Abstract
The effect of phospholipids on the activity of isoform ACA8 of Arabidopsis thaliana plasma membrane (PM) Ca2+-ATPase was evaluated in membranes isolated from Saccharomyces cerevisiae strain K616 expressing wild type or mutated ACA8 cDNA. Acidic phospholipids stimulated the basal Ca2+-ATPase activity in the following order of efficiency: phosphatidylinositol 4-monophosphate>phosphatidylserine>phosphatidylcholine≅phosphatidylethanolamine≅0. Acidic phospholipids increased Vmax-Ca2+ and lowered the value of K0.5-Ca2+ below the value measured in the presence of calmodulin (CaM). In the presence of CaM acidic phospholipids activated ACA8 by further decreasing its K0.5-Ca2+ value. Phosphatidylinositol 4-monophosphate and, with lower efficiency, phosphatidylserine bound peptides reproducing ACA8 N-terminus (aa 1–116). Single point mutation of three residues (A56, R59 and Y62) within the sequence A56-T63 lowered the apparent affinity of ACA8 for phosphatidylinositol 4-monophosphate by two to three fold, indicating that this region contains a binding site for acidic phospholipids. However, the N-deleted mutant Δ74-ACA8 was also activated by acidic phospholipids, indicating that acidic phospholipids activate ACA8 through a complex mechanism, involving interaction with different sites. The striking similarity between the response to acidic phospholipids of ACA8 and animal plasma membrane Ca2+-ATPase provides new evidence that type 2B Ca2+-ATPases share common regulatory properties independently of structural differences such as the localization of the terminal regulatory region at the N- or C-terminal end of the protein.
Introduction
In plant cells cytoplasmic free Ca2+ acts as a second messenger in the transduction of various endogenous and environmental signals. Its concentration is controlled through regulation of a number of passive and active Ca2 + transport proteins localized both at the plasma membrane (PM) and at intracellular membrane systems such as the tonoplast and the endoplasmic reticulum Citation[1], Citation[2]. The plant PM Ca2 + -ATPase plays a central role in the transduction of Ca2 + -mediated signals, in particular by re-establishing the low basal free Ca2 + concentration after a stimulus-induced Ca2 + influx from the extra-cellular environment Citation[3–8].
The PM Ca2 + -ATPase of plant cells is a P-type ATPase pertaining to the type 2B subfamily characterized by the direct interaction with calmodulin (CaM) at a short sequence consisting of 15–30 residues which form an amphipathic α-helix Citation[9–12]. While in the PM Ca2 + -ATPase of animal cells (PMCA) the CaM binding domain is localized at the extended cytosolic C-terminus of the enzyme, plant type 2B Ca2 + -ATPases have a short C-terminus and an extended cytosolic N-terminus containing the CaM binding domain Citation[9–15]. Despite this important structural difference, animal and plant type 2B Ca2 + -ATPases share not only the mechanism of Ca2 + transport in exchange with protons Citation[16–20], but also a number of common regulatory features. In particular, in both cases binding of CaM hampers the action of an auto-inhibitory domain partially overlapping the CaM binding domain, setting the enzyme in an activated state characterized by high Vmax and low K0.5 for free Ca2 + Citation[9–12], Citation[21], Citation[22]. The mechanism of auto-inhibition is not completely understood, but evidence has been provided that both PMCA C-terminus and the N-terminus of ACA8, an isoform of PM Ca2 + -ATPase of Arabidopsis thaliana, interact with a region of the small cytoplasmic loop connecting transmembrane segments 2 and 3 Citation[9], Citation[10], Citation[23–25].
The activity of PMCA is regulated also by acidic phospholipids, which activate the enzyme through a complex mechanism, only partially overlapping that of CaM Citation[9], Citation[10], Citation[26]. In fact, acidic phospholipids are able to stimulate the enzyme activated by CaM or by proteolytic cleavage of its C-terminal auto-inhibitory domain, further lowering its K0.5 for free Ca2 + Citation[27], Citation[28]. Based on these results a model has been proposed according to which the Ca2 + -ATPase has three possible activity states: a basal, low activity, state A and two activated states, B1 and B2 Citation[9], Citation[28]. Binding of CaM or removal of the CaM-binding domain would shift PMCA from state A (low Vmax-Ca2 + , high K0.5-Ca2 + ) to state B1 (high Vmax-Ca2 + , low K0.5-Ca2 + ). State B2 (high Vmax-Ca2 + , very low K0.5-Ca2 + ) would be reached, from state A or state B1, only upon addition of phospholipids. Two lipid-binding sites have been identified in PMCA: One overlaps the CaM binding site while the other is localized within the small cytoplasmic loop connecting the second and the third transmembrane segment Citation[29–31]; binding to the latter site would be responsible of the K0.5-Ca2 + decrease to the value characteristic of state B2 Citation[32].
