Abstract
Genetic hypertension is associated with alterations in lipid metabolism, membrane lipid composition and membrane-protein function. 2-Hydroxyoleic acid (2OHOA) is a new antihypertensive molecule that regulates the structure of model membranes and their interaction with certain peripheral signalling proteins in vitro. While the effect of 2OHOA on elevated blood pressure is thought to arise through its influence on signalling proteins, its effects on membrane lipid composition remain to be assessed. 2OHOA administration altered the lipid membrane composition of hypertensive and normotensive rat plasma membranes, and increased the fluidity of reconstituted liver membranes from hypertensive rats. In spontaneously hypertensive rats (SHR), treatment with 2OHOA increased the cholesterol and sphingomyelin content while decreasing that of phosphatidylserine-phosphatidylinositol lipids. In addition, monounsaturated fatty acid levels increased as well as the propensity of reconstituted membranes to form HII-phases. These data suggest that 2OHOA regulates lipid metabolism that is altered in hypertensive animals, and that it affects the structural properties of liver plasma membranes in SHR. These changes in the structural properties of the plasma membrane may modulate the activity of signalling proteins that associate with the cell membrane such as the Gαq/11 protein and hence, signal transduction.
Abbreviations | ||
SHR | = | spontaneously hypertensive rats |
WKY | = | Wistar Kyoto normotensive rats |
2OHOA | = | 2-hydroxyoleic acid |
CHO | = | cholesterol |
SM | = | sphingomyelin |
PC | = | phosphatidylcholine |
PE | = | phosphatidylethanolamine |
PS | = | phosphatidylserine |
PI | = | phosphatidylinositol |
PL | = | phospholipid |
FA | = | fatty acid |
SFA | = | saturated fatty acid |
UFA | = | unsaturated fatty acids |
MUFA | = | monounsaturated fatty acids |
PUFA | = | polyunsaturated fatty acids |
DPH | = | 1,6-diphenyl-1,3,5-hexatriene |
BP | = | blood pressure |
Introduction
The properties and function of cell membranes appear to be altered in hypertensive humans and animal models of hypertension Citation[1]. Structural changes in membrane lipids that affect membrane fluidity and/or specific lipid domains have been described in hypertension Citation[2–4] and consequently, a number of membrane functions are affected Citation[4–7]. However, how the changes in membrane lipid composition influence cell function is a complex issue Citation[8]. The nature and proportion of lipids in the membrane defines its structural properties, and influences the localization and activity of G proteins and PKC, pivotal cell signaling elements that participate in important physiological activities including the control of blood pressure Citation[7], Citation[9], Citation[10]. On the other hand, the nonlamelar HII phase propensity of membranes, induced by high concentrations of cis-monounsaturated fatty acids and PE lipids, affects the binding of the heterotrimeric Gi proteins and their Gα and Gβγ subunits Citation[11]. Moreover, fatty acids like oleic acid (18:1Δc9), but not its chemical analogs, elaidic (18:1Δt9) and stearic (18:0) acid, not only modulate membrane lipid structure but also regulate the activity of G proteins and related signaling proteins Citation[12], Citation[13]. Other unsaturated fatty acids, such as linoleic (18:2, n-6) and α-linolenic acid (18:3, n-3), can also regulate the structural properties of membranes Citation[14]. Therefore, membrane composition and structure influences G protein-membrane interactions and consequently, cell signalling.
Dietary fat intake regulates the abundance of different membrane lipid species and has an effect on the biochemical status of tissues Citation[15–17]. The Mediterranean diet is characterized by high olive oil consumption, which contains a high proportion of oleic acid (∼80%) with minor percentages of linoleic and linolenic acids. High olive oil intake, as a rich source of natural unsaturated fatty acids, has been shown to have beneficial effects on cardiovascular health Citation[18]. However, the molecular mechanisms underlying the beneficial effects of olive oil are not fully understood. Fatty acids are important components of the hydrophobic part of biological membranes, where they are mainly found as phospholipid moieties. The synthetic fatty acid 2-hydroxyoleic is a structural derivative of oleic acid (OA) that has antihypertensive pharmacological properties and is able to modulate the HII phase propensity in model membranes Citation[19–21]. 2OHOA was able to decrease systolic BP in normotensive rats, while displaying no histological toxicity in several rat organs Citation[19]. Furthermore, administration of 2OHOA to adult spontaneously hypertensive SHR rats with established hypertension decreased high blood pressure, whereas their normotensive counterpart, i.e., WKY rats, remained unaffected Citation[21]. If membrane lipid structure is involved in the cell signalling pathway, then structural alterations of cell membranes will influence such pathways, and may be implicated in pathological situations in which such signalling is involved. Thus, modulating the behaviour of the cell membrane may be a means to reverse human pathologies Citation[22]. While the pharmacological activity of 2OHOA is thought to arise through this mechanism, the effect of this drug on membrane lipid composition in vivo has yet to be described. Therefore, the aim of this study was to analyse the effect of 2OHOA administration on the structural properties of the plasma membrane isolated from adult SHR, an animal model with established hypertension, as well as from its normotensive counterpart, Wistar Kyoto (WKY) rats. Accordingly, we examined the plasma membrane composition and structure in liver tissue from these two rat models. Although liver tissue is not implicated in the direct regulation of blood pressure, it represents an important organ functionally involved in lipid metabolism, it is exposed to the drug after oral administration and its relative amount facilitates the types of studies undertaken here.
