Abstract
The electrophysiological technique of patch-clamp was used to characterize the pore properties of site-directed mutants in the Vibrio cholerae general diffusion porin OmpU. Changes in conductance and selectivity were observed, thus confirming the predicted pore location of these residues, based on homology with the Escherichia coli porins OmpF and OmpC. Some mutants acquire a weak selectivity for cations, which mirrors the properties of the homologous, deoxycholic acid sensitive, OmpT porin of V. cholerae. However, the mutants remain insensitive to deoxycholic acid, like wildtype OmpU. This result suggests that channel selectivity is not an important determinant in the sensitivity to this drug, and is in agreement with our finding that the neutral deoxycholic acid, and not deoxycholate, is the actual active form in channel block. Modifications in the kinetics of spontaneous closures were also noted, and are similar to those found for the E. coli channels. In addition, mutants at the D116 residue on the L3 loop display marked transitions to sub-conductance states. The results reported here are compared to a phenotypical characterization of the mutants in terms of permeability to maltodextrins and β-lactam antibiotic sensitivity. No strict correlations are observed, suggesting that distinct, but somewhat overlapping, molecular determinants control electrophysiological properties and substrate permeability.
Introduction
The general diffusion porins of Gram-negative bacteria form water-filled channels in the outer membrane, and facilitate the diffusion of nutrients, metabolites and signaling molecules in and out of the cell Citation[1], Citation[2]. High resolution crystal structures of many different types of porins have been published over the years (for reviews, see Citation[3–5]). General diffusion porins have a trimeric quaternary structure, and each monomer forms a β-barrel with a single central pore. In all of the crystal structures solved to date, porins possess rather short periplasmic turns connecting the beta strands while the loops on the extracellular side tend to be significantly longer, with the size of each loop varying from one porin to another. The pores contain a constriction zone formed from the inward fold of one of these long extracellular loops, generally loop 3, and this region has a diameter varying from 1–2 nm, with a characteristic separation of charge across the constriction zone. The barrel wall usually contains a cluster of basic residues, while the L3 loop generally has several acidic residues. This segregation of charges sets up an electric field across the pore Citation[6], and the specific spatial placement of these charges is thought to be a major determinant of the characteristic properties of the channel.
The generation of numerous mutants, along with some crystal structures of the mutated pores and functional studies, has allowed some relationships between porin function and structure to be revealed for the Escherichia coli porins OmpF, OmpC and PhoE (reviewed in Citation[5]). For OmpF and OmpC, a clear relationship between selectivity and the charge constellation at the constriction zone has been established. For those mutants in which a positive charge was removed at the constriction zone, the cationic selectivity was increased as would be expected due to less repulsion of permeating cations. Conversely, removing a negative charge from the constriction zone resulted in cationic selectivity decreased. Effects on conductance have been less robust, as expected since conductance is a reflection of not only pore diameter, but also of the thermodynamics and electrostatics of the interactions between the channel wall and the permeating ions Citation[7]. Computational studies on porins have established the importance of charged residues of the constriction zone in determining conductance Citation[8], Citation[9].
Mutations at the constriction zone also affect voltage dependence and the spontaneous oscillations of the channel between open and closed forms, and between states of various conductance. In E. coli OmpF and OmpC, mutations of the basic amino acid cluster leads to increased voltage sensitivity Citation[10–12]. Although a few hypotheses have been presented Citation[13], a molecular basis for the voltage-dependence of these porins is still unknown. There have been several accounts of mutations affecting the kinetic fluctuations of the channels between various states. In some cases, the disruption of interactions at the tip or at the root of the L3 loop with adjacent barrel residues appear to destabilize the L3 loop and possibly allow motion of the loop across the pore Citation[14], Citation[15]. In other cases, changes in the electrostatic profile of the constriction zone have been suggested to be at the root of the kinetic fluctuations Citation[16].