The Ca2 + -ATPase of plant cell PM is activated by acidic phospholipids with an order of efficiency similar to that observed for PMCA Citation[33]. Acidic phospholipids lower the value of K0.5-Ca2 + of the plant PM Ca2 + -ATPase below that measured upon tryptic cleavage of the CaM-binding domain, suggesting that the mechanism of activation of plant PM Ca2 + -ATPase by acidic phospholipids only partially overlaps with that of CaM. However, nothing is known on the number and localization of phospholipid binding site(s) involved in the activation of the enzyme by acidic phospholipids Citation[33].
In PM vesicles isolated from plant cells a detailed biochemical analysis of Ca2 + -ATPase activity is hampered by its very low abundance as compared to other P type ATPases such as the PM H+-ATPase Citation[34]. Moreover, A. thaliana expresses three isoforms of PM Ca2 + -ATPase Citation[5–8], which may have different regulatory properties. Over-expression of a single isoform of the enzyme in a yeast strain devoid of endogenous Ca2 + -ATPases allows not only to circumvent these problems, but also to use site-directed mutagenesis for structure function analysis Citation[14], Citation[35]. In this work, we have analysed the effect of phospholipids on the activity of wild type and mutant ACA8, the first cloned isoform of A. thaliana PM Ca2 + -ATPase Citation[13], expressed in Saccharomyces cerevisiae Citation[14], Citation[35]. We present evidence that the complex activation of ACA8 by acidic phospholipids results from their interaction with – at least – two different sites, one of which overlaps the CaM-binding site in the N-terminus of the protein.
Materials and methods
Yeast strain, transformation and growth media
Saccharomyces cerevisiae strain K616 (MATa pmr1::HIS3 pmc1::TRP1 cnb1::LEU2, ade2, ura3) was used for expression of ACA8, Δ74-ACA8 and the specified single point ACA8 mutants. Transformed yeast cells were selected for uracil prototrophy on synthetic complete medium lacking uracil (SC-URA) as previously described Citation[35].
Isolation of yeast microsomes and ER membrane fraction
Plant pumps were expressed in yeast grown in SC-URA medium containing 2% (w/v) galactose, 1% (w/v) raffinose, 50 mM succinic acid/Tris (pH 5.5), 0.7% (w/v) yeast nitrogen base and 10 mM CaCl2, for 24 h at 30°C. After growth medium separation by centrifugation, yeast cells were homogenized as reported in ref. Citation[35] with the addition of 1 µg ml−1 of pepstatin and 1 µg ml−1 of chymostatin in the homogenization solution. Microsomes were harvested as previously described Citation[35], Citation[36]. Yeast ER fraction was isolated from microsomes by sucrose gradient centrifugation Citation[35], Citation[36]. Protein concentration was determined using the Bio-Rad assay (Bio-Rad, Hercules, CA, USA; cat. no. 500-001).
Assays of Ca2 + -ATPase activity
Ca2 + -ATPase activity in yeast microsomes or ER-enriched fraction (ca. 4–5 µg of protein per sample) was measured as Ca2 + -dependent MgITP hydrolysis as previously described Citation[35], Citation[36]. Free Ca2 + concentration was buffered at specified concentrations with 1 mM EGTA. Phospholipids were dissolved, dried under N2 flux and micro-dispersed as previously described Citation[33]. The phospholipid suspension was sonicated to clarity Citation[33] and immediately used for membrane treatment. Phospholipids were from biological sources and contained different acyl chains; concentration of phospholipid solutions was computed assuming complete solubilization and taking account of average fatty acid composition. Membrane proteins were pre-incubated in assay buffer plus or minus phospholipids for 20 min at 4°C before starting the reaction by addition of MgITP. When specified, 1 µM bovine brain CaM was supplied. Samples were incubated at 25°C for 60 min. ACA8 hydrolytic activity was determined as the difference between the activities measured in the presence and absence of Ca2 + ; the activity measured in the absence of Ca2 + did not exceed 40 nmol mg−1 min−1. Under the above described conditions the reaction catalysed by ACA8 is linear for up to 90 min (data not shown). All the assays were performed with three replicates and repeated at least three times, with similar results.
Purification of peptides
Production and purification of peptides reproducing the first 116 aminoacids of ACA8 tagged with GST (GST-1M-I116) or 6His (6His-1M-I116) is reported in ref. Citation[15]. Peptide purity exceeded 75%.