Materials and methods
Materials
1,6-diphenyl-1,3,5-hexatriene (DPH) was obtained from Molecular Probes (Leiden, The Netherlands) while the N-(2-Hydroxy ethyl) piperazine-N′-(2-ethanesulfonic acid) sodium salt (Hepes) and Tris(hydroxymethyl)aminomethane hydrochloride (Tris) were obtained from Sigma Chem. Co. (Madrid, Spain). The primary polyclonal antibodies raised against Na+/K+ ATPase, Gαq/1 and poly (ADP ribose) polymerase (PARP) were purchased from Santa Cruz Biotechnology (California, USA), the primary monoclonal antibody against calnexin was from BD Biosciences Transduction Laboratories (Heidelberg, Germany), the horseradish peroxidase-linked donkey anti-rabbit Ig secondary antibody, the enhanced chemiluminescense Western-blot detection system and ECL hyperfilm were all obtained from Amersham Biosciences (UK, Ltd), whereas nitrocellulose membranes were from Schleicher & Schüll (Dassel, Germany). The bicinchoninic (BCA) protein assay kit was manufactured by Pierce (Rockford, IL, USA). Lipid standards, cholesterol, 1,2-dipalmitoyl-sn-glycero-3-phosphatidylethanolamine, 1,2-Diacyl-sn-glycero-3-phosphocholine, 1,2-Diacyl-sn-glycero-3-phospho-L-serine and N-Acyl-4-sphingenyl-1-O-phosphorylcholine were purchased from Sigma-Aldrich (Madrid, Spain). HPLC-grade solvents for lipid analysis were from Carlo Erba (Val de Reuil, France).
Animals, treatments and blood pressure measurements
Male SHR and age-matched normotensive WKY rats (16-week-old, 250–300 g) were obtained from Charles River Laboratories (Barcelona, Spain). The rats were kept at a constant temperature (24±1°C) with a 12 h dark/light cycle fed with the same rodent diet containing 2.9% fat (reference SAFE A04: 0.40% stearic acid, 0.70% oleic/palmitoleic acid, 1.25% linoleic/linolenic acid; daily consumption ∼20 g). Twelve SHR and WKY rats were randomly assigned to a control group that was administered the vehicle alone (n=6) or a group that was administered 2OHOA (600 mg·kg−1, resuspended in water, n=6). Rats were treated orally by gavage every 12 h for 7 days. Systolic blood pressure (BP) was measured in warmed, restrained conscious rats, 6 h after drug administration at least in triplicate using the tail-cuff method with a computerized oscillometric system recorder (Nyprem system from Cibertec, Barcelona, Spain) Citation[21]. Eighteen hours after the last treatment the rats were sacrificed by decapitation, their liver was removed, immediately frozen in liquid nitrogen and stored at −80°C. All experiments were carried out according to the guidelines laid down by the Institutional Committee of Ethics in Animal Research (Comissió de Bioètica de la Universitat de les Illes Balears).
Preparation of liver plasma membranes
Plasma membrane preparations were isolated from 6 livers from each rat strain, SHR and WKY that did or did not receive 2OHOA, by a procedure adapted from Neville Citation[23]. Briefly, the livers were homogenized in 50 mm Tris-HCl, 1 mM EDTA, 2 mm MgCl2, 1 mM PMSF, pH 7.4 (Tris buffer) with a blade-type homogenizer and the samples were centrifuged at 800 g for 10 min at 4°C. The pellets were discarded and the supernatants were then centrifuged twice at 40,000 g for 20 min at 4°C. The pellet was resuspended in the homogenization buffer and the plasma membrane fraction was purified on a discontinuous sucrose gradient (42.3% and 44% (w/w) sucrose) and centrifugated at 90,000 g for 2 h, according to the method described Citation[23]. Finally, the membranes floating at the top of each tube were removed, resuspended in Tris buffer and sedimented at 100,000 g for 90 min at 4°C. The final pellet was stored in Tris buffer. Aliquots of these preparations were used for electron microscopy characterization, Western-blot analysis and to determine the membrane lipid and protein contents.
Immunoblot analysis
Protein determination and quantitative immunoblotting of Na+/K+ ATPase, G protein αq/11, PARP and calnexin in purified liver plasma membranes was performed as described elsewhere Citation[21]. Proteins (around 10 µg) were separated on 8–10% SDS-PAGE electrophoresis, transferred to nitrocellulose membranes and probed overnight at 4°C with the primary antibodies against Na+/K+ ATPase (1:2.000), Gaq/11 (1:5.000), PARP (1:1.000) and calnexin (1:1.000). After removing the primary antibodies, membranes were incubated with the horseradish peroxidase-linked donkey anti-rabbit (1:5.000) or anti-mouse Ig secondary antibody (1:2.000) for 2 h at room temperature. Immunoreactivity was detected using enhanced chemiluminescence (ECL) Western-blot detection system followed by exposure to ECL hyperfilm. Quantification was performed by image analysis, using standard four point curves of different protein contents loaded on the same gels. The relative protein concentration was determined from the standard linear plot of total protein loaded vs. integrated optical density. This quantification procedure was repeated at least 3 times for each sample on different gels. Values were normalized to the protein content of the vehicle-treated rats (taken as 100%).
Electron microscopy observation
A small pellet of the isolated plasma membranes was fixed with 1.6% glutaraldehyde and 1% osmium tetroxide in 0.1 M phosphate buffer, pH 7.4 according to the protocols of the laboratory (Laboratorio Científico – Técnico, University of Balearic Islands, Spain). Electron micrographs were taken with a TEM Hitachi H-600 (TEM-STEM) and used for morphological criteria of membrane isolated.
