 bp upstream from the translational start. A 1158 bp fragment including the start codon was amplified from the MrACT1 promoter region and was cloned to the EcoRI and SpeI sites of the vector pSA405A (Abe et al., Citation2008) to generate the plasmid pAW001 (), in which the original pCpCAB promoter was thereby substituted by the promoter pMrACT1 transcriptionally fused to cGFP.
Fig 1. Schematic diagram of the partial 5′ region of Micrasterias radians var. evoluta Actin1 gene plus partial coding region as determined after TAIL PCR and 5´RACE (a) and the structure of the pMrACT1:cGFP cassette in the plasmid pAW001 (b). The A-residue in the start codon was defined as position 1. At 204 bp upstream the presumed TATA-box is located. Open boxes with white background represent the coding sequence, dashed boxes represent introns and boxes with grey background represent the 5′ flanking region
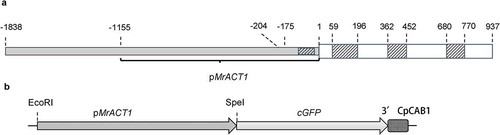
Efficient transient protoplast transformation via PEG mediated transfection using heterologous and endogenous promoters
The supercoiled plasmid pAW001 containing the endogenous pMrACT1 promoter was delivered to the isolated protoplasts of M. radians var. evoluta via PEG mediated transfection. GFP expression could be detected in protoplasts after 2 days of incubation. As shown in , the GFP protein was targeted to the nuclei and cytoplasm. This indicated that the pMrACT1 promoter was functional and can be used for transgene expression in this alga. The transformation efficiency was strongly affected by the incubation conditions during the PEG-mediated DNA delivery (). When the protoplasts were mixed with 20 µg plasmid DNA and incubated at room temperature (RT) for an extended period of 40 min, the transformation efficiency was increased to 1.53%. An incubation period of 50 min leads to a decrease in transformation efficiency due to reduction of cell viability. Heat shock at 42°C following the addition of PEG solution obtained a higher yield of transiently transformed protoplasts. A threefold increase of the transformation efficiency was found using a 5 min heat shock rather than 1 min. Therefore, a 5 min heat shock followed by 25 min of incubation at room temperature was established as a standard protocol for further PEG-mediated transfection of M. radians var. evoluta protoplasts. The survival rate ranged between 78.2% and 85%. Further cultivation of the protoplasts showed that they survived in the regeneration medium for 2 weeks even though the number of cells showing GFP signal decreased. The protoplasts were found to be unable to regenerate the normal M. radians var. evoluta morphology and did not undergo cell division.
Fig 2. Transient GFP expression in protoplasts of Micrasterias radians var. evoluta (a–c) and the effect of different transfection conditions on the transformation efficiency (d). Fluorescent microscopy pictures show GFP fluorescence (a), bright field (b) and merged images (c) of the protoplasts (Zeiss Axio Imager A2). Protoplasts were transformed with the plasmid pAW001 containing endogenous promoter pMrACT1. The DNA amount was 20 µg for each transfection. The volume of protoplast suspension for transfection assay was 200 µl. The survival rates (no. of surviving cells related to the initial no. of protoplasts) were: 85.0% for the 20 RT; 84.0% for 30 RT; 82.5% for 40 RT; 78.2% for 50 RT; 83.9% for 1 HS + 29 RT; 82.6% for 3 HS + 27 RT; 81.6% for 5 HS + 25 RT. Scale bar, 20 µm. The numbers below x-axis (d) designate the duration of the transfection incubations in minutes, RT – room temperature; HS – 42°C heat shock. The transformation efficiency shows mean values with SD from three replicates
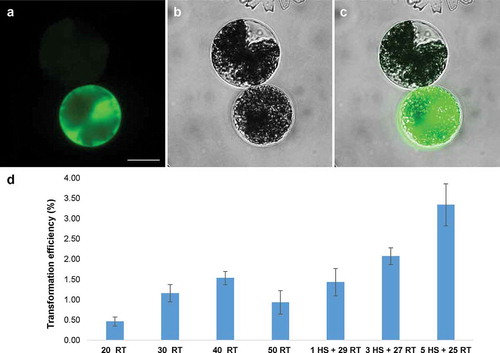
Several heterologous promoters were tested for activity with the established protocol for transient expression in protoplasts of M. radians var. evoluta. The used plasmids contained a GFP reporter driven by various promoters from different organisms such as ubiquitin promoter from Z. mays (pZmUBI) (Christensen & Quail, Citation1996), Actin1 promoter from O. sativa (pOsACT1) (Zeidler et al., Citation1999), pCpCAB promoter from Closterium (Abe et al., Citation2008) and a-tubulin1 gene promoter from M. scalaris (pMsTUA1) (Regensdorff et al., Citation2018). As shown in , the promoters pOsACT1 and pZmUBI gave no GFP signal. Cells showing GFP fluorescence were found in the transformation assays with the plasmids pSA405A carrying pCpCAB and pMD containing pMsTUA1. However, the expression efficiency of the promoter pCpCAB was much lower than that of the promoter pMsTUA1 and the pMrACT1. The activities displayed by these promoters were in the following descending order: pMrACT1 > pMsTUA1 > pCpCAB.