Overlay assay
The interaction between phospholipids and the N-terminus of ACA8 was analysed by incubating a membrane spotted with 50 and 250 pmol of different phospholipids with the peptide 6His-1M-I116 or with the peptide GST-1M-I116. After spotting of phospholipids, the 0.2 µm nitrocellulose membrane was dried and saturated for 2 h with 1% (w/v) BSA, 40 mM BTP-Hepes, pH 7. Overlay was performed by incubating the membrane for 2 h at room temperature with the same solution used in the saturation step with the addition of 2.5 µM GST-1M-I116 or 5 µM 6His-1M-I116. Immunodecoration with an antiserum against the portion 17V-T31 of ACA8 was performed as previously described Citation[13] except that the antiserum was diluted 1:5000. Experiments were performed at least three times, with similar results.
Results
Activation of ACA8 by acidic phospholipids
In a first set of experiments we analysed the effect of increasing concentrations of phospholipids on the activity of ACA8 at 40 µM free Ca2 + (): ACA8 activity is hardly affected by phosphatidylcholine (PC) or phosphatidylethanolamine (PE), while it is strongly stimulated by the acidic phospholipids phosphatidylserine (PS) and phosphatidylinositol 4-monophosphate (PI-4P).
Figure 1. Effect of increasing concentrations of different phospholipids on ACA8 hydrolytic activity. Assays were performed on ER enriched fraction from S. cerevisiae expressing ACA8 in presence of 40 µM free Ca2 + . The effect of phospholipids is expressed as percentage of stimulus relative to the activity measured in absence of added phospholipids (82 nmol Pi min−1 mg−1 protein). Results are from one experiment representative of at least three giving similar results. Assays were performed on three replicates and SE of the assay did not exceed 7% of the reported values.
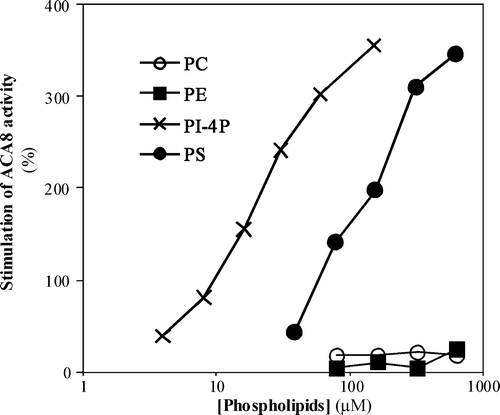
Analysis of the dependence of activation on the concentration of phospholipid in six independent experiments shows that, while maximal stimulation is similar for PS and PI-4P (respectively 465±47% and 433±49%), the apparent affinity for PI-4P is much higher than that for PS: in fact half-maximal stimulation is reached at 30±2 µM PI-4P but at 240±8 µM PS.
ACA8 expressed in Saccharomyces cerevisiae is strongly activated by CaM Citation[14]. Measurements of ACA8 activity as a function of free Ca2 + concentration in the presence and absence of CaM () show that percentage stimulation of ACA8 activity by CaM decreases with the increase of free Ca2 + concentration. Data analysis shows that CaM determines a five fold increase of Vmax-Ca2 + and a moderate decrease of apparent K0.5-Ca2 + ().
Figure 2. Effect of PS on ACA8 hydrolytic activity as a function of free Ca2 + concentration. Assays were performed on ER enriched fraction from S. cerevisiae expressing ACA8 in the presence (squares) or absence (circles) of 600 µM PS and in the presence (open symbols) or absence (closed symbols) of 1 µM CaM. Free Ca2 + concentration was buffered at 2.5–40 µM with 1 mM EGTA. Results (±SE, n=3) are from one experiment representative of three.
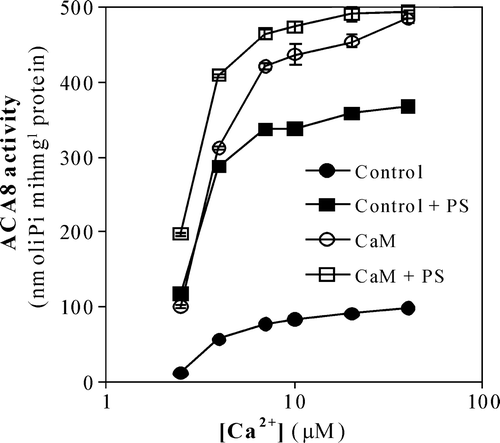
Table I. Effect of PS on the kinetic parameters of wild type ACA8 and of Δ74-ACA8 mutant measured in absence and in presence of 1 µM CaM. Values of Vmax and apparent K0.5 for free Ca2 + were evaluated by regression analysis of Ca2 + -ATPase activity measured as a function of free Ca2 + concentration as in ; r2>0.96. Results (±SE) are from three independent experiments.
shows that the stimulating effect of PS also decreases with the increase of free Ca2 + concentration: 600 µM PS increases Vmax-Ca2 + about four fold and decreases the value of K0.5-Ca2 + well below the value measured in the presence of CaM ().
