Abstract
The Saccharomyces cerevisiae integral membrane protein Ssy1p functions with Ssy5p and Ptr3p to sense extracellular amino acids. Signal transduction leads to processing and nuclear localization of Stp1p and Stp2p, transcriptional activators of many amino acid transporter genes. Ssy1p is structurally related to amino acid permeases, but unable to transport amino acids. We isolated SSY1 mutants that constitutively activate a target promoter. Dose-response analysis showed that the mutants are hyperresponsive, requiring less inducer to give strong signaling than does the wild type. Another mutant (Ssy1pT639I) turned out to be hyporesponsive, i.e., it signals only at high inducer concentration. In accordance with a transporter-like mechanism for Ssy1p function we suggest that the hyper- and hyporesponsive mutant forms differ from the wild-type sensor by being more and less inclined, respectively, to adopt an outward-facing, signaling conformation. Coordinate conformational dynamics of the sensor complex was supported by additive effects of combinations of constitutive SSY1, PTR3 and SSY5 alleles. Assuming structural similarity of Ssy1p to the distantly related bacterial leucine transporter LeuTAa, several activating substitutions were located near the substrate binding site while others were on the periphery of Ssy1p. We suggest analyses of transporter-like sensors as an approach to understand key features of transporters.
Introduction
Recent studies have revealed examples of nutrient sensors that have evolved from nutrient transporters but possess no detectable transport activity, having instead gained the ability to activate signaling pathways [reviewed in Citation[1], Citation[2]]. Extensively studied examples are the Saccharomyces cerevisiae glucose sensors Rgt2p and Snf3p and the amino acid sensor Ssy1p, which initiate signal transduction events in response to the extracellular presence of the respective nutrients. Reports are also emerging that point to the existence of transporter-like sensors in plant and mammalian cells [reviewed in Citation[1], Citation[3]].
The amino acid sensor Ssy1p is believed to be part of the so-called SPS sensor complex, which also includes the two membrane-associated proteins Ptr3p and Ssy5p Citation[4–6]. The notion that Ssy1p interacts directly with the sensed amino acid was initially based on its membership of the amino acid-polyamine-organocation (APC) transporter superfamily, which has 24 members in S. cerevisiae [Citation[7], Citation[8], TransportDB database: (http://www.membranetransport.org/)]. Ssy1p is predicted to possess 12 membrane-spanning domains Citation[9] but differs from the other 23 APC members by having a particularly long, ∼280 amino acids, cytoplasmic N-terminal domain. Overproduction of this domain in wild-type cells inhibits amino acid sensing Citation[4], Citation[10], whereas its overproduction in ssy1Δ cells leads to weak, constitutive signaling Citation[10]. In addition, two extracellular loops are ∼20 and ∼35 amino acids longer, respectively, than the corresponding loops in the other amino acid transporters of the yeast APC family. The receptor nature of Ssy1p is supported by its plasma membrane location Citation[5] and the failure to detect amino acid uptake even upon overexpression of SSY1 Citation[4], Citation[11], Citation[12]. The finding that SSY1 can be mutated to cause constitutive signaling Citation[13] makes it further unlikely that the role of Ssy1p in signaling could be to mediate transport of the amino acid followed by its intracellular sensing. On the contrary, high levels of intracellular leucine competitively inhibit the signaling Citation[14].
The functions of other SPS sensor components are also being unraveled. Ssy5p is a chymotrypsin-like protease responsible for processing of the transcription factor Stp1p (and Stp2p) involved in the activation of amino acid permease genes Citation[15], Citation[16]. Ssy5p is synthesized as a zymogen, which is processed into a prodomain of 381 amino acid residues, and a protease domain of 318 residues Citation[17]. The prodomain likely functions as a catalyst for folding the protease domain and as an inhibitor of its protease activity Citation[16], Citation[17]. Because of transducin-like features, Ptr3p has been suggested to mediate conformational changes in the SPS complex Citation[6]. Thus it is generally agreed that the steps in amino acid sensing and signal transduction include binding of an extracellular amino acid molecule to Ssy1p (resulting in stabilization of a signaling conformation of the SPS sensor complex), activation of the Ssy5p protease and subsequent cleavage and translocation of the transcription factors Stp1p and Stp2p to the nucleus Citation[18], Citation[19], and transcriptional activation of amino acid permease genes.
Key aspects of Ssy1p function will rely on knowledge of its structure. However, integral membrane proteins are dramatically under-represented among proteins for which a three-dimensional structure has been determined (http://www.mpibp-frankfurt.mpg.de/michel/public/memprotstruct.html), and the carrier-type transporters are no exception (reviewed Citation[20]). Within the Major Facilitator Superfamily (MFS), only the E. coli lactose permease (LacY) and glycerol–3-phosphate transporter (GlpT) structures have been determined to atomic resolution Citation[21], Citation[22]. In the neurotransmitter sodium symporter (NSS) family, only the structure of the bacterial LeuTAa leucine transporter is known Citation[23]. The X-ray structures of these transporters, each of which has 12 membrane-spanning helices, represent a major step forward in our understanding of the transport of solutes by membrane proteins. The structures have replaced previous simplistic models of these membrane proteins with a detailed picture of oblique helices of various lengths, possessing kinks and other types of irregular features constituting the transporter architecture.
The LacY, GlpT and LeuTAa structures support the conventional alternating-access mechanism of transport, in which the protein undergoes conformational transitions exposing the ligand binding site (or sites in the case of a symporter), either to the outside or the inside of the cell, but not to both sides simultaneously. The dose-response behavior of SSY1 mutant cells hyperresponsive to extracellular amino acids Citation[13] together with the sequence similarity of Ssy1p to the yeast amino acid transporters led us Citation[14] to propose that Ssy1p and other transporter-like sensors function by shifting between conformations that are similar to those of transporters. We further proposed that the outward-facing conformation activates signaling, and that binding of the sensed ligand increases the activation energy for the conformational shift, thereby effectively hindering transport of the ligand through the sensor. This model was tested by investigating the effect of increased concentrations of intracellular leucine, which, as expected from the model, had an inhibitory effect on signaling Citation[14].
In the initial screen for constitutively signaling SSY1 mutants, a single mutant was obtained, in which residue 382 was changed from threonine to lysine Citation[13]. To extend our understanding of signaling through Ssy1p, we wished to determine if mutations affecting other parts of Ssy1p can cause constitutive and/or hyperresponsive signaling. We therefore selected and analyzed constitutively signaling mutants after random PCR mutagenesis of SSY1. We also analyzed an ssy1 mutant previously proposed to be affected in ligand specificity and found that it is primarily a hyporesponsive mutant. The positions of the mutant sites and their influence on sensor function are rationalized in light of the transporter-like functional model and on the basis of an Ssy1p structural model derived in part from the structure of the distantly related leucine transporter LeuTAa from Aquifex aeolicus. To further our knowledge of how the sensor complex functions, we also investigated the effects on signaling when constitutive alleles of SSY1 were co-expressed with constitutive alleles of PTR3 and SSY5.
Materials and methods
Strains, plasmids and media
Details of strain and plasmid constructions are described in Supplementary Materials (online version only), including (strains) and (primers used). Standard media were prepared as described Citation[6].
Mutagenesis of SSY1
Libraries of SSY1 PCR fragments with randomly introduced mutations were constructed with Mutazyme polymerase as recommended by the supplier (Stratagene) with primers SSY1-10 (5′-CCT TGA GGA ATA TGA GTT CTG TCA ACC AA – 3′) and SSY1-12 (5′-CTG TCC ATT TCC CTC CTC CCA CTG TCC – 3′) using 50 ng pSSY1 as template. The PCR program was: an initial 95°C denaturation step for 2 min followed by 40 cycles of 95°C denaturation for 30 sec, 63.4°C annealing for 30 sec, 72°C elongation for 3 min. Finally an elongation step at 72°C for 10 min was included and followed by cooling to 4°C. The PCR was carried out on a Biometra Tgradient thermal cycler (Germany).
