Abstract
A study related to the development and characterization of a new gene delivery system was performed. The approach consists in both the pre-condensation of plasmid DNA with an arginine-based cationic surfactant, arginine–N-lauroyl amide dihydrochloride (ALA), which was found not to be toxic, and the incorporation of the blood protein transferrin (Tf) into the formulations.Two cationic liposome formulations were used, one composed of a mixture of dioleoyl trimethylammoniopropane and cholesterol (DOTAP:Chol) and the other a pH sensitive formulation constituted of DOTAP, Chol, Dioleoyl phosphatidylethanolamine (DOPE) and cholesteryl hemisuccinate (CHEMS).Particles with different ALA/DNA and cationic lipid/DNA charge ratios were produced and a physicochemical characterization of the systems developed was performed. DNA conformational changes in the presence of ALA were studied by Circular Dichroism (CD) and the ALA binding to DNA was followed by surface tension measurements. Insight into the structure and morphology of the various ALA-complexes (complexes composed of ALA, DNA, Tf and liposomes) was obtained by cryogenic-Transmission Electron Microscopy (cryo-TEM) and the sizes of the ALA-complexes were determined through Photon Correlation Spectroscopy (PCS). We found that the transfection capacity of these systems is directly related with the presence of ALA and the lipidic composition. Complexes based on the pH sensitive liposome formulation present better transfection profiles. The correlation between the inner structure, density and size of the ALA-complexes and their biological activity is discussed. Overall, we demonstrate that the presence of ALA improves the transfection efficiency when conjugated with cationic liposome systems.
Introduction
The success of gene therapy is mainly dependent on the development of gene delivery vectors. Vectors based on virus have been described as good candidates for efficient gene delivery. However, the antigenicity, the possibility of virus recombination, potential oncogenic effects, the difficulty in large scale production, the instability in storage and the high costs associated with vector virus production (Crystal [Citation1995], Nyberg-Hoffman & Aguilar-Cordova [Citation1999], Patil et al. [Citation2005], Verma & Somia [Citation1997]), have instigated the improvement of non-viral gene vectors. Gene delivery systems based on cationic liposomes have become the most applied devices among the non-viral gene delivery systems (Audouy et al. [Citation2000], Mountain [Citation2000], Ulrich [Citation2002]). Among the advantages of these lipoplexes are lack of immunogenicity, safety, ability to package large DNA molecules (Willard, [Citation2000]) and ease of preparation (Lasic & Templeton [Citation1996]). However, despite all efforts to develop efficient non-viral vectors, they still present lower transfection efficiency than the viral vectors, and it becomes important to adopt new strategies where the implementation of novel engineered and non-toxic lipid-like molecules should be considered.
In the current work, we present a quite new approach consisting in combining the use of an amino-acid-based cationic amphiphile, ALA, and the protein transferrin (Tf). The DNA carriers are prepared by making a pre-condensation step of the gene material with the cationic amphiphile ALA and Tf, followed by the complexation with cationic liposomes. The two cationic liposome formulations used were DOTAP:Chol (DC) and DOTAP:CHEMS:DOPE:Chol (CatpH). While DC is a widely studied formulation composed of the biodegradable cationic lipid (DOTAP) and a well tolerated in vivo lipid, cholesterol (Chol), the CatpH formulation presents the specificity of containing a pair of lipids (CHEMS:DOPE) described as conferring pH sensitivity to the liposome (Fattal et al. [Citation2004]).
To the best of our knowledge, ALA is the first cationic amphiphile based on an amino-acid structure used in gene delivery. The pre-condensing agent ALA was found to be non-toxic; it has also been reported as biodegradable (Moran et al. [Citation2001]). Furthermore, the ALA head group is divalent which can be of advantage since it has been reported that multivalent lipids present superior transfection activity than their monovalent counterparts (Byk et al. [Citation1998], Remy et al. [Citation1994]). Beyond the condensation of the plasmid DNA, the use of ALA was thought to facilitate the escape of the gene material from the endosome. Such polar amphiphiles present higher water solubility than most lipids (Lléres et al. [Citation2002], Yoshikawa et al. [Citation2002]), which is thought to destabilize the endosomal membrane and to induce DNA release into the cytoplasm (Pinnaduwage et al. [Citation1989]). Tf has been described as an enhancer of transfection both by increasing vector association with cells (da Cruz et al. [Citation2001]), specially tumor cells that over express their receptors (Wagner et al. [Citation1994]), and as facilitating DNA escape from the endosome through membrane destabilization (Simoes et al. [Citation1999]). In order to take the advantage of these properties, Tf was used to enhance the transfection activity of ALA-associated complexes. The transfection efficiency is found to be highly dependent on the liposome formulation used.
CD and surface tension measurements gave insight into DNA conformational state changes and DNA binding in the presence of ALA, respectively. Cryo-TEM was used to determine the structure/morphology of the ALA-complexes and of the liposomes alone. Particle sizes were measured by PCS.
The inner structure, morphology, size and composition of the ALA-complexes were correlated with their intrinsic biological activity. The final complex size was found to be an important aspect for the efficiency of gene delivery vehicles; we believe that in formulations with high transfection efficiencies only the fraction of smaller complexes is effectively delivering DNA to cells. The study also demonstrated the importance of the lipidic composition for transfection; the pH sensitive pair DOPE:CHEMS is believed to act synergistically with ALA and Tf to help the release of the DNA- complexes from the endosome. Nevertheless, the presence of ALA seems to be the main factor responsible for the excellent transfection results obtained for the ALA-CatpH-complexes.
Materials and methods
The synthesis, chemical structure and physicochemical properties of Arginine–N-lauroyl amide dihydrochloride, ALA, can be found in the literature (Infante et al. [Citation1997]). Dioleoyltrimethylammoniopropane, DOTAP, dioleoylphosphatidylethanolamine, DOPE, cholesterol, Chol, were purchased from Avanti Polar Lipids (Avanti Polar Lipids, Alabaster, AL, US). Cholesteryl hemisuccinate, CHEMS, was purchased from Sigma (St. Louis, MO, US). Stock solutions of all lipids were prepared in chloroform and kept at −20°C until use.