This result prompted us to investigate the effect of PS on the dependence of ACA8 activity on free Ca2 + concentration in the presence of CaM (). Under these conditions a stimulating effect of PS is evident only at low, non-saturating free Ca2 + concentrations; data analysis shows that in the presence of CaM PS does not affect the value of Vmax-Ca2 + but significantly lowers the value of K0.5-Ca2+, which attains a value similar to that determined in the presence of PS alone ().
Identification of phospholipid binding sites
Activation of PMCA by acidic phospholipids results from lipid binding to two different sites, one of which is localized in the C-terminus and overlaps the CaM-binding domain Citation[29–31]. To test whether acidic phospholipids bind to ACA8 N-terminus, which contains the CaM-binding site, we tested the ability of peptides reproducing the first 116 aa of ACA8 Citation[15], Citation[26] to bind different phospholipids in an overlay experiment. This technique has been successfully used to analyse binding of different fusion proteins to phospholipids because neither GST nor His-tag generate any signal Citation[37–39]. shows that both peptide 6His-1M-I116 and peptide GST-1M-I116 bind PI-4P and, with less efficiency, PS, but barely bind PC.
Figure 3. Binding of phospholipids to ACA8 N-terminus. Different phospholipids (50 and 250 pmol) were spotted onto a nitrocellulose membrane which was then incubated with or without purified His- or GST-tagged N-terminus of ACA8 (6His-1M-I116 and GST-1M-I116) as described in the Experimental Procedures. Bound protein was visualized by immunodecoration with an antiserum directed against the portion 17V-T31 of ACA8. Results are from one experiment representative of five.
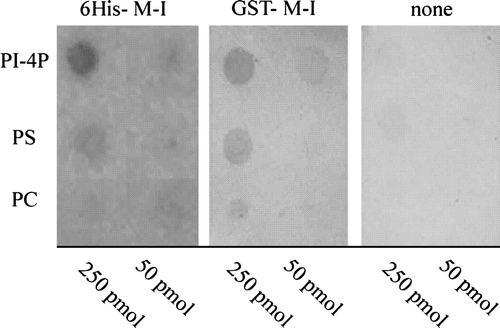
To better localize the site in ACA8 N-terminus which binds acidic phospholipids and check whether it plays a role in enzyme activation, we analysed the effect of increasing concentrations of PI-4P on the activity of single point ACA8 mutants obtained by alanine scanning mutagenesis of the aa stretch A56-T63, within the CaM-binding domain Citation[14]. The results reported in show that three of these mutants, namely mutants A56S, R59A and Y62A, are half-saturated by PI-4P concentrations two to three fold higher than that required by the wild type enzyme.
Figure 4. Effect of increasing concentrations of PI-4P on the activity of single point ACA8 mutants. Assays were performed on microsomal fraction from S. cerevisiae expressing wild type or single point ACA8 mutants in the presence of 10 µM free Ca2 + . Enzyme activation (f/fmax) is expressed as the ratio between PI-4P-dependent stimulus of Ca2 + -ATPase activity at the indicated PI-4P concentration and maximal stimulation (fmax) evaluated at saturating PI-4P concentration. Values of fmax ranged between 56% for the most deregulated mutant (R58A) (14) and 383% (wild type). Results are from one experiment representative of three giving similar results. Assays were performed on three replicates and SE of the assay did not exceed 5% of the reported values.
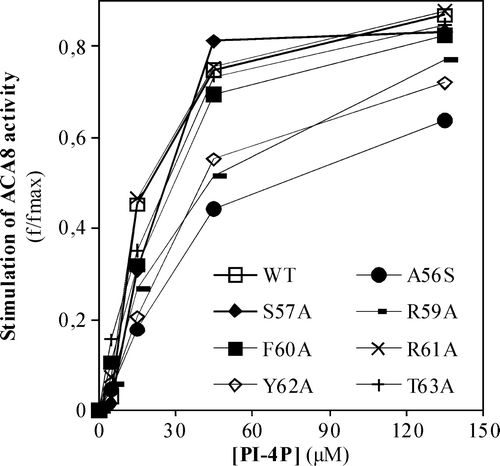
If acidic phospholipids bind only to a site overlapping the CaM-binding domain, the N-deleted mutant Δ74-ACA8, which lacks the first 74 aa containing the CaM-binding domain Citation[13–15], Citation[35] should be insensitive to them. However, results reported in show that Δ74-ACA8 is activated both by PS and by PI-4P. Results reported in also show that the apparent affinities for PS or PI-4P of Δ74-ACA8 and of the wild type enzyme in the presence of CaM are markedly higher than that of the wild type enzyme in the basal, auto-inhibited state: In three independent experiments half-maximal stimulation of the wild type enzyme in the presence of CaM and of Δ74-ACA8 was observed at 7±2 PI-4P and at 65±9 (wild type ACA8) or 80±7 µM (Δ74-ACA8) PS.