Sample lipid preparation
Total lipids from rat liver plasma membranes isolated from SHR and WKY rats that did or not receive 2OHOA treatment were extracted with chloroform/methanol (2:1, v:v) and quantified by methods previously described Citation[24], Citation[25]. For X-ray diffraction analysis, multilamellar lipid vesicles (MLV) 15% (w/w) of total membrane lipid extracts were prepared in 10 mM Hepes, 100 mM NaCl, 1 mM EDTA, pH 7.4 (Hepes buffer). Lipid mixtures were hydrated, thoroughly homogenized with a pestle-type minihomogenizer (Sigma) and vortexed until a homogeneous mixture was obtained. Then, the suspensions were submitted to five temperature cycles (heating to 70°C and cooling to 4°C). Samples were stored at −80°C under argon and allowed to equilibrate at 4°C for 48 h before taking the measurements Citation[12].
For fluorescence spectroscopy experiments, membrane lipid extracts and the fluorescence probe DPH (PL:probe, 200:1 mol:mol) were dissolved in chloroform-metanol (2:1, v:v), evaporated under argon and vacuum-dried for at least 3 h. The lipid film was resuspended in Hepes buffer by vortex shaking at about 45°C. The lipid suspension was submitted to five freeze/thaw cycles to ensure the complete hydration of the lipid vesicles. To obtain large unilamellar vesicles (LUV), the resulting multilamellar suspension was passed 11 times through polycarbonate membranes (0.1 µm) in an extruder (Avanti Polar Lipids, Inc.).
Analysis of lipid classes and fatty acid methyl esters
Lipid classes were analysed by HPLC as described previously Citation[26]. Briefly, lipid extracts were dissolved in chloroform:methanol (2:1, v:v), passed through 0.2 µm filters and subsequently analysed by liquid chromatography (2690 Alliance, Waters, Co, Milford, MA, USA), using a Lichrosphere column (250×4.6 mm, 5-µm particle size, Merck) and an evaporative light-scattering detector (ELSD, DDL31 Eurosep, Ins., Cergy-Pontoise). A ternary gradient of hexane, 2-propanol and methanol was applied with a flow rate of 0.8 ml/min. Commercial purchased lipid standards were used to identify and quantify the lipid classes. Cholesterol and each phospholipid mass were determined using calibration curves from lipid standards. The quantification was based on regression analyses of curves with correlation coefficients higher than 0.999. Each lipid mass was converted from grams to moles using the molecular weight of the commercial lipid standard.
Fatty acid methyl esters were analysed by gas chromatography as described previously Citation[2], using a Hewlett-Packard 5890 series II gas chromatograph equipped with a flame ionisation detector (Hewlett-Packard Co, Avondale, USA) and a Supelcowax 10 capillary silica column (60 m and 0.25 mm internal diameter: Sulpelco Co, Bellefonte, USA). Fatty acid methyl esters were identified by comparison of their retention time against those of standards and they were quantified by internal standardization (tricosanoic methyl ester, 23:0), using peak area integration.
X-ray diffraction analysis
Small and Wide-Angle (SAXS and WAXS) Synchrotron radiation X-ray scattering data were collected simultaneously, using standard procedures on the Soft Condensed Matter beamline A2 of HASYLAB at the Deutsches Elektronen Synchrotron (DESY). In the kinetic study, samples were heated from 5–70°C, kept at this temperature for 5 min, and then cooled to 5°C at a scan rate of 1°C/min. To work in quasiequilibrium conditions, the systems were allowed to equilibrate for 15 min at each temperature before taking measurements. The data collection conditions were as described previously Citation[12].
Fluorescence measurements
Membrane fluidity was studied by steady-state fluorescence polarization, using a DPH fluorescent probe. Experiments were carried out on a MPF-66 fluorescence spectrophotometer (Perkin-Elmer), in which the cell-holder was temperature regulated. Large unilamellar vesicle (LUV) membrane lipid extracts and the fluorophore DPH (lipid:probe, 200:1 mol:mol) were excited at 360 nm and emission was registered at 428 nm. The band width was 4 nm for excitation and emission. Samples were equilibrated for 5 min at each temperature prior to measurement. All fluorescence studies were done immediately after sample preparation. In our experimental conditions, the inner filter effect was critical. Therefore, samples were diluted until the optical density of the membrane lipid suspension was adjusted to 0.08 at the maximum excitation wavelength of the DPH probe (360 nm). Light scattering was checked by a control experiment with unlabeled liposomes. Both parameters, fluorescence intensity and polarization, were affected by the scattered light, so the scattered light intensity and polarization were determined by measuring unlabeled control samples in the same conditions as the labeled mixtures. Corrections for the scattered light contribution were carried out as described previously Citation[27]. Fluorescence polarization was calculated according to the equation:where IVV and IVH are fluorescence intensity values measured with the excitation and emission polarizers in parallel and perpendicular, respectively. G is the instrumental factor. All fluorescence polarization data are mean values of two independent experiments.
Statistical analysis
Data are reported as the mean±SEM. The statistical significance of the differences between SHR or WKY rats treated with 2OHOA or vehicle was analysed by the unpaired t-test. Values were considered significantly different, if the p value was <0.05.
Results
SHR and WKY rat treated with 2-OHOA or vehicle showed the following systolic blood pressure values at the end of the treatment (). Liver plasma membranes from these rats were isolated as indicated in Materials and Methods and subsequently characterized by electron microscopy () and the presence of plasma membrane marker Na+/K+ ATPase and membrane-associated Gαq/11 (). Plasma membrane fractions showed the presence of bile canaliculi (BC) and opaque regions that correspond to junctional complexes (indicated by arrows). Na+/K+ ATPase and Gαq/11 protein immunoreactivities were about 2-fold greater in SHR membranes after exposure to 2OHOA than in control SHR cells, the latter displaying low (61%) or similar (117%) levels to cell membranes from WKY rats that were administered 2OHOA or vehicle (100%). No microsomal and nuclear membranes were present in any of these preparations (data not shown).
