Abstract
Aquaporins are important molecules that control the moisture level of cells and water flow in plants. Plant aquaporins are present in various tissues, and play roles in water transport, cell differentiation and cell enlargement involved in plant growth and water relations. The insights into aquaporins’ diversity, structure, expression, post-translational modification, permeability properties, subcellular location, etc., from considerable studies, can lead to an understanding of basic features of the water transport mechanism and increased illumination into plant water relations. Recent important advances in determining the structure and activity of different aquaporins give further details on the mechanism of functional regulation. Therefore, the current paper mainly focuses on aquaporin structure-function relationships, in order to understand the function and regulation of aquaporins at the cellular level and in the whole plant subjected to various environmental conditions. As a result, the straightforward view is that most aquaporins in plants are to regulate water flow mainly at cellular scale, which is the most widespread general interpretation of the physiological and functional assays in plants.
Abbreviations | ||
AQPs | = | aquaporins |
MIPs | = | major intrinsic proteins |
TIPs | = | tonoplast intrinsic proteins |
NIPs | = | nodulin like plasma membrane intrinsic proteins |
SIPs | = | small intrinsic proteins |
NPA | = | asparagine-proline-alanine |
ABA | = | Abscisic acid |
Introduction
The discovery of aquaporins has shown a new insight into the mechanism of water-transmembrane transportation, which provides solid molecular basis for fast and reversible regulation of transmembrane water transport and gives strong support to the idea that such high water permeability might be required for certain physiological processes Citation[1], Citation[2]. Aquaporins, or major intrinsic proteins (MIPs), are channel-forming membrane proteins with the extraordinary ability to combine a high flux with a high specificity for water across biomembranes. They belong to a well conserved and ancient family of proteins called MIPs with molecular weights in the range of 26–34 kDa Citation[3], and with members found in nearly all living organisms Citation[3]. The aquaporin family in plants is large, indicating complex and regulated water transport within the plant body in order to adapt to different environmental conditions, which includes more than 150 membrane channel proteins Citation[4]. Regulation of aquaporin-mediated water flow, through indirect or direct means, appears to be a mechanism by which plants can control cellular and tissue water movement Citation[5]. All aquaporin isoforms probably work together in an orchestrated manner, where each individual aquaporin isoform displays a specific localization pattern, substrate specificity, and regulatory mechanism Citation[6–8]. The structure, function and gene regulation of aquaporins as well as research methodology are reviewed in this study.
Species diversity of plant aquaporins
The physiological role of water channel proteins is particularly important in plants because of their continuous water recruitment Citation[7], Citation[8]. Many MIP family genes have been identified in plants, such as the members in Arabidopsis, tobacco, spinach, tomato, the ice plant (Mesembryanthemum crystallinum), radish, and snapdragon Citation[2], Citation[3], Citation[9]. The related metabolite facilitator proteins or aquaporins from 38 genes of Arabidopsis thaliana were listed in . The permeability values establish limits on aquaporin tissue densities required for physiological function and suggest significant structural and functional differences among plant aquaporins Citation[10], Citation[11].
There are two highly important aspects of plant aquaporins Citation[2]. One aspect is their tremendous diversity in plants. In the Arabidopsis genome, 38 AQP genes () have been identified Citation[2], Citation[11], Citation[12]. The other aspect is the discovery that some plant aquaporins are multifunctional channel proteins, allowing some small neutral solutes across cellular membranes, such as glycerol, CO2, ammonia (NH3), urea, boron, and hydrogen peroxide Citation[13–17]. The diversity of aquaporin isoforms in plants can also be explained in part by their presence in multiple subcellular compartments Citation[7], Citation[9], Citation[18]. Thus, the capacity of some of these proteins to transport small non-electrolytes in addition to water, together with their broad range of sub-cellular localizations, provides new clues to explain the great diversity of aquaporins in plants.
Table I. 38 genes encoding metabolite facilitator proteins or aquaporins are encoded in the Arabidopsis thaliana genome-tremendous diversity*.
Aquaporins are differentially expressed in different organs and membranes. In the plant kingdom, a single plant expresses a considerably large number of MIP homologues. These homologues can be subdivided into four groups with highly conserved amino acid sequences and intron positions in each group: The tonoplast intrinsic proteins (TIPs) Citation[19–21], the plasma membrane intrinsic proteins (PIPs) Citation[22], Citation[23], the nodulin-like plasma membrane intrinsic proteins (NIP) Citation[24], and the small intrinsic proteins (SIP) Citation[25]. There are various isoforms of plant TIP: Alpha (seed), gamma, Rt (root), and Wsi (water-stress-induced). These proteins may allow the diffusion of water, amino acids and/or peptides from the tonoplast interior to the cytoplasm Citation[21–26]. The plasma membrane aquaporins (PIPs) can be divided into two subfamilies: PIP1 with inactive or low water channel activity and PIP2 with high water channel activity Citation[27]. The high diversity of aquaporins reveals novel facets of plant biomembrane functions Citation[6], Citation[28].
Discovery of the aquaporin family of water channel proteins has provided a molecular explanation for rapid water movements across the plasma membranes of cells while diffusion still works in parallel Citation[9], Citation[26], Citation[28], Citation[29]. This may be one reason for the abundance and diversity of aquaporins, in particular in plants. Their activity may be required for fine regulation at the gene and/or protein levels, which is influenced directly or indirectly by cell metabolism, for example, via phosphorylation of the aquaporin proteins and water stress Citation[6], Citation[29–33].
The numerous aquaporin isoforms of plants have specific expression patterns throughout plant growth-development and in response to environmental stimuli. Once a protein is involved, the cell has the ability to regulate its abundance (transcriptional or post-transcriptional) or to modulate its activity Citation[2], Citation[32], Citation[34]. Water permeability of the plasma membrane and tonoplast through the aquaporins depends on their quantity, activity, and substrate specificity Citation[33]. Partly because of this very high diversity, the knowledge about plant aquaporin expression and subcellular localization is far from complete Citation[34].
Recent studies have shown that their primary structure, transport substrate, functional regulation, gene expression profile, protein amount, and intracellular localization are diversified. Thus, future investigations on the aquaporin family of proteins will provide important information not only on the physiology of membrane transport processes in many cell types, but also on the targeting and trafficking signals that allow proteins to enter distinct intracellular vesicular pathways in cells Citation[6], Citation[32], Citation[34], Citation[35].
Aquaporin gene expression and diurnal fluctuations in plants
Because of the potentially important roles of aquaporins in regulating water flow in plants, studies documenting aquaporin gene expression in specialized tissues involved in water and solute transport are important Citation[35–37]. The high level of expression of ZmTIP1 in maize tissues (root epidermis, small parenchyma cells surrounding mature xylem vessels in the root, etc.) facilitates rapid flow of water through the tonoplast to permit osmotic equilibration between the cytosol and the vacuolar content, and to permit rapid transcellular water flow through living cells when required. Specific plasma membrane aquaporins of the PIP1 subfamily are expressed in sieve elements and guard cells Citation[37] Barley HvPIP2:1 is a plasma membrane aquaporin and its expression was down-regulated after salt stress in barley Citation[38].