Figure 5. Effect of increasing concentrations of PS (panel A) or PI-4P (panel B) on the activity of N-deleted mutant Δ74-ACA8. Assays were performed on ER enriched fraction from S. cerevisiae expressing wild type or Δ74-ACA8 in the presence of 5 µM free Ca2 + and in the presence or in the absence of 1 µM CaM. Enzyme activation (f/fmax) is expressed as the ratio between phospholipid-dependent stimulus of Ca2 + -ATPase activity at the indicated phospholipid concentration and maximal stimulation (fmax) evaluated at saturating phospholipid concentration. Values of fmax of wild type, wild type in presence of CaM and Δ74-ACA8 were respectively 637%, 29% and 72% (PS, panel A) and 547%, 39% and 67% (PI-4P, panel B). Results (±SE, n=3) are from one experiment representative of three.
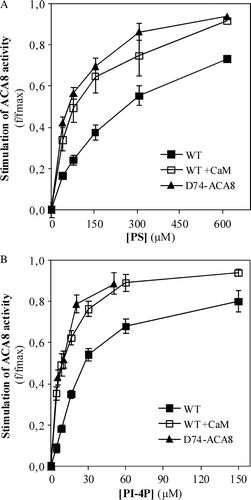
Analysis of the effect of PS on the dependence of Δ74-ACA8 activity on free Ca2 + concentration indicates that, as for the wild type enzyme in the presence of CaM, PS hardly affects Vmax-Ca2 + but significantly lowers the value of K0.5-Ca2 + ().
Discussion
The results reported in this paper show that ACA8, an A. thaliana PM Ca2 + -ATPase isoform, is activated by acidic phospholipids. The efficiency of the tested phospholipids (PI-4P > PS > PC≅0) matches that reported for PMCA, as well as for radish PM Ca2 + -ATPase Citation[33], Citation[40] and suggests a correlation between the number of negative charges on the phospholipids and their ability to activate the PM Ca2 + -ATPase. A direct comparison between concentrations of phospholipids required for half-maximal activation of Ca2 + -ATPase in our experiments performed on membrane vesicles with the slightly lower concentrations required for half-maximal activation of solubilised and purified PMCA Citation[26], Citation[27], Citation[30] is unfeasible. Moreover, effective concentrations of applied phospholipid solutions, computed assuming complete solubilization, cannot give a reliable estimate of the protein affinity for membrane localised phospholipids Citation[30]. Regulation of the PM Ca2 + -ATPase activity by phospholipids, and in particular by phosphatidylinositol phosphates, may be relevant from a physiological point of view. In fact, several pieces of evidence indicate that also in plants phosphatidylinositol metabolism is involved in the transduction of endogenous and environmental stimuli Citation[41–43].
ACA8 activation by acidic phospholipids results from binding to different binding sites. Acidic phospholipids bind to the N-terminus of ACA8 and analysis of the affinity of ACA8 single point mutants for PI-4P indicates that the binding site is localized in the same region of ACA8 N-terminus which contains the auto-inhibitory domain and the CaM binding domain. However, Δ74-ACA8 mutant, in which this region is deleted Citation[14], Citation[35], is activated by acidic phospholipids, indicating that acidic phospholipids activate ACA8 also through a different mechanism.
Indeed, the mechanism of ACA8 activation by acidic phospholipids is dual. In the absence of CaM, acidic phospholipids strongly stimulate ACA8 activity determining both an increase of Vmax-Ca2 + and a marked decrease of K0.5-Ca2 + , the value of which becomes lower than that measured in the presence of CaM. In the presence of CaM, as well as on the Δ74-ACA8 mutant, PS does not affect Vmax-Ca2 + and only determines a further decrease of K0.5-Ca2 + .