Table 1. GFP expression efficiencies using promoters from different genes and species in Micrasterias radians var. evoluta in per cent of surviving protoplasts. The efficiency shows mean values with SD from 3 replicates
Transformation of Micrasterias radians var. evoluta vegetative cells via particle bombardment
The plasmids pAW001, pMD and pSA405A bearing promoters found active in M. radians var. evoluta were coated to gold particles and successfully delivered to vegetative cells by particle bombardment. The GFP signal was detected after 3 days of cultivation under dim light in cells bombarded with the plasmids pMD and pAW001 (), while no signal was found in the transformation with plasmid pSA405A. As in the comparative assays performed with the PEG mediated protoplast transfection, the promoter activity of pMrACT1 was higher than that of the other two promoters.
Fig 3. GFP expression in vegetative cell of Micrasterias radians var. evoluta cultured for 3 days after particle bombardment with plasmid pAW001. Pictures showing (a) GFP fluorescence (b) cell in bright field, and (c) merged images, taken with the Zeiss Axio Imager A2. Scale bar: 20 µm
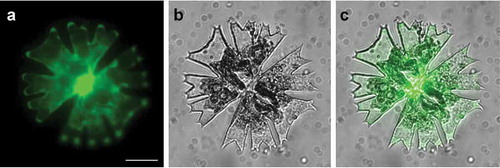
Attempts were made to further determine the optimum conditions for the bombardment of M. radians var. evoluta by variation of parameters such as helium pressure, bombardment distance and gold particle size () using the plasmid pAW001. Comparing the three pressures used, it was found that 1100 psi was sufficient to attain a relatively high number of cells expressing GFP and further increase of pressure (1350 and 1550 psi) did not improve the numbers but caused instead more cell damage. Using a pressure of 1100 psi, a higher number of GFP expressing cells were observed when the sample was placed at the shorter distance of 6 cm from the stopping screen. Utilization of both the 0.6 µm and 1.0 µm gold particles resulted in similar numbers of GFP expressing cells. The bombarded samples were observed in situ every second day. The number of cells showing GFP fluorescence started decreasing after 5 days, which was mainly due to a decrease of cell vitality. When the transformants were transferred to liquid culture, a few of them recovered and still displayed cell division. However, some cells kept a high level of GFP expression for a long period (3 weeks) but were unable to divide.
Table 2. Numbers of GFP expressing cells of Micrasterias radians var. evoluta by particle bombardment with different DNA delivery parameters. Number of transformants was calculated as mean values (± SD, n = 3)
Lifeact tagged GFP was expressed for labelling actin filaments
As a proof of concept, the construct pMD-Lifeact which contains a chimeric gene containing the M. scalaris a-tubulin1 gene (MsTUA1) promoter, driving the expression of the Lifeact actin-binding sequence translationally fused to the cGFP reporter sequence (Regensdorff et al., Citation2018), was introduced into M. radians var. evoluta vegetative cells. Particle bombardment was carried out under the optimum condition determined before. The transformed cells were observed under a confocal laser scanning microscope (CLSM) for actin structures after 3 days of cultivation on the original agar plate. It was confirmed that the Lifeact-cGFP was able to be efficiently expressed and to visualize F-actin cables in M. radians var. evoluta cells (). The Lifeact fluorescence labelled dense filament bundles which seemed to be distributed throughout the cytosol including the tips of the lobes and the isthmus area and branch into a fine meshwork (). In the lobe area, some of the long bundles were aligned along the longitudinal axis ().
