Abstract
The preservation of bone mass relies on adequate proliferation, differentiation, secretion of matrix proteins and rate of apoptosis of the bone-forming osteoblasts. Although growing body of evidence indicates that the transient receptor potential (TRP) channels play important roles in numerous cellular functions, limited information is available about the TRP channels in osteoblasts. Here, we inventoried the gene expression and addressed some roles of the TRP channels in various osteoblast-like cells. The transcripts of canonical TRP (TRPC) channels were revealed for TRPC1, TRPC3, TRPC4 and TRPC6 in human MG-63, SaOS and U2 OS osteoblasts while transcripts for TRPC2, TRPC4, TRPC6 and TRPC7 were observed in the murine MC3T3 osteoblasts. PCR products were shown for the melastatin-related TRP (TRPM) channels TRPM4, TRPM6, TRPM7 and TRPM8 in all cell lines. The TRPM1 was specifically expressed by murine MC3T3 cells while the TRPM3 transcripts were revealed solely in human osteoblast-like cells. Transcripts for TRPV2 and TRPV4 were shown in osteoblastic cells. By interfering RNA approaches, the TRPC1 channels in osteoblasts were shown to be responsible for the capacitative calcium entry (CCE) and for the stimulation of cell proliferation by platelet-derived growth factor. On the other hand, interfering RNA-mediated abrogation of the expression of TRPM7, known as calcium and magnesium channels, resulted in the reduction of both basal and growth factor-stimulated osteoblastic cell proliferation. Our results provide the first complete reference for the gene expression of TRP channels in osteoblasts and point to their importance in cell proliferation.
Introduction
The bone is a dynamic tissue that is continuously being remodelled following two coordinated processes. The osteoclasts are constantly breaking down old bone (known as the resorption process) as the osteoblasts are replacing it with new tissue (termed the bone formation process). The osteoblastic cells ensure bone formation and tissue mineralization through the secretion of bone matrix components (type I collagen and non-collagenous proteins) and also provide factors essential for the differentiation of osteoclasts. By regulating osteoclast differentiation, the osteoblasts not only play a central role in bone formation, but also in the regulation of bone resorption Citation[1]. Therefore, the maintenance of bone remodelling equilibrium relies on the adequate proliferation, migration, differentiation, secretion of matrix proteins and apoptosis rate of the osteoblastic cells.
The cloning and characterization of members of the transient receptor potential (TRP) cation channel family have exploded during recent years, leading to a plethora of data on the roles of TRP channels in a variety of tissues and species (for a review Citation[2]). Nevertheless, few studies have investigated the expression of TRP channels and addressed their roles in osteoblastic cells. All TRP channels contain six putative transmembrane domains, which are thought to assemble as homo- or hetero-tetramers to form the active channels. All TRPs are cation channels, although the permeability for different mono- and divalent cations varies greatly between isoforms. They are activated by a wide range of stimuli including intra- and extracellular messengers, chemical, mechanical and osmotic stress, and some by the filling state of intracellular Ca2+ stores. Based on amino acid homologies, the mammalian TRP channel superfamily has been divided into seven families: TRPC, TRPM, TRPV, TRPML, TRPP, TRPA and TRPN. The TRPC (‘Canonical’) and TRPM (‘Melastatin’) subfamilies consist of seven and eight different channels, respectively (i.e. TRPC1–TRPC7 and TRPM1–TRPM8). The TRPV (‘Vanilloid’) subfamily presently comprises six members (TRPV1–TRPV6). The TRPML (‘Mucolipin’) family comprises three members, and the TRPP (‘Polycystin’) family three channel-like and five non-channel members, respectively. The most recently proposed subfamily, TRPA (‘Ankyrin’), has only one mammalian member, TRPA1, and finally, the TRPN (no mechanoreceptor potential C, or NOMPC) has so far only been detected in Caenorhabditis elegans, Drosophila, and zebra fish. There has been increasing interest in first three families because of their involvement in several human diseases while last four families are yet insufficiently characterized.
Little information is presently available about the expression of TRP channels in bone cells. TRPC3 has been considered as a candidate for mediating store-operated non-selective cation entry into the rat osteoblast-like ROS 17/2.8 cells Citation[3–5], a Ca2+ influx activated by either 1alpha,25-dihydroxy-vitamin D3 (1alpha,25(OH)2D3) or thapsigargin-induced depletion of Ca2+ stores. Recently, we reported the gene expression of numerous TRC channels in human osteoblast-like MG-63 cells and suggested their involvement in the stimulation of osteoblast proliferation by growth factors Citation[6]. While we observed that TRPC channels play important role in the stimulation of osteoblast proliferation, the exact member(s) of the TRPC family was (were) not identified. Also, we have reported the expression of TRPM6 and TRPM7 in human osteoblast-like cells Citation[7]. We demonstrated that TRPM7 channels are involved in the intracellular homeostasis of magnesium and in the basal osteoblast proliferation. Recently, the expression of TRPV4 channels has been shown in osteoblasts and osteoclasts Citation[8]. A physiologic role of TRPV4 in bone adaptation to mechanical stress was proposed as TRPV4 deficient mice were protected from the bone loss induced by unloading. TRPV5 has been shown in human and murine osteoclasts Citation[9], with predominant localization to the ruffled border membrane. In regard to bone metabolism, TRPV5 deficiency leads to an increase in osteoclast size and number, in which bone resorption is non-functional.