The simplest interpretation of these results is that binding of acidic phospholipids to the N-terminus binding site activates the enzyme by hampering the effect of the auto-inhibitory region, similar to CaM. In an helical wheel projection of ACA8 N-terminal regulatory region () the three residues (A56, R59 and Y62) that when mutated determine a two-three fold decrease of the affinity for PI-4P localize in the side of the α-helix which contains the auto-inhibitory domain Citation[14]. It is thus highly likely that binding of acidic phospholipids to this side of the α-helix would hamper the intramolecular interaction of this region which leads to auto-inhibition, determining an increase of Vmax-Ca2 + and a decrease of K0.5-Ca2 + , similar to those induced by CaM binding to the same region. On the other hand, the very low value of K0.5-Ca2 + in the presence of acidic phospholipids would result from their interaction with a different site, which might have a higher affinity for phospholipids: In fact, PS or PI-4P concentrations required for half-maximal stimulation of ACA8 in the presence of CaM and of the Δ74-ACA8 mutant are about one third of that required for ACA8 activation in the absence of CaM.
Figure 6. Helical wheel projection of ACA8 CaM-binding domain. A helical wheel projection of aa stretch R43-K68 of ACA8 was made using the program Binding Site Analysis in The Calmodulin Target Data Base. Residues involved in phospholipid binding are shown in bold; residues involved in auto-inhibition are underlined and those important in CaM recognition are in grey.
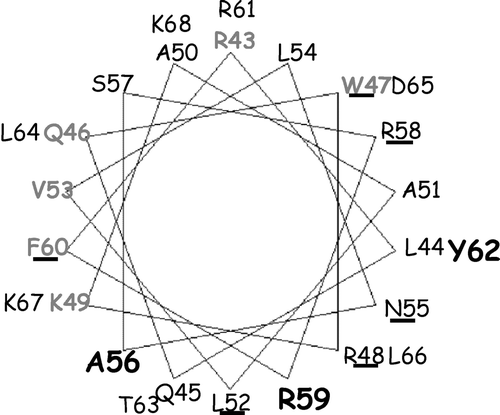
The mechanism of activation of ACA8 by acidic phospholipids is strikingly similar to that described for PMCA Citation[9], Citation[28]. In particular, in both cases acidic phospholipids hamper the auto-inhibitory action of the terminal domain by binding the same region bound by CaM, shifting the enzyme from state A to state B1 Citation[9], Citation[28–31]. This result, together with the previously reported observation that the N-terminus of ACA8 binds to a region of the small cytoplasmic loop connecting transmembrane segments 2 and 3 homologous to that bound by PMCA C-terminus Citation[23–25], strongly suggests that the terminal regulatory region of type 2B Ca2 + -ATPases exerts its auto-inhibitory action through a same mechanism independently of its localization at the C- or N-terminal end of the protein. Indeed, a PMCA mutant obtained by moving the C-terminal region to the N-terminus of the protein maintains auto-regulatory properties similar to those of the wild type Citation[44].
As for PMCA, ACA8 transition to state B2 Citation[9], Citation[28] requires also binding of acidic phospholipids to a different, high affinity site as yet unidentified. Although we cannot exclude that this effect of acidic phospholipids on ACA8 activity is due to a change in membrane structure and fluidity, the striking similarity between the response of ACA8 and that of PMCA suggests the possibility that two distinct phospholipid binding sites are present also in ACA8. In PMCA the second phospholipid binding site has been localized at the terminal end of the small cytoplasmic loop: its deletion produces an enzyme with low K0.5-Ca2 + and low Vmax-Ca2 + , which is still able to respond to CaM with the increase of Vmax-Ca2 + , suggesting that in the absence of acidic phospholipids this region would be responsible of maintaining PMCA in a state of low affinity for calcium Citation[32]. Unfortunately, alignment of ACA8 with PMCA and other P-type ATPases indicates that the phospholipid-binding domain identified between the second and the third transmembrane segment of PMCA is localized in an insertion not conserved in the plant type 2B Ca2 + -ATPases. Thus, further work is needed to ascertain if and where ACA8 contains a second binding site for acidic phospholipids.
Acknowledgements
This project was supported by the Italian Ministry for University and Research in the PRIN 2005 and PRIN 2007 frame. Plasmids encoding ACA8 single point mutants were kindly provided by Dr Lone Bækgaard, KVL, Copenhagen (Denmark). Declaration of interest: The authors report no conflicts of interest. The authors alone are responsible for the content and writing of the paper.