In the past few years, our attention has been devoted to the properties of the OmpU and OmpT porins of the human pathogen, Vibrio cholerae, the agent of cholera.
The expression of these trimeric porins is differentially regulated in cells by the transcriptional regulator ToxR under the control of environmental factors Citation[17], Citation[18], such that OmpU is believed to be expressed preferentially over OmpT when the bacteria are inside the host. Although neither porin is required for virulence Citation[19], the presence of OmpU allows a better colonization of the gut and production of virulence factors than that of OmpT Citation[20]. In addition, OmpT confers increased sensitivity to bile acids relative to OmpU Citation[20]. Altogether these observations suggest that the production of OmpU inside the host, in place of OmpT, allows for a better survival and pathogenic ability in the gut environment. Whether or not these features depend on the pore characteristics of each porin remains to be determined. We have shown that OmpU and OmpT indeed differ widely in their overall functional properties, such as gating kinetics, selectivity, voltage-dependence, sensitivity to deoxycholic acid and acidic pH Citation[21–23].
No crystal structure exists for the V. cholerae general diffusion porins, although the 3-D structure of the V. cholerae VceE, the outer membrane component of the tripartite drug efflux pump is known Citation[24]. In an attempt to determine if the pore of OmpU is constructed on a similar motif as that of E. coli OmpF, and in the hope to generate mutants in OmpU that confer OmpT-like properties, we obtained a homology model of OmpU Citation[25], and engineered mutations at critical residues of the constriction zone, based on the mutational studies on E. coli porins. Our results show that such mutations indeed alter the electrophysiological parameters of OmpU, in some cases in a manner that is different from similar mutations of homologous residues in OmpF.
Materials and methods
Strains and plasmids
The ompU WT or mutant genes were expressed in the porin deficient V. cholerae strain KKV884 Citation[19]. The pBAD24 plasmid was used for all constructs except for the D116A mutant which expressed better from the pNL9 plasmid Citation[25].
Chemicals and media
Luria-Bertani (LB) broth contained 1% tryptone, 1% NaCl, and 0.5% yeast extract. Tryptone and yeast extract were from Difco Laboratories. N-Octyl-oligo-oxyethylene (octyl-POE) was purchased from Axxora. Other chemicals were from Sigma.
Buffers
The following buffers were used for the electrophysiological studies: buffer A (150 mM KCl, 10 µM CaCl2, 0.1 mM K-EDTA, 5 mM HEPES pH 7.2), buffer B (Buffer A + 20 mM MgCl2), buffer C (buffer A except with 50 mM KCl). All buffers were filtered through a 0.2 µm filter before use.
Protein purification
Cells were grown in LB broth at 37°C with appropriate antibiotics (0.1 mg/ml ampicillin, 0.1 mg/ml streptomycin) and 0.05% L-arabinose. The proteins were purified as described Citation[21], Citation[23]. Briefly, porin was extracted with 1% octyl-POE and purified by ion exchange chromatography (MonoQ HR 10/10), followed when necessary by size exclusion chromatography (Hiload 26/20 Superdex 200). The purity of the sample was assessed by silver staining and the concentration determined with a BCA assay (Pierce). Unboiled samples showed that the proteins migrated as high molecular weight species on SDS-PAGE, attesting of the trimeric structure of the purified material. Some amount of monomeric species could also be found, due to the SDS-sensitivity of OmpU, as documented Citation[21]. The proportion of monomeric species somewhat varied amongst mutants; in particular D116N and D116A, tended to achieve a monomeric conformation, which was also sometimes detected in electrophysiology experiments (see Results). Samples were kept at −80°C in the elution buffer until use.