The abundance of water channel proteins is a critical parameter to understand their function at the tissue, cell, or subcellular levels. Studies regarding the aquaporin mRNA abundance have led to the following conclusions: (i) Many aquaporins are highly expressed in the vascular tissues, (ii) some tonoplast aquaporins are highly expressed in meristems, where vacuolar biogenesis takes place, and (iii) aquaporins are highly expressed in the tissues that can experience high water or metabolite flux Citation[39–42]. It is therefore of interest to study the expression patterns of AQP isoforms in order to further elucidate their involvement in plant water transport Citation[6], Citation[18], Citation[19].
Under water stress conditions, the expression of the tonoplast aquaporin gene in cauliflower is subject to a precise regulation that can be correlated with important cytological changes in the cells Citation[43]. Therefore, the expression of aquaporin genes may be independently regulated in an organ- and stage-specific manner, while the amount of aquaporin proteins can be regulated at the translational level and by the rate of protein turnover Citation[13], Citation[33], Citation[43], Citation[44]. Meanwhile, there are numerous reports providing clear evidence that the abundance of aquaporins can be regulated by developmental and environmental factors Citation[6], Citation[13], Citation[32]. Monitoring aquaporin gene expression patterns in many plant species in specific tissues, cell types or in response to phytohormones or environmental factors has highlighted the putative roles of water channels Citation[13], Citation[32], Citation[44–46]. Currently, modulation of aquaporin expression in plants is considered the strategy of choice for elucidating the role of aquaporins in plant biology and membrane biology Citation[13].
In Lotus japonicus roots, the PIP1-type water channel shows diurnal fluctuations that are correlated with the diurnal variation in root hydraulic conductivity Citation[46]. Harmer et al. (2000) found that the mRNA level of an Arabidopsis tonoplast aquaporin, GTIP, cycles in a circadian manner Citation[47]. The plasma membrane aquaporin in the motor cells of Samanea saman also shows a diurnal and a circadian regulation Citation[48], Citation[49]. There is a diurnal rhythm in PsPIP2-1 expression in lateral roots that is strongly correlated with diurnal changes in Lpr. Taproots also display a rhythm in PsPIP2-1 expression, but this is offset from that of Lpr Citation[128].
Identifying how many phenotypes are associated with differences in gene expression and how many gene-expression differences are associated with a phenotype is important to understand the molecular basis and evolution of complex traits. Similarly, the comprehensive expression profile of the members of the aquaporin gene family provides a novel basis to allocate the stress-related biological function to each aquaporin gene.
Aquaporin localization in plant cells
Regardless of whether all or only the majorities of the plant MIPs are aquaporins, it is clear that a large number of aquaporins are present in plants. Some are localized in the tonoplast, some in the plasma membrane and some possibly localized in endomembranes Citation[4], Citation[6], Citation[11], Citation[18], Citation[20], Citation[21], Citation[26], Citation[32], Citation[45]. MIP-B has been found in fractions containing tonoplast proteins, distinct from both plasma membrane and tonoplast, and also distinct from the fractions in which MIP-A is located Citation[19], Citation[34], Citation[50]. Only a small amount of MIP-B is located in the plasma membrane fractions, as predicted by sequence alignments. Based on the distribution of PIP proteins within plant cells, Kirch et al. (2000) suggested that the control of plant water channel activity might appear through an analogous vesicle shuttle mechanism Citation[51]. It is possible that the subcellular localization (in the plasma membrane or intracellular vesicles) of aquaporin tetrarners is dependent on the level of phosphorylation of the subunits Citation[5], Citation[52], Citation[53].
Multiple aquaporin genes could allow for variable expression in different tissues, cells and intracellular biomembranes during development, under changing external conditions and in response to rapidly changing demands for water movement Citation[4], Citation[6], Citation[11], Citation[18], Citation[20], Citation[21], Citation[26], Citation[32], Citation[45], Citation[50], Citation[52]. Hufte et al. (1992) found that a presumptive tonoplast aquaporin (Phaseollus a-TIP) is located in multivesicular bodies in transgenic tobaccos that express Phaseollus a-TIP Citation[53]. Aquaporins have been also localized in plasmalemmasomes, invaginating domains of the plasma membrane that protrude into the vacuole Citation[54], Citation[55]. As suggested by Robinson et al. (1996), clustering of aquaporins in the plasmalemmasomes may provide the means for achieving a rapid osmotic balance, and therefore, turgor maintenance in mesophyll cells Citation[54]. A recent study reported the localization and interaction of ZmPIP1s and ZmPIP2s in living maize cells Citation[129].
Repeated experiments have produced the same distribution, and clearly indicate that putatively plasma membrane-located aquaporins show a more complex pattern, and might be localized to different subcellular biomembranes or compartments. Thus, direct determination of the location of each aquaporin within tissues is still required to understand its function in plants.
Aquaporin structure and selectivity in plants
The structures of various aquaporins have provided significant insights into the molecular mechanisms of water permeation. The structure of aquaporins is highly conserved in animals, plants, yeast, and bacteria Citation[4]. All MIP family proteins share six putative transmembrane domains with the N- and C-termini facing the cytosol (A).
Figure 1. Schematic illustration: The atomic structure of the water pore and the mechanism of its selectivity. (A) Share six putative transmembrane domains with the N- and C-termini facing the cytosol; (B) Water molecules passing the channel are forced by the protein's electrostatic forces to flip at the center of the channel.
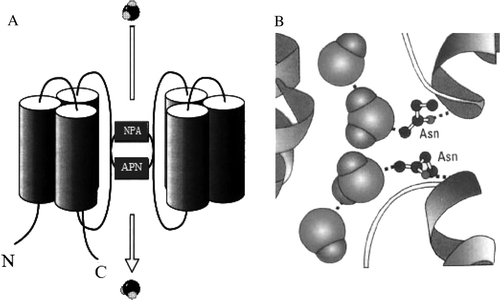
The six transmembrane domains were predicted to be a-helices, packed together with the pore-forming domains outside and towards the center of an aquaporin tetramer Citation[4], Citation[26], Citation[28], Citation[56]. There are five loops (A–E) joining the transmembrane helices. The first cytosolic loop and the third extracytosolic loop are hydrophobic and contain the conserved asparagine-proline-alanine (NPA) sequence Citation[8], Citation[57], Citation[58]. The two halves of the protein show obverse symmetry, with the hydrophobic loops containing the NPA motif (B) overlapping in the middle of the lipid bilayer to form two hemipores that together create a narrow channel proposed to be similar in shape to an hour-glass Citation[57–59].
Aquaporin polypeptides generally form homotetramers in the membrane Citation[60–62], with each monomer forming a single water pore Citation[59]. Water molecules passing the channel are forced by the protein's electrostatic forces to flip at the center of the channel. Water is thought to flow across the water channel pore in a direction down its potential gradient Citation[1], Citation[63].