The plasmid expression vector used was: pCMVSPORT- LacZ (kindly provided by Professor Patrick Aebischer, École Polytechnique de Lausanne, Switzerland) – containing the gene coding for Escherichia coli β-gal, under the control of the CMV promoter. pDNA was dissolved in a saline solution (20 mM Hepes buffer, pH 7.4 and 100 mM NaCl) and its concentration was determined spectrophotometrically.
The liposomes were prepared by the ethanol injection method (Campbell, [Citation1995]). Briefly, a lipid film containing DOTAP:Chol at 1:1 mol ratio and DOTAP:CHEMS:DOPE:Chol at 50:14.8:22.2:13 molar ratio was prepared by mixing the lipids and drying them from chloroform solution under nitrogen flow. The dried lipid film was dissolved in ethanol and injected in a saline solution under vortex for 5 minutes; the final concentration of ethanol was 7% v/v. The liposomes were then sonicated for 10 minutes and filter-sterilized utilizing 0.22 µm pore-diameter filters (Schleicher & Schuell BioScience, Germany). The suspension was stored at 4°C until use. The ALA-complexes were obtained by mixing in a sequential manner pDNA, ALA and holo-transferrin (Sigma Chemical, St. Louis, MO); after an incubation time of 15 min the liposomes were added; another incubation period of 15 min followed after which the ALA-complexes were ready to use; all complexes were used immediately after preparation. The ALA-complexes were prepared varying both the ALA/DNA (A/D) charge ratio and the cationic lipid/DNA (L/D) charge ratio; all in moles per charge.
HeLa cells are an immortal cell line derived from human cervical cancer cells and were obtained from American Type Culture Collection, MD. Cells were incubated at 37°C in a humidified atmosphere containing 5% CO2 and maintained in Dulbecco's Modified Eagle's Medium with High Glucose (DMEM-HG) (Sigma) supplemented with 10% heat inactivated fetal calf serum (FCS) (Biochrom, Berlin, Germany), 0.5 g sodium bicarbonate/l, 1.10 g HEPES/l and 100 µg/ml of streptomycin and 100 units/ml of penicillin (Sigma). Cells were propagated by diluting the cell suspension 1/10 every 3–4 days. For use in transfection studies HeLa cells were seeded in 48 multi-well culture dishes (Corning Costar Corp., Cambridge, MA) in a final volume of 1 ml, 1 day before with a cell density of 25×103 cells/well. Cell viability was determined by Trypan blue exclusion. The β-galactosidase activity was determined from 48 h posttransfection by measuring o-nitrophenyl β-D-galactopyranoside (ONPG) cleavage using a photometric assay. After washing with PBS (pH 7.4), cells were lysed by the addition of lysis buffer (0.1% w/v Triton-X, 0.25 M Tris base, pH 8, 70 µl/well) and freeze-thawed once. PBS (pH 7.4, 50 µl) and a solution of ONPG (1.5 mg ml-1, 150 µl) in buffer (sodium dibasic phosphate, 60 mM; magnesium chloride, 1 mM; potassium chloride, 10 mM; β-mercaptoethanol, 50 mM, pH 8) were added to each well. After incubation at 37°C for 45 min, the absorbance at 405 nm was determined in a plate reader and compared to the on-plate β-galactosidase standard curve. The expression of β-galactosidase was normalized with the protein content of the lysates that was quantified by the Sedmak method (Sedmak & Grossberg [Citation1977]), using bovine serum albumin as standard.
CD measurements were performed with a model Jasco-720 spectropolarimeter. Spectra were acquired in a 1 cm path length quartz cuvette at 25°C, with the temperature being maintained with a Peltier device. Three scans were averaged per spectrum, operating from 220–360 nm at a scan speed of 10 nm/min and a bandwidth of 1 nm. Measurements were performed under a constant nitrogen flow, to purge the ozone generated by the light source of the instrument.
The surface tension of the solutions at different ALA to pDNA molar charge ratios (A/D) was measured using a Krüss Tensiometer, model K6, with a metal ring of reference RI 0111, also purchased from Krüss, Germany. We started by measuring the surface tension of a pDNA solution in buffer HBS; in the subsequent measurements aliquots of ALA solution were added to the initial pDNA vessel and mixed with an electromagnetic stirrer prior to surface tension determination.For cryo-TEM imaging of the ALA-complexes vitrified samples were prepared and imaged according to a procedure described elsewhere (Adrian et al. [Citation1984], Bellare et al. [Citation1988], Talmon [Citation1996]). A Philips CM 120 Bio-Twin microscope equipped with a post-column energy filter, using an Oxford CT3500 cryoholder and its workstation, was used. All images were recorded digitally through a CCD camera (Gatan MSC791).
ALA-complexes were characterized with respect to their size using a Coulter N4 Plus (Coulter Corporation, Miami, FL, US). The Coulter N4 Plus is a photon correlation spectroscopy-based technique. The detection angle is fixed at 90°.
Results
Gene delivery efficiency
ALA is an arginine-based cationic surfactant with two positive charges in its head-group and a dodecyl chain. The transfection capacity of the ALA-DC and ALA-CatpH complexes prepared as described above was evaluated and is represented in . Transfection studies were performed for ALA/DNA and ALA/DNA/Tf complexes where the charge ratio between ALA and DNA was varied. No evidence of transfection was detected (data not shown), suggesting that the amphiphile alone, or in conjunction with transferrin, is not an efficient DNA vehicle.
Figure 1. Transfection efficiency of ALA-DC and ALA-CatpH complexes as a function of the ratio A/D (a, c) and L/D (b, d). The cationic lipid to pDNA charge ratio (L/D) was kept constant at 4/1 for a and c, whereas for b and d the ALA to pDNA (A/D)ratio was kept at 10/1.