Patch clamp electrophysiology
Pure proteins were reconstituted into soybean phospholipids (Phosphatidylcholine Type II-S, from Sigma) at a protein:lipid ratio of 1:2,000 to 1:5,000 (w:w). The experiments were performed on unilamellar blisters formed from liposomes when placed in buffer B Citation[26]. Patch pipettes were filled with Buffer A and had a resistance of ∼10 MΩ. They were brought into contact with the blister membrane to generate a 0.5–1.0 GΩ seal. Patches were excised by air exposure and the bath solution was exchanged for buffer A, or buffer C (selectivity experiments), or buffer A + 0.008% deoxycholic acid (pH 7.2). Currents were recorded in the voltage-clamp mode with an Axopatch 1D amplifier and a CV-4 headstage (Axon Instruments). The current was filtered at 1 KHz and sampled at 1.25 ms intervals and stored on a PC computer using the Acquire software (Bruxton).
Data analysis
All analysis is based on patches containing a single trimer. Dwell time analysis results were generated with Pclamp 9.0 software (Axon instruments), while Po measurements were taken using an in-house developed application. Po was calculated as the ratio of the observed integrated current obtained over a 1-min long recording to the total current expected for the same duration if the current value remained at the fully open level. All graphs were made using Sigmaplot 8.0.
Results
A homology model of OmpU structure was obtained from the structures of the homologous E. coli porins OmpF, OmpC and PhoE Citation[25]. A shows a view of a modeled OmpU monomer from the extracellular side. The four numbered residues were chosen for mutational study on the basis that mutations at homologous residues in OmpF and OmpC (B) lead to marked modifications in the pore properties Citation[5]. The following mutants were prepared by site-directed mutagenesis: R46A, R86A, D116A and D116N, and R134A. Unfortunately, we were unable to purify the R86A mutant in a stable trimeric configuration, and the study of this mutant was not pursued. Two types of mutations were designed at residue D116 to change size and charge independently.
Figure 1. Mutations investigated in this study. (A) Location of the mutated residues in the homology model of OmpU Citation[25], as visualized using the VMD software Citation[37]. (B) Table of homologous residues in V. cholerae OmpU, and E. coli OmpF and OmpC.
![Figure 1. Mutations investigated in this study. (A) Location of the mutated residues in the homology model of OmpU Citation[25], as visualized using the VMD software Citation[37]. (B) Table of homologous residues in V. cholerae OmpU, and E. coli OmpF and OmpC.](/cms/asset/dec95dc9-b71d-4f06-9ffc-72797ceb08bf/imbc_a_345631_f0001_b.gif)
shows typical current recordings obtained from the WT and the 4 mutants at a pipette voltage of +50 mV. As porins are in the open state over 90% of the time, the current dwells at a level corresponding to 3 open monomers, and denoted ‘O3’ in . Spontaneous closures of 1 monomer (labeled ‘C1’) occur occasionally. Changes in the activity patterns are readily observable for the mutants. In particular, mutations at D116 affect the propensity of the channel to close, and closures to the C1 level are more frequent and more prolonged. On the other hand, the R46A appears even less dynamic than WT, and R134A is qualitatively similar to WT. In order to quantify the changes in spontaneous activity, we have measured the open probability Po, and the average dwell times of the fully open state O3 and of the monomeric closure C1. The Po of the WT channel is ∼0.99, and decreases slightly for D116N (Po = 0.87) and D116A (Po = 0.96), but remains close to 1.0 for the other two mutants. However, an analysis of the dwell times in the open and closed states is more revealing of kinetic changes, because short-lived events do not greatly affect the open probability. clearly shows that the two arginine mutants have an average open time comparable to WT while the durations of the closures to the C1 level are much reduced. On the other hand, mutations at the D116 residue have the opposite effect, i.e., a much shorter dwell time at the fully open level due to more frequent interruptions to a closed C1 level, which is itself prolonged, in particular for the D116N mutant.
Figure 2. Electrophysiological behavior. Representative current traces of WT and the investigated mutants were obtained at a pipette potential of +50 mV. ‘O3’ marks the current level through all three open monomers; ‘C1’ marks the current level upon closure of 1 monomer.