Figure 1. Electron micrographs of plasma membranes isolated from SHR control rats (SHR-c), SHR rats treated with 2OHOA (SHR-t) and WKY rats treated with 2OHOA (WKY-t). No differences between control and treated WKY membranes were observed. Several bile canaliculi (BC) and regions of higher opacity (indicated by arrows) are shown.
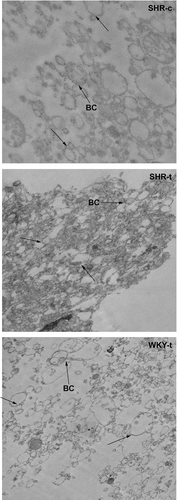
Figure 2. Effects of 2OHOA treatment on Na+/K+ ATPase and G protein αq/11 levels in liver plasma membranes from SHR and WKY rats. The upper panel shows a representative immunoblot of 3 animals per group (around 10 µg total protein). Columns show the levels of liver plasma membrane Na+/K+ ATPase and G protein αq/11 quantified against standard curves and normalized to the protein content of vehicle-treated rats (taken as 100%). ***p < 0.001 and *p < 0.05 vs. vehicle-treated rats.
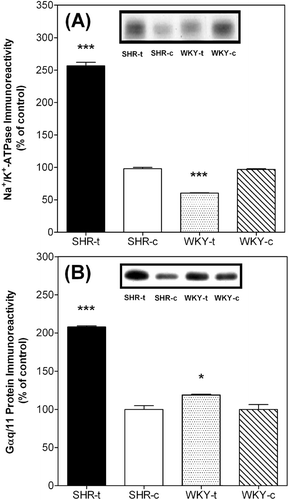
Table I. Effect of 2-hydroxyoleic acid (2OHOA) treatment on systolic blood pressure in spontaneously hypertensive rats (SHR) and Wistar Kyoto (WKY) rats.
Lipid composition
The effect of 2OHOA treatment on the lipid and fatty acid composition of SHR and WKY membranes was evaluated ( and ). Notice that control membranes of both strains (SHR-c and WKY-c) differ significantly in the phospholipid composition. Comparison between SHR and WKY rats indicated that the main effect of 2OHOA on lipid composition was an increase in SM content and in the CHO/PL molar ratio on both strains. 2OHOA treated SHR membranes showed a marked decrease in PS/PI content and an increase in CHO, SM and PC when compared to control SHR membranes (). On the other hand, the differential effect of 2OHOA treatment on normotensive WKY rats was a decrease in PE and PC content without affecting PS/PI.
Table II. Lipid composition of liver plasma membrane from SHR and WKY rats treated with 2-hydroxyoleic acid or vehicle.
Table III. Fatty acid composition of liver plasma membrane from SHR and WKY rats treated with 2-hydroxyoleic acid or vehicle.
The main fatty acids (FAs) in SHR plasma membranes exposed to 2OHOA or not were palmitic (16:0), stearic (18:0), oleic (18:1, n-9), linoleic (18:2, n-6) and arachidonic (20:4, n-6) acids (). 2OHOA treatment induced changes in the levels of certain FAs species that were particularly reflected by a decrease in saturated fatty acids (SFA: palmitic and stearic) and polyunsaturated arachidonic acid. These changes were coupled with an increase in the unsaturated C-18 FAs, oleic and linoleic acid. It is noteworthy that the low oleic acid content in control SHR membranes (7% of total FAs) increased to 22% following exposure to 2OHOA and total polyunsaturated fatty acid (PUFA) content was not altered, although the levels of the fatty acids 18:2 and 20:4 changed in opposite directions. In contrast, 2OHOA treated WKY rats showed increased levels of stearic acid (without affecting oleic acid content) and linoleic acid and decreased levels of arachidonic acid with no marked differences in UFA/SFA ratio.
Polarization of DPH fluorescence
Plasma membrane lipid extracts from SHR and WKY rats were reconstituted in liposomes and the polarisation of the fluorescent DPH probe embedded in reconstituted membranes was analysed (). Exposure to 2OHOA reduced the fluorescence polarisation in reconstituted SHR membranes, indicating a higher degree of fluidity. While similar differences were observed in membranes from WKY rats, these differences were considerably smaller.
Lipid phase behaviour
Membranes reconstituted from lipids isolated from SHR and WKY animals were analysed by X-ray diffraction (). All membrane preparations showed complex X-ray diffraction patterns in the kinetic study (panels A–E), that were further analysed in quasi-equilibrium conditions (panels F–J). All four types of reconstituted membranes displayed a non-lamellar HII structure that was indexed by at least three orders of diffraction peaks. Moreover, liposomes from untreated SHR membranes and those from WKY animals either exposed to 2OHOA or not demonstrated broad diffraction peaks in the SAXS region and no sharp peaks in the WAXS region at lower temperatures. Therefore, these structures were assigned to lamellar Lα phases. All samples exhibited reversible thermotropic behaviour on cooling.
Figure 4. Sequence of X-ray diffraction scattering patterns from reconstituted membranes of total lipid extracts from SHR-c (A, F), SHR-t (B, G), SHR-t plus extra added 2OHOA (C, H), WKY-c (D, I) and WKY-t liver plasma membranes (E, J). The sequence of patterns were acquired in kinetic conditions (A–E) with a scan rate of 1°C/min and quasi-equilibrium conditions (F–J) after equilibrating the sample for 15 min at each temperature. Successive diffraction patterns were collected for 15 s each min. The HII phase was identified by 3–4 diffraction peaks indicated (panels F–J). The Lα phase was identified by the first order reflection peak on the SAXS and the absence of peak on the WAXS region.