Fig 4. Intracellular distribution of actin filaments in a vegetative cell of Micrasterias radians var. evoluta, visualized with Lifeact-cGFP. GFP fluorescence, cell in bright field and merged images of the F-actin in entire cell (a–c) and lobes (d–e) were taken with a confocal laser scanning microscope (CLSM) equipped with a VisiScope live cell imaging system. Scale bar: 10 µm
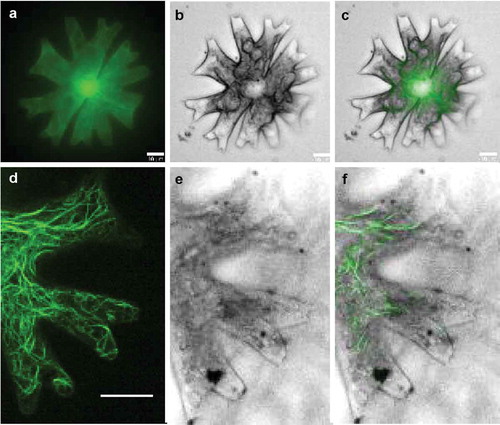
Discussion
Owing to its important phylogenetic position the green algal lineage and unique cell properties, the genus Micrasterias, within the Zygnematophyceae, represents an attractive model for studying aspects of cell biology and physiology (Meindl, Citation1993; Domozych et al., Citation2016; Lutz-Meindl, Citation2016). Micrasterias radians var. evoluta is a candidate model organism for experimental research due to its growth properties and closed life cycle under laboratory conditions (Zhou & von Schwartzenberg, Citation2020). Under our standard growth conditions, the alga showed a growth rate of ~0.062 mg dry weight per day and ml. Although this biomass production is considerably lower than that of the microalga Chlorella sp., which can gain about 10 times more dry weight per day (Watanabe & Saiki, Citation1997), it is largely sufficient for biological experiments.
For avoidance of microbial impacts in physiological and molecular experiments with this algal species, a standard axenic culture had to be established. Thus, a cocktail of antibiotics, which was described for purification of diatoms, flagellates and multicellular chlorophytes (Droop, Citation1967), was utilized to remove microbial contaminants associated with the cell surface of M. radians var. evoluta. A treatment of the culture with antibiotic mixtures failed since vegetative algal cells were susceptible to the antibiotics. However, an axenic culture was obtained by germination of zygospores previously treated with broad spectrum antibiotics ampicillin and chloramphenicol which are effective against prokaryotes, and actidione which is effective against fungi (Schneider-Poetsch et al., Citation2010). The strategy used to obtain an axenic culture of M. radians var. evoluta might be applicable also for other Zygnematophycean species, provided that zygospores are available and able to germinate under laboratory conditions. However, the composition of the antibiotic cocktail might have to be adapted with respect by the nature of the original algal–bacterial/fungi community.
With the standard axenic culture of M. radians var. evoluta, we developed protocols for genetic transformation of both, protoplasts and vegetative cells that could be further used for different experimental purposes. A suitable promoter enabling efficient gene expression in transgenic cells is one of the major requirements for the establishment of a robust transformation system. It was reported that several heterologous promoters were able to drive GFP (and cGFP) expression in M. denticulata (Vannerum et al., Citation2010). However, it seems difficult to exploit this protocol as a routine in molecular studies of Micrasterias due to the low expression efficiency that might result from the incompatibility of the heterologous promoters. Therefore, we isolated 1838 bp sequence upstream from the start codon of MrACT1 gene by two rounds of thermal asymmetric interlaced (TAIL)-PCR. 1158 bp sequence including one intron and the ATG was demonstrated to possess high promoter activity in driving the cGFP expression via PEG mediated protoplasts transformation of M. radians var. evoluta. The promoter and the transformation protocol could be used in future research involving cell-based experiments for gene functional studies, subcellular localization and in vivo protein-protein interactions (Sheen, Citation2001). For PEG-mediated transfection of M. radians var. evoluta protoplasts we determined that heat shock during the co-incubation of the protoplasts with DNA plays an important role in promoting transfection efficiency, which was also reported for Physcomitrella patens (Schaefer, Citation1994) and Elaeis guineensis (Masani et al., Citation2014).