References
- White PJ, Broadley MR. Calcium in plants. Ann Bot 2003; 92: 487–511
- Hetherington AM, Brownlee C. The generation of Ca2 + signals in plants. Annu Rev Plant Biol 2004; 55: 401–427
- Beffagna N, Romani G, Sforza MC. H+ fluxes at plasmalemma level: in vivo evidence for a significant contribution of the Ca2 + -ATPase and for the involvement of its activity in the abscisic acid-induced changes in Egeria densa leaves. Plant Biol 2000; 2: 168–175
- Romani G, Bonza MC, Filippini I, Cerana M, Beffagna N, De Michelis MI. Involvement of the plasma membrane Ca2 + -ATPase in the short term response of Arabidopsis thaliana cultured cells to oligogalacturonides. Plant Biol 2005; 6: 192–200
- Shiøtt M, Romanowsky SM, Bækgaard L, Keyed Jakobsen M, Palmgren MG, Harper JF. A plasma membrane Ca2 + Pump is required for normal pollen tube growth and fertilization. Proc Natl Acad Sci USA 2004; 101: 9502–9507
- Shiøtt M, Palmgren MG. Two plant Ca2 + pumps expressed in stomatal guard cells show opposite expression patterns during cold stress. Physiol Plant 2005; 124: 278–283
- Cerana M, Bonza MC, Harris R, Sanders D, De Michelis MI. Abscisic acid stimulates the expression of two isoforms of plasma membrane Ca2 + -ATPase in Arabidopsis thaliana seedlings. Plant Biol 2006; 8: 572–578
- George L, Romanowsky SM, Harper JF, Sharrock RA. The ACA10 Ca2 + -ATPase regulates adult vegetative development and inflorescence architecture in Arabidopsis. Plant Physiol 2008; 146: 716–728
- Carafoli E. Calcium pump of the plasma membrane. Physiol Rev 1991; 71: 129–153
- Carafoli E. Biogenesis: Plasma membrane calcium ATPase: 15 years of work on the purified enzyme. FASEB J 1994; 8: 993–1002
- Geisler M, Axelsen K, Harper JF, Palmgren MG. Molecular aspects of higher plant P-type Ca2 + -ATPases. Biochim. Biophys. Acta 2000; 1465: 52–78
- Bækgaard L, Fuglsang AT, Palmgren MG. Regulation of plant plasma membrane H+- and Ca2 + -ATPases by terminal domains J Bioenerg Biomembr 2005; 37: 369–374
- Bonza MC, Morandini P, Luoni L, Geisler M, Palmgren MG, De Michelis MI. Cloning of At-ACA8, a plasma membrane localized, calmodulin regulated Calcium-ATPase of Arabidopsis thaliana and localization of its calmodulin binding domain at the N-terminus. Plant Physiol 2000; 123: 1495–1505
- Bækgaards L, Luoni L, De Michelis MI, Palmgren MG. The plant plasma membrane Ca2 + pump ACA8 contains overlapping as well as physically separated autoinhibitory and calmodulin-binding domains. J Biol Chem 2006; 281: 1058–1065
- Luoni L, Bonza MC, De Michelis MI. Calmodulin/Ca2 + -ATPase interaction at the Arabidopsis thaliana plasma membrane is dependent on calmodulin isoform showing isoform-specific Ca2 + dependencies. Physiol Plant 2006; 126: 175–186
- Niggli V, Sigel E, Carafoli E. The purified Ca2 + pump of human erythrocytes catalyzes an electroneutral Ca2 + /H+ exchange in reconstituted liposomal systems. J Biol Chem 1982; 257: 2350–2356
- Rasi-Caldogno F, Pugliarello MC, De Michelis MI. The Ca2 + -transport ATPase of plant plasma membrane catalyzes an H+/Ca2 + exchange. Plant Physiol 1987; 83: 994–1000
- Hao L, Rigaud JL, Inesi G. Ca2 + /H+ countertransport and electrogenicity in proteoliposomes containing erythrocyte plasma membrane Ca-ATPase and exogenous lipids. J Biol Chem 1994; 269: 14268–14275
- Salvador JM, Inesi G, Rigaud JL, Mata AM. Ca2 + transport by reconstituted synaptosomal ATPase is associated with H+ countertransport and net charge displacement. J Biol Chem 1998; 273: 18230–18234
- Luoni L, Bonza MC, De Michelis MI. H+/Ca2 + exchange driven by the plasma membrane Ca2 + -ATPase of Arabidopsis thaliana reconstituted in proteoliposomes after calmodulin-affinity purification. FEBS Lett 2000; 482: 225–230
- Rasi-Caldogno F, Carnelli A, De Michelis MI. Controlled proteolysis activates the plasma membrane Ca2 + pump of higher plants. Plant Physiol 1993; 103: 385–390
- Olbe M, Sommarin M. The spinach plasma membrane Ca2 + pump is a 120 kDa polypeptide regulated by calmodulin binding to a terminal region. Physiol Plant 1998; 103: 35–44