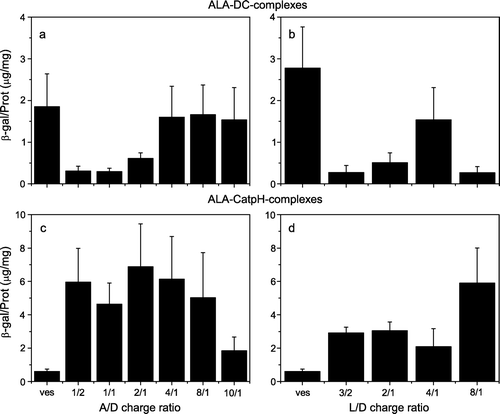
In a and b, the measured transfection capacity of the ALA-based complexes containing DC liposomes is presented. In a the transfection efficiency is presented as a function of the ALA/DNA (A/D) charge ratio while the cationic lipid/DNA (L/D) charge ratio was kept constant at 4/1. An enhancement of transfection efficiency of ALA-DC-complexes is observed as A/D charge ratio increases. However, complexes composed only of DC-liposomes, Tf and pDNA present the same transfection efficiency (first bar, a) as that observed for the best ALA-DC-complexes tested, indicating that, at least with that liposome formulation, ALA is not contributing to the transfection. By choosing an optimal A/D charges ratio and varying the L/D charge ratio (b) a maximum in transfection for a L/D charge ratio of 4 was observed. However, the DC-liposomes, with Tf and pDNA seem to be more efficient than the ALA-DC-complexes (first bar, b). Therefore, when DC liposomes are used there is no advantage for transfection of using the pre-condensation step with ALA.
The transfection results for ALA-CatpH-complexes can be seen in c and d. The first bar of each figure corresponds to the transfection mediated by ternary complexes composed of pDNA, Tf and liposomes. The subsequent bars correspond to the different A/D or L/D ratios. For the tested complexes, the pre-condensation of DNA with ALA improves the transfection efficiency. In c, keeping L/D constant at 4/1, a maximum in transfection is observed for the ratio A/D of 2/1 and a minimum for the ratio of 10/1. In d, keeping A/D constant (2/1), in the highest transfection is observed for the highest L/D ratio (8/1). This gives an indication that for good transfection DNA does not need to have a high amount of compacting agent, prior to its addition to the liposomes. In contrast to the ALA-DC-complexes, the ALA-CatpH-complexes present higher transfection efficiencies compared to the corresponding ternary complexes.
Characterization of the pre-condensing agent
Circular dichroism was used to determine the conformational changes of pDNA in the presence of different concentrations of ALA. In these experiments, we monitored the spectra for different charge ratios between ALA and pDNA (A/D). As can be seen in , for pDNA alone (solid dark line) we have a CD spectrum with the characteristic features of double-stranded B-form DNA, consisting of a positive band at around 275 nm, a negative signal, with approximately the same intensity, at 245 nm, and a maximum in absorbance, at the crossover point, near 257 nm (Guschlbauer et al. [Citation1981], Gray et al. [Citation1992], Spink & Chaires [Citation1997], Braun et al. [Citation2003]). At the A/D charge ratio of 2/1 (dash-dotted dark line), the only change observed in the spectrum, by comparison with pDNA alone, was a decrease in the amplitude of the positive band which becomes more pronounced for the spectra of ratios 4/1 and 8/1. Additionally, for the spectra at the A/D ratios of 4/1 and 8/1 we observe a red shift of the wavelength crossover from 257–259 nm. As more ALA was added, A/D = 10/1 and 16/1, crossover point continues to suffer a pronounced red shift; up to 264 nm; the amplitude of the positive band decreases whereas the amplitude of the negative one increases. Similar CD spectral changes have been seen on in vitro compaction of DNA into phage virus heads (Dorman & Maestre [Citation1973]). Furthermore, when dehydrating DNA in the presence of ethanol up to about 60% (w/w) the same CD spectral features are seen; DNA is believed to be in the B conformation, although in a compacted form (Gray et al. [Citation1992]).
Figure 2. Circular dichroism of plasmid DNA (5 µg/ml) as a function of the ratio between ALA to pDNA (A/D) concentrations. ALA and pDNA concentrations are calculated per charge. pDNA in HBS curve is represented by a solid dark line and corresponds to the B-form of DNA. The following curves correspond to the A/D ratios of: 2/1 (dash-dotted dark line); 4/1 (dotted dark line); 8/1 (solid grey line); 10/1 (dash-dotted grey line); 16/1 (dotted grey line). T = 25°C.
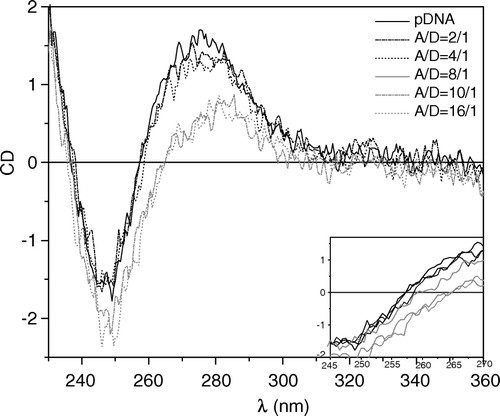
Surface tension (γ) measurements were performed for a pDNA solution in the presence of ALA, see . At low concentrations, we observe a lowering of γ. Near an A/D ratio of 1:2 there is a break in γ due to the onset of association of surfactant to DNA, commonly known as the critical association concentration (CAC), and a plateau is reached. As DNA becomes saturated with surfactant, the surfactant monomer concentration and the activity start to increase again; a lowering of γ is observed until the monomer concentration reaches the critical micellar concentration, CMC, after which γ becomes constant and normal micelles start to form in the bulk phase (Holmberg et al. [Citation2003]). As can be seen from the figure, saturation of pDNA by the micelles is reached slightly above a A/D ratio of 2/1, after which ALA monomers start to go into the bulk solution lowering γ. Above the CMC (A/D∼6/1), ALA micelles start to form and γ keeps constant. These results tell us that at A/D = 2:1, pDNA is saturated with ALA micelles and above this ratio no further binding of ALA molecules to DNA occurs.
Liposome characteristics
The DC and CatpH-liposomes were studied by cryo-TEM (results not shown). Both systems were polydisperse and presented the ability to self-assemble into hexagonal lipidic arrangements, which can be important from a delivery point of view. The mean size of the liposome was determined by PCS. The DC-liposomes presented a mean diameter of 289 nm and the CatpH-liposomes one of 284 nm (data not shown). PCS is a technique which monitors the mean size through a mass-weighted distribution that favors larger sizes (Coldren et al. [Citation2003]). Furthermore, the liposome formulations studied exhibit a broad size distribution, indicating that the use of PCS alone deprives us from getting a complete description of the systems. In an attempt to better characterize these liposome systems, their size distribution was determined by measuring the diameter of a large number of liposomes from cryo-TEM images (). Large liposomes were not taken into account because they were difficult to measure on the cryomicrographs. A population of 826 liposomes was analyzed for the DC-liposome formulation; the number-average radius obtained was 46.2 nm. For the CatpH-liposome formulation a number of 924 liposomes were measured; the number-average radius obtained was 60 nm.