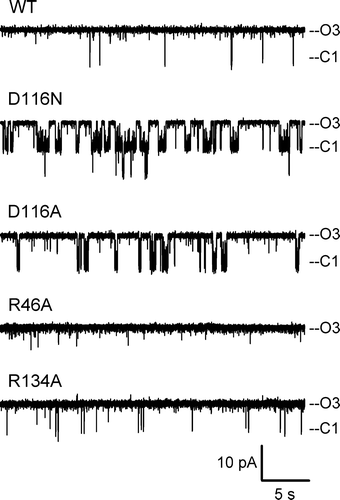
Figure 3. Dwell time analysis. The average (± SEM) dwell times of the current at the O3 and the C1 levels are shown (n=3–5, except for R134A where n=2).
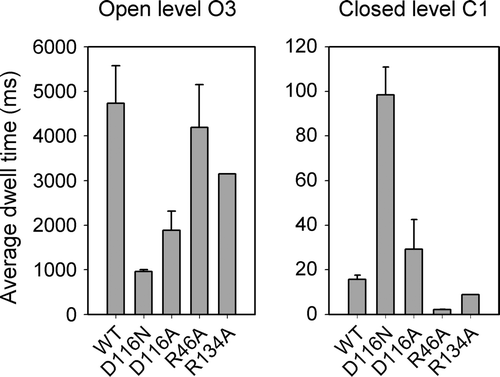
The homology model shown in predicts the pore location of the residues under study, and therefore we expected changes in pore properties such as conductance and selectivity. Monomeric conductance is typically obtained from the current-voltage relationship (I/V plot) of the spontaneous closures to the C1 level. However, due to the paucity of closing events in R46A, it was difficult to make these measurements. Therefore, we opted to measure trimeric conductance instead, given by the value of the current at the O3 level. An I/V plot of these measurements for WT and the four mutants in given in . A regression analysis through the data points for the individual mutants yielded the conductance values given in . Wildtype OmpU and the D116 mutants have similar trimeric conductance of ∼1 nS in 150 mM KCl, but the arginine mutants have a slightly increased value. This may be due to a decreased protrusion of the mutated side chain inside the pore at these locations, although conductance is not strictly correlated with pore dimensions (see Discussion). To quantify selectivity, we performed the I/V plot in asymmetric conditions (150 mM KCl on the pipette side, and 50 mM KCl on the bath side), and obtained the reversal potentials given in . Again, the arginine mutants are distinct from the D116 mutants. Both R46A and R134A become slightly more cation-selective, while the mutation at D116 strongly diminishes cation-selectivity.
Figure 4. Trimeric conductance. Current-voltage plots of the conductance of a single trimer of WT and each of the investigated OmpU mutants. Three to eight patches were used, depending on the protein, but not all voltages were repeated. Error bars (SEM) are given only for data points with at least three measurements.
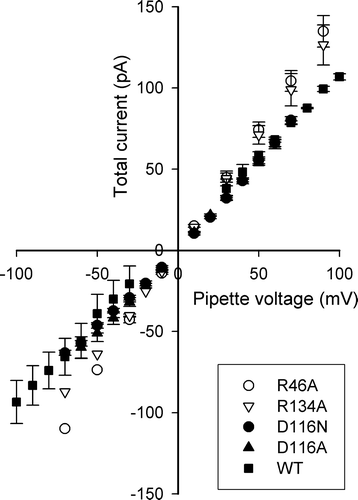
Table I. Electrophysiological parameters obtained for WT and mutant OmpU.
We have reported that the homologous V. cholerae porin OmpT is blocked by low concentrations of the bile component deoxycholic acid, while OmpU is resistant to this compound Citation[22]. Since deoxycholic acid acts as an open channel blocker of OmpT, we wanted to test if some of the pore mutations in OmpU might have conferred sensitivity to deoxycholic acid. In particular the ion selectivity of the D116 mutants of OmpU is similar to that of OmpT Citation[21]. However, we found that all the mutants are still insensitive to the presence of 0.008% deoxycholic acid, just as WT OmpU (data not shown). These results suggest that it is not the cation-selectivity of OmpU that makes it resistant to deoxycholic acid, and are in agreement with our previous finding that it is the neutral form of deoxycholic acid (and not the anion deoxycholate) that is the active blocker of OmpT Citation[22].