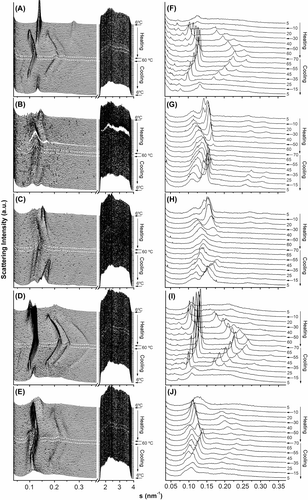
Reconstituted membranes from control SHR formed a HII phase at ca. 20°C and displayed a continuous increase in the peak intensity up to 70°C (the highest temperature measured), whereas those from 2OHOA-treated SHR already adopted a HII phase at 5°C with smaller cylinder diameters (d=9.0 and 6.2 nm at 40°C in control and 2OHOA-treated SHR, respectively; ). Indeed, liposomes from 2OHOA-treated SHR showed weaker structural organisation, as deduced from the compressibility factor (−0.05 and −0.01 nm/°C for control and SHR membranes exposed to 2OHOA, respectively) and broader peaks. Finally, we also observed some very weak diffraction peaks in SHR membranes in the s range 0.07–0.14 nm−1, which could not be unambiguously assigned to common structures formed by model lipid membranes. These peaks seemed to follow the thermal behaviour of a HII phase and therefore, although a more complete exploration of them was beyond the scope of this study, they were somehow associated to this organization. Additionally, we analysed the effect of exposure to 2OHOA on the structural properties of the reconstituted membranes obtained from SHR animals treated with 2OHOA (lipid extract:2OHOA, 10:1 molar ratio). The X-ray diffraction patterns did not change significantly, although the peaks were broader reflecting more poorly defined structures (, panels C and H). The main effect of this additional 2OHOA was a slight decrease in the d parameter from 6.2–6.0 nm at 40°C, and an increase in the compressibility factor of the HII phase from −0.012 and −0.025 nm/°C ().
Figure 5. Dependency of the repeat distance with temperature for the reconstituted membranes from total lipid extract from SHR-c (□), SHR-t (▪), SHR-t plus extra added 2OHOA (♦), WKY-c (○) and WKY-t (•) liver plasma membranes. Phases represented are Lα (dotted lines) and HII (continuous lines). Non-identified phases have been omitted. The heating sequence is only shown.
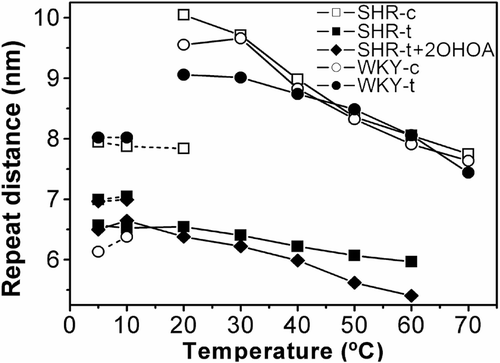
Liposomes reconstituted with membrane lipid extract of vehicle- and 2OHOA-treated WKY (, panels D, E, I, J) showed a Lα phase (d=9.1 and 8.0 nm at 10°C for control and 2OHOA treated animals, respectively) that transformed into HII phase with similar cylinder diameters (d=8.8 and 8.7 nm at 40°C for control and 2OHOA treated animals, respectively, ), although the compressibility factors differed (−0.044 and −0.032 nm/°C for control and 2OHOA treated animals, respectively). Like SHR membranes, model WKY membranes also showed diffraction peaks that could not be assigned to specific phases but that also followed the thermal behaviour of the HII phase.
In summary, the structural effects of 2OHOA on membranes reconstituted from lipid extracts of SHR and WKY rats treated with 2OHOA were: (i) More compact but less organized structures; (ii) HII phases at lower temperatures; and (iii) diffraction peaks from unidentified structures or phases with a d parameter larger but related to the HII structure. Notably, the main effect of 2OHOA was to promote greater curvature and some degree of disorder on the reconstituted membrane.
Discussion
We recently showed that 2OHOA is an effective antihypertensive agent, normalizing high BP in adult spontaneously hypertensive rats (SHR) Citation[21]. Due to the potential importance of 2OHOA in membrane lipid therapy, we set out to obtain information about structural effects of 2OHOA treatment on plasma membranes of these animals, considering those of normotensive Wistar Kyoto (WKY) rats as membranes within healthy physiogical parameters. It is noteworthy that control membranes of both strains differed in lipid composition. In addition, the effects of 2OHOA on membrane lipid composition and structure followed a different pattern in hypertensive SHR and normotensive WKY rats. These structural differences could be related to a different phenotype of SHR and WKY rats, since large genetic abnormalities have been described associated to the genotype of both strains Citation[28]. Therefore, WKY rat strains must be carefully evaluated as ‘healthy controls’ for structural studies. Given the potential interest of these studies for human health, we will mainly focus the discussion on SHR rats. First, 2OHOA changed the lipid composition and increased the monounsaturated fatty acids (MUFAs) in phospholipids of liver plasma membranes in SHR when compared to their respective controls. The significantly higher CHO/PL and SM/PC content in SHR liver plasma membranes exposed to 2OHOA could reflect an effect of 2OHOA on CHO metabolism, CHO liver uptake and/or secretion, and SM metabolism. CHO and SM are structural components of cell membranes that interact strongly with each other (e.g., lipid rafts), and it has been reported that each one influences the metabolism of the other Citation[29–31]. However, the physiological significance of their association is unclear. On the other hand, hydrolysis of membrane SM results in generation of ceramide. Recently, ceramide signalling has been considered a novel cell regulatory pathway implicated in the mechanisms for blood pressure control Citation[32]. Thus, if sphingolipid metabolism is enhanced by 2OHOA, then our data will suggest that the net result would be the formation of second messengers and consequently an increased sphingolipid signalling process. This idea is in agreement with the observed antihypertensive effect of 2OHOA treatment in SHR rats.