Using the efficient transient expression system, different heterologous promoters were tested for their activities in gene expression in M. radians var. evoluta. The promotor activity was deduced from the relative number of protoplasts displaying visible GFP fluorescence. Promoters suitable for GFP expression usually caused GFP fluorescence of different levels among the transformed protoplasts, presumably due to the different physiological states of the cells.
The pOsACT1 and pZmUBI promoters are both capable of driving strong gene expression in land plants such as rice and Physcomitrella (McElroy et al., Citation1990; von Schwartzenberg et al., Citation2007; Lindner et al., Citation2014). However, transformation of M. radians var. evoluta using these promoters yielded no GFP expressing cells. The promoter pCpCAB1 (Abe et al., Citation2008) from C. psl complex representing an organism from the same order of Desmidiales is active in M. radians var. evoluta protoplasts, while the activity was 100-fold lower than that of the endogenous promoter pMrACT1. The plasmid pMD generated in the laboratory of H. Buschmann (Osnabrück University, Germany) was found to have high capability for driving gene expression in M. radians var. evoluta. Apparently the Mougeotia scalaris MsTUA1 promoter, which showed activity in tobacco leaves as well (Regensdorff et al., Citation2018), can be used in a broad range of organisms. The high expression efficiency achieved by using the pMsTUA1, together with the homologous pMrACT1 are helpful for transgene expression including resistance genes for antibiotic selection in future. Further studies will reveal whether pMrACT1 shows activity also in distantly related algae or seed plants. Despite the relatively high efficiency of 3.88% of transformed protoplasts (related to the number of surviving cells) a crucial limitation of the PEG-mediated transfection is that so far no Desmidiaceae protoplast was successfully regenerated into intact vegetative cells. Presumably factors like the loss of cell polarity in protoplasts make it impossible for the cells to regenerate and undergo cell division. In this respect Desmidiales seem to differ from Zygnematales algae such as Zygnema and Spirogyra where protoplast regeneration has been reported (Ohiwa, Citation1977; Zhou & von Schwartzenberg, Citation2020).
Since protoplast transformation did not allow regeneration and cell division, we applied biolistic transformation using M. radians var. evoluta vegetative cells. It was confirmed that the transformation efficiency of the plasmid pAW001 was higher than that of constructs containing heterologous promoters. For standardization of the biolistic transformation protocol, the influence of a few factors on the transformation efficiency and the recovery of the transformants were investigated by using the parameters employed by Vannerum et al. (Citation2010) as a starting point. Factors like lower acceleration pressures coupled to a short shooting distance were found to improve the transformation rate and recovery of transformants. This might be due to the reduction of stress imposed during the bombardment process enabling cells to survive and continue growth at higher rates.
Using the established transformation platform, the F-actin in M. radians var. evoluta was labelled by expression of the Lifeact tagged cGFP protein. The distribution pattern of the filamentous network was similar to the results published by Meindl et al. (Citation1994) for M. denticulata in which actin bundles were visualized by fluorescently labelled phalloidin. Lifeact allows an efficient and highly specific labelling of F-actin in the cell so that the filaments in the complete cytosol including the lobe tips could be clearly visualized with low background ( and d). Furthermore, unlike the restriction for labelling of fixed samples by usage of phalloidin, the major advantages of in vivo expression of actin-binding tags are that it confers a relatively low toxicity and facilitates live-cell imaging analysis (Riedl et al., Citation2008; Melak et al., Citation2017). Tagging actin with GFP derivatives might help to study actin dynamics and its role in Micrasterias growth and morphogenesis in future. Aside from the actin protein, the subcellular localization of other gene products related to the morphological phenotypes could also be achieved by employing this transient expression system using the GFP fusion protein.
In conclusion, we propose the M. radians var. evoluta as a potential model organism for future molecular studies on Desmidiaceae, as this alga exhibits the presented features such as easy culturing under axenic conditions, the completion of the life cycle and the possibility of transient genetic transformation.