Figure 4. Liposome size distributions obtained from cryo-TEM images taken for the systems: (a) DC and (b) CatpH,. A population of 826 particles was analyzed for the system DC and a population of 924 particles was analyzed for the CatpH system. The later presents a broader size distribution.
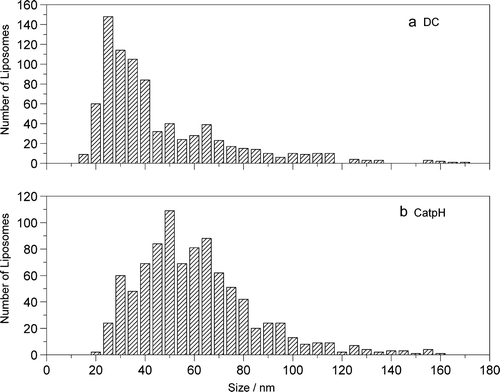
Comparing the two distributions in , it can be inferred that the CatpH-liposome formulation presents a broader size distribution than the DC-liposome formulation, meaning that CatpH-liposomes have a higher degree of polydispersity.
Colloidal aspects of the amino acid-based complexes – size and structure
The efficacy of systems based on cationic liposomes to deliver gene material into target cells has been described to be very dependent on their size (Akao et al. [Citation1991], Almofti et al. [Citation2003], Rakhmanova et al. [Citation2004], Ross & Hui [Citation1999], Staggs et al. [Citation1996]).
In a, the mean sizes of ALA-DC-complexes are presented as a function of the A/D charge ratio; the L/D ratio was kept constant at 4/1. The complexes at the ratio 1/1 are the ones with higher mean size, above 900 nm; for all the other A/D charge ratios, the mean sizes of the lipoplexes vary between 600 and 800 nm. In b, the mean size of the ALA-DC-complexes is presented as a function of the ratio L/D. The mean size of the lipoplexes increases with the L/D ratio. For L/D = 3/2 and 2/1 their mean size is about 300 nm as for the liposomes alone; for L/D = 4/1 they increase significantly in size to values around 700–800 nm; for L/D = 8/1 the lipoplexes become larger, approximately 900 nm.
Figure 5. The mean sizes ALA-DC and ALA-CatpH complexes as a function of the ratio A/D (a, c) and L/D (b, d) as measured by PCS. The cationic lipid to pDNA charge ratio (L/D) was kept at 4/1 for Figures (a) and (c). The ALA to pDNA ratio (A/D) was kept constant at 10/1 for Figures (b) and (d).
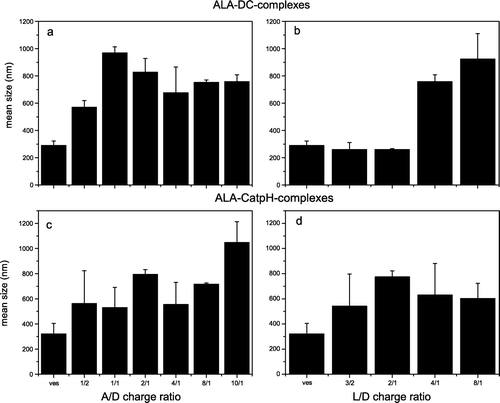
The mean sizes of the ALA-CatpH-complexes are represented in c and d. In c, the mean size of the complexes is illustrated as a function of the A/D ratio. The size varies between 500 and 800 nm with the A/D ratio, ranging from 2/1 to 8/1; the complexes at the ratio 10/1 are the ones with higher mean size, at around 1000 nm; the liposomes present a mean size of 300 nm (first bar in the graph). The mean size of the complexes as a function of the ratio L/D is depicted in d; it varies roughly from 500–800 nm; the larger aggregates are those with L/D = 2/1.
In many studies, the importance of the inner structure of the lipoplexes as well as their colloidal stability has been related with efficiency of transfection. In most cases, these studies concerned particles of simple liposomes and DNA, whereas in our study we are dealing with a more complex system, since we introduced a pre-condensation step with ALA and also a protein as part of the formulation of the ALA-complexes. Since the ALA-CatpH complexes presented higher transfection efficiency than the homologous ALA-DC-complexes, we chose the ones with higher and lower transfection efficiency for structure analysis by cryo-TEM. In this way we expected to see if there were some significant differences which could explain the observed transfection efficiencies. Those with higher expression corresponded to the ratios L/D = 4/1 and A/D = 2/1; the ones with lower expression corresponded to the ratios L/D = 4/1 and A/D = 10/1, as can be seen in c.
The cryomicrographs in correspond to the ALA-DC-complexes. a cryomicrographs were taken to a sample at the L/D ratio of 4/1 and the A/D ratio of 2/1. As can be seen from the images, many liposomes seem to be undisturbed. However, these liposomes seem to be rich in DNA, as inferred from their beam sensitivity (Wasan et al. [Citation1999]). Together with the DNA rich-liposomes we see some denser particles aggregating; no clear inner structure is indicated. The same observations are visualized in b, for the sample at an L/D ratio of 4/1 and an A/D ratio of 10/1. However, in this sample we observe mainly the DNA-rich liposomes; the denser particles (ALA-complexes) are in a smaller number than in the previous sample.
Figure 6. Cryo-TEM micrographs of the ALA-DC complexes at the L/D = 4/1; (a) A/D = 2/1; (b) A/D = 10/1. Unperturbed liposomes coexisting with some of the formed complexes (denser and darker particles) are also seen. T = 25°C.