Previous studies of cell membranes from SHR rats and humans with hypertension reported altered membrane fluidity Citation[4], Citation[6], Citation[33]. 2OHOA treatment was accompanied by an increase in the fluidity of liposomes reconstituted from liver plasma membranes from SHR rats that was in agreement with data previously reported Citation[6] but not explainable with the lipid composition found. The increase in fluidity should diminish restrictions to the lateral diffusion of components within the membrane, and possibly favour the formation of microdomains. Although our model system (reconstituted membranes) does not provide direct information about the local environment of a biological membrane, these data indicate that variations in the lipid components determine a complex effect on the overall fluid properties. Therefore, the reductions in blood pressure provoked/caused by 2OHOA administration to SHR rats Citation[21] could be supported by the regulatory effects on membrane lipid composition reported in the present study.
The organization of biological membranes into microdomains seems to play a key role in the regulation of membrane-protein interactions Citation[34] and lipids of a given type (e.g., CHO, SM) are able to cluster to form microdomains, such as lipid rafts. Some proteins (e.g. G proteins) that associate with lipid rafts have important biological functions related to signal transduction, and are involved in physiological processes such as the control of blood pressure Citation[35]. Lipid rafts with CHO, SM and PC saturated lipids have been observed in model lipid bilayers and membrane systems isolated from natural membranes Citation[36]. Considering our experimental data, the increase in the SM and CHO/PL ratio observed in 2OHOA treated SHR membranes could be associated with the increased presence of organized domains. The lateral partitioning of CHO and SM could favour association of membrane proteins to specific domains. This possibility would be consistent with the increase in the membrane levels of Gαq/11 proteins and Na+/K+ ATPase observed here in 2OHOA-treated rats. Gαq/11 proteins are involved in the regulation of the phospholipase C (PLC)/inositol phosphate (IP) signalling pathway. Accordingly, several abnormalities in receptor-mediated vasodilation, G-protein expression, and PLC activity, have been reported in hypertensive subjects Citation[7], Citation[37] and in animal models of hypertension Citation[38], Citation[39]. Recent studies have demonstrated that lipids rafts isolated from basolateral plasma membranes of rat hepatocytes were also enriched for Na+/K+ ATPase activity Citation[40]. The increase in the Na+/K+ ATPase immunoreactivity observed in this study would be in line with these observations. Therefore, the structural change induced by 2OHOA, possibly associated with an increase in the density of specific domains, may have functional implication favouring the localization of signalling and other proteins to membranes.
Alterations in phosphoinositide metabolism have been related to the pathogenesis of hypertension Citation[41], Citation[42]. The decrease observed in the content of PS-PI in SHR membranes could be related to the effect of 2OHOA on phosphoinositide turnover and/or membrane polar lipid synthesis or membrane lipid incorporation. From the structural point of view, an increase in the molar percentage of any of these PL would alter the negative charge of the plasma membrane and as a consequence, could favour G protein-membrane interactions. Indeed, it has recently been shown that some specific PLs, such as PS, may play an important role in the interaction of these signalling proteins Citation[43].
Alterations in the phospholipid fatty acid profile have also been associated with genetic hypertension Citation[2], Citation[3], Citation[44], Citation[45]. Comparing FA composition in SHR animals (control and 2OHOA-treated), we conclude that an important effect of 2OHOA treatment was: (i) An increase of Δ9-desaturase, and (ii) depletion of Δ6-and Δ5-desaturase activities, giving high content in oleic acid and reduced proportion of arachidonic acid. It is noteworthy that 2OHOA treatment had a similar effect on PUFA profile in SHR and WKY strains, increasing 18:2 and decreasing 20:4 fatty acid levels while maintaining total PUFA content constant. However, 2OHOA treatment in WKY rats also produced some alteration in PUFA metabolism concerning the conversion of linoleic into arachidonic acid when compared to its control rats, although with minor decreases in enzyme activities than it was the case in SHR. It has been reported that PUFA biogenesis is impaired in SHR compared to WKY rats in liver microsomal total lipids Citation[46]. Working with plasma membranes, our data indicate that: (i) PUFA metabolism is sensitive to 2OHOA treatment and its control might be important in the regulation of SHR blood pressure disease, and (ii) a specific effect of 2OHOA treatment is the increase in oleic acid (∼ 300%), probably due to an activation of Δ9-desaturase activity. The latter data could explain in part the healthy effects of the Mediterranean diet in cardiovascular diseases.
It has been demonstrated that specific FAs such as C18:1 and C18:2 induce HII-phase in model PE membranes at lower temperature Citation[12], Citation[14]. Reconstituted membrane lipids from SHR animals treated with 2OHOA showed a greater tendency to form non-lamellar HII structures. In model membranes with different lipid compositions, it has been shown that a HII-phase propensity influences the localization and activity of some membrane proteins such as G and PKC proteins Citation[9], Citation[11], Citation[13]. This experimental correlation between the non-lamellar phase propensity and an increase in G protein localization Citation[11] or PKC activation Citation[47] demonstrates the influence of membrane structure on the activity of membrane-associated proteins. Many functional responses are probably caused by structural membrane changes that affect bulk lipid fluidity, hexagonal phase propensity and/or specific lipid domains.
In summary, the changes observed in the structural properties of plasma membranes from SHR rats suggest that the lipid metabolism in hypertensive animals was modulated by the antihypertensive molecule 2OHOA, altering the structural properties of liver plasma membrane. Therefore, protein-membrane interactions and/or altered expression of proteins such as those observed here for Gαq/11 protein, could be modulated by exposure to 2OHOA through changes in the structural properties of the plasma membrane.