Supplementary information
The following supplementary material is accessible via the Supplementary Content tab on the article’s online page at https://doi.org/10.1080/09670262.2020.1768298
Supplementary table 1: The composition of C-medium used for Micrasterias radians var. evoluta cultivation.
Supplementary table 2: Primers used for amplification of partial coding sequence and 5′ flanking region of Actin1 gene from Micrasterias radians var. evoluta.
Supplementary fig. 1: Alignment of partial of Micrasterias radians var. evoluta Actin1 genomic DNA (gDNA) sequence and nucleotide sequence obtained by the ligation based 5′ RACE-PCR (5′ RACE).
Supplementary fig. 2: Alignment of the partial coding sequences from Actin genes of various organisms and partial MrACT1 gDNA sequence.
Author contributions
H. Zhou: design of the project, genetic transformation of M. radians var. evoluta protoplasts and vegetative cells, writing of manuscript, reviewing and developing the manuscript for submission. A. Wilkens: experiments for purification of the axenic culture, isolation and characterization of the endogenous promoter, genetic transformation of M. radians var. evoluta protoplasts and vegetative cells, reviewing and developing the manuscript for submission. D. Hanelt: reviewing and developing the manuscript for submission. K. von Schwartzenberg: design of the project, reviewing and developing the manuscript for submission.
TEJP-2020-0016-File007.pdf
Download PDF (509.9 KB)TEJP-2020-0016-File006.pdf
Download PDF (349.4 KB)Acknowledgements
The authors thank H. Sekimoto (Japan Women’s University) for kindly sharing the constructs pSA405A and H. Buschmann (Osnabrück University) for gifting plasmid pMD as well as scientific exchange about the bombardment technique. Technical assistance by V. Schwekendiek and S. Körner is gratefully acknowledged.
Disclosure statement
No potential conflict of interest was reported by the authors.
Supplemental Material
The following supplementary material is accessible via the Supplementary Content tab on the article’s online page at https://doi.org/10.1080/09670262.2020.1768298.
Additional information
Funding
References
- Abe, J., Hiwatashi, Y., Ito, M., Hasebe, M. & Sekimoto, H. (2008). Expression of exogenous genes under the control of endogenous HSP70 and CAB promoters in the Closterium peracerosum-strigosum-littorale complex. Plant and Cell Physiology, 49: 625–632.
- Abe, J., Hori, S., Tsuchikane, Y., Kitao, N., Kato, M. & Sekimoto, H. (2011). Stable nuclear transformation of the Closterium peracerosum-strigosum-littorale complex. Plant and Cell Physiology, 52: 1676–1685.
- Affenzeller, M.J., Darehshouri, A., Andosch, A., Lütz, C. & Lütz-Meindl, U. (2009). Salt stress-induced cell death in the unicellular green alga Micrasterias denticulata. Journal of Experimental Botany, 60: 939–954.
- Cheng, S., Xian, W., Fu, Y., Marin, B., Keller, J., Wu, T., Sun, W., Li, X., Xu, Y., Zhang, Y., Wittek, S., Reder, T., Günther, G., Gontcharov, A., Wang, S., Li, L., Liu, X., Wang, J., Yang, H., Xu, X., Delaux, P.M., Melkonian, B., Wong, G.K.-S. & Melkonian, M. (2019). Genomes of subaerial Zygnematophyceae provide insights into land plant evolution. Cell, 179: 1057–1067.
- Christensen, A.H. & Quail, P.H. (1996). Ubiquitin promoter-based vectors for high-level expression of selectable and/or screenable marker genes in monocotyledonous plants. Transgenic Research, 5: 213–218.
- Dallmeier, K. & Neyts, J. (2013). Simple and inexpensive three-step rapid amplification of cDNA 5’ ends using 5’ phosphorylated primers. Analytical Biochemistry, 434: 1–3.
- Delwiche, C.F. & Cooper, E.D. (2015). The evolutionary origin of a terrestrial flora. Current Biology, 25: R899–R910.
- Domozych, D.S., Popper, Z.A. & Sørensen, I. (2016). Charophytes: evolutionary giants and emerging model organisms. Frontiers in Plant Science, 7: 1470.