To account for the molecular selectivity of MIP family channels, the pore so formed was hypothesized to function via a size-exclusion mechanism Citation[1]. Inhibition of the water channel by mercuric chloride is thought to be the result of the sulfhydryl reagent binding a cysteine residue located in a close proximity to the pore, resulting in the physical blockage of the molecular flow through the pore Citation[5], Citation[63], Citation[64]. The gating mechanism successfully unifies a significant body of biochemical and genetic evidence that has identified specific amino-acid residues governing plant aquaporin gating, and suggests how the closed structure might be stabilized or destabilized Citation[5], Citation[65], Citation[66].
Nevertheless, plant aquaporin structures have been reported only at low resolution. Further investigation is necessary to determine the atomic structure of the water pore and the mechanism of its selectivity Citation[5], Citation[65], Citation[67], Citation[130]. Moreover, the specificity of more plant aquaporins also needs to be determined, and plausible plant metabolites need to be tested in order to identify regions important to specificity Citation[5], Citation[9], Citation[20], Citation[32].
The availability of a large body of high resolution structural data along with numerous atomic-scale simulation studies have resulted in an unprecedented level of understanding of the mechanism of function and selectivity in aquaporin. Meanwhile these results clearly demonstrate that modification of the expression patterns or gating properties of selected aquaporins might represent possible targets for manipulating the control of water fluxes within plants.
Regulation of aquaporin water permeability in plants
Land plants have evolved to cope with rapid changes in the availability of water by regulating all aquaporins that lie within the plasma membrane Citation[1]. Regulation of aquaporin trafficking may also represent a way to modulate membrane water permeability, and the factors affecting and regulating aquaporin behaviors possibly include phosphorylation, heteromerization, pH, Ca2 + , pressure, solute gradients, temperature drought, flooding, etc., which suggests plant aquaporins are involved in a versatile and dynamic regulation of water movement Citation[1–3], Citation[5], Citation[9], Citation[20], Citation[32], Citation[50], Citation[57], Citation[58]. The abundance and activity of aquaporins in the plasma membrane and tonoplast may be regulated, hence enabling the plant to tightly control water fluxes into and out of its cells, as well as within the cells Citation[5], Citation[6], Citation[19], Citation[50]. Regulation of aquaporin trafficking may also represent a way to modulate membrane water permeability ().
Figure 2. Schematic three-dimensional structure: Water molecules passing through the aquaporin in the plant biomembrane. This Figure is reproduced in colour in Molecular Membrane Biology online.
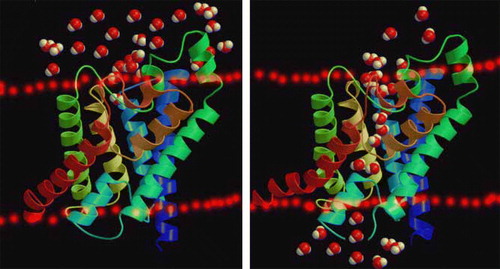
Here, our aim is to integrate recent molecular and biophysical data on the mechanisms regulating aquaporin activity in plant membranes and to relate them to putative changes in protein structure.
Functional regulation of aquaporin activity by phosphorylation
Aquaporin activity may also be directly regulated. Phosphorylation is a major mechanism used by cells as a molecular ‘switch’ to regulate protein activity Citation[5], Citation[19], Citation[20], Citation[30]. The kinases responsible for protein phosphorylation are induced in response to a number of signals, including drought or water stress Citation[19], Citation[64], attacks by pathogens Citation[68], the plant hormones auxin and abscisic acid Citation[19], Citation[69], and light Citation[6], Citation[20]. So we aim to discover how the regulation of aquaporin phosphorylation in plants may be controlled according to developmental stages or environmental cues.
Mutation of the putative phosphorylation sites in alpha-TIP (Ser7Ala, Ser23Ala and Ser99Ala) reduce the apparent water transport activity of alpha-TIP in oocytes, suggesting that phosphorylation of alpha-TIP occurs in the oocytes and participates in the control of water channel activity Citation[29], Citation[35], Citation[70]. Phosphorylation of the putative PM-AQP was thought to activate the water channel composed of PM-AQP. Dephosphorylation of the phosphorylated PM-AQP was also observed during petal closing at 5°C, suggesting the inactivation of the water channel Citation[71].
As demonstrated by Johansson et al. (2002), species- and stress-specific changes in cell hydraulic conductivity may be caused by phosphorylation of aquaporins Citation[26], Citation[35]. The phosphorylation of soybean nodulin 26 on Ser-262, which is catalyzed by a symbiosome membrane-associated calcium-dependent protein kinase, stimulates its intrinsic water transport rate. Soybean nodulin 26 phosphorylation is enhanced further by osmotic stresses (for instance, water deprivation and salinity) Citation[72].
Phosphorylation of the plasma membrane aquaporin PM28A is carried out by a Ca2 + -dependent membrane-bound protein kinase and depends also on the apoplastic water potential Citation[26], Citation[35]. Three PIP2 isoforms (ZmPIP2;1, ZmPIP2;3 and ZmPIP2;4) were detected in the phosphorylated band. The water channel activity of these isoforms was partially inhibited by H7, a PKC inhibitor, suggesting an important effect of phosphorylation on channel function Citation[73]. Both tonoplast and plasma membrane aquaporins have putative phosphorylation sites that are conserved among different members of this family Citation[24].
From current research results, the crucial physiological role of aquaporin phosphorylation and dephosphorylation in plants can be seen, even if the molecular consequence of this modification remains unknown.
Effects of turgor and osmotic pressure on aquaporin activity in plants
Aquaporins are intrinsic membrane proteins characterized by six transmembrane helices that selectively allow water or other small uncharged molecules to pass along the osmotic gradient. However, there is no direct evidence for mechanosensitivity of aquaporin proteins, although some studies have illustrated the potential for MIPS to be involved in volume or turgor homeostasis in plants Citation[3], Citation[74], Citation[75].A different mechanism of mechanical inhibition was recently reported in young maize roots Citation[76]. The dependence of mercury-induced closure of water channels on tissue turgor indicates that changes in turgor can induce changes in the conformation of the plasma membrane, or even in the aquaporins themselves Citation[77]. In more recent experiments, membrane permeability parameters (hydraulic conductivity, permeability and reflection coefficient) of Chara cells were measured using a cell pressure probe when the concentration of variously sized osmolytes was increased Citation[78]. Water-channel activity, inferred from the membrane permeability parameters, was seen to decrease with increasing osmotic pressure and with increasing osmolyte size. Acohesion/tension mechanism was proposed to account for the gating of Chara water channels, which suggests that the osmotic tension generated within the channel by a high osmotic potential leads to the structural collapse and closure of the protein channel. Similar effects of high salinity on membrane water permeability as reported in several species could result, at least partly, from the same mechano-sensitive mechanism Citation[79–81].
However, the functional significance of water channels in maintaining water balance under osmotic stress remains unclear. Under drought or salt stress conditions, transcript or protein abundance of some aquaporins are upregulated, whereas others are downregulated.