Isolation of constitutively signaling SSY1 mutants
Two variations of the screen developed by Gaber et al. Citation[13] were performed. Screen A: Strain M4941 Citation[13] is deleted for TRK1 and TRK2, which encode potassium transporters. This causes a requirement for 100 mM potassium ions in the medium to allow a normal growth rate. In addition, it is deleted for SSY1 and harbors plasmid pAGP1-KAT1, in which the KAT1 gene, encoding an Arabidopsis thaliana potassium channel, is placed under the control of the promoter of the yeast AGP1 gene, which is subject to strong, positive regulation by amino acid signaling through Ssy1p. Growth of this strain on SD, in which the potassium concentration is 7 mM, is slow unless amino acid signaling is active. The strain was transformed with 0.5 µg pPEP16 linearized with BamHI and 0.5 µg Mutazyme-amplified SSY1, using the Gietz lab yeast transformation kit and procedure II (Genomics ONE, Buffalo, NY, USA). The transformation reaction was plated onto SC without uracil and tryptophan but supplemented with 100 mM KCl to allow initial growth irrespective of the functionality of the inserted SSY1 alleles. After 20 h at 30°C, transformants were replica plated to SD. After further 2 to 4 days at 30°C, fast-growing, potentially constitutive SSY1 mutants appeared. Transformants that seemed to grow fast were streaked to single colonies on SD and re-tested for their fast growth by spotting diluted cell suspensions onto SD plates and onto control plates of SD supplemented with 0.2 mM leucine. DNA was isolated from confirmed fast-growing transformants using Yeast DNA Extraction Reagent Kit (Pierce, Rockford, Illinois, USA). E. coli strain DH10 B-U (pyrF-287) Citation[24] was transformed with the yeast DNA preparations, and transformants containing plasmids complementing the uracil deficiency of DH10B-U were selected on M9 minimal medium Citation[25] supplemented with thiamine (1 µg/ml), glucose (0.2%) and casamino acids (0.2%). Plasmid DNA was then isolated from the E. coli transformants and analyzed with restriction enzymes to confirm recombination of the SSY1 ORF into pPEP16. Yeast strain M5380 (ssy1Δ AGP1::lacZ) was then transformed with the plasmids, and β-galactosidase assays were carried out on extracts of the transformants grown on SD to monitor the activation of the AGP1 promoter in the absence of amino acids. Plasmids containing SSY1 alleles that activated the AGP1 promoter were sequenced by custom DNA sequencing at MWG Biotech (Germany) using primers SSY1-14 (5′ GGA AAC AGG CGT GTG ATA AGA G 3′), SSY1-15 (5′ CGC CAT TGA CGA AAT AAA CGA GC 3′), SSY1-16 (5′ GTC CAA CAT TTG ACC TGG CTG 3′) and SSY1-17 (5′ CGA AAT TTG CAG CAA GAG AGG TG 3′).
Screen B: A second screen for SSY1 constitutive mutants was carried out as above with the modification that the amount of pSSY1 template DNA was increased 5 times in the PCR in order to decrease the mutation frequency. However, multiple SSY1 mutants were also obtained in this screen, perhaps reflecting difficulties in controlling the mutation frequency when long templates like the SSY1 ORF (2.3 kb) are PCR-amplified with Mutazyme.
SSY1 alleles that constitutively activated the AGP1 promoter were further analyzed by transforming M5445 (ssy1Δ STP1::ZZ-kanMX) with the respective mutant plasmids and measuring Stp1p processing as described Citation[6], Citation[26].
Site-directed mutagenesis
Site-specific mutations were introduced into the SSY1 ORF using pPEP7 as template and the QuickChange II XL kit (Stratagene) as described by the manufacturer. The primers used for mutagenesis are listed in the Supplementary Materials . Introduction of the mutations was verified by sequencing, and the pPEP7 derivatives were subsequently used as template for PCR amplification of the mutant SSY1 ORFs using primers SSY1-3 (5′ GCT AGC AGT TCT GTC AAC CAA ATA TAT GAC C 3′) and SSY1-4 (5′ CTC GAG CTA AAT CAG CCA GGT AAC CAA C 3′) and Dynazyme DNA polymerase (Finnzymes). Each mutant SSY1 allele was then recombined in vivo into pPEP16 linearized with BamHI in yeast strain M5380 as described above.
Dose-response analysis
A few features of the present technique for dose-response analysis should first be mentioned. Signaling was measured as the amount of processed transcription factor Stp1p after exposure of the cells to amino acid for 10 min divided by the sum of unprocessed and processed Stp1. As described Citation[6], Citation[26], this is a measure that is well determined by genetic tagging of Stp1p and determination of the relative amounts of the processed and unprocessed forms by quantitative western analysis. Representative blots are presented in of the online supplementary material. As apparent in , none of the response curves goes to complete Stp1p processing. As we have previously shown Citation[6], this is due to a steady-state loss of the centromeric plasmid with the respective SSY1 allele during exponential growth, leaving some ssy1Δ cells, which are non-responsive. It should also be noted () that in the SSY1 wild type the EC50 of phenylalanine is 20-fold higher than the EC50 of leucine, as previously found Citation[14]. The absolute values of EC50 are two-fold lower in the present study, which we take to be another feature of the slightly different experimental system.
Figure 1. Constitutive SSY1 mutants have increased apparent affinity for leucine, phenylalanine and L-norvaline. Exponentially growing M5445 cells expressing SSY1 wild type or SSY1 constitutive alleles (SSY1-102, SSY1-130, SSY1-137, SSY1-150 and SSY1-151) were exposed for 10 min to leucine, phenylalanine or L-norvaline at various concentrations, and the EC50 for Stp1p processing was determined. Experiments were performed at least twice. SEM is shown by error-bars.
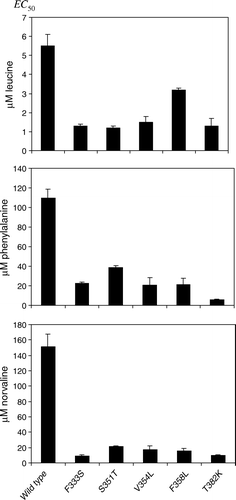
Figure 2. Dose-response analysis of SSY1 mutants. Exponentially growing M5445 cells expressing SSY1 wild type, SSY1-137 or ssy1-23 harbored on centromeric plasmids were induced with a range of concentrations of leucine, phenylalanine or L-norvaline. Stp1p processing was quantified, and the EC50 was estimated. Circles: M5445/pSSY1-137. Triangles: M5445/pSSY1. Squares: M5445/pSSY1-23.
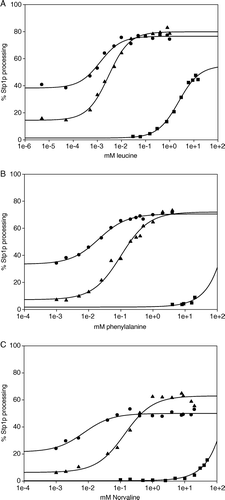
EC50 values were estimated from dose-response relationships by SigmaPlot 2000 (SYSTAT Software Inc.) using the constraints that the basal level of Stp1p processing cannot be negative, and that the maximal processing cannot be more than 100% Citation[14].