- Droop, M.R. (1967). A procedure for routine purification of algal cultures with antibiotics. British Phycological Bulletin, 3: 295–297.
- Gontcharov, A.A. & Melkonian, M. (2011). A study of conflict between molecular phylogeny and taxonomy in the Desmidiaceae (Streptophyta, Viridiplantae): analyses of 291 rbcL sequences. Protist, 162: 253–267.
- Hirano, N., Marukawa, Y., Abe, J., Hashiba, S., Ichikawa, M., Tanabe, Y., Ito, M., Nishii, I., Tsuchikane, Y. & Sekimoto, H. (2015). A receptor-like kinase, related to cell wall sensor of higher plants, is required for sexual reproduction in the unicellular charophycean alga, Closterium peracerosum-strigosum-littorale complex. Plant and Cell Physiology, 56: 1456–1462.
- Hori, K., Maruyama, F., Fujisawa, T., Togashi, T., Yamamoto, N., Seo, M., Sato, S., Yamada, T., Mori, H., Tajima, N., Moriyama, T., Ikeuchi, M., Watanabe, M., Wada, H., Kobayashi, K., Saito, M., Masuda, T., Sasaki-Sekimoto, Y., Mashiguchi, K., Awai, K., Shimojima, M., Masuda, S., Iwai, M., Nobusawa, T., Narise, T., Kondo, S., Saito, H., Sato, R., Murakawa, M., Ihara, Y., Oshima-Yamada, Y., Ohtaka, K., Satoh, M., Sonobe, K., Ishii, M., Ohtani, R., Kanamori-Sato, M., Honoki, R., Miyazaki, D., Mochizuki, H., Umetsu, J., Higashi, K., Shibata, D., Kamiya, Y., Sato, N., Nakamura, Y., Tabata, S., Ida, S., Kurokawa, K. & Ohta, H. (2014). Klebsormidium flaccidum genome reveals primary factors for plant terrestrial adaptation. Nature Communications, 5: 3978.
- Ichimura, T. (1971). Sexual cell division and conjugation-papilla formation in sexual reproduction of Closterium strigosum. In Proceedings of the 7th International Seaweed Symposium, 1971, 208–214. University of Tokyo Press.
- Jiao, C., Sørensen, I., Sun, X., Sun, H., Behar, H., Alseekh, S., Philippe, G., Palacio Lopez, K., Sun, L., Reed, R., Jeon, S., Kiyonami, R., Zhang, S., Fernie, A.R., Brumer, H., Domozych, D.S., Fei, Z. & Rose, J.K.C. (2020). The genome of the charophyte alga Penium margaritaceum bears footprints of the evolutionary origins of land plants. Cells. https://doi.org/10.1016/j.cell.2020.04.019.
- Kanda, N., Ichikawa, M., Ono, A., Toyoda, A., Fujiyama, A., Abe, J., Tsuchikane, Y., Nishiyama, T. & Sekimoto, H. (2017). CRISPR/Cas9-based knockouts reveal that CpRLP1 is a negative regulator of the sex pheromone PR-IP in the Closterium peracerosum-strigosum-littorale complex. Scientific Reports, 7: 17873.
- Kiermayer, O. (1968). The distribution of microtubules in differentiating cells of Micrasterias denticulata bréb. Planta, 83: 223–236.
- Lacalli, T. (1975). Morphogenesis in Micrasterias: I. Tip growth. Development, 33: 95–115.
- Lindner, A.C., Lang, D., Seifert, M., Podlesakova, K., Novak, O., Strnad, M., Reski, R. & von Schwartzenberg, K. (2014). Isopentenyltransferase-1 (IPT1) knockout in Physcomitrella together with phylogenetic analyses of IPTs provide insights into evolution of plant cytokinin biosynthesis. Journal of Experimental Botany, 65: 2533–2543.
- Liu, Y.G. & Chen, Y. (2007). High-efficiency thermal asymmetric interlaced PCR for amplification of unknown flanking sequences. Biotechniques, 43: 649–650, 652, 654.
- Liu, Y.G., Mitsukawa, N., Oosumi, T. & Whittier, R.F. (1995). Efficient isolation and mapping of Arabidopsis thaliana T-DNA insert junctions by thermal asymmetric interlaced PCR. The Plant Journal, 8: 457–463.