Relationships between aquaporins and hormones in plants
Abscisic acid (ABA) is a well-recognized mediator of water stress responses. The responsiveness of each aquaporin to ABA is different, implying that the regulation of aquaporin expression involves both ABA-dependent and ABA-independent signaling pathways. Both energy-input and tension-gating mechanisms might be used by the plant to sense changes in turgor pressure and surrounding water availability, and to adapt the membrane water permeability in an ABA-dependent manner Citation[76]. In many species including sunflower, barley, sorghum and maize Citation[82–84], exogenous ABA enhanced root Lp. Short-term effects (i.e., within 1–3 h) of ABA on root aquaporin gene expression have also been described in different species including rice, Craterostigma plantagineum and Arabidopsis Citation[11], Citation[85–87]. In tobacco flowers, ABA induces expression of the aquaporin gene NtAQP1 Citation[88]. The effect of ABA in rapid positive regulation of aquaporin gating might suggest a direct binding to the channel. However, for both water stress and ABA, comprehensive studies using macro- or micro-arrays are needed to determine whether the complement of aquaporin genes varies in a coordinated fashion, and parallels changes in root water transport Citation[69], Citation[88].
Expression of certain plant aquaporins is regulated by gibberellic acid, or water deprivation. Gibberellic acid is also known to activate the promoter of the aquaporin PIP1b Citation[89], Citation[90]. The significance of promoter regions for an abscisic acid- and gibberellic acid-induced gene expression could be restricted to a region between −1450bp and −1112 bp upstream of the transcription start point by transient transformation of a bicistronic vector into tobacco protoplasts Citation[91]. Gibberellic acid and abscisic acid suppress the levels of mRNAs of RsPIP2-1, RsPIP2-2 and RsPIP2-3 and the protein level of RsPIP2-1 in roots. On the other hand, the protein levels of RsPIP1-group members and RsTIPs are scarcely changed by these phytohormones Citation[92].
Brassinolide may control aquaporin activities in Arabidopsis thaliana, which has been shown to be involved in the modification of the water-transport properties of cell membranes Citation[93], however, mRNA and protein levels of aquaporin isoforms in roots and shoots of radish are not affected by brassinolide treatment Citation[93]. Ethylene significantly increases stomatal conductance, root hydraulic conductivity (Lp), and root oxygen uptake in hypoxic seedlings. Ethylene can increase plasma membrane permeability permitting more water to cross the cells Citation[94]. At the whole plant level, increased water transport in hypoxic aspen seedlings exposed to ethylene has been explained in terms of enhanced aquaporin activity, probably due to a direct effect of ethylene on the phosphorylation of aquaporins Citation[95]. The Lp of root cortex cells was maximally stimulated ≥30-fold after a 1 h exposure to 1 µM ABA, but these effects disappeared over the next hour. In the same studies it was also noted that in contrast to ABA, auxin (IAA) and cytokinin (kinetin) reduced the cell Lp by three- to four-fold Citation[96]. Studies at the cell level provide more direct evidence for cell membranes and aquaporins involved in stimulus-induced regulation of root Lp Citation[1–3], Citation[5], Citation[7], Citation[97].
In addition, valuable insights into the knowledge of the molecular mechanisms by which important hormones in animals, such as vasopressin, secretin, oestrogen, glucagon and hormone peptide YY (PYY), regulate the expression and intracellular trafficking of some aquaporins will be provided in papers by authors who have been well recognized for their studies on aquaporin regulation in animal cells. Nevertheless, in plants it is expected that future studies will allow us to uncover new hormone-induced re-localization of aquaporins. Furthermore, the information will be interpreted in line with the accompanying changes in hormone and function.
Effects of water and nutrient stress on aquaporins in plants
The role of aquaporins in plant water status under water stress is a complex issue, because the expression of different aquaporin genes may be stimulated, reduced, or unchanged under abiotic stress Citation[1], Citation[98–100]. The expression of some genes that encode plasma membrane aquaporins, such as Arabidopsis RD28 and the NeMip2 and NeMip3 genes of Nicotiana excelsior, is stimulated under drought stress Citation[101], Citation[102]. Conversely, expression of the Mesembryanthemum crystallinum MIPA plasma membrane aquaporin gene is down-regulated under salt stress Citation[103], whereas expression of the Arabidopsis PIP1a gene is not altered significantly by stress conditions Citation[104].
Drought stress induced in rice seedlings appeared to increase the physiological functioning of water-channels by increasing the root water channel activity or by increasing the aquaporin amount compared with unstressed control seedlings Citation[105]. Under water-deficit conditions, expression of the tonoplast aquaporin gene in cauliflower is subject to a precise regulation that can be correlated with important cytological changes in the cells Citation[42]. PIP1b overexpression had no beneficial effect on transgenic tobacco under salt stress, whereas during drought stress it had a negative effect, causing faster wilting Citation[106]. Since drought stress decreases the osmotic permeability of root protoplasts but does not have any influence on the amount of PIP1 and PIP2 aquaporins, it is plausible that drought affected the protein functionality Citation[52]. The accumulation of transcripts arising from NgMIP2, NgMIP3 and NgMIP4 diminishes dramatically in drought-stressed plants. This down-regulation of MIP gene expression may result in reduced membrane water permeability and may encourage cellular water conservation during periods of dehydration stress Citation[107].
Aquaporin functionality fits well with the overall water relation responses, since the two-phase adaptation to salinity may imply two types of aquaporin regulation. Salinity can negatively affect root water uptake. Work has shown that exposure of roots to salt induces changes in aquaporin expression at multiple levels. These changes include a coordinated transcriptional down-regulation and subcellular relocalization of both PIPs and TIPs Citation[108]. On the other hand, salt and water stresses induce the accumulation of ZmTIP2-3 transcripts Citation[109]. Similar findings on the dominance of symplastic water transport were obtained by using transgenic tobacco plants expressing an antisense construct of the tobacco NtAQP1 gene that encodes another PIP1b isoform Citation[25], while Barley HvPIP2;1 is a plasma membrane aquaporin and its expression was down-regulated after salt stress in barley Citation[38]. Two aquaporin-encoding transcripts were found to be down-regulated during the first 15 and 60 min of a salt treatment, respectively. The expression level of the two transcripts then recovered and, after 7 d, expression had become higher than in plants grown in standard conditions Citation[105], Citation[110–116].
It is the incoming nutrient supply that is registered as deficient, not the plant's nutrient status. At some point, close to the initiation of these responses, changes in water channel activity may be involved. The reduction of root hydraulic conductivity in wheat plants affected by nutrient (N-, P- and S-) deficiencies, suggested that either the activity or the density of water channels in the root cell plasma membrane is diminished during nutrient deficiency, but the manner in which monitoring of nutrient stress is transduced into an hydraulic response is also unknown Citation[106], Citation[117–125].
The role of aquaporins in plant water status under water stress is a complex issue, because the expression of different aquaporin genes may be stimulated, reduced, or unchanged under abiotic stress. Indeed, this complex expression pattern suggests that maintenance of a reasonable water status under salt and drought stresses requires both increased symplastic water transport via aquaporins in some cells and tissues and reduced water transport via aquaporins in other cells and tissues. Additional evidence for in vivo function of aquaporins is required to conclusively define their contribution to osmotic adaptation under stress conditions.