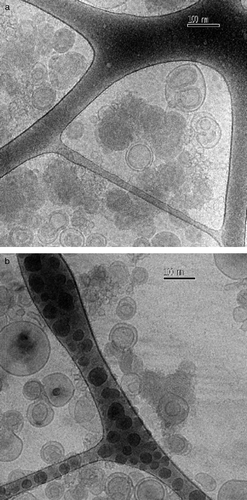
On the other hand, the ALA-CatpH complexes present quite different features. In a and b we have the cryomicrographs of the sample having a L/D ratio of 4/1 and an A/D ratio of 2/1. Only a reduced number of DNA-rich liposomes are observed in the overall images taken. Dense individual particles are characteristic for this sample. In a, a clear lamellar arrangement within the particle imaged is seen; the electron density profile along the straight line drawn is represented as an inset; perfectly arranged and equally separated minima give us a repeat distance of 6.7 nm; a similar lamellar arrangement can be seen for the particle represented in b (black arrow). In a rough estimate, the maximum length of a fully extended alkyl chain in nanometers (nm) is given by the equation lmax=0.15 + 0.127xnc, where nc is the number of carbon atoms in the chain (Evans & Wennerström [Citation1999]). Here we have a mixture of two lipids, DOTAP and DOPE, both having 18 carbons in the alkyl chains; the result for lmax is therefore 2.44 nm. Considering that in the liquid state the membrane thickness is approximately 1.6×lmax, this would give a bilayer thickness of 3.9 nm for the CatpH system. Moreover, a DNA molecule with one hydration shell has a diameter of ca. 2.5 nm; adding it to the bilayer thickness we get a repeat distance of approximately 6.4 nm, which agrees well with the observed value. The observation of an internal organization for this sample can be the basis for the high level of transfection measured. However, it is important to compare this finding with the observed lipoplexes for the sample that transfected the least, the sample at an L/D ratio of 4/1 and an A/D ratio of 10/1 (c). For this sample, no vesicles were seen in the cryomicrographs; only complexes like the ones in c were observed; they seem less dense than in the former sample and no internal arrangement was observed.
Figure 7. Cryo-TEM micrographs of the ALA-CatpH complexes at the L/D = 4/1. (a, b) A/D = 2/1; the electron density of the straight line represented in the image can be seen in the inset of this image; a spacing periodicity of 6.7 nm is shown for the multilamellar structures formed; the arrow in (b) indicates the same kind of striation as found in (a). (c) A/D = 10/1. No liposomes are seen. Some moderately dense particles are visualized. T = 25°C. This figure is reproduced in colour in Molecular Membrane Biology online.
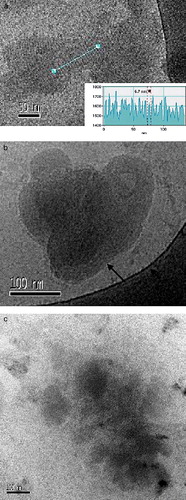
Discussion
The influence of vector size on the delivery of genetic material
One of the most important physicochemical properties of cationic liposome-based gene vectors is their size. It is clear from the literature that lipid-DNA complexes can be very heterogeneous in size (Goncalves et al. [Citation2004], Simberg et al. [Citation2001], Xu et al. [Citation1999]). Furthermore, lipoplex size has been correlated with lipofection efficiency (Almofti et al. [Citation2003], Rakhmanova et al. [Citation2004], Ross & Hui [Citation1999]). The use of larger lipoplexes has been argued beneficial to overcome serum inhibition during the transfection process (Ross & Hui [Citation1999]). However, other researchers argue that smaller lipoplexes are more efficient (Akao et al. [Citation1991]) and not the larger ones as proposed elsewhere (Staggs et al. [Citation1996], Zhang et al. [Citation1997]). In view of these different opinions the size issue was also considered in the present study.
Since all the systems presented here have transferrin in their composition, we believe that the main pathway used by the ALA-complexes to surpass the cell membrane would be endocytosis. Hence, there should be a limit in the size of the complexes that enter the cell; from the literature we know that the cut off size is around 200 nm (Rejman et al. [Citation2004]). Since all systems studied presented a high degree of polydispersity, no clear correlation between the highly transfecting ALA-complexes and 200 nm sized complexes was found. However, a correlation between formulations transfecting poorly and large complexes is observed, both for the ALA-DC-complexes at A/D = 1/1 and L/D = 4/1 and for the ALA-CatpH-complexes at A/D = 10/1 and L/D = 4/1 (). It is intriguing that we do not find any clear correlation between efficiency of transfection and smaller complex size. A possible explanation lies in the size distribution profile of these complexes. With this in mind we propose that only the smaller complexes enter the cell membrane. For instance, the ALA-CatpH-complexes that transfect more (A/D= 2/1 and L/D = 4/1) present a mean size of around 800 nm (c), however, from the cryo-TEM images taken of this formulation we saw many dense complexes with sizes ranging from 100–300 nm (b). Consequently, possibly only the smaller dense complexes visualized would be effective in entering the cell and their DNA vector successfully expressed.