- Liu, Y.G. & Whittier, R.F. (1995). Thermal asymmetric interlaced PCR: automatable amplification and sequencing of insert end fragments from PI and YAC clones for chromosome walking. Genomics, 25: 674–681.
- Lutz-Meindl, U. (2016). Micrasterias as a model system in plant cell biology. Frontiers in Plant Science, 7: 999.
- Masani, M.Y., Noll, G.A., Parveez, G.K., Sambanthamurthi, R. & Prufer, D. (2014). Efficient transformation of oil palm protoplasts by PEG-mediated transfection and DNA microinjection. PLoS ONE, 9: e96831.
- McElroy, D., Zhang, W., Cao, J. & Wu, R. (1990). lsolation of an efficient actin promoter for use in rice transformation. Plant Cell, 2: 163–171.
- Meindl, U. (1993). Micrasterias cells as a model cystem for research on morphogenesist. Microbiology Reviews, 57: 415–433.
- Meindl, U. & Lütz, C. (1996). Effects of UV irradiation on cell development and ultrastructure of the green alga Micrasterias. Journal of Photochemistry and Photobiology B: Biology, 36: 285–292.
- Meindl, U., Zhang, D., & Hepler, P.K. (1994). Actin microfilaments are associated with the migrating nucleus and the cell cortex in the green alga Micrasterias: studies on living cells. Journal of Cell Science, 107: 1929–1934.
- Melak, M., Plessner, M. & Grosse, R. (2017). Correction: actin visualization at a glance. Journal of Cell Science, 130: 1688.
- Murray, M.G. & Thompson, W.F. (1980). Rapid isolation of high molecular weight plant DNA. Nucleic Acids Research, 8: 4321–4326.
- Nishiyama, T., Sakayama, H., de Vries, J., Buschmann, H., Saint-Marcoux, D., Ullrich, K.K., Haas, F.B., Vanderstraeten, L., Becker, D., Lang, D., Vosolsobe, S., Rombauts, S., Wilhelmsson, P.K.I., Janitza, P., Kern, R., Heyl, A., Rumpler, F., Villalobos, L., Clay, J.M., Skokan, R., Toyoda, A., Suzuki, Y., Kagoshima, H., Schijlen, E., Tajeshwar, N., Catarino, B., Hetherington, A.J., Saltykova, A., Bonnot, C., Breuninger, H., Symeonidi, A., Radhakrishnan, G.V., Van Nieuwerburgh, F., Deforce, D., Chang, C., Karol, K.G., Hedrich, R., Ulvskov, P., Glockner, G., Delwiche, C.F., Petrasek, J., Van de Peer, Y., Friml, J., Beilby, M., Dolan, L., Kohara, Y., Sugano, S., Fujiyama, A., Delaux, P.M., Quint, M., Theissen, G., Hagemann, M., Harholt, J., Dunand, C., Zachgo, S., Langdale, J., Maumus, F., Van Der Straeten, D., Gould, S.B. & Rensing, S.A. (2018). The Chara genome: secondary complexity and implications for plant terrestrialization. Cell, 174: 448–464.
- Ohiwa, T. (1977). Preparation and culture of Spirogyra and Zygnema protoplasts. Cell Structure and Function, 2: 249–255
- Oñate-Sánchez, L. & Vicente-Carbajosa, J. (2008). DNA-free RNA isolation protocols for Arabidopsis thaliana, including seeds and siliques. BMC Research Notes, 1: 93.
- Regensdorff, M., Deckena, M., Stein, M., Borchers, A., Scherer, G., Lammers, M., Hansch, R., Zachgo, S. & Buschmann, H. (2018). Transient genetic transformation of Mougeotia scalaris (Zygnematophyceae) mediated by the endogenous alpha-tubulin1 promoter. Journal of Phycology, 54: 840–849.
- Rensing, S.A. (2017). Why we need more non-seed plant models. New Phytologist, 216: 355–360.
- Riedl, J., Crevenna, A.H., Kessenbrock, K., Yu, J.H., Neukirchen, D., Bista, M., Bradke, F., Jenne, D., Holak, T.A., Werb, Z., Sixt, M. & Wedlich-Soldner, R. (2008). Lifeact: a versatile marker to visualize F-actin. Nature Methods, 5: 605–607.