Concluding remarks
The mechanism by which water passes through biological membranes was a matter of debating until the discovery of the aquaporin water channels. Because aquaporins are a family of membrane channels primarily responsible for conducting water across cellular membranes, the discovery of aquaporins in plants has resulted in a paradigm shift in the understanding of plant-water relations. Water flux across cell membranes has been shown to occur not only through the lipid bilayer, but also through aquaporins, which are members of the major intrinsic protein super-family of channel proteins Citation[2], Citation[3], Citation[5], Citation[9], Citation[20], Citation[32], Citation[57], Citation[117–126]. As has been found in other organisms, plant MIPs function as membrane channels permeable to water (aquaporins) and in some cases to small nonelectrolytes. Aquaporins greatly increase the membrane permeability for water, but may also be regulated, allowing cellular control over the rate of water influx/efflux Citation[1–7]. As a result, aquaporins provide a unique molecular entry point into the water relations of plants and establish fascinating connections between water transport, plant growth and development and adaptive responses of plants to their ever-changing environment Citation[2]. Biochemical and biophysical techniques can be employed to gather information on the basic functional characteristics of plant aquaporins. In fact, the physiological role of aquaporins in plants is much less well understood. The growing knowledge of how aquaporins regulate water passage eventually could help agricultural producers boost survivability and production of drought-stricken and flood-ridden crops Citation[127]. Thus, a challenge for future research will be to understand how plants respond to stresses by integrating these mechanisms in time and space, to constantly adjust the water transport and solute transport properties of their membranes Citation[128–130].
Plants counteract fluctuations in water supply by regulating all aquaporins in the cell plasma membrane. Aquaporins can provide spatial markers to explore the intricate flows of water and solutes that play a critical role throughout all stages of plant life-cycle Citation[2]. The rate of transmembrane water flux may be controlled by changing the abundance or the activity of the aquaporins. Indeed, there are observations showing the alteration of water permeabilities in responses of plants to biotic or abiotic stresses such as high salinity, nutrient deprivation, or extreme temperatures Citation[1–3], Citation[5], Citation[9], Citation[20], Citation[120–130]. In plants, aquaporins are likely to be important both at the whole plant level, for the transport of water to and from the vascular tissues, and at the cellular level, for buffering osmotic fluctuations in the cytosol Citation[19]. Modulation of xylem hydraulic conductivity via sap effects on pit membrane structure may be as important as the regulation of aquaporin activity in leaf and root cell membranes in influencing saturated plant hydraulic conductivity.
By combining molecular biology with plant physiology, it should be possible to determine the role that aquaporins play in water transport in the plant Citation[1–7]. There is growing evidence that suggests that aquaporins play different roles throughout plant development, including nutrient acquisition, carbon fixation, cell signaling and stress responses Citation[115–126]. Therefore, aquaporin genomic information is important because assigning physiological function via transgenic reduction or removal of gene expression requires sequence information for precise targeting. Direct determination of the location of each aquaporin within tissues is still required to understand its function in the plant. A powerful tool in elucidating the aquaporin function is given by the reverse genetics that can also reveal unexpected function of water channel proteins, which benefit our understanding of sequence-structure and structure-function relationships in plants Citation[2], Citation[4], Citation[5], Citation[9], Citation[117–126]. This should be done both at the transcript and at the protein level because aquaporin turnover appears to be variable, such as when comparing constitutively expressed and inducible aquaporins. The transcriptional and/or post-translational regulation of aquaporins would determine changes in membrane water permeability. Both phosphorylation and translocation to/from vesicles have been reported as post-translational mechanisms. Much isolated information has been accumulated, but their function is far from being understood in plants, and there is a long way to go to fully understand the significance of these proteins Citation[124–130].
Aquaporins are thought to be involved in the regulation of trans-cellular water flow for long-distance transport in the root and leaf tissues. Their role is also critical for short-distance water transport and osmotic adjustments within a cell and between the cytoplasm and the cell wall space. The abundance of aquaporins is regulated developmentally in a cell-specific manner and by environmental signals. The activity of aquaporins is also regulated by different post-translational regulation mechanisms, which provide an efficient way for rapid and reversible regulation of the water membrane permeability Citation[128–130]. Now our research project aims at understanding the function and regulation of aquaporins at the cellular and molecular level and in the whole plant subjected to various environmental conditions.
Acknowledgements
This work is jointly supported by 973 Program of China (2007CB106803), Shao Ming-An's Innovation Team Projects of Education Ministry of China and Northwest A&F University, International Cooperative Partner Plan of Chinese Academy of Sciences, Doctoral Foundation of Qingdao Agricultural University (630523) and Project of Shandong Provincial Education Department (J06K57). Special thanks are also given to three reviewers for their valuable comments. Declaration of interest: The authors report no conflicts of interest. The authors alone are responsible for the content and writing of the paper.
References
- Maurel C. Aquporins and water permeability of plant membranes. Annu Rev Plant Physiol Plant Mol Biol 1997; 48: 399–420
- Maurel C, Chrispeels MJ. Aquaporins. A molecular entry into plant water relations. Plant Physiol 2001; 125: 135–138
- Tyreman SD, Niemietz CM, Bramley H. Plant aquaporins: Multifunctional water and solute channels with expanding roles. Plant Cell Environ 2002; 25: 173–194