- Falchetto R, Vorherr T, Brunner J, Carafoli E. The plasma membrane Ca2 + pump contains a site that interacts with its calmodulin-binding domain. J Biol Chem 1991; 266: 2939–2936
- Falchetto R, Vorherr T, Carafoli E. The calmodulin-binding site of the plasma membrane Ca2 + pump interacts with the transduction domain of the enzyme. Protein Sci 1992; 1: 1613–1621
- Luoni L, Meneghelli S, Bonza MC, De Michelis MI. Auto-inhibition of Arabidopsis thaliana plasma membrane Ca2 + -ATPase involves an interaction of the N-terminus with the small cytoplasmic loop. FEBS Lett 2004; 574: 20–24
- Niggli V, Adunyah ES, Carafoli E. Acidic phospholipids, unsaturated fatty acids and limited proteolysis mimic the effect of calmodulin on the purified erythrocyte Ca2 + -ATPase. J Biol Chem 1981; 256: 8588–8592
- Choquette D, Hakim G, Filoteo AG, Plishker GA, Bostwick JR, Penniston JT. Regulation of plasma membrane Ca2 + -ATPases by lipids of the phosphatidylinositol cycle. Biochem Biophys Res Comm 1984; 125: 908–915
- Enyedi A, Flura M, Sarkadi B, Gardos G, Carafoli E. The maximal velocity and the calcium affinity of the red cell calcium pump may be regulated independently J Biol Chem 1987; 262: 6425–6430
- Zvaritch E, James P, Vorherr T, Falchetto R, Modyanov N, Carafoli E. Mapping of functional domains in the plasma membrane Ca2 + pump using trypsin proteolysis. Biochem 1990; 29: 8070–8076
- Brodin P, Falchetto R, Vroherr T, Carafoli E. Identification of two domains which mediate the binding of activating phospholipids to the plasma-membrane Ca2 + pump. Eur J Biochem 1992; 204: 939–946
- Filoteo AG, Enyedi A, Penniston JT. The lipid-binding peptide from the plasma membrane Ca2 + pump binds calmodulin, and the primary calmodulin-binding domain interacts with lipid. J Biol Chem 1992; 267: 11800–11805
- De Tezanos Pinto F, Adamo HP. Deletion in the acidic lipid-binding region of the plasma membrane Ca2 + pump. A mutant with high affinity for Ca2 + resembling the acidic lipid-activated enzyme. J Biol Chem 2002; 277: 12784–12789
- Bonza MC, Luoni L, De Michelis MI. Stimulation of plant plasma membrane Ca2 + -ATPase activity by acidic phospholipids. Physiol Plant 2001; 112: 315–320
- Rasi-Caldogno F, Pugliarello MC, Olivari C, De Michelis MI. Identification and characterization of the Ca2 + - ATPase which drives active transport of Ca2 + at the plasma membrane of radish seedling. Plant Physiol 1989; 90: 1429–1434
- Bonza MC, Luoni L, De Michelis MI. Functional expression in yeast of an N-deleted form of At-ACA8, a plasma membrane Ca2 + -ATPase of Arabidopsis thaliana, and characterization of a hyperactive mutant. Planta 2004; 218: 814–823
- Meneghelli S, Luoni L, De Michelis MI. Heparin stimulates a plasma membrane Ca2 + -ATPase of Arabidopsis thaliana. J Biochem 2008; 143: 253–259
- Deak M, Casamayor A, Currie RA, Downes CP, Alessi DR. Characterization of a plant 3-phosphoinositide-dependent protein kinase-1 homologue which contains a pleckstrin homology domain. FEBS Lett 1999; 451: 220–226
- Stevenson JM, Perera IY, Boss WF. A phospatidylinositol 4-kinase pleckstrin homology domain that binds phosphatidylinositol 4-monophosphate. J Biol Chem 1998; 273: 22761–22767
- Jensen RB, La Cour T, Albrethsen J, Nilesen M, Skriver K. FYVE zinc-finger proteins in the plant model Arabidopsis thaliana: identification of PtdIns3P-binding residues by comparison of classic and variant FYVE domains. Biochem J 2001; 359: 165–173
- Missiaen L, Raeymaekers L, Wuytack F, Vrolix M, De Smedt H, Casteels R. Phospholipid-protein interactions of the plasma-membrane Ca2 + -transporting ATPase. Biochem J 1989; 263: 687–694
- Stevenson JM, Perera IY, Heilmann I, Persson S, Boss WF. Inositol signaling and plant growth. Trends Plant Sci 2000; 5: 252–258
- Meijer HJG, Munnik T. Phospholipid-based signaling in plants. Annu Rev Plant Biol 2003; 54: 265–306
- Wang X. Lipid signaling. Curr Opin Plant Biol 2004; 7: 329–336
- Adamo HP, Grimaldi ME. Functional consequences of relocating the C-terminal calmodulin-binding autoinhibitory domains of the plasma membrane Ca2 + pump near the N-terminus. Biochem J 1998; 331: 763–766