In addition to the kinetic changes in the rate and duration of spontaneous closures discussed above, the D116 mutants also showed a propensity at displaying transitions to so-called sub-conductance states, as show in some representative examples in . Here we find that the channel closes to a current level that is only a fraction of the monomeric conductance. Transitions to this level occur as closures from the O3 level, either individually (for ex., the last event of the top trace of D116A) or as a precursor to a full monomeric closure (for ex., the last event of the top trace of D116N), and as re-openings in the midst of a prolonged closure (for ex., the first event of the top trace of D116N). Sub-conductance states are also observed with monomers of D116N (C). Because the D116N mutant is somewhat unstable, the trimers have a tendency to dissociate into monomers, and therefore, it is possible to obtain patches that contain a single monomer. We found that the monomer has the same conductance as the trimer, but has a reduced dwell time at the C1 state (47±9 ms, as opposed to ∼90 ms for the trimer). C shows two segments from the same trace of a D116N monomer (note the different time scale from panels A and B). The dashed line was placed at the same current level as those of panel A, and corresponds to the level reached by the transient reopening in the last event. Note that some even smaller substates are also present individually, or as transition steps to or from the monomeric closure. Sub-conductance states are also observed in WT OmpU, as previously documented Citation[21]; they are, however, less frequent and of short duration. It is possible that the sub-conductance state is more obvious in the D116 mutants because these mutants tend to have closures of longer duration.
Discussion and conclusion
The V. cholerae OmpU porin shares 37% similarity (23% identity) with the E. coli OmpF porin. Many electrophysiological features, such as conductance and overall kinetic behavior, are also similar Citation[21]. For these reasons, it is anticipated that homologous pore-exposed residues control similar functions in channel properties of the two porins. To test this hypothesis, we have engineered mutations in OmpU at selected residues shown to control pore function in OmpF and OmpC Citation[5]. Overall, we have found that the mutations have indeed affected the pore properties of OmpU. Although, we cannot entirely dismiss the possibility that indirect effects (such as through a salt bridge for example) or long-range interactions might be at play, the simplest explanation for our observations – in particular the effects on selectivity – is that these residues are indeed located in the pore, as predicted in the homology model. Mutations at the arginine residues R46 and R134 increase cation-selectivity, and mutations at D116 decrease it. This behavior mirrors what was found for the homologous pore-exposed residues of OmpF and OmpC Citation[27–29]. It is important to keep in mind that the physicochemical rules governing selectivity are quite distinct for large water-filled channels like porins from those applied to small, highly selective channels. Indeed, as discussed by Phale et al. Citation[28], the selectivity of various OmpF mutants is closely related to the ratio of transfer efficiency of cations to anions, and less influenced by differences in hydration of ions or binding wells as in specific channels. Therefore, it has been shown experimentally and confirmed computationally that the removal of positive charges exposed to the pore lumen decreased the repulsion of cations, and therefore enhanced cation-selectivity, while the converse is true for the removal of negative charges exposed to the pore. This also provides a logical and straightforward explanation for our results. It is important to note that Phale and colleagues also showed that mutations at a residue further removed from the pore lumen had no effect on the pore properties Citation[28]. Similarly, Bezrukov and colleagues indicated that the effect of titrable residues on channel selectivity is diminished the further they are from the ionic paths in the channel Citation[30].