- Fu D, Lu M. The structural basis of water permeation and proton exclusion in aquaporins (Review). Mol Mem Biol 2007; 24: 366–374
- Maurel C. Plant aquaporins: Novel functions and regulation properties. FEBS Let 2007; 581: 2227–2236
- Kaldenhoff R, Fischer M. Functional aquaporin diversity in plants. BBA-Biomembranes 2006; 1758: 1134–1141
- Chrispeels MJ, Agre P. Aquaporins: Water channel proteins of plant and animal cells. TIBS 1994; 19: 421–425
- Kjelbom P, Larsson C, Johansson I. Aquaporins and water homeostasis in plants. Trends Plant Sci 1999; 4: 308–314
- Tyreman SD, Bohnert HJ, Maurel C. Plant aquaporins: Their molecular biology, biophysics and significance for plant water relations. J Exp Bot 1999; 50: 1055–1071
- Verkman AS, Yang B. Aquaporin and ion conductance (letter). Science 1997; 275: 1491–1492
- Weig A, Deswarte C, Chrispeels MJ. The major intrinsic protein family of Arabidopsis has 23 members that form three distinct groups with functional aquaporins in each group. Plant Physiol 1997; 114: 1347–1357
- Ishikawa F, Suga S, Uemura T. Novel type aquaporin SIPs are mainly localized to the ER membrane and show cell-specific expression in Arabidopsis thaliana. FEBS Let 2005; 579: 5814–5820
- Hachez C, Zelazny E, Chaumont F. Modulating the expression of aquaporin genes in planta: A key to understand their physiological functions?. BBA–Biomembranes 2006; 1758: 1142–1156
- Gerbeau P, Amodeo G, Hnezler T. The water permeability of Arabidopsis plasma membrane is regulated by divalent cations and pH. Plant J 2002; 30: 71–81
- Henzler T, Steudle E. Transport and metabolic degradation of hydrogen peroxide in Chara corallina: A model calculations and measurement with the pressure probe suggest transport of H2O2 across water channels. J Exp Bot 2000; 51: 2053–2066
- Ciavatta VT, Morillon R, Pullman GS. An aquaglyceroporin is abundantly expressed early in the development of the suspensor and the embryo proper of loblolly pine. Plant Physiol 2001; 127: 1556–1567
- Dordas C, Brown PH. Evidence for channel mediated transport of boric acid in squash (Cucurbita pepo). Plant Soil 2001; 235: 95–103
- Takata K, Matsuzaki T, Tajika Y. Aquaporins: Water channel proteins of the cell membrane. Prog Histochem Cytochem 2004; 39: 1–83
- Johansson I, Karlsson M, Johanson U. The role of aquaporins in cellular and whole plant water balance. BBA–Biomembranes 2000; 1465: 324–342
- Chaumont F, Moshelion M, Daniels MJ. Regulation of plant aquaporin activity. Biol Cell 2005; 97: 749–764
- Johanson U, Gustavsson S. A new subfamily of major intrinsic proteins in plants. Mol Biol Evol 2002; 19: 456–461
- Johansson I, Larsson C, BE. The major integral proteins of spinach leaf plasma membranes are putative aquaporins and are phosphorylated in response to Ca2 + and apoplastic water potential. Plant Cell 1996; 8: 1181–1191
- Suga S, Maeshima M. Water channel activity of radish plasma membrane aquaporins heterologously expressed in yeast and their modification by site-directed mutagenesis. Plant Cell Physiol 2004; 45: 823–30
- Kjelbom P, Larsson C, Johansson I. Aquaporins and water homeostasis in plants. Trends Plant Sci 1999; 4: 308–314
- Siefritz F, Tyree MT, Lovisolo C. PIP1 plasma membrane aquaporins in tobacco: From cellular effects to function in plants. Plant Cell 2002; 14: 869–876
- Verkman AS, Mitra AK. Structure and function of aquaporin water channels. Am J Physiol Renal Physiol 2000; 278: 13–28
- Chaumont F, Jung R. Plasma membrane intrinsic proteins from maize cluster in two sequence subgroups with differential aquaprorin activity. Plant Physiol 2000; 122: 1025–1034
- Schaffner AR. Aquaporin function, structure and expression: Are there more surprises to surface in water relations. Planta 1998; 204: 131–139
- Maurel C, Kado RT, Guer J. Phosphorylation regulates the water channel activity of the seed-specific aquaporin α-TIP. EMBO J 1995; 14: 3028–3035
- Wallace IS, Choi WG, Roberts DM. The structure, function and regulation of the nodulin 26-like intrinsic protein family of plant aquaglyceroporins. BBA–Biomembranes 2006; 1758: 1165–1175
- Shao HB, Chu LY, Cheruth AJ, Zhao CX. Water-deficit stress-induced anatomical changes in higher plants. C R Biologies 2008; 331: 215–225
- Maurel C, Javot H, Lauvergeat V. Molecular physiology of aquaporins in plants. Int Rev Cyto 2002; 215: 105–148
- Suga S, Imagawa S, Maeshima M. Specificity of the accumulation of RNA and proteins of the plasma membrane and tonoplast aquaporins in radish organs. Planta 2001; 212: 294–304
- Santoni V, Gerbeau P, Javot H. The high diversity of aquaporins reveals novel facets of plant membrane functions. Curr Opin Plant Biol 2000; 3: 476–481
- Johansson I, Karlsson M, Shukla VK. Water transport activity of the plasma membrane aquaporin PM28A is regulated by phosphorylation. Plant Cell 1998; 10: 451–460
- Fotiadis D, Jeno P, Mini T. Structural characterization of two aquaporins isolated from native spinach leaf plasma membranes. J Biol Chem 2001; 276: 1707–1714
- Fraysse LC, Wells B, McCann MC. Specific plasma membrane aquaporins of the PIP1 subfamily are expressed in sieve elements and guard cells. Biol Cell 2005; 97: 519–534
- Katsuhara M, Akiyama Y, Koshio K. Functional analysis of water channels in barley roots. Plant Cell Physiol 2002; 43: 885–893
- Barrieu F, Chaumont F, Chrispeels M. High expression of the tonoplast aquaporin ZmTIP1 in epidermal and conducting tissues of maize. Plant Physiol 1998; 117: 1153–1164
- Chrispeels MJ, Crawford NM, Schroeder JI. Proteins for transport of water and mineral nutrients across the membranes of plant cells. Plant Cell 1999; 11: 661–675
- Ionenko IF, Anisimov AV. Radial diffusion transport of water in various zones of maize root and its sensitivity to mercury chloride. Rus J Plant Physiol 2007; 54: 224–229
- Barrieu F, Marty-Mazars D, Thomas D. Desiccation and osmotic stress increase the abundance of mRNA of the tonoplast aquaporin BobTIP26-1 in cauliflower cells. Planta 1999; 209: 77–86
- Uehlein N, Fileschi K, Eckert M. Arbuscular mycorrhizal symbiosis and plant aquaporin. Phytochem 2007; 68: 122–129
- Shiota H, Sudoh T, Tanaka I. Expression analysis of genes encoding plasma membrane aquaporins during seed and fruit development in tomato. Plant Sci 2006; 171: 277–285
- Kaldenhoff R, Eckert M. Features and function of plant aquaporins. J Photochem Photobiol B: Biology 1999; 52: 1–6
- Henzler T, Waterhouse RN, Smyth AJ. Diurnal variations in hydraulic conductivity and root pressure can be correlated with the expression of putative aquaporins in the roots of Lotus japonicas. Planta 1999; 210: 50–60