Acknowledgements
This work was supported by the grants: SAF2004-05249, BFU2005-00749, AGL2005-00572 (Ministerio de Educación y Ciencia, Spain); PRIB-2004-10131 (Govern Balear); I-02-066EC and II-05-051EC from Deutsches Elektronen-Synchrotron DESY, HASYLAB (Hamburg, Germany); by the IHP-Contract HPRI-CT-2001-00140 of the European Commission; and FIS PI031218 (Instituto de Salud Carlos III, Ministerio de Salud y Consumo, Spain). R.A. and O.V. hold contracts from the Ramón y Cajal Programme. We thank M. Pocoví and F. Hierro at the ‘Serveis Cientificotècnics of the Universitat de les Illes Balears’ for their skilful technical assistance.
References
- Zicha J, Kunes J, Devynck MA. Abnormalities of membrane function and lipid metabolism in hypertension: a review. Am J Hypertens 1999; 12: 315–331
- Vazquez CM, Mate A, Angeles de la Hermosa M, Planas JM, Ruiz-Gutierrez V. Abnormalities in lipid composition of brush-border membranes isolated from renal cortex of spontaneously hypertensive rats. Am J Hypertens 2001; 14: 578–584
- Chi Y, Gupta RK. Alterations in heart and kidney membrane phospholipids in hypertension as observed by 31P nuclear magnetic resonance. Lipids 1998; 33: 1023–1030
- Foucher C, Narce M, Nasr L, Delachambre MC, Poisson JP. Liver microsomal membrane fluidity and microsomal desaturase activities in adult spontaneously hypertensive rats. J Hypertens 1997; 15: 863–869
- Feldman RD, Tan CM, Chorazyczewski J. G protein alterations in hypertension and aging. Hypertension 1995; 26: 725–732
- Vazquez CM, Zanetti R, Ruiz-Gutierrez V. Lipid composition and fluidity in the jejunal brush-border membrane of spontaneously hypertensive rats. Effects on activities of membrane-bound proteins. Biosci Rep 1996; 16: 217–226
- Escriba PV, Sanchez-Dominguez JM, Alemany R, Perona JS, Ruiz-Gutierrez V. Alteration of lipids, G proteins, and PKC in cell membranes of elderly hypertensives. Hypertension 2003; 41: 176–182
- Vigh L, Escriba PV, Sonnleitner A, Sonnleitner M, Piotto S, Maresca B, Horvath I, Harwood JL. The significance of lipid composition for membrane activity: new concepts and ways of assessing function. Prog Lipid Res 2005; 44: 303–344
- Escriba PV, Ozaita A, Ribas C, Miralles A, Fodor E, Farkas T, Garcia-Sevilla JA. Role of lipid polymorphism in G protein-membrane interactions: nonlamellar-prone phospholipids and peripheral protein binding to membranes. Proc Natl Acad Sci USA 1997; 94: 11375–11380
- Kinnunen PKJ. On the molecular-level mechanism of peripheral protein-membrane interactions induced by lipids forming inverted nonlamellar phases. Chem Phys Lipids 1996; 81: 151–166
- Vogler O, Casas J, Capo D, Nagy T, Borchert G, Martorell G, Escriba PV. The Gbetagamma dimer drives the interaction of heterotrimeric Gi proteins with nonlamellar membrane structures. J Biol Chem 2004; 279: 36540–36545
- Funari SS, Barcelo F, Escriba PV. Effects of oleic acid and its congeners, elaidic and stearic acids, on the structural properties of phosphatidylethanolamine membranes. J Lipid Res 2003; 44: 567–575
- Yang Q, Alemany R, Casas J, Kitajka K, Lanier SM, Escriba PV. Influence of the membrane lipid structure on signal processing via G protein-coupled receptors. Mol Pharmacol 2005; 68: 210–217
- Prades J, Funari SS, Escriba PV, Barcelo F. Effects of unsaturated fatty acids and triacylglycerols on phosphatidylethanolamine membrane structure. J Lipid Res 2003; 44: 1720–1727
- Escudero A, Montilla JC, Garcia JM, Sanchez-Quevedo MC, Periago JL, Hortelano P, Suarez MD. Effect of dietary (n-9), (n-6) and (n-3) fatty acids on membrane lipid composition and morphology of rat erythrocytes. Biochim Biophys Acta 1998; 1394: 65–73
- Herrera MD, Perez-Guerrero C, Marhuenda E, Ruiz-Gutierrez V. Effects of dietary oleic-rich oils (virgin olive and high-oleic-acid sunflower) on vascular reactivity in Wistar-Kyoto and spontaneously hypertensive rats. Br J Nutr 2001; 86: 349–357
- Ruiz-Gutierrez V, Vazquez CM, Santa-Maria C. Liver lipid composition and antioxidant enzyme activities of spontaneously hypertensive rats after ingestion of dietary fats (fish, olive and high-oleic sunflower oils). Biosci Rep 2001; 21: 271–285
- Estruch R, Martinez-Gonzalez MA, Corella D, Salas-Salvado J, Ruiz-Gutierrez V, Covas MI, Fiol M, Gomez-Gracia E, Lopez-Sabater MC, Vinyoles E, Aros F, Conde M, Lahoz C, Lapetra J, Saez G, Ros E. Effects of a Mediterranean-style diet on cardiovascular risk factors: a randomized trial. Ann Intern Med 2006; 145: 1–11
- Alemany R, Teres S, Baamonde C, Benet M, Vogler O, Escriba PV. 2-hydroxyoleic acid: a new hypotensive molecule. Hypertension 2004; 43: 249–254
- Barcelo F, Prades J, Frau J, Funari SS, Alemany R, Escriba PV. The hypothensive drug 2-hydroxyoleic acid modifies the structural properties of model membranes. Mol Membr Biol 2004; 21: 261–268