An extensive mutational analysis of the three main porins of E. coli, OmpF, OmpC and PhoE, has revealed that effects on conductance are varied and depend of the porin under study, while effects of selectivity are more robust Citation[5]. This is the case here for OmpU as well. Just like for OmpC Citation[11], Citation[31], mutations at the R46 and R134 residues increase conductance; however, mutations at D116 have no effect, as seen in OmpF Citation[28], Citation[29]. As indicated above, effects on selectivity are conserved among OmpF, OmpC and OmpU. These differences in the effects on conductance and selectivity suggest that selectivity is perhaps more sensitive to the nature of the side chains at specific locations than conductance. Despite the conservation of key residues, the overall geometry of the pore is somewhat different between OmpU and OmpF. OmpU is anticipated to be a slightly smaller pore than OmpF, on the basis of the pore dimensions obtained from the homology model (the distance between D113 and R82 of OmpF is 8.2 Å, but only 7.9 Å between D116 and R86 of OmpU), and the fact that it is more cation-selective than OmpF. Vrouenraets and colleagues have shown that OmpF can be made highly cation-selective simply by decreasing the pore dimensions Citation[32]. A correlation between charge distribution and selectivity in porins has been substantiated by experimental and computational studies. For example, OmpF can be turned into a strongly cation-selective channel by decreasing ionic strength Citation[9], Citation[30], but becomes anion-selective at low pH Citation[33]. On the other hand, effects on conductance appear to require more drastic modifications, such as multiple mutations or deletion of part of the L3 loop, as shown for OmpF Citation[28], Citation[29].
Changes in the kinetics of the spontaneous closures due to pore mutations have also been reported for OmpC Citation[16], Citation[27]. Interestingly, the pattern for OmpU and OmpC is similar, with a marked increase in frequency and duration of closures brought forth by mutations of the L3 loop aspartate (D116 for OmpU, D105 for OmpC). The impact of a pore exposed residue on the channel kinetics is somewhat surprising, and points to a role of the L3 loop in spontaneous gating, but the actual molecular basis for this process is still unknown. It is generally believed that the L3 loop is too strongly involved in H-bonding with the barrel wall to allow any gross physical movement of the L3 loop across the pore. However, the current interruptions seen as monomeric closures might be due to subtle conformational changes that change the geometry of the pore and/or the distribution of charges, or structural distortions in the L3 loop itself. This is also true for sub-conductance states, which are observed in OmpF and OmpC as well, and display a modified kinetic behavior upon mutations at the constriction zone Citation[16], Citation[27], Citation[34].
We have previously published a phenotypic characterization of OmpU mutants in terms of bacterial physiological properties Citation[25]. summarizes some of the results from this previous study and the electrophysiological study reported here. Here we are presenting in a qualitative fashion the observations made on these mutants regarding their sensitivity to β-lactam antibiotics known to use general diffusion porins for cell entry, and regarding their ability to grow on maltodextrins as sole carbon source in the absence of maltoporin (maltodextrins have a molecular weight larger than the wildtype porin cut-off and should support growth in the absence of maltoporin only if the mutant porin has an enlarged pore size). We do not intend to reproduce here the data in its full details, which can be found in Reference Citation[25], but a qualitative summary is useful for the discussion below. The side by side comparison of these properties for the different mutants illustrates the difficulty to find a unifying explanation for these observations, and therefore suggests that strict correlations between the conductance properties and the flow of substrates through porins cannot be established. For example, the increase conductance in R46A might correlate with an increased pore size, which in turns helps the entry of maltodextrins. But this is not seen for R134A, which also has an increased conductance. By the same token, an increased pore size would be expected to lead to increased sensitivity to antibiotics. No substantial effects were seen with the R46A and R134A mutants. The D116A mutant appears more resistant to some antibiotics, and this is not correlated with changes in conductance. It is unlikely that the enhanced gating to closed state is responsible for the resistance, because D116N, a channel that is also closed more often than WT, is not more resistant to these antibiotics. We have previously argued that D116 might be part of a binding site for some antibiotics Citation[25], as been shown for the homologous D113 of OmpF by computational modeling Citation[35]. Thus, specific substitutions at the site might have an impact on drug binding, with no other effect on conductance. Our results are in agreement with previous reports that show variability in the correlation between conductance and maltodextrin permeability in OmpF Citation[28], Citation[29], Citation[36]. Altogether, these results indicate that electrophysiological properties, such as conductance and selectivity, and permeability to specific substrates are governed by specific determinants in the pore's geometry and charge configuration with various degrees of overlap.