- Harmer SL, Hogenesch JB, Straume M. Orchestrated transcription of key pathways in Arabidopsis by the circadian clock. Science 2000; 290: 2110–2113
- Moshelion M, Becker D, Biela A. Plasma membrane aquaporins in the motor cells of Samanea saman: Diurnal and circadian regulation. Plant Cell 2002; 14: 727–739
- Moshelion M, Moran NF. Chaumont. Dynamic changes in the osmotic water permeability of protoplast plasma membrane. Plant Physiol 2004; 135: 2301–2317
- Javot H, Maurel C. The role of aquaporins in root water uptake. Ann Bot 2002; 90: 301–313
- Kirch HH, Vera-Estrella R, Golldack D. Expression of water channel proteins in Mesembryanthemum crystallinum. Plant Physiol 2000; 123: 111–124
- Morillon R, Lassalles JP. Water deficit during root development: Effects on the growth of roots and osmotic water permeability of isolated root protoplasts. Planta 2002; 214: 392–399
- Hufte H, Hubbard L, Reizer J. Vegetative and seed-specific forms of tonoplast intrinsic protein in the vacuolar membrane of Arabidopsis thaliana. Plant Physiol 1992; 99: 561–570
- Robinson DG, Sieber H, Kammerloher W. PIP1 aquaporins are concentrated in plasmalemmasomes of Arabidopsis thaliana mesophyll. Plant Physiol 1996; 111: 645–649
- Frangne N, Maeshima M, SchBffner AR. Expression and distribution of a vacuolar aquaporin in young and mature leaf tissues of Brassica napus in relation to water fluxes. Planta 2001; 212: 270–278
- Heymann JB, Engel A. Structural clues in the sequences of the aquaporins. J Mol Biol 2000; 295: 1039–1053
- Tyreman SD, Niemietz CM, Bramley H. Plant aquaporins: Multifunctional water and solute channels with expanding roles. Plant Cell Environ 2002; 25: 173–194
- Shibashi K. Aquaporin subfamily with unusual NPA boxes. BBA–Biomembranes 2006; 1758: 989–993
- Jung JS, Preston GM, Smith BL. Molecular structure of the water channel through aquaporin CHIP – the hourglass model. J Biol Chem 1994; 269: 14658–14654
- Daniels MJ, Chrispeels M, Yeager M. Projection structure of a plant vacuolar membrane aquaporin by electron cryo-crystallography. J Mol Biol 1999; 294: 1337–1349
- Engel A, Fujiyoshi Y, Agre P. The importance of aquaporin water channel protein structures. EMBO J 2000; 19: 800–806
- Fotidias D, Jenner P, Mini T. Structural characterization of two aquaporins isolated from native spinach leaf plasma membrane. J Biol Chem 2001; 276: 1707–1714
- Martínez-Ballesta MC, Diaz R, Martínez V. Different blocking effects of HgCl2 and NaCl on aquaporins of pepper plants. J Plant Physiol 2003; 160: 1487–1492
- North GB, Nobel PS. Heterogeneity in water availability alters cellular development and hydraulic conductivity along roots of a desert succulent. Ann Bot 2000; 85: 247–255
- Hedfalk K, Törnroth-Horsefield S, Nyblom M. Aquaporin gating. Curr Opin Stru Biol 2006; 16: 447–456
- Vera-Estrella R, Barkla BJ, Bohnert HJ. Novel regulation of aquaporins during osmotic stress. Plant Physiol 2004; 135: 2318–2329
- Savage DF, Stroud RM. Structural basis of aquaporin inhibition by mercury. J Mol Biol 2007; 368: 607–617
- Clint KG, Cappiello D, McBride SM. Functional characterization of a putative aquaporin from Encephalitozoon cuniculi, a microsporidia pathogenic to humans. Int J Parasitol 2006; 36: 57–62
- Bienert GP, Schjoerring JK, Jahn TP. Membrane transport of hydrogen peroxide. BBA–Biomembranes 2006; 1758: 994–1003
- Harvengt P, Vlerick A, Fuks B. Lentil seed aquaporins form a hetero-oligomer which is phosphorylated by a Mg2 + -dependent and Ca2 + -regulated kinase. Biochem J 2000; 352: 183–190
- Azad AK, Sawa Y, Ishikawa T. Phosphorylation of plasma membrane aquaporin regulates temperature dependent opening of tulip petals. Plant Cell Physiol 2004; 45: 608–617
- Guenther JF, Roberts DM. Water-selective and multifunctional aquaporins from Lotus japonious nodules. Planta 2000; 210: 741–748
- Fetter K, Van Wilder V, Moshelion M. Interactions between plasma membrane aquaporins modulate their water channel activity. Plant Cell 2004; 16: 215–228
- Steudle E, Zimmermann U, Zillikens J. Effect of cell turgor on hydraulic conductivity and elastic modulus of Elodea leaf cells. Planta 1982; 154: 371–380
- Hohmann S, Bill RM, Kayingo G. Microbial MIP channels. Trends Micro 2000; 8: 33–38
- Wan X, Steudle E, Hartung W. Gating of water channels (aquaporins) in cortical cells of young corn roots by mechanical stimuli (pressure pulses): Effects of ABA and of HgCl2. J Exp Bot 2004; 55: 411–422
- Hejnowics Z, Sievers A. Reversible closure of water channels in parenchymatic cells of sunflower hypocotyl depends on turgor status of the cells. J Plant Physiol 1996; 147: 516–520
- Ye Q, Wiera B, Steudle E. A cohesion/tension mechanism explains the gating of water channels (aquaporins) in Chara internodes by high concentration. J Exp Bot 2004; 55: 449–461
- Azaizeh H, Gunse B, Steudle E. Effects of NaCl and CaCl2on water transport across root cells of maize (Zea mays L.) seedlings. Plant Physiol 1992; 99: 886–894
- Carvajal M, Cooke DT, Clarkson DT. Responses of wheat plants to nutrient deprivation may involve the regulation of water-channel function. Planta 1996; 199: 372–381
- Martinez-Ballesta MC, Martinez V, Carvajal M. Regulation of water channel activity in whole roots and in protoplasts from roots of melon plants grown under saline conditions. Aust J Plant Physiol 2000; 27: 685–691
- Tournaire-Roux C, Sutka M, Javot H. Cytosolic pH regulates root water transport during anoxic stress through gating of aquaporins. Nature 2003; 425: 393–397
- Németh-Cahalan K, Hall JK. pH and calcium regulate the water permeability of aquaporins. J Biol Chem 2000; 275: 6777–6782
- Gunsé B, Poschenrieder C, Barceló J. Water transport properties of roots and root cortical cells in proton- and Al-sensitive maize varieties. Plant Physiol 1997; 113: 595–602
- Maggio A, Joly RJ. Effects of mercuric chloride on the conductivity of tomato root system. Plant Physiol 1995; 109: 331–335
- Wan X, Zwiazek JJ. Mercuric chloride effects on root water transport in aspen seedlings. Plant Physiol 1999; 121: 939–946
- Barone LM, Shih C, Wasserman BP. Mercury-induced conformational changes and identification of conserved surface loops in plasma membrane aquaporins from higher plants. J Biol Chem 1997; 272: 30672–30677
- Kiegle E, Moore CA, Haseloff J. Cell-type-specific calcium responses to drought, salt and cold in the Arabidopsis root. Plant J 2000; 23: 267–278
- Alleva K, Niemietz CM, Sutka M. Plasma membrane of Beta vulgaris storage root shows high water channel activity that is regulated by cytoplasmic pH, and a dual range of calcium concentrations. J Exp Bot 2006; 57: 609–621