Composition and structure of the complexes in relation to the transfection efficiency
One question that arises from the transfection results is: why are the ALA-CatpH complexes better DNA carriers than the ALA-DC ones? As mentioned above, no large differences can be seen from the images of the two liposome formulations; they are both polydisperse and form hexagonal arrangements at higher concentrations. Hexagonal lipid arrangements have previously been associated with the DNA escape from the endosome in terms of promoting fusion between complexes and the targeted membrane, or a membrane destabilization. Such a phenomenon would allow the release/dissociation of the plasmid DNA and facilitate its entering into the nucleus, and consequently increasing the transfection efficiency (Koltover et al. [Citation1998], Lin et al. [Citation2000], Smisterova et al. [Citation2001], Zuhorn et al. [Citation2005]). Since both liposome systems present this hexagonal arrangement, they would present similar advantages as gene vectors. However, the CatpH-based system proved to be more efficient than the equivalent DC-based system in delivering the genetic material. A possible explanation can be based on the liposome compositions themselves. Thus the fact that the ALA-CatpH-complexes contain the pair DOPE:CHEMS can be of importance in their self-assembly and their interaction with the cells. DOPE is a zwitterionic lipid known to allow a better matching of the charge density between the lipid and DNA (Zuidam & Barenholz [Citation1997]). This has also been related with an easier counterion release from the lipid surface when interacting with DNA (Zuidam et al. [Citation1999b]), and to lowering the hydration of the lipid surface (Zuidam et al. [Citation1999a]). Thus, its presence in the CatpH-based formulation can be responsible for the distribution of ALA and DOTAP within the complex, allowing a higher degree of organization within the ALA-CatpH-complexes. Indeed, a closer look at the size distributions obtained from the cryomicrographs () tells us that the CatpH-liposome system presents a broader size distribution than the DC-liposome one. In a study on the stability of vesicles, where the bending elasticity and the spontaneous curvature of the bilayer were measured by analysis of the vesicle size distribution, it was demonstrated that the more polydisperse the vesicle system, the smaller the bending constant, K, of the bilayer (Coldren et al. [Citation2003]). By comparison with the liposome formulations studied, this gives indication that the CatpH-liposome formulation has a smaller bending constant than the DC-liposomes, suggesting that the CatpH-liposome formulation has a less rigid bilayer, which would contribute to a facile bilayer adjustment, and result in the formation of complexes with a higher degree of organization. Actually, we found evidence of this improved self-assembly for the ALA-CatpH complexes as can be seen from a, where a lamellar inner arrangement with a repeat distance of 6.7 nm was observed. We believe we are facing commonly observed DNA/liposome complexes, where a multilamellar arrangement of lipids with DNA distributed within the lamellar lipid stacks is observed (Mel'nikova et al. [Citation1999], Rädler et al. [Citation1997], Rosa et al. [Citation2007]). Approximate calculations presented in the results section further corroborate the possibility of having a lamellar arrangement, since a repeat distance of 6.7 nm fits well with an eighteen carbon chain bilayer plus the intercalation of DNA molecules with one hydration shell. Another reason for the CatpH-based system to be a better gene carrier than the DC-based one can be the presence of CHEMS in the formulation. CHEMS is a cholesterol-based and pH sensitive molecule which together with DOPE is believed to perturb the endosomal membrane once the pH starts to decrease (Fattal et al. [Citation2004]). This perturbation can lead to the release of the gene carrier contents from the endosome into the cytoplasm and to the subsequent DNA release. In fact such a correlation can be seen in d, where we have the transfection efficiency of the ALA-CatpH-complexes as a function of the L/D ratio. A/D is kept constant at a ratio of 10/1. From this plot we can see that the transfection efficiency increases with the L/D ratio, thus, as a function of the increase of the pair CHEMS:DOPE within the complexes. Moreover, a striking improvement in transfection efficiency is observed for the ALA-CatpH-complexes (c) when comparing with that of the ternary complexes containing only CatpH liposomes, Tf and DNA (c, first bar). As mentioned above, the ALA-CatpH complexes are characterized by a first step where DNA is pre-condensed with ALA and then the protein transferrin and the CatpH liposomes are introduced to the mixture. Since the only difference between the ALA-CatpH complexes and the ternary ones lies in the presence of ALA within the former ones we can safely say that this amino acid-based cationic amphiphile is the main component responsible for this remarkable improvement in transfection efficiency. Thus, we must carefully identify which aspects of ALA that could contribute to these interesting results.
Effects of the pre-condensing agent on transfection
Generally, cationic amphiphiles present some degree of toxicity towards cells and are, therefore, not very efficient gene delivery vehicles. However, this is not the case for ALA, since, as could be seen from c, the introduction of ALA in the ternary complexes greatly improved transfection. Cytotoxicity studies performed for ALA have shown that it is not toxic. The fact that ALA is constituted by an arginine-based head group can be responsible for these results. Another feature of ALA that can explain these observations is the fact that it is a divalent amphiphile. Studies on the transfection efficiency of designed artificial lipid-based systems, where lipid homologue series with different head group valencies were used, have shown that the higher the valency the better is the transfection results; this was explained by, not only the ability to better condense and protect DNA, but also to the quite low cytotoxicity profile of these lipids with higher valencies (Byk et al. [Citation1998], Remy et al. [Citation1994]).
From CD measurements alone, we could conclude that the degree of DNA compaction increases with the ratio between ALA and DNA, A/D. However, surface tension measurements show that effective amphiphile binding to the DNA backbone occurs when an A/D ratio of approximately 2/1 is reached. These results might appear counterintuitive; how can CD measurements tell us that the degree of DNA compaction increases with A/D, and surface tension results tell us that above A/D∼2/1 no further ALA binding to DNA occurs? Surface tension measurements depend on DNA concentration, and we know that for lower concentrations, as is the case of the CD measurements, it is expected that the A/D ratio at which ALA fully binds to DNA increases. Studies of DNA compaction by the presence of different condensing agents have been explored through the use of Fluorescence Microscopy (FM) (Dias et al. [Citation2001], Melnikov et al. [Citation1995a], Yoshikawa et al. [Citation2002]). Generally, it is found that, in the dilute regime, the addition of a condensing agent to DNA results in a coil to globule transition of the DNA molecules, passing through a region of coexistence between coils and globules at intermediate concentrations (Melnikov et al. [Citation1995a], Melnikov et al. [Citation1995b], Ichiba & Yoshikawa [Citation1998], Kidoaki & Yoshikawa [Citation1999], Dias et al. [Citation2001], Yoshikawa [Citation2001], Karlsson et al. [Citation2002], Yoshikawa et al. [Citation2002]). In the case of cationic amphiphiles, the coexistence region was explained by a mechanism of ‘double cooperativity’, where once a cationic amphiphile would bound to a DNA molecule other amphiphiles would cooperatively bind to that DNA molecule resulting in two populations in solution, one of completely folded DNA molecules and the other with unfolded ones (Melnikov et al. [Citation1995a], Melnikov et al. [Citation1995b], Dias et al. [Citation2001]). Thus, in the presence of ALA, once the CAC is reached, two DNA populations exist in solution, the compacted DNA molecules being responsible for the CD results observed.
From a transfection point of view, we can say that the reason why we observe higher transfection efficiency for the ALA-CatpH complexes at an ALA to DNA ratio of 2/1 is probably due to the effective binding of the ALA molecules to the DNA backbone, which contributes to a high degree of protection.
Overall, one can say that the introduction of a pre-condensation step of DNA in liposome-based gene delivery is a viable approach and that the use of a non-toxic engineered divalent amino acid-based amphiphile as a pre-condensing agent is of extreme importance in improving transfection. Nevertheless, the lipidic composition also plays a relevant role; the pair DOPE:CHEMS is believed to act synergistically with ALA and transferrin helping the DNA associated with the complexes to escape from the endosome and consequently, to enhance the transfection in comparison with the complexes composed of DOTAP:Chol liposomes. ALA, however, seems to be the main responsible for the remarkable transfection results obtained for the ALA-CatpH-complexes.