Table II. Comparison of properties for the different mutants relative to WT.
Acknowledgements
We thank Guillaume Duret and Valérie Simonet for providing some wildtype OmpU data. The support of grant # E-1597 from the Welch Foundation is acknowledged. Declaration of interest: The authors report no conflicts of interest. The authors alone are responsible for the content and writing of the paper.
References
- Nikaido H. Molecular basis of bacterial outer membrane permeability revisited. Microbiol Mol Biol Rev 2003; 67: 593–656
- Nikaido H. Outer membrane. Escherichia coli and Salmonella, cellular and molecular biology, FC Neidhart. ASM Press, Washington, DC 1996; 29–47
- Schulz GE. The structures of general porins. Bacterial and Eukaryotic porins, R Benz. Wiley-Vch Verlag GmbH & Co, Weinheim 2004; 25–40
- Delcour AH. Structure and function of pore-forming beta-barrels from bacteria. J Mol Microbiol Biotechnol 2002; 4: 1–10
- Delcour AH. Solute uptake through general porins. Front Biosci 2003; 8: d1055–1071
- Karshikoff A, Spassov V, Cowan SW, Ladenstein R, Schirmer T. Electrostatic properties of two porin channels from Escherichia coli. J Mol Biol 1994; 240: 372–384
- Hille B. Ionic channels of excitable membranes. Sinauer Associates, Sunderland, MA 1992
- Schirmer T, Phale PS. Brownian dynamics simulation of ion flow through porin channels. J Mol Biol 1999; 294: 1159–1167
- Im W, Roux B. Ion permeation and selectivity of OmpF porin: a theoretical study based on molecular dynamics, Brownian dynamics, and continuum electrodiffusion theory. J Mol Biol 2002; 322: 851–869
- Bishop ND, Lea EJ, Mobasheri H, Spiro S. Altered voltage sensitivity of mutant OmpC porin channels. FEBS Lett 1996; 379: 295–298
- Delcour AH, Adler J, Kung C. A single amino acid substitution alters conductance and gating of OmpC porin of Escherichia coli. J Membr Biol 1991; 119: 267–275
- Van Gelder P, Saint N, Phale P, Eppens EF, Prilipov A, van Boxtel R, Rosenbusch JP, Tommassen J. Voltage sensing in the PhoE and OmpF outer membrane porins of Escherichia coli: role of charged residues. J Mol Biol 1997; 269: 468–472
- Robertson KM, Tieleman DP. Molecular basis of voltage gating of OmpF porin. Biochem Cell Biol 2002; 80: 517–523
- Miedema H, Vrouenraets M, Wierenga J, Eisenberg B, Schirmer T, Basle A, Meijberg W. Conductance and selectivity fluctuations in D127 mutants of the bacterial porin OmpF. Eur Biophys J 2006; 36: 13–22
- Liu N, Delcour AH. The spontaneous gating activity of OmpC porin is affected by mutations of a putative hydrogen bond network or of a salt bridge between the L3 loop and the barrel. Protein Eng 1998; 11: 797–802
- Liu N, Samartzidou H, Lee KW, Briggs JM, Delcour AH. Effects of pore mutations and permeant ion concentration on the spontaneous gating activity of OmpC porin. Protein Eng 2000; 13: 491–500
- Lee SH, Hava DL, Waldor MK, Camilli A. Regulation and temporal expression patterns of Vibrio cholerae virulence genes during infection. Cell 1999; 99: 625–634
- Klose KE. Regulation of virulence in Vibrio cholerae. Int J Med Microbiol 2001; 291: 81–88