In the current study, we have inventoried the expression of TRPC, TRPM and TRPV channels in three well known human osteoblast-like cells, namely MG-63, U2 OS and SaOS, and in the murine MC3T3 osteoblast cell line. In addition, we have addressed the roles of TRPC1 and TRPM7 channels in osteoblast proliferation. The potential involvement of TRP channels in bone metabolism is discussed.
Materials and methods
Cell culture
Osteoblast-like cell lines were from the American Type Culture Collection (ATCC; Rockville, MD, USA). Human osteoblastic cells MG-63 were grown in a 1:1 mixture of phenol-free DMEM/Ham's F12 medium (DMEM/F12; Sigma, Oakville, Ontario, Canada). Human cell lines SaOS and U2 OS were maintained in McCoy's 5A medium (Hyclone, Logan, UT, USA). Murine osteoblast cell line MC3T3 was grown in alphaMEM medium (Sigma). All media were supplemented with 10% fetal bovine serum (FBS; Cansera, Etobicoke, Ontario, Canada), 2 mM L-glutamine (Invitrogen, Burlington, Ontario, Canada) and penicillin/streptomycin solution (Invitrogen). Cells were cultured in 5% CO2 at 37°C and were harvested once a week with a Trypsin-EDTA solution (Invitrogen).
Reverse-transcription polymerase chain reaction
Total RNA was extracted from cells using TriZol (Invitrogen) according to the manufacturer's instructions. Reverse transcription (RT) reactions were carried out with Omniscript RT kit (Qiagen, Mississauga, Ontario, Canada) using hexamers. Amplifications by polymerase chain reaction (PCR) were conducted with Taq PCR core kit (Qiagen) using specific primer sets for human and murine TRP channels (). Each primer was complementary to distinct exons according to GenBank sequences to ensure the specific amplification of transcripts. Briefly, PCR amplifications were carried out for 30–40 cycles according to incubation of 1 min at 94°C, 30 sec at 58°C and 1 min at 72°C. Amplification products were resolved by electrophoresis on 2% agarose gel with ethidium bromide revelation.
Table I. Sequences of the primers used for PCR amplification.
Measurements of intracellular calcium
Cells were cultured in 4-wells Labtek dishes (Nalge Nunc, Naperville, IL, USA) for five days in supplemented media. Cells were then transferred to HEPES-buffered saline solution (mM: 121 NaCl, 5.4 KCl, 0.8 Mg2SO4, 25 HEPES, 1.8 CaCl2 and 6.0 NaHCO3 at pH 7.3) and loaded with 2 µM Fluo-3 AM (Invitrogen) with an equivalent volume of 20% Pluronic F127 (Invitrogen) for 45 min at 37°C in the dark. Thereafter the cells were washed with HEPES-buffered saline solution and the loaded dye was allowed to deesterify for 45 min at room temperature in the dark. Following transfer to a Ca2+-free solution (HEPES-buffered saline solution without CaCl2), additions were made in an open chamber configuration at room temperature. The cells were examined with a laser scanning confocal (Bio-Rad) microscope (Nikon TE300) with an Apochromatic 40× N.A. 1.0 objective lens. Fluorescence was excited by an argon laser at 488nm and emissions were collected with a 515 filter. The release of Ca2+ from endoplasmic reticulum was induced by the addition of 5µM thapsigargin (Sigma), an inhibitor of endoplasmic reticulum Ca2+ pumps. Following addition of Ca2+ to the buffer (final concentration of 1.8 mM), a store-operated influx could be obtained. Inhibition of store-operated channels was accomplished by the addition of 1-[β-[3-(4-methoxyphenyl)propoxy]-4-methoxyphenethy]-1H-imidazole hydrochloride (SKF96365; Sigma) before the addition of Ca2+ to the media. Data were analyzed with the Laser Sharp 2.1T, Time Course 1.0 software. The fluorescence of 30–40 cells per field was analyzed. The individual fluorescence intensities of treated cells were compared to the fluorescence intensity before the stimulation and were used to obtain a mean fluorescence ratio for each experiment. The area under the curve was computed using GraphPad Prism version 4 (GraphPad Software, San Diego CA, US).