Results
Isolation of SSY1 mutants that constitutively activate the AGP1 promoter
To address structure-function relationships of Ssy1p we set out to isolate new SSY1 mutants that activate amino acid signaling in the absence of extracellular activating amino acids. The screen was performed using a modified version (see Materials and Methods) of the potassium channel reporter system that was designed and employed Citation[13] for the isolation of the first constitutive SSY1 mutant. The screening setup makes growth dependent on amino acid signaling. The presence of a mutation causing constitutive signaling therefore leads to rapid growth on SD medium, which does not contain amino acids. Strain M4941 (trk1Δ trk2Δ ssy1Δ pAGP1-KAT1) was transformed with a mixture of a SSY1 fragment library made by error-prone PCR and a linearized, URA3-based acceptor plasmid with sequence overlaps that allow in vivo recombination with the SSY1 fragments. Among approximately 10,000 uracil-independent transformants selected on SD medium, 25 rapidly-growing transformants were identified. Each of the URA3-based plasmids isolated from these transformants contained functional SSY1 alleles, but only 7 conferred constitutive expression of the AGP1 promoter when tested in strain M5380 (ssy1Δ, AGP1::PAGP1-lacZ) for β-galactosidase production (alleles SSY1-130 through SSY1-135 and SSY1-137, of the online supplementary material; screen A in Experimental procedures). In a second, similar screen (B), 19 rapidly-growing transformants of strain M4941 were identified. Rescue of the plasmids and their analysis in strain M5380 verified the presence of constitutive SSY1 alleles on 12 of them (SSY1-138 through SSY1-149, Figure S2). The two screens resulted in a collection of constitutive SSY1 mutants that display AGP1 promoter activation ranging from ca. 2- to 10-fold activation in the absence of amino acids.
Nucleotide sequence analysis of the constitutive SSY1 alleles
Sequences of the mutant SSY1 alleles were determined to identify the amino acid substitutions that confer constitutivity (See details in Supplementary Materials, ). Two mutants contained each a single nucleotide exchange, namely SSY1-130 and SSY1-137, which harbor the T1051A and T998C nucleotide substitutions, respectively, resulting in the amino acid substitutions F333S and S351T. In addition, 10 double mutants, and 8 multiple mutants were obtained. The two independent screens yielded some mutants with nucleotide substitutions at the same position/codon. Two mutations at nucleotide G1819 were obtained (G1819A, G1819T), yielding amino acid substitutions V607I and V607L, respectively. At G2080-T2081, three different mutants were identified (G2080T, G2080A, and T2081G), resulting in the amino acid substitutions V694F/I/G. G2369T was obtained three times, as a result of at least two independent events, resulting in the amino acid substitution G790V. G2362A was obtained twice in screen A, to yield the A788T amino acid substitution.
Construction of single-site SSY1 mutants that confer constitutive signaling
To determine the significance of specific amino acid substitutions in some of the mutants with more than one substitution, we constructed single substitutions by site-directed mutagenesis of the SSY1 wild-type gene; SSY1-150 through SSY1-155. Thus, single-site mutants with the exchanges V354L, F358L, V607I, V694F, A788T and G790V were constructed and tested for AGP1 activation in M5380 cells (Figure S2). V354L, F358L, V694F and G790V conferred constitutive signaling, whereas V607I and A788T did not.
Constitutive SSY1 mutants confer increased levels of processed Stp1p
Signaling from Ssy1p is more directly and more quantitatively determined by measuring processing of the transcription factor Stp1p than by measuring the activity of a target promoter Citation[6], Citation[26]. Plasmids containing the single-exchange alleles were therefore introduced into strain M5445 (ssy1Δ STP1::ZZ), which expresses Stp1p fused at its C-terminus to a 15.5-kDa tandem doublet of an immunoglobulin G-binding domain (Z) of Staphylococcus aureus protein A, enabling quantitative Western analysis of Stp1p processing as described Citation[6], Citation[26]. shows that the absence of functional Ssy1p in M5445/pRS316 results in a basal level of 5% Stp1p processing, whereas wild-type Ssy1p in M5445/pSSY1 yields 12% processed Stp1p in the absence of extracellular amino acids. Alleles SSY1-135 and SSY1-155 conferred only slight increases in processed Stp1p (16% and 17%, respectively). A majority of the mutants resulted in a basal level of Stp1p processing in the range of 20% to 30%, whereas a few strongly affected mutants contained 37% to 53% processed Stp1p.
Table I. Basal Stp1p processing of SSY1 mutations expressed in M5445.
Constitutive SPS sensor components interact additively to increase Stp1p processing
In dose-response analyses of constitutively signaling mutants we previously found hyperresponsive alleles of PTR3 and SSY5 Citation[6], Citation[17], suggesting that amino acid sensing involves co-ordinate conformational changes of all three components of the SPS sensor complex, Ssy1p, Ptr3p and Ssy5p. One prediction of this interpretation is an additive interaction between constitutive mutations. To provide co-expression of the constitutive SSY1-102 allele with constitutive PTR3 and SSY5 alleles we recombined SSY1-102 into the chromosome of strain M5447 (STP1::ZZ), yielding strain M5754. As expected, this strain harboring an empty vector (pRS316) exhibited an increased basal level of processed Stp1p when grown in SD (). Strain M5754 was transformed with various pRS316 derivatives expressing constitutive SSY5 and PTR3 alleles, and the basal levels of Stp1p processing in the absence of extracellular amino acids were measured (). The results show that co-expression of a chromosomally located SSY1-102 gene with Ssy5p protease domain mutants (SSY5-6 or SSY5-15) or PTR3-5 results in a significantly increased Stp1p processing over that of the single mutants. In contrast, signaling by either PTR3- or SSY5-constitutive mutants in ssy1Δ cells is abolished Citation[6]. Thus, the interdependency of the SPS components and the additive interaction between the constitutive mutations supports the notion of co-ordinate conformational changes in a dynamic complex of the three SPS sensor components.
Table II. Constitutive SPS sensor components interact co-operatively to increase Stp1p processing.
Table SI. Strains used in this study.
Table SII. Primers used for introduction of site-specific mutations in SSY1.
Table SIII. Positions of mutations and corresponding amino acid substitutions in SSY1 mutants.
An interesting exception to the general picture is that introduction of SSY5-13 into the SSY1-102 strain did not result in a significant increase in Stp1p processing (). The SSY5-13 mutant has a mutation in the pro-domain of Ssy5p and confers activation of AGP1 due to a slightly increased basal level of processed Stp1p; nevertheless, the EC50 for leucine in cells expressing SSY5-13 is similar to the EC50 obtained for wild-type cells Citation[17], i.e., SSY5-13 is not hyperresponsive. The present result is in accordance with the hypothesis Citation[17] that the Ssy5 prodomain mutant is affected in its interaction with the Ssy5 protease domain, but in a way independent of the putative conformational shifts of the SPS sensor.
Similarly, to test co-expression of the constitutive SSY5-6 allele with different constitutive SSY1 and PTR3 alleles, we recombined SSY5-6 into the chromosome of M5447 (STP1::ZZ), yielding strain M5755. As expected, the chromosomally located SSY5-6 gene results in an increased basal level of processed Stp1p (). When M5755 was transformed with plasmids expressing SSY1-102, SSY1-137, SSY1-151 or PTR3-5, the basal level of Stp1p processing was further increased, supporting the notion of co-ordinate conformational changes in the sensor complex.
Constitutive SSY1 mutants have increased apparent affinity for leucine, phenylalanine and L-norvaline
Amino acid substitutions at position 382 strongly (T382K, T382R) or modestly (T382H and T382L) activate the AGP1 promoter in the absence of extracellular amino acids Citation[13]. In growth assays the T382K and T382R mutants were classified as fully constitutive, whereas the T382H and T382L mutants were classified as hyperresponsive; i.e., signaling was elicited by lower extracellular concentrations of leucine, isoleucine, phenylalanine, citrulline, serine and glutamate than in the wild type. Because an increased sensitivity was observed with each of the elicitors tested in these assays, Gaber et al. Citation[13] concluded that the specificity towards amino acids was unaltered for the T382H and T382L mutants, and suggested that the mutations did not primarily affect the amino acid binding site. Rather, the behavior of the mutants was rationalized by an increased tendency of Ssy1p to assume a signaling, outward-facing conformation Citation[13], Citation[14].