Acknowledgements
We would like to thank Maria Rosa Infante and María del Carmen Morán, CSIC, CID, Barcelona for the synthesis and generous offer of the amino acid-based surfactant, ALA. We are grateful to Gunnel Karlsson for aid in cryo-TEM instrumentation. This work was supported by grants from the Fundação para a Ciência e Tecnologia (FCT, project POCTI SFRH/BD/8357/2002, SFRH/BD/6135/2001, FEDER-POCTI/QUI/58689/2004 and POCTI/QUI/45344/02), the Swedish Research Council (VR), and a grant from an EU Research Training Network, CIPSNAC (contract number: MRTN-CT-2003–504932).
References
- Adrian M, Dubochet J, Lepault J, McDowall AW. Cryo-electron microscopy of viruses. Nature 1984; 308: 32–36
- Akao T, Osaki T, Mitoma J, Ito A, Kunitake T. Correlation between physicochemical characteristics of synthetic cationic amphiphiles and their DNA transfection ability. Bull Chem Soc Japan 1991; 64: 3677–3681
- Almofti MR, Harashima H, Shinohara Y, Almofti A, Li WH, Kiwada H. Lipoplex size determines lipofection efficiency with or without serum. Mol Memb Biol 2003; 20: 35–43
- Audouy S, Molema G, de Leij L, Hoekstra D. Serum as a modulator of lipoplex-mediated gene transfection: dependence of amphiphile, cell type and complex stability. J Gene Med 2000; 2: 465–476
- Bellare JR, Davis HT, Scriven LE, Talmon Y. Controlled environment vitrification system-an improved sample preparation technique. J Electron Microsc Tech 1988; 10: 87–111
- Braun CS, Jas GS, Choosakoonkriang S, Koe GS, Smith JG, Middaugh CR. The structure of DNA within cationic lipid/DNA complexes. Biophys J 2003; 84: 1114–1123
- Byk G, Dubertret C, Escriou V, Frederic M, Jaslin G, Rangara R, Pitard B, Crouzet J, Wils P, Schwartz B, Scherman D. Synthesis, activity, and structure-activity relationship studies of novel cationic lipids for DNA transfer. J Med Chem 1998; 41: 229–235
- Campbell MJ. Lipofection reagents prepared by a simple ethanol injection technique. Biotechniques 1995; 18: 1027–1032
- Coldren B, van Zanten R, Mackel MJ, Zasadzinski JA, Jung HT. From vesicle size distributions to bilayer elasticity via cryo-transmission and freeze-fracture electron microscopy. Langmuir 2003; 19: 5632–5639
- Crystal RG. Transfer of genes to humans: early lessons and obstacles to success. Science 1995; 270: 404–410
- da Cruz MT, Simoes S, Pires PP, Nir S, de Lima MC. Kinetic analysis of the initial steps involved in lipoplex – cell interactions: effect of various factors that influence transfection activity. Biochim Biophys Acta 2001; 1510: 136–151
- Dias RS, Lindman B, Miguel MG. Interactions between DNA and surfactants. Progr Colloid Polymer Sci 2001; 118: 163–167
- Dorman BP, Maestre MF. Experimental differential light-scattering correction to the circular dichroism of bacteriophage T2. PNAS 1973; 70: 255–259
- Evans DF, Wennerström H. The colloidal domain – where physics, chemistry, biology and technology meet. Wiley-VCH, New York 1999
- Fattal E, Couvreur P, Dubernet C. ‘Smart’ delivery of antisense oligonucleotides by anionic pH-sensitive liposomes. Adv Drug Del Rev 2004; 56: 931–946
- Goncalves E, Debs RJ, Heath TD. The effect of liposome size on the final lipid/DNA ratio of cationic lipoplexes. Biophys J 2004; 86: 1554–1563
- Gray DM, Ratliff RL, Vaughan MR. Circular dichroism spectroscopy of DNA. Methods in Enzymology 1992; 211: 389–406
- Guschlbauer, W, Marck, C, Thiele, D. 1981. Circular dichroism: application to the study of polynucleotides and nucleic acids. Struct Asp Biomol:228–254.
- Holmberg, K, Jönsson, B, Kronberg, B, Lindman, B. 2003. Surfactants and polymers in aqueous solution. West Sussex: John Wiley &Sons Ltd.
- Ichiba Y, Yoshikawa K. Single chain observation on collapse transition in giant DNA induced by negatively-charged polymer. Biochem Biophys Res Comm 1998; 242: 441–445
- Infante MR, Pinazo A, Seguer J. Non-conventional surfactants from amino acids and glycolipids: structure, preparation and properties. Colloid Surf A-Physicochem Eng Asp 1997; 123: 49–70
- Karlsson L, van Eijk MCP, Soderman O. Compaction of DNA by gemini surfactants: effects of surfactant architecture. J Colloid Interface Sci 2002; 252(2)290–296
- Kidoaki S, Yoshikawa K. Folding and unfolding of a giant duplex-DNA in a mixed solution with polycations, polyanions and crowding neutral polymers. Biophys Chem 1999; 76: 133–143
- Koltover I, Salditt T, Rädler JO, Safinya CR. An inverted hexagonal phase of cationic liposome-DNA complexes related to DNA release and delivery. Science 1998; 281: 78–81
- Lasic DD, Templeton NS. Liposomes in gene therapy. Adv Drug Deliv Rev 1996; 20: 221–266
- Lin AJ, Slack NL, Ahmad A, Koltover I, George CX, Samuel CE, Safinya CR. Structure and structure-function studies of lipid/plasmid DNA complexes. J Drug Targeting 2000; 8: 13–27
- Lléres D, Clamme J, Dauty E, Blessing T, Krishnamoorthy G, Duportail G, Mély Y. Investigation of the stability of dimeric cationic surfactant/DNA complexes and their interaction with model membrane systems. Langmuir 2002; 18: 10340–10347
- Mel'nikova YS, Mel'nikov SM, Löfroth JE. Physico-chemical aspects of the interaction between DNA and oppositely charged mixed liposomes. Biophys Chem 1999; 81: 125–141
- Melnikov SM, Sergeyev VG, Yoshikawa K. Discrete coil-globule transition of large DNA induced by cationic surfactant. J Am Chem Soc 1995a; 117: 2401–2408