- Schaefer, D. (1994). Molecular genetic approaches to the biology of the moss Physcomitrella patens. PhD Thesis, University of Lausanne.
- Schaefer, D., Zryd, J.P., Knight, C.D. & Cove, D.J. (1990). Stable transformation of the moss Physcomitrella patens. Molecular and General Genetics MGG, 226: 418–424.
- Schneider-Poetsch, T., Ju, J., Eyler, D.E., Dang, Y., Bhat, S., Merrick, W.C., Green, R., Shen, B. & Liu, J.O. (2010). Inhibition of eukaryotic translation elongation by cycloheximide and lactimidomycin. Nature Chemical Biology, 6: 209–217.
- Sheen, J. (2001). Signal transduction in maize and Arabidopsis mesophyll protoplasts. Plant Physiology, 127: 1466–1475.
- Škaloud, P., Nemjova, K., Vesela, J., Cerna, K. & Neustupa, J. (2011). A multilocus phylogeny of the desmid genus Micrasterias (Streptophyta): evidence for the accelerated rate of morphological evolution in protists. Molecular Phylogenetics and Evolution, 61: 933–943.
- Sørensen, I., Fei, Z., Andreas, A., Willats, W.G., Domozych, D.S. & Rose, J.K. (2014). Stable transformation and reverse genetic analysis of Penium margaritaceum: a platform for studies of charophyte green algae, the immediate ancestors of land plants. The Plant Journal, 77: 339–351.
- Vannerum, K., Abe, J., Sekimoto, H., Inzé, D. & Vyverman, W. (2010). Intracellular localization of an endogenous cellulose synthase of Micrasterias denticulata (Desmidiales, Chlorophyta) by means of transient genetic transformation. Journal of Phycology, 46: 839–845.
- Vannerum, K., De Rycke, R., Pollier, J., Goossens, A., Inze, D. & Vyverman, A.W. (2012). Characterization of a RABE (RAS gene from rat brain e) gtpase expressed during morphogenesis in the unicellular green alga Micrasterias denticulata (Zygnematophyceae, Streptophyta). Journal of Phycology, 48: 682–692.
- Vannerum, K., Huysman, M.J., De Rycke, R., Vuylsteke, M., Leliaert, F., Pollier, J., Lütz-Meindl, U., Gillard, J., De Veylder, L. & Goossens, A. (2011). Transcriptional analysis of cell growth and morphogenesis in the unicellular green alga Micrasterias (Streptophyta), with emphasis on the role of expansin. BMC Plant Biology, 11: 128.
- Volland, S., Bayer, E., Baumgartner, V., Andosch, A., Lütz, C., Sima, E. & Lütz-Meindl, U. (2014). Rescue of heavy metal effects on cell physiology of the algal model system Micrasterias by divalent ions. Journal of Plant Physiology, 171: 154–163.
- von Schwartzenberg, K., Nunez, M.F., Blaschke, H., Dobrev, P.I., Novak, O., Motyka, V. & Strnad, M. (2007). Cytokinins in the bryophyte Physcomitrella patens: analyses of activity, distribution, and cytokinin oxidase/dehydrogenase overexpression reveal the role of extracellular cytokinins. Plant Physiology, 145: 786–800.
- von Schwartzenberg, K., Bornfleth, S., Lindner, A.-C. & Hanelt, D. (2013). The Microalgae and Zygnematophyceae Collection Hamburg (MZCH) – living cultures for research on rare streptophytic algae. Algological Studies, 142: 77–107.
- Watanabe, Y. & Saiki, H. (1997). Development of a photobioreactor incorporating Chlorella sp. for removal of CO2 in stack gas. Energy Conversion and Management, 38: S499–S503.
- Zeidler, M., Hartmann, E. & Hughes, J. (1999). Transgene expression in the moss Ceratodon purpureus. Journal of Plant Physiology, 154: 641–650.
- Zhou, H. & von Schwartzenberg, K. (2020). Zygnematophyceae – from living algae collections to the establishment of future models. Journal of Experimental Botany, 71: 3296–3304, 10.1093/jxb/eraa09.