Development of the Stp1p processing assay enabled us to quantify amino acid signaling more precisely and at an earlier point in the pathway Citation[14], Citation[26], and it was revealed that also the T382K mutant is hyperresponsive Citation[6]. In order to explore whether the mutants isolated in the present study could add to this picture, we estimated their EC50 by measuring Stp1p processing in response to different concentrations of amino acids. In a first experiment, all mutants SSY1-130 through SSY1-148 exhibited a reduced EC50 toward leucine compared to the wild type (not shown). Subsequently, the mutants with the single exchanges F333S, S351T, V354L, F358L, and T382K, respectively, were analyzed in more detail. L-leucine and L-phenylalanine were used as inducers because they are the two most potent inducers so far investigated Citation[13], and L-norvaline was used because of its high solubility and similarity to leucine. As shown in , all five mutants have a reduced EC50 for the three tested amino acids; i.e., less inducer is necessary to give strong signaling; in other words, they exhibit an increased apparent affinity for these inducers. Dose-response curves for one of the new mutants, SSY1-137 (F333S) are compared with those of the wild type in .
We interpret the dose responses to mean that F333S, S351T, V354L, and F358L are more prone to adopt an outward-facing signaling conformation, as previously suggested for the T382K substitution Citation[13], Citation[14]. However, since amino acid binding is hypothesized to inhibit shifts between the two conformations Citation[14], it is a priori conceivable that some of such mutations indirectly influence the binding site itself. Indeed, the data in suggest a minor but significant influence of some of the mutations on the amino acid binding site. Thus, when the responses to phenylalanine and L-norvaline are compared, the five hyperresponsive mutants quantitatively analyzed for responsiveness fall into two classes: SSY1-102 (T382K) shows very similar drops to ca. 5% in EC50 relative to that of the wild type for the two amino acids. Consistently, in the, albeit less quantitative, spot analysis by Gaber et al. Citation[13] all analyzed mutants affected at position 382 showed this type of behavior toward the tested, broader range of amino acids, i.e., the relative increase in responsiveness did not depend on the amino acid. The mutants affected in the other four positions, on the other hand, exhibit smaller relative drops in EC50 for phenylalanine than for L-norvaline. The data for leucine show a somewhat different pattern of ratios, but we suspect that the assay does not work well in the very low micromolar range because of significant leucine uptake during the 10-minute exposure period.
Spatial model of Ssy1p
Predictions of structural consequences for the signal-activating amino acid substitutions in Ssy1p are far from straightforward because the structure of an APC family member has not yet been determined. To examine whether a comparison of Ssy1p to other yeast members of the APC superfamily may be informative, the positions of the amino acid substitutions of the six constitutive single-site SSY1 mutants obtained in this investigation and the previously identified T382K substitution Citation[13] were analyzed by aligning Ssy1p with the 17 transporters forming the core of the yeast APC family (Bap2p, Bap3p, Agp1p, Gnp1p, Tat1p, Hip1p, Gap1p, Tat2p, Mmp1p, Sam3p, Agp2p, Can1p, Alp1p, Lyp1p, Put4p, Dip5p, and Agp3p), using the MultAlin software [Citation[27], http://prodes.toulouse.inra.fr/multalin/multalin.html]. The outcome was that none of the sites that gave rise to constitutive signaling mutations are conserved.
To examine whether comparisons of Ssy1p to the solved 3D structures of LacY, GlpT and LeuTAa make sense, we first compared the amino acid sequences of these proteins using the FFAS03 server (http://ffas.burnham.org), which enables detection of remote homologies beyond the reach of other commonly used methods Citation[28]. The scores obtained by alignment of Ssy1p with LacY and GlpT were −0.662 and −0.605, respectively, values that both indicate differences too large for meaningful modeling, which according to Jaroszewski et al. Citation[28] generally requires a score below −9.5 as a single criterion. However, alignment of Ssy1p with LeuTAa yielded a score of −9.25, i.e., close to this limit. Importantly, comparisons of the LeuTAa sequence to the aforementioned 17 yeast APC family members resulted in scores in the range of −14 to −17, encouraging for utilization of the relationship between the APC and NSS families. The FFAS03 algorithm aligned Ssy1p sequences from positions 282 to 800 with the LeuTAa sequences from positions 10 to 501 (). The alignment was further used for assigning the corresponding positions of the Ssy1p mutants into the LeuTAa helix structure (A) and modeling of Ssy1p at (http://ffas.burnham.org) using the Jackal modeling method and the All-atom model (B).
Figure 3. Amino acid sequence alignment of S. cerevisiae Ssy1p and A. aeolicus LeuTAa. The sequences were aligned using the FFAS03 algorithm Citation[28]. Transmembrane α-helices (TM1 through TM12) and an extracellular α-helix (EL2) of LeuTAa are highlighted in grey. Positions of single amino acid substitutions in Ssy1p that result in constitutive signaling are indicated by stars: F333S, S351T, V354L, F358L, T382K, V694F and G790V; substitution T639I, causing hypo-responsiveness, is also indicated by a star.
![Figure 3. Amino acid sequence alignment of S. cerevisiae Ssy1p and A. aeolicus LeuTAa. The sequences were aligned using the FFAS03 algorithm Citation[28]. Transmembrane α-helices (TM1 through TM12) and an extracellular α-helix (EL2) of LeuTAa are highlighted in grey. Positions of single amino acid substitutions in Ssy1p that result in constitutive signaling are indicated by stars: F333S, S351T, V354L, F358L, T382K, V694F and G790V; substitution T639I, causing hypo-responsiveness, is also indicated by a star.](/cms/asset/319b261c-3f0e-40f7-b98d-03e80179236c/imbc_a_277184_f0003_b.gif)
Figure 4. (A) Positions of amino acid substitutions that influence signaling by Ssy1p indicated in the secondary structure of LeuTAa. The activating substitutions F333S in TM2, S351T, V354L and F358L in TM3, T382K in EL3, V694F in TM9, and G790V in TM12 are indicated by green spheres, whereas the substitution T639I in TM8, which leads to hypo-responsiveness, is indicated by a red sphere. (B) Model of Ssy1p based on its similarity to LeuTAa. Modeling was carried out at (http://ffas.ljcrf.edu/ffas-cgi/cgi/ffas.pl) using the Jackal modeling method and the All-atom model. As above, substitutions in Ssy1p are indicated by green and red spheres.
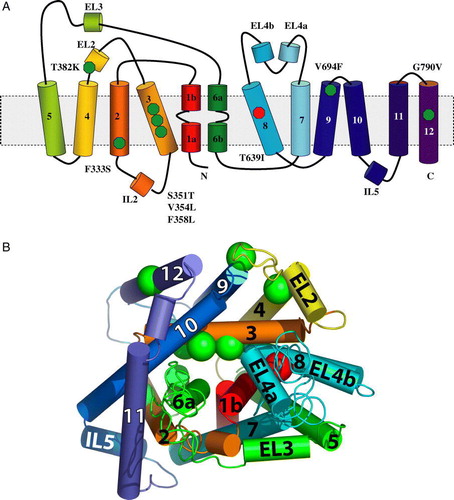
The signal-activating amino acid substitutions are highlighted in the resulting tentative Ssy1p 3D model (B). According to the model, the mutated positions are found spatially clustered in one half of the protein. Substitutions T382K at the end of EL2 and V694F at the end of TM9 are located close to each other at the rim of the protein, facing the outside of the cell near the hydrophilic, negatively charged heads of the membrane phospholipids. G790V is also at the rim of Ssy1p but in the middle of TM12, facing the lipid bilayer. It may be noted that EL2, TM9 and TM12 form the dimer interface of the LeuTAa dimer crystal Citation[23]. The remaining four constitutive substitutions are assigned positions in the interior of Ssy1p; F333S is located to TM2, and S351T, V354L and F358L are located to TM3. Strikingly, the corresponding four positions in LeuTAa are located near the binding sites for leucine and the two sodium ions. In LeuTAa, V104 and Y108 (corresponding to V354 and F358 in Ssy1p, ) are part of the hydrophobic pocket that cradles the aliphatic side chain of leucine; in addition, Y108 is part of the extracellular gate and highly conserved among NSS proteins Citation[29]. The Ssy1p F333S substitution is close to LeuTAa E62, which stabilizes the structure of the unwound region in TM6.