- Melnikov SM, Sergeyev VG, Yoshikawa K. Transition of double-stranded DNA chains between random coil and compact globule states induced by cooperative binding of cationic surfactant. J Am Chem Soc 1995b; 117: 9951–9956
- Moran C, Clapes P, Comelles F, Garcia T, Perez L, Vinardell P, Mitjans M, Infante MR. Chemical structure/property relationship in single-chain arginine surfactants. Langmuir 2001; 17: 5071–5075
- Mountain A. Gene therapy: the first decade. Trends Biotechnol 2000; 18: 119–128
- Nyberg-Hoffman C, Aguilar-Cordova E. Instability of adenoviral vectors during transport and its implication for clinical studies. Nat Med 1999; 5: 955–957
- Patil SD, Rhodes DG, Burgess DJ. DNA-based therapeutics and DNA delivery systems: a comprehensive review. AAPS J 2005; 7: E61–77
- Pinnaduwage P, Schmitt L, Huang L. Use of a quaternary ammonium detergent in liposome mediated DNA transfection of mouse L-cells. Biochim Biophys Acta 1989; 985: 33–37
- Rädler JO, Koltover I, Salditt T, Safinya CR. Structure of DNA-cationic liposome complexes: DNA intercalation in multilamellar membranes in distinct interhelical packing regimes. Science 1997; 275: 810–814
- Rakhmanova VA, Pozharski EV, MacDonald RC. Mechanisms of lipoplex formation: dependence of the biological properties of transfection complexes on formulation procedures. J Memb Biol 2004; 200: 35–45
- Rejman J, Oberle V, Hoekstra D. Size-dependent internalization of particles via the pathways of clathrin- an caveolae-mediated endocytosis. Biochem J 2004; 377: 159–169
- Remy JS, Sirlin C, Vierling P, Behr JP. Gene-transfer with a series of lipophilic DNA-binding molecules. Bioconjugate Chem 1994; 5: 647–654
- Rosa M, Morán MC, Miguel MG, Lindman B. The association of DNA and stable catanionic amino acid-based vesicles. Colloid Surf A: Physicochem Eng Asp 2007; 301: 361–375
- Ross PC, Hui SW. Lipoplex size is a major determinant of in vitro lipofection efficiency. Gene Therapy 1999; 6: 651–659
- Sedmak JJ, Grossberg SE. A rapid, sensitive, and versatile assay for protein using Coomassie brilliant blue G250. Anal Biochem 1977; 79: 544–552
- Simberg D, Danino D, Talmon Y, Minsky A, Ferrari ME, Wheeler CJ, Barenholz Y. Phase behavior, DNA ordering, and size instability of cationic lipoplexes – relevance to optimal transfection activity. J Biol Chem 2001; 276: 47453–47459
- Simoes S, Slepushkin V, Pretzer E, Dazin P, Gaspar R, de Lima MCP, Duzgunes N. Transfection of human macrophages by lipoplexes via the combined use of transferrin and pH-sensitive peptides. J Leukocyte Biol 1999; 65: 270–279
- Smisterova J, Wagenaar A, Stuart MCA, Polushkin E, ten Brinke G, Hulst R, Engberts J, Hoekstra D. Molecular shape of the cationic lipid controls the structure of cationic lipid/dioleylphosphatidylethanolamine-DNA complexes and the efficiency of gene delivery. J Biol Chem 2001; 276: 47615–47622
- Spink CH, Chaires JB. Thermodynamics of the binding of a cationic lipid to DNA. J Am Chem Soc 1997; 119: 10920–10928
- Staggs DR, Burton DW, Deftos LJ. Importance of liposome complexing volume in transfection optimization. Biotechniques 1996; 21: 792–798
- Talmon Y. Transmission electron microscopy of complex fluids: the state of the art. Berichte Der Bunsen-Gesellschaft-Phys Chem Chem Phys 1996; 100: 364–372
- Ulrich AS. Biophysical aspects of using liposomes as delivery vehicles. Biosci Rep 2002; 22: 129–150
- Verma IM, Somia N. Gene therapy -promises, problems and prospects. Nature 1997; 389: 239–242
- Wagner E, Curiel D, Cotten M. Delivery of drugs, proteins and genes into cells using transferrin as a ligand for receptormediated endocytosis. Adv Drug Deliv Rev 1994; 14: 113–136
- Wasan EK, Harvie P, Edwards K, Karlsson G, Bally MB. A multi-step lipid mixing assay to model structural changes in cationic lipoplexes used for in vitro transfection. Biochim Biophys Acta-Biomembr 1999; 1461: 27–46
- Willard HF. Genomics and gene therapy: artificial chromosomes coming to life. Science 2000; 290: 1308–1309
- Xu YH, Hui SW, Frederik P, Szoka FC. Physicochemical characterization and purification of cationic lipoplexes. Biophys J 1999; 77: 341–353
- Yoshikawa K. Controlling the higher-order structure of giant DNA molecules. Adv Drug Deliv Rev 2001; 52: 235–244
- Yoshikawa K, Yoshikawa Y, Kanbe T. All-or-none folding transition in giant mammalian DNA. Chem Phys Lett 2002; 354: 354–359
- Zhang YP, Reimer DL, Zhang GY, Lee PH, Bally MB. Self-assembling DNA-lipid particles for gene transfer. Pharm Res 1997; 14: 190–196
- Zuhorn IS, Bakowsky U, Polushkin E, Visser WH, Stuart MC, Engberts JB, Hoekstra D. Nonbilayer phase of lipoplex-membrane mixture determines endosomal escape of genetic cargo and transfection efficiency. Mol Ther 2005; 11: 801–810
- Zuidam NJ, Barenholz Y. Electrostatic parameters of cationic liposomes commonly used for gene delivery as determined by 4-heptadecyl-7-hydroxycoumarin. Biochim Biophys Acta-Biomembr 1997; 1329: 211–222
- Zuidam NJ, Barenholz Y, Minsky A. Chiral DNA packaging in DNA-cationic liposome assemblies. Febs Lett 1999a; 457: 419–422
- Zuidam NJ, Hirsch-Lerner D, Margulies S, Barenholz Y. Lamellarity of cationic liposomes and mode of preparation of lipoplexes affect transfection efficiency. Biochim Biophys Acta-Biomembr 1999b; 1419: 207–220