- Provenzano D, Lauriano CM, Klose KE. Characterization of the role of the ToxR-modulated outer membrane porins OmpU and OmpT in Vibrio cholerae virulence. J Bacteriol 2001; 183: 3652–3662
- Provenzano D, Klose KE. Altered expression of the ToxR-regulated porins OmpU and OmpT diminishes Vibrio cholerae bile resistance, virulence factor expression, and intestinal colonization. Proc Natl Acad Sci USA 2000; 97: 10220–10224
- Simonet VC, Baslé A, Klose KE, Delcour AH. The Vibrio cholerae porins OmpU and OmpT have distinct channel properties. J Biol Chem 2003; 278: 17539–17545
- Duret G, Delcour AH. Deoxycholic acid blocks Vibrio cholerae OmpT but not OmpU porin. J Biol Chem 2006; 281: 19899–19905
- Duret G, Simonet V, Delcour AH. Modulation of Vibrio cholerae porin function by acidic pH. Channels 2007; 1: 70–79
- Federici L, Du D, Walas F, Matsumura H, Fernandez-Recio J, McKeegan KS, Borges-Walmsley MI, Luisi BF, Walmsley AR. The crystal structure of the outer membrane protein VceC from the bacterial pathogen Vibrio cholerae at 1.8 Å resolution. J Biol Chem 2005; 280: 15307–15314
- Pagel M, Simonet V, Li J, Lallemand M, Lauman B, Delcour AH. Phenotypic characterization of pore mutants of the Vibrio cholerae porin OmpU. J Bacteriol 2007; 189: 8593–8600
- Delcour AH, Martinac B, Adler J, Kung C. Modified reconstitution method used in patch-clamp studies of Escherichia coli ion channels. Biophys J 1989; 56: 631–636
- Liu N. 1999. Structure-function relationships of E. coli OmpC porin – the effects of site-directed mutations on the porin channel function. PhD Thesis, University of Houston, USA.
- Phale PS, Philippsen A, Widmer C, Phale VP, Rosenbusch JP, Schirmer T. Role of charged residues at the OmpF porin channel constriction probed by mutagenesis and simulation. Biochemistry 2001; 40: 6319–6325
- Saint N, Lou KL, Widmer C, Luckey M, Schirmer T, Rosenbusch JP. Structural and functional characterization of OmpF porin mutants selected for larger pore size. II. Functional characterization. J Biol Chem 1996; 271: 20676–20680
- Alcaraz A, Nestorovich EM, Aguilella-Arzo M, Aguilella VM, Bezrukov SM. Salting out the ionic selectivity of a wide channel: the asymmetry of OmpF. Biophys J 2004; 87: 943–957
- Lakey JH, Lea EJ, Pattus F. OmpC mutants which allow growth on maltodextrins show increased channel size and greater voltage sensitivity. FEBS Lett 1991; 278: 31–34
- Vrouenraets M, Wierenga J, Meijberg W, Miedema H. Chemical modification of the bacterial porin OmpF: gain of selectivity by volume reduction. Biophys J 2006; 90: 1202–1211
- Nestorovich EM, Rostovtseva TK, Bezrukov SM. Residue ionization and ion transport through OmpF channels. Biophys J 2003; 85: 3718–3729
- Baslé A, Iyer R, Delcour AH. Subconductance states in OmpF gating. Biochim Biophys Acta 2004; 1664: 100–107
- Vidal S, Bredin J, Pagès JM, Barbe J. Beta-lactam screening by specific residues of the OmpF eyelet. J Med Chem 2005; 48: 1395–1400
- Bredin J, Saint N, Mallea M, D E, Molle G, Pages JM, Simonet V. 2002. Alteration of pore properties of Escherichia coli OmpF induced by mutation of key residues in anti-loop 3 region Biochem J 363:521–528.
- Humphrey W, Dalke A, Schulten K. VMD – Visual Molecular Dynamics. J Mol Graphics 1996; 14: 33–38