The results of the dose-response analyses described above ( and , and Citation[13]) may be rationalized in terms of the spatial positions of the residue exchanges according to the LeuTAa-based model. Thus, the data suggested that 382 is a position that has very minor significance for the amino acid binding site itself, whereas the four mutants affected in positions 333, 351, 354 and 358 exhibited differential effects toward different amino acids, suggesting slight changes of the binding site. This is consistent with the spatial model, in which position 382 is far from the binding site, and the other positions are closer.
It should be noted that the rationalization of the spatial positions of the amino acid substitutions described above is based on a model only. The direct identity between LeuTAa and Ssy1p is only 14%, and the similarity is 20% when conservative substitutions are included. If one were to disregard the structure of LeuTAa and only predict the topology of Ssy1p using the hidden Markow model according to Citation[9], the three exchanges S351T, V354L and F358L would not be within TM3, but would lie in close proximity to the inside leaf of the plasma membrane. However, transmembrane helices are often much longer and displaced compared to such predictions. As a very relevant example, TM3 of LeuTAa was predicted to be 22 amino acid residues long, but the structure showed that it is 37 amino acids long. Furthermore, considering the consistently favorable scores of the comparisons between LeuTAa and yeast amino acid transporters, we find that the FFAS03 server alignment and structure modeling based on LeuTAa is a much stronger basis for proposals on Ssy1p structure than is a TM prediction that builds on principles on known transmembrane helices in general.
Ssy1pT639I is a hyporesponsive mutant form, with a strongly decreased apparent affinity for leucine, phenylalanine and L-norvaline
Bernard and André Citation[10] reported an ssy1 mutant (ssy1-23, encoding Ssy1pT639I) that is competent in AGP1 promoter activation by 5 mM leucine, but not by 5 mM phenylalanine, methionine or isoleucine, and proposed that the mutant is defective in the recognition of specific amino acids. Since leucine is a much more potent inducer than phenylalanine, isoleucine or methionine [Citation[13], Citation[14], Citation[30], this work], we considered the possibility that the ssy1-23 mutant may instead represent a generally hyporesponsive form of Ssy1p, i.e., one that requires higher concentrations of any inducing amino acid for initiation of signal transduction. To investigate this possibility we subjected the ssy1-23 mutant to dose-response experiments and found that the EC50 for leucine was increased to 2.6 mM, i.e., 500-fold more than the EC50 for the wild type SSY1 allele (A).
Dose-response experiments were also carried out with phenylalanine (B). However, the solubility of phenylalanine did not allow for a full response curve. As seen in B, 20 mM phenylalanine only results in 9% processed Stp1p, precluding a reliable estimate of EC50. Nevertheless, a rough estimate tells us that it is in the range of 200 mM.
A similar pattern was found with L-norvaline. As seen in and C, wild-type Ssy1p yields an EC50 of 168 µM. Again, the EC50 for Ssy1pT639I could not be reliably determined, but again a rough estimate indicates that it is in the range of 200 mM; i.e., Ssy1pT639I requires very high concentrations of L-norvaline to confer signaling.
In conclusion, we have found that the apparent affinity of Ssy1pT639I toward any of the three tested amino acids is several hundred-fold lower than that of wild-type Ssy1p. We therefore consider the mutant form a generally hyporesponsive form of the Ssy1 sensor, and we interpret this to be due to an alteration of the equilibrium between the conformations more strongly favoring the non-signaling conformation, which according to our model Citation[14] is inward-facing. In support of this hypothesis the basal level of Stp1p processing in this mutant is strongly decreased (). According to the LeuTAa-based model of Ssy1p, the T639I substitution in the hyporesponsive mutant lies in TM8 ().
Figure 5. Model of the mechanism of amino acid signaling. In the absence of extracellular amino acids the plasma membrane receptor Ssy1p has a strong preference for an inward-facing conformation and is present in a complex with Ptr3p and the Ssy5p protease, which is inhibited by its interaction with the Ssy5p prodomain. Binding of an extracellular amino acid to Ssy1p stabilizes an altered conformation of the whole complex in which Ssy1p is outward-facing. This event results in the release of the inhibitory Ssy5p prodomain and processing of Stp1p. The figure is a simplified view, and does not consider how phosphorylation/dephosphorylation and ubiquitination Citation[15], Citation[16], Citation[36], Citation[37] may participate in signaling.
![Figure 5. Model of the mechanism of amino acid signaling. In the absence of extracellular amino acids the plasma membrane receptor Ssy1p has a strong preference for an inward-facing conformation and is present in a complex with Ptr3p and the Ssy5p protease, which is inhibited by its interaction with the Ssy5p prodomain. Binding of an extracellular amino acid to Ssy1p stabilizes an altered conformation of the whole complex in which Ssy1p is outward-facing. This event results in the release of the inhibitory Ssy5p prodomain and processing of Stp1p. The figure is a simplified view, and does not consider how phosphorylation/dephosphorylation and ubiquitination Citation[15], Citation[16], Citation[36], Citation[37] may participate in signaling.](/cms/asset/0eee0cef-1e3a-438f-bcd5-ec00a21df753/imbc_a_277184_f0005_b.jpg)
Figure S1. Representative immunoblots, forming the basis of dose-response relationships presented in of the article. Extracts from exponentially growing M5445 cells expressing SSY1 wild type, SSY1-137 or ssy1-23 harboured on centromeric plasmids were analysed. The processing of transcription factor Stp1p was monitored in response to induction with a range of concentrations of leucine and L-norvaline, using a chromosomally encoded, translational fusion (Stp1p-ZZ) in which a doublet of the IgG-binding Z domain of the Staphylococcus aureus protein A had been fused to the C-terminus of Stp1p as described (Poulsen et al. 2005b). The unprocessed and processed forms of Stp1p-ZZ are seen as the slow- and fast-migrating bands, respectively, differing in molecular mass by 10 kDa, the amount by which Stp1 is truncated in the signalling (Andréasson & Ljungdahl [Citation2002]). With SSY1 wild type and SSY1-137 the range of leucine concentrations was from 0.005 µM to 1000 µM, whereas 0.03 mM to 15 mM leucine was used with ssy1-23 (panel A, quantification shown in A of the article). L-Norvaline was used in a concentration range from 0.001 mM to 20 mM for SSY1 wild type and SSY1-137, whereas a range from 0.1 mM to 50 mM norvaline was used with ssy1-23 (panel B, quantification shown in C of the article).
![Figure S1. Representative immunoblots, forming the basis of dose-response relationships presented in Figure 2 of the article. Extracts from exponentially growing M5445 cells expressing SSY1 wild type, SSY1-137 or ssy1-23 harboured on centromeric plasmids were analysed. The processing of transcription factor Stp1p was monitored in response to induction with a range of concentrations of leucine and L-norvaline, using a chromosomally encoded, translational fusion (Stp1p-ZZ) in which a doublet of the IgG-binding Z domain of the Staphylococcus aureus protein A had been fused to the C-terminus of Stp1p as described (Poulsen et al. 2005b). The unprocessed and processed forms of Stp1p-ZZ are seen as the slow- and fast-migrating bands, respectively, differing in molecular mass by 10 kDa, the amount by which Stp1 is truncated in the signalling (Andréasson & Ljungdahl [Citation2002]). With SSY1 wild type and SSY1-137 the range of leucine concentrations was from 0.005 µM to 1000 µM, whereas 0.03 mM to 15 mM leucine was used with ssy1-23 (panel A, quantification shown in Figure 2A of the article). L-Norvaline was used in a concentration range from 0.001 mM to 20 mM for SSY1 wild type and SSY1-137, whereas a range from 0.1 mM to 50 mM norvaline was used with ssy1-23 (panel B, quantification shown in Figure 2C of the article).](/cms/asset/a0939815-6345-44de-91df-edecbe860faa/imbc_a_277184_f0006_b.gif)
Figure S2. SSY1 mutations that cause constitutive activation of the AGP1 promoter. β-Galactosidase activity in extracts of strain M5380 (ssy1Δ AGP1::PAGP1-lacZ) transformed with centromeric plasmids expressing wild type SSY1 or the mutant SSY1 alleles obtained in screen A (SSY1-130 through SSY137), screen B (SSY1-138 through SSY1-149) or by site-directed mutagenesis (SSY1-150 through SSY155). The -galactosidase activities are the means of at least two independent determinations with less than 20% deviation.