- Lemieux MJ. Eukaryotic major facilitator superfamily transporter modeling based on the prokaryotic G1p T crystal structure (Review). Mol Mem Biol 2007; 24: 333–341
- Qiu QS, Wang ZZ, Zhang N, Cai QG. Aquaporins in the plasma membrane of leaf callus protoplasts of Acnitia deliciosa var. deliciosa cv. Hayward. Aust J Plant Physiol 2000; 27: 71–75
- Ren G, Cheng A, Melnyk P. Polymorphism in the packing of aquaporin-1 tetramers in 2-D crystals. J Struc Biol 2000; 130: 45–53
- Fu D, Libson A, Miercke LJW. Structure of a glycerol-conducting channel and the basis for its selectivity. Science 2000; 290: 481–486
- Zhu CF, Schraut D, Hartung W. Differential responses of maize MIP genes to salt stress and ABA. J Exp Bot 2005; 56: 2971–2981
- Quintero JM, Fournier JM, Benlloch M. Water transport in sunflower root systems: Effects of ABA, Ca2 + status and HgCl2. J Exp Bot 1999; 50: 1607–1612
- Hose E, Steudle E, Hartung W. Abscisic acid and hydraulic conductivity of maize roots: A study using cell- and root-pressure probes. Planta 2000; 211: 874–882
- Kaldenhoff R, Kölling A, Richter G. A novel blue light- and abscisic acid-inducible gene of Arabidopsis thaliana encoding an intrinsic membrane protein. Plant Mol Biol 1993; 23: 1187–1198
- Liu JW, Wakayama Y, Inoue M. Immunocytochemical studies of aquaporin 4 in the skeletal muscle of mdx mouse. J Neurol Sci 1999; 164: 24–28
- Mariaux JB, Bockel C, Salamini F. Desiccation-and abscisic acid-responsive genes encoding major intrinsic proteins (MIPs) from the resurrection plant Craterostigma Plantagineum. Plant Mol Biol 1998; 38: 1089–1099
- Siefritz F, Biela A, Eckert M. The tobacco plasma membrane aquaporin NTAQP1. J Exp Bot 2001; 52: 1953–1957
- Suga S, Komatsu S, Maeshima M. Aquaporin isoforms responsive to salt and water stresses and phytohormones in radish seedlings. Plant Cell Physiol 2002; 43: 1229–1237
- Morillon R, Catterou M. Brassinolide may control aquaporin activities in Arabidopsis thaliana. Planta 2001; 212: 199–204
- Woltering EJ. Interorgan translocation of 1-aminocyclopropane-1-carboxylic acid and ethylene coordinates senescence in emasculated Cymbidium flowers. Plant Physiol 1990; 92: 837–845
- Kamaluddin M, Zwiazek JJ. Ethylene enhances water transport in hypoxic aspen. Plant Physiol 2002; 128: 1–8
- Zhao CX, Chang-Xing, Deng XP, Zhang SQ. 2004. Advances in the studies on water uptake by plant roots. Acta Bot Sin 46:505–514.
- Bohnert HJ, Nelson DE, Jensen RG. Adaptations to environmental stresses. Plant Cell 1995; 7: 1099–1111
- Kirch HH, Vera-Estrella R, Golldack D. Expression of water channel proteins in Mesembryanthemum crystallinum. Plant Physiol 2000; 123: 111–124
- Kawasaki S, Borchert C, Deyholos M. Gene expression profiles during the initial phase of salt stress in rice. Plant Cell 2001; 13: 889–905
- Yamaguchi-Shinozaki K, Koizumi M, Urao S. Molecular cloning and characterization of 9 cDNAs for genes that are responsive to desiccation in Arabidopsis thaliana: Sequence analysis of one cDNA clone that encodes a putative transmembrane channel protein. Plant Cell Physiol 1992; 33: 217–224
- Yamada S, Katsuhiro M, Kelly WB. A family of transcripts encoding water channel protein: Tissue-specific expression in the common ice plant. Plant Cell 1995; 7: 1129–1142
- Grote K, Trzebiatovski PV, Kaldenhoff R. RNA levels of plasma membrane aquaporins in Arabidopsis thaliana. Protoplasma 1998; 204: 139–144
- Lu Z, Neumann PM. Water stress inhibits hydraulic conductance and leaf growth in rice seedlings but not the transport via mercury-sensitive water channels in the root. Plant Physiol 1999; 120: 143–151
- Aharon R, Shahak Y, Wininger S. Overexpression of a plasma membrane aquaporin in transgenic tobacco improves plant vigor under favorable growth conditions but not under drought or salt stress. Plant Cell 2003; 15: 439–447
- Smart LB, Moskal WA, Cameron KD. MIP genes are down-regulated under drought stress in Nicotiana glauca. Plant Cell Physiol 2001; 42: 686–693
- Boursiac Y, Chen S, Luu D-T. Early effects of salinity on water transport in Arabidopsis roots – molecular and cellular features of aquaporin expression. Plant Physiol 2005; 139: 790–805
- Lopez F, Bousser A, Sissoeff I. Diurnal regulation of water transport and aquaporin gene expression in maize roots: Contribution of PIP2 proteins. Plant Cell Physiol 2003; 44: 1384–1395
- Nakashima K, Yamaguchi-Shinozaki K. Regulons involved in osmotic stress-responsive and cold stress-responsive gene expression in plants. Physiol Plant 2006; 126: 62–71
- Bohnert HJ, Jensen RG. Strategies for engineering water-stress tolerance in plants. Trends Biotech 1996; 14: 89–97
- Noctor G. Metabolic signaling in defence and stress: The central roles of soluble redox couples. Plant Cell Environ 2006; 29: 409–425
- Chaves MM, Maroco J, Pereira J. Understanding plant responses to drought – from genes to the whole plant. Funct Plant Biol 2003; 30: 239–264
- Clarkson DT, Carvajal M, Henzler T. Root hydraulic conductance: Diurnal aquaporin expression and the effects of nutrient stress. J Exp Bot 2000; 51: 61–70
- Postaire O, Verdoucq L, Maurel C. Aquaporins in plants: From molecular structures to integrated functions. Adv Bot Res 2007; 47: 1–68
- Tmrnroth-Horsefield S, Wang Y, Hedfalk K, et al. Structural mechanism of plant aquaporin gating. Nature 2006; 439: 688–694
- Shao HB, Liang ZS, Shao MA. LEA protein in higher plants: Structure, functions and gene expression regulation. Biointerfaces 2005; 45: 131–135
- Shao HB, Jiang SY, Li FM, Chu LY, Zhao CX, Shao MA, Zhao XN, Li F. Some advances in plant stress physiology and their implications in the systems biology era. Biointerfaces 2007; 54: 33–36
- Wu G, Zhang C, Chu LY, Shao HB. 2007. Responses of higher plants to abiotic stresses and agricultural sustainable development. J Plant Interactions 4:132–147 (invited Review).
- Shao HB, Chu LY, Lu ZH, Kang CM. Main antioxidants and redox signaling in higher plant cells. Int J Biol Sci 2008; 4: 12–18
- Beaudette PC, Chlup M, Yee J, Neil Emery RJ. Relationships of root conductivity and aquaporin gene expression in Pisum sativum: Diurnal patterns and the response to HgCl2 and ABA. J Exp Bot 2007; 58: 1291–1300
- Zelazny E, Borst JW, Muylaert M, Batoko H, Hemminga MA, Chaumont F. FRET imaging in living maize cells reveals that plasma membrane aquaporins interact to regulate their subcellular localization. PNAS 2007; 104: 12359–12364
- Wang Y, Tajkhorshid E. Molecular mechanisms of conduction and selectivity in aquaporin water channels. J Nutr 2007; 137: 1509–1515