- Alemany R, Vogler O, Teres S, Egea C, Baamonde C, Barcelo F, Jakobs KH, Escriba PV. Antihypertensive action of 2-hydroxioleic acid in SHR mediated by enhancement of the PKA pathway and downregulation of RHO-kinase. J Lipid Res 2006; 47: 1762–1770
- Escriba PV. Membrane-lipid therapy: a new approach in molecular medicine. Trends Mol Med 2006; 12: 34–43
- Neville DM, Jr. Isolation of an organ specific protein antigen from cell-surface membrane of rat liver. Biochim Biophys Acta 1968; 154: 540–552
- Folch J, Lees M, Sloane Stanley GH. A simple method for the isolation and purification of total lipides from animal tissues. J Biol Chem 1957; 226: 497–509
- Ruiz-Gutierrez V, Muriana FJ, Guerrero A, Cert AM, Villar J. Plasma lipids, erythrocyte membrane lipids and blood pressure of hypertensive women after ingestion of dietary oleic acid from two different sources. J Hypertens 1996; 14: 1483–1490
- Perona JS, Ruiz-Gutierrez V. Quantification of major lipid classes in human triacylglycerol-rich lipoproteins by high-performance liquid chromatography with evaporative light-scattering detection. J Sep Sci 2004; 27: 653–659
- Kuhry JG, Duportail G, Bronner C, Laustriat G. Plasma membrane fluidity measurements on whole living cells by fluorescence anisotropy of trimethylammoniumdiphenylhexatriene. Biochim Biophys Acta 1985; 845: 60–67
- Johnson ML, Ely DL, Turner ME. Genetic divergence between the Wistar-Kyoto rat and the spontaneously hypertensive rat. Hypertension 1992; 19: 425–427
- Slotte JP, Bierman EL. Depletion of plasma-membrane sphingomyelin rapidly alters the distribution of cholesterol between plasma membranes and intracellular cholesterol pools in cultured fibroblasts. Biochem J 1988; 250: 653–658
- Slotte JP. Sphingomyelin-cholesterol interactions in biological and model membranes. Chem Phys Lipids 1999; 102: 13–27
- Subbaiah PV, Billington SJ, Jost BH, Songer JG, Lange Y. Sphingomyelinase D, a novel probe for cellular sphingomyelin: effects on cholesterol homeostasis in human skin fibroblasts. J Lipid Res 2003; 44: 1574–1580
- Johns DG, Dorrance AM, Leite R, Weber DS, Webb RC. Novel signaling pathways contributing to vascular changes in hypertension. J Biomed Sci 2000; 7: 431–443
- Tsuda K, Iwahashi H, Minatogawa Y, Nishio I, Kido R, Masuyama Y. Electron spin resonance studies of erythrocytes from spontaneously hypertensive rats and humans with essential hypertension. Hypertension 1987; 9(III)19–24
- Brown DA, London E. Functions of lipid rafts in biological membranes. Annu Rev Cell Dev Biol 1998; 14: 111–136
- Simons K, Vaz WL. Model systems, lipid rafts, and cell membranes. Annu Rev Biophys Biomol Struct 2004; 33: 269–295
- Pralle A, Keller P, Florin EL, Simons K, Horber JK. Sphingolipid-cholesterol rafts diffuse as small entities in the plasma membrane of mammalian cells. J Cell Biol 2000; 148: 997–1008
- Marcil J, Schiffrin EL, Anand-Srivastava MB. Aberrant adenylyl cyclase/cAMP signal transduction and G protein levels in platelets from hypertensive patients improve with antihypertensive drug therapy. Hypertension 1996; 28: 83–90
- Shimizu E, Ohyanagi M, Masutani M, Iwasaki T. A decrease in the amount and function of the stimulatory GTP-binding protein in the small resistance arteries of spontaneously hypertensive rats. Hypertens Res 2002; 25: 743–749
- Di Fusco F, Anand-Srivastava MB. Enhanced expression of Gi proteins in non-hypertrophic hearts from rats with hypertension-induced by L-NAME treatment. J Hypertens 2000; 18: 1081–1090
- Mazzone A, Tietz P, Jefferson J, Pagano R, LaRusso NF. Isolation and characterization of lipid microdomains from apical and basolateral plasma membranes of rat hepatocytes. Hepatology 2006; 43: 287–296
- Koutouzov S, Marche P, Girard A, Meyer P. Altered turnover of polyphosphoinositides in the erythrocyte membrane of the spontaneously hypertensive rat. Hypertension 1983; 5: 409–414
- Riozzi A, Heagerty AM, Ollerenshaw JD, Swales JD. Erythrocyte phosphoinositide metabolism in essential hypertensive patients and their normotensive offspring. Clin Sci (Lond) 1987; 73: 29–32
- Hessel E, Heck M, Muller P, Herrmann A, Hofmann KP. Signal transduction in the visual cascade involves specific lipid-protein interactions. J Biol Chem 2003; 278: 22853–22860
- Chi Y, Gupta RK. Alterations in membrane fatty acid unsaturation and chain length in hypertension as observed by 1H NMR spectroscopy. Am J Hypertens 1998; 11: 340–348
- Millanvoye-Van Brussel E, Simon J, Freyss-Beguin M. Altered phospholipid fatty acid content and metabolism in heart cell cultures from newborn spontaneously hypertensive rats. Am J Hypertens 1994; 7: 953–959
- Savelli JL, Narce M, Fustier V, Poisson JP. Desaturase activities are depleted before and after weaning in liver microsomes of spontaneously hypertensive rats. Prostaglandins Leukot Essent Fatty Acids 2002; 66: 541–547
- Slater SJ, Kelly MB, Taddeo FJ, Ho C, Rubin E, Stubbs CD. The modulation of protein kinase C activity by membrane lipid bilayer structure. J Biol Chem 1994; 269: 4866–4871