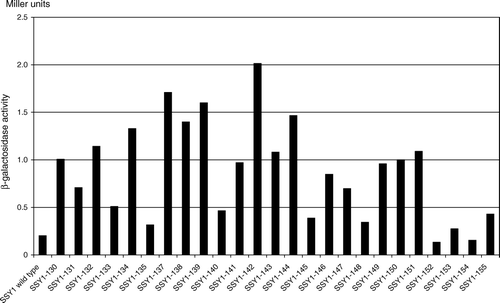
Discussion
Structure-function analysis of Ssy1p is limited by the absence of experimentally determined 3D-structures of closely related proteins. Even for the distantly related proteins for which structures have been determined, structure at the atomic level is only known for a single conformation. Thus, E.coli GlpT has only been crystallized in the inward-facing conformation Citation[22], and a high-resolution structure of E. coli LacY is so far also available only for the inward-facing conformation Citation[21], Citation[31], Citation[32]. In addition, we found that the APC-superfamily member Ssy1p aligns with these two MFS-family members with poor scores. Conversely, S. cerevisiae APC family transporters consistently aligned with LeuTAa with scores that are encouraging for modeling. It may be noted that the crystallized conformation of LeuTAa occludes the ligands, one leucine molecule plus two sodium ions, suggesting that this form is a reaction intermediate between the inward-facing and the outward-facing forms. Whether similar intermediates exist in the APC superfamily is not known, a fact that, however, does not affect the principal points of discussion in the present paper.
In this investigation we isolated a number of constitutive SSY1 mutants that are characterized by having an increased level of Stp1p processing and AGP1 promoter activation in the absence of extracellular amino acids. In addition, the mutants are hyperresponsive, i.e., they require less inducer to give strong signaling. A SSY1 mutant that is hyporesponsive, i.e., it only signals in the presence of high inducer concentrations, was also identified and characterized. According to our hypothesis Citation[14], the hyperresponsive mutants are more prone to adopt an outward-facing, signaling conformation than the wild type, whereas the hyporesponsive mutant is more disposed to remain in an inward-facing, non-signaling conformation. The notion that changes in the equilibrium between conformations will affect apparent affinities without necessarily altering the binding site itself is apparent from thermodynamic considerations [14] but can also be intuitively understood as a need for a higher ligand concentration to occupy the binding site if accessibility favors the other side of the membrane.
The finding that Ssy1p is distantly related to LeuTAa () prompted us to make a structure-function analysis of Ssy1p using the structure of LeuTAa as template to assign the positions of amino acid substitutions in the Ssy1p mutants (). According to the resulting tentative model the signal-activating amino acid substitutions are located near the extracellular gate (F358L, T382K and V694F) and the leucine binding site (F333S, S351T, V354L and F358L), respectively. As discussed above, our and previous data Citation[13] on the responses of mutants with exchanges in position 382 to various amino acids versus the behavior of the new mutants () suggest two slightly different classes of hyperresponsive mutants in accordance with the spatial model. Nevertheless, all the constitutive SSY1 mutations analyzed here fit the notion that they alter the differences in energy of alternative conformations of Ssy1p, favoring an outward-facing, signaling conformation. The Ssy1p T382K and V694F substitutions lie in the proximity of the corresponding LeuTAa residues Y108 and D404, which are part of the extracellular gate Citation[23] (A and 4B), and we propose that the substitutions favor conformational rearrangements that comprise an opening of the extracellular gate. In agreement with this, the behavior of chimeric transporters Citation[33] derived from NSS family members, suggests Citation[23] that EL2 participates in conformational changes during transport.
F358 and F512 in Ssy1p may be part of the extracellular gate similar to Y108 and F253 in LeuTAa, where the two aromatic residues in the crystallized conformation obstruct access to the extracellular space. Thus, it may be speculated that the Ssy1p substitutions (S351T, V354L and F358L) near the substrate binding site favor an opening of the extracellular entrance, which is coordinated with a signaling conformation of the sensor complex, explaining the constitutive properties of the mutants. Additional support for the functional importance of the F358 position in Ssy1p comes from studies of the K+ neutral amino acid transporter KAAT1, in which a substitution (Y147F) in the corresponding position has increased transport activity and altered substrate selectivity Citation[34].
Yamashita et al. Citation[23] suggested that binding of substrates to LeuTAa causes movements in TM1 and TM6, as well as in TM3 and TM8, that may coordinate opening and closing of the gates. The positions of the exchanges causing hyper-responsiveness and constitutivity are consistent with the possibility that similar movements take place in Ssy1p. The hyporesponsive Ssy1p exchange (T639I) resides in TM8 according to the Ssy1p model (). Interestingly, TM8 of APC family members and the GAT family of GABA transporters constitutes a 30-residue consensus amphipathic region that potentially may be involved in substrate binding and conformational changes during the transport process Citation[35]. Substituting the polar threonine residue at position 639 with a hydrophobic leucine residue may affect movements of TM8 by disturbing its polar surface. The suggestion that TM8 participates in movements helping to open and close the outward and inward gates is consistent with the interpretation that the T639I substitution favors an inward-facing, non-signaling conformation of Ssy1p.
The present study increases our understanding of Ssy1p by suggesting functions of specific residues of the sensor and their significance for signaling. The isolation of constitutive and hyperresponsive mutants with exchanges at six new positions, the identification of a hyporesponsive mutant, and the additivity of the effects of the SSY1, PTR3 and SSY5 mutants all support the notion Citation[6], Citation[13], Citation[14] that the equilibrium between a signaling and a non-signaling conformation of the complex of the components encoded by the three genes is a fundamental characteristic of the sensing mechanism (). Thus, binding of an extracellular amino acid to the plasma membrane receptor Ssy1p stabilizes a shift from an inward- to an outward-facing conformation. Ptr3p and Ssy5p take part in the conformational change accompanying this event, resulting in the release of the inhibitory Ssy5p prodomain and processing of Stp1p and Stp2p.
More broadly, our work suggests that studies of Ssy1p and other transporter-like sensors may be useful in providing dynamic information about the mechanisms that bring about shifts in conformation of transporters, information that may not easily be unveiled with mutant forms of transporters that have lost all or most functionality. This opens a new line of investigation to gain insight into structure-function relationships and conformational changes in genuine transporters because the sensors provide an alternative readout of conformations.
Finally, we wish to point to the possibility that, analogous to LacYC154G Citation[21], hypo- and hyperresponsive forms of Ssy1p and other transporter-like sensors may be suitable for crystallization and X-ray structure determination because they may favor a single conformation that is stable enough to crystallize.
Knowledge about Ssy1p constitutive mutants may also be used to increase our understanding of neurotransmitter transporters through a characterization of engineered mutant forms of LeuTAa. Residue substitution in LeuTAa corresponding to the signal-activating/-deactivating Ssy1p substitutions may thus yield mutant forms that can be analyzed in terms of structure and transport kinetics, helping to obtain mechanistic insight in transport.
Supplementary material
Plasmids
Plasmid pSSY1 (Gaber et al. [Citation2003]) was derived by Jørgensen et al. ([Citation1998]) from vector pRS316 (Sikorski & Hieter [Citation1989]) by insertion of SSY1 including its promoter and terminator regions. Plasmid pPEP7 is a pCR2.1 (Invitrogen) derivative containing the SSY1 ORF generated by amplification of SSY1 using primers SSY1-3 (5′-GCT AGC AGT TCT GTC AAC CAA ATA TAT GAC C-3′) and SSY1-4 (5′-CTC GAG CTA AAT CAG CCA GGT AAC CAA C-3′) and pSSY1 as template. Plasmid pPEP16 was made as follows. SSY1 promoter (665 bp) and terminator (535 bp) fragments were produced by PCR using primer pair SSY1-5 (5′- AAG CTT CCG ATC AAG GTA AGG AGG ACA TTC C-3′) and SSY1-6 (5′-GGA TCC CAG CGT CAT TCC AAC CTT GCT CC-3′) and primer pair SSY1-7 (5′-GGA TCC CAG TTA CAC AGC TTA TAA GGT TCT CGG-3′) and SSY1-8 (5′-TCT AGA CCA GGG CTT CTT TGG CTT GCC-3′), respectively. The fragments were treated with HindIII + BamHI and BamHI + XbaI, respectively, and inserted in tandem into pRS316 digested with HindIII + XbaI. Plasmid pPEP16 can thus be linearized by BamHI, which cuts between the promoter and terminator, allowing in vivo recombination with a PCR-mutagenized fragment containing SSY1.
Allele replacement
Chromosomal replacement of the SSY1, PTR3 and SSY5 wild-type alleles with the constitutively signaling mutant alleles SSY1-102, PTR3-5, and SSY5-6, respectively, was carried out as described by Erdeniz et al. (1997). The primers for generating the constitutive alleles were: SSY1 adaptamer A (5′ AAT TCC AGC TGA CCA CCA TGA TGA GTT CTG TCA ACC AAA TAT ATG 3′), SSY1 adaptamer B (5′ GAT CCC CGG GAA TTG CCA TGC TAA ATC AGC CAG GTA ACC AAC 3′), PTR3 adaptamer A (5′ AAT TCC AGC TGA CCA CCA TGA TGC ACT CTC ATA GAC AAA AG 3′), PTR3 adaptamer B (5′ GAT CCC CGG GAA TTG CCA TGT TAT TTG TGG AAT ATT TGC TTA C 3′), SSY5 adaptamer A (5′ AAT TCC AGC TGA CCA CCA TG ATG GTC AGA TTT TTT GGT TTA AAC 3′), SSY5 adaptamer B (5′ GAT CCC CGG GAA TTG CCA TGT CAT CCA TCT AGT TGT GGA TC 3′). Adaptamer a, adaptamer b, internal 5′ primer and internal 3′ primer for K. lactis URA3 were those described by Erdeniz et al. ([Citation1997]). Initial PCR amplifications were performed on a Biometra T-gradient cycler using Dynazyme DNA polymerase (Finnzymes) as recommended by the supplier. The cycling program was as follows: 120 sec at 94°C, then a cycle of 94°C for 15 sec, 54°C for 30 sec, and 72°C for 120 sec repeated 30 times. The last cycle was followed by 10 min at 72°C and subsequent cooling to 4°C. PCR fragments were purified on agarose gels using Qiagen Gel Extraction columns (Qiagen). Fusion fragments were produced using Dynazyme polymerase and ca. 50 ng of each of the mutant allele fragments and the appropriate K. lactis URA3 fragment in a cycling program as above, except that the annealing and elongation steps were 54°C for 30 sec and 72°C for 4 min, respectively. Strains M5359 and M5447 were transformed with ca. 100 ng of the Fusion L and Fusion R fragments using a Gietz lab yeast transformation kit and the standard transformation procedure II (Genomics ONE, Buffalo, NY), and transformants were selected on SC plates without uracil. Pop-out of the selective marker to leave a single copy of the mutant SSY1, PTR3 or SSY5 allele, respectively, on the chromosome was carried out by selection on SC plates with 5-fluoro-orotic acid (1 mg/ml).
The screening system for identification of constitutive SPS sensor components used in this study and in previous studies (Gaber et al. [Citation2003], Poulsen et al. [Citation2005b], Poulsen et al. [Citation2006]) relies on the capture of constitutive SPS sensor alleles expressed from their native promoters on a centromeric plasmid. To test whether the mutant genes expressed from their natural chromosomal location exhibit the same constitutive phenotype as observed when they are plasmid-borne, we recombined the constitutive alleles SSY1-102 (Gaber [et al. Citation2003]) and PTR3-5 and SSY5-6 (Poulsen [et al. Citation2005b]) into the chromosome of M5359 (AGP1::PAGP1-lacZ) by allele replacement, yielding strains M5394, M5395 and M5396. In the absence of extracellular amino acids a 4- to 5-fold increase in AGP1 promoter activity was found in the chromosomally integrated SPS sensor mutants relative to the wild type (not shown). This is in accordance with the results obtained previously with the plasmid-borne SPS sensor mutant alleles (Gaber [et al. Citation2003], Poulsen [et al. Citation2005b], Poulsen [et al. Citation2006]), showing that mutant behavior is essentially independent of whether the mutant genes are located on the chromosome or the plasmid.
Acknowledgements
We thank Lisbeth F. Petersen for skilful technical assistance. We thank Anette Henriksen and Leila Lo Leggio for help in making B. This work was supported by a grant from the Carlsberg Foundation to Peter Poulsen.
References
- Boles E, André B. Role of transporter-like sensors in glucose and amino acid signalling in yeast. Top Curr Genet 2004; 9: 121–153
- Holsbeeks I, Lagatie O, Van Nuland A, Van de Velde S, Thevelein JM. The eukaryotic plasma membrane as a nutrient-sensing device. Trends Biochem Sci 2004; 29: 556–563
- Hyde R, Taylor PM, Hundal HS. Amino acid transporters: roles in amino acid sensing and signaling in animal cells. Biochem J 2003; 373: 1–18
- Forsberg H, Ljungdahl PO. Genetic and biochemical analysis of the yeast plasma membrane Ssy1p-Ptr3p-Ssy5p sensor of extracellular amino acids. Mol Cell Biol 2001; 21: 814–826
- Klasson H, Fink GR, Ljungdahl PO. Ssy1p and Ptr3p are plasma membrane components of a yeast system that senses extracellular amino acids. Mol Cell Biol 1999; 19: 5405–5416
- Poulsen P, Wu B, Gaber RF, Kielland-Brandt MC. Constitutive signal transduction by mutant Ssy5p and Ptr3p components of the SPS amino acid sensor system in Saccharomyces cerevisiae. Eukaryot Cell 2005; 4: 1116–1124
- Chang AB, Lin R, Studley WK, Tran CV, Saier MH, Jr. Phylogeny as a guide to structure and function of membrane transport proteins. Mol Membr Biol 2004; 21: 171–181
- Nelissen B, De Wachter R, Goffeau A. Classification of all putative permeases and other membrane plurispanners of the major facilitator superfamily encoded by the complete genome of Saccharomyces cerevisiae. FEMS Microbiol Rev 1997; 21: 113–134
- Krogh A, Larsson B, von Heijne G, Sonnhammer EL. Predicting transmembrane protein topology with a hidden Markov model: application to complete genomes. J Mol Biol 2001; 305: 567–580
- Bernard F, André B. Genetic analysis of the signalling pathway activated by external amino acids in Saccharomyces cerevisiae. Mol Microbiol 2001; 41: 489–502
- Didion T, Regenberg B, Jørgensen MU, Kielland-Brandt MC, Andersen HA. The permease homologue Ssy1p controls the expression of amino acid and peptide transporter genes in Saccharomyces cerevisiae. Mol Microbiol 1998; 27: 643–650
- Iraqui I, Vissers S, Bernard F, de Craene J-O, Boles E, Urrestarazu A, André B. Amino acid signaling in Saccharomyces cerevisiae: a permease-like sensor of external amino acids and F-box protein Grr1p are required for transcriptional induction of the AGP1 gene, which encodes a broad-specificity amino acid permease. Mol Cell Biol 1999; 19: 989–1001
- Gaber RF, Ottow K, Andersen HA, Kielland-Brandt MC. Constitutive and hyperresponsive signaling by mutant forms of Saccharomyces cerevisiae amino acid sensor Ssy1. Eukaryot Cell 2003; 2: 922–929
- Wu B, Ottow K, Poulsen P, Gaber RF, Albers E, Kielland-Brandt MC. Competitive intra- and extracellular nutrient sensing by the transporter homologue Ssy1p. J Cell Biol 2006; 173: 327–331
- Abdel-Sater F, El Bakkoury M, Urrestarazu A, Vissers S, André B. Amino acid signalling in yeast: Casein kinase I and the Ssy5 endoprotease are key determinants of endoproteolytic activation of the membrane-bound Stp1 transcription factor. Mol Cell Biol 2004; 24: 9771–9785
- Andréasson C, Heesen S, Ljungdahl PO. Regulation of transcription factor latency by receptor-activated proteolysis. Genes Dev 2006; 20: 1563–1568
- Poulsen P, Lo Leggio L, Kielland-Brandt MC. Mapping of an internal protease cleavage site in the Ssy5p component of the amino acid sensor of Saccharomyces cerevisae and functional characterization of the resulting pro- and protease domains by gain-of-function genetics. Eukaryot Cell 2006; 5: 601–608
- Andréasson C, Ljungdahl PO. Receptor-mediated endoproteolytic activation of two transcription factors in yeast. Genes Dev 2002; 16: 3158–3172
- Andréasson C, Ljungdahl PO. The N-terminal regulatory domain of Stp1p is modular and fused to an artificial transcription factor confers full SPS-sensor control. Mol Cell Biol 2004; 24: 7503–7513
- Sobczak I, Lolkema JS. Structural and mechanistic diversity of secondary transporters. Curr Opin Microbiol 2005; 8: 161–167
- Abramson J, Smirnova I, Kasho V, Verner G, Kaback HR, Iwata S. Structure and mechanism of the lactose permease of Escherichia coli. Science 2003; 301: 610–615
- Huang Y, Lemieux MJ, Song J, Auer M, Wang D. Structure and mechanism of the glycerol-3-phosphate transporter from Escherichia coli. Science 2003; 301: 616–620
- Yamashita A, Singh SK, Kawate T, Jin Y, Gouaux E. Crystal structure of a bacterial homologue of Na+/Cl− -dependent neurotransmitter transporters. Nature 2005; 437: 216–223
- Boyd AC, Davidson H, Stevenson B, McLachlan G, Davidson-Smith HH, Porteous DJ. pSURF-2, a modified BAC vector for selective YAC cloning and functional analysis. Biotechniques 1999; 27: 164–170, 172, 175
- Sambrook J, Fritsch EF, Maniatis T. Molecular Cloning, a laboratory manual2nd edn. Cold Spring Harbor Laboratory Press, New York 1989
- Poulsen P, Wu B, Gaber RF, Ottow K, Andersen HA, Kielland-Brandt MC. Amino acid sensing by Ssy1. Biochem Soc Trans 2005; 33: 261–264
- Corpet F. Multiple sequence alignment with hierarchical clustering. Nucleic Acids Res 1988; 16: 10881–10890
- Jaroszewski L, Rychlewski L, Li Z, Li W, Godzik A. 2005. FFAS03: a server for profile-profile sequence alignments. Nucleic Acids Res 33: Web Server issue W284–W288.
- Beuming T, Shi L, Javitch JA, Weinstein H. A comprehensive structure-based alignment of prokaryotic and eukaryotic neurotransmitter/Na+ symporters (NSS) aids in the use of the LeuT structure to probe NSS structure and function. Mol Pharmacol 2006; 70: 1630–1642
- Didion T, Grauslund M, Kielland-Brandt MC, Andersen HA. Amino acids induce expression of BAP2, a branched-chain amino acid permease gene in Saccharomyces cerevisiae. J Bacteriol 1996; 178: 2025–2029
- Guan L, Kaback HR. Lessons from lactose permease. Annu Rev Biophys Biomol Struct 2006; 35: 67–91
- Mirza O, Guan L, Verner L, Iwata S, Kaback HR. Structural evidence for induced fit and a mechanism for sugar/H+ symport in LacY. EMBO J 2006; 25: 1177–1183
- Stephan MM, Chen MA, Penado KM, Rudnick G. An extracellular loop region of the serotonin transporter may be involved in the translocation mechanism. Biochemistry 1997; 36: 1322–1328
- Liu Z, Stevens BR, Feldman DH, Hediger MA, Harvey WR. K+ amino acid transporter KAAT1 mutant Y147F has increased transport activity and altered substrate selectivity. J Exp Biol 2003; 206: 245–254
- Hu L, King SC. Functional sensitivity of polar surfaces on transmembrane helix 8 and cytoplasmic loop 8–9 of the Escherichia coli GABA (4-aminobutyrate) transporter encoded by gabP: mutagenic analysis of a consensus amphipathic region found in transporters from bacteria to mammals. Biochem J 1998; 330: 771–776
- Eckert-Boulet N, Larsson K, Wu B, Poulsen P, Regenberg B, Nielsen J, Kielland-Brandt MC. Deletion of RTS1, encoding a regulatory subunit of protein phosphatase 2A, results in constitutive amino acid signalling via increased Stp1p processing. Eukaryot Cell 2006; 5: 174–179
- Spielewoy N, Flick K, Kalashnikova TI, Walker JR, Wittenberg C. Regulation and recognition of SCFGrr1 targets in the glucose and amino acid signaling pathways. Mol Cell Biol 2004; 24: 8994–9005
- Andréasson C, Ljungdahl PO. Receptor-mediated endoproteolytic activation of two transcription factors in yeast. Genes Dev 2002; 16: 3158–3172
- Boyd AC, Davidson H, Stevenson B, McLachlan G, Davidson-Smith HH, Porteous DJ. pSURF-2, a modified BAC vector for selective YAC cloning and functional analysis. Biotechniques 1999; 27: 164–170, 172, 175
- Erdeniz N, Mortensen UH, Rothstein R. Cloning-free PCR-based allele replacements methods. Genome Res 1997; 7: 1174–1183
- Gaber RF, Ottow K, Andersen HA, Kielland-Brandt MC. Constitutive and hyperresponsive signaling by mutant forms of Saccharomyces cerevisiae amino acid sensor Ssy1. Eukaryot Cell 2003; 2: 922–929
- Jørgensen MU, Bruun MB, Didion T, Kielland-Brandt MC. Mutations in five loci affecting GAP1-independent uptake of neutral amino acids in yeast. Yeast 1998; 14: 103–114
- Poulsen P, Wu B, Gaber RF, Kielland-Brandt MC. Constitutive signal transduction by mutant Ssy5p and Ptr3p components of the SPS amino acid sensor system in Saccharomyces cerevisiae. Eukaryot Cell 2005b; 4: 1116–1124
- Poulsen P, Lo Leggio L, Kielland-Brandt MC. Mapping of an internal protease cleavage site in the Ssy5p component of the amino acid sensor of Saccharomyces cerevisae and functional characterization of the resulting pro- and protease domains by gain-of-function genetics. Eukaryot Cell 2006; 5: 601–608
- Sikorski RS, Hieter P. A system of shuttle vectors and yeast host strains designed for efficient manipulation of DNA in Saccharomyces cerevisiae. Genetics 1989; 122: 19–27