Cell proliferation
The cells were cultured for three days in 96-well plates (Sarstedt, Montréal, Québec, Canada) with supplemented media and proliferation was determined by the microtiter tetrazolium (MTT) assay after 48 h of treatment with 10% FBS, 25 ng/ml platelet-derived growth factor BB (PDGF) or culture medium alone. Briefly, 3-(4,5-dimethylthiazol-2-yl)-2,5-diphenyltetrasodium bromide (MTT, Sigma) was added to the media at a final concentration of 0.5 mg/ml. Four hours later, formazan crystals generated by cellular reduction of the MTT reagent were dissolved in dimethylsulfoxide (Sigma) for 30 min at 37°C and the absorbance was determined at 575 nm. Results are expressed as the proliferation of cells from the MTT activity versus initial MTT values (absorbance values from cells at the initial day of treatment).
Interference with siRNA
Small interfering RNAs obtained from Qiagen (Mississauga, Ontario, Canada) were directed against human TRPM7 (si-hTRPM7, SI00119196), murine TRPM7 (si-mTRPM7, SI02694727), human TRPC3 (si-hTRPC3, SI03078775), human TRPC1 (si-hTRPC1, SI00050449) and a non-targeting control (Mock). Transfection of the siRNAs was performed using HiPerFect reagent following the manufacturer's instructions. To examine the role of TRPC1 and TRPM7 in cell proliferation, cells plated in 96-well plates (Sarstedt) were transfected for 48 h, and thereafter used for the measurement of intracellular Ca2+ or incubated in appropriate conditions for 48 h with the addition of MTT for the last 4 h of incubation.
Statistical analysis
Statistical differences were evaluated by ANOVA with Dunnett's post-test using GraphPad Prism 4. A level of p < 0.05 was considered significant.
Results
Gene expression of the TRPC channels in osteoblastic cells
The presence of messenger RNAs for TRPC channels was investigated in various human osteoblastic cell models by RT-PCR using specific primers (). As shown in A, PCR products for the TRPC1 (472 bp), TRPC3 (404 bp with R1 primer in MG-63 and SaOS cells, and 611 bp with R2 primer for U2 OS cells) and TRPC6 (692 bp with F1 primer in MG-63 and SaOS cells, and 824 bp with F2 primer for U2 OS cells) were obtained with the total RNA from human osteoblast-like MG-63, SaOS and U2 OS cells. Interestingly, RT-PCR for the TRPC4 led to the amplification of three major bands of 1200, 900 and 700 bp in MG-63 and SaOS cells suggesting the expression of transcripts for splice variants while no PCR product was revealed with the RNA from U2 OS. The gene expression of TRPC5 and TRPC7 was not revealed in human osteoblastic cells using total or messenger RNA, and TRPC2 is considered as a pseudogene in human Citation[10]. In addition, we investigated the expression of TRPC channels in the murine osteoblastic MC3T3 cells. The gene expression of TRPC2, TRPC4, TRPC6 and TRPC7 was revealed in murine MC3T3 cells with the observation of PCR amplification products of 890, 599, 687 and 525 bp, respectively (B). The expression of TRPC1, TRPC3, and TRPC5 was not observed with total or messenger RNA.
Figure 1. Gene expression of the TRPC channels in osteoblast-like cells. Complementary DNA synthesised with total RNA isolated from human (A) or murine (B) cells was used for PCR amplifications using specific primers for each human TRPC (hTRPC) and murine TRPC (mTRPC) channels. Representative data are shown from RNA isolations of at least three independent cultures. Left lane: 100 bp ladder.
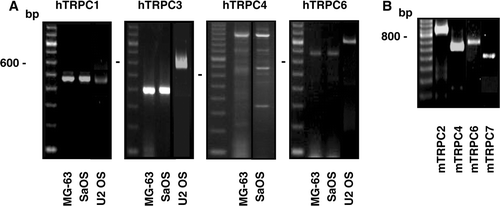
Gene expression of the TRPM channels in osteoblastic cells
The presence of messenger RNAs for TRPM channels was investigated by RT-PCR in various osteoblastic cell lines. Specific PCR products were obtained with the RNA from human osteoblast-like MG-63, U2OS and SaOS cells indicating the gene expression of TRPM3 (395 bp), TRPM4 (541 bp), TRPM6 (441 bp), TRPM7 (975 bp) and TRPM8 (539 bp) (A). Gene expression of TRPM1, TRPM2 and TRPM5 was not revealed using total or messenger RNA. As shown in B, the gene expression of TRPM1, TRPM4, TRPM6, TRPM7 and TRPM8 was revealed in the murine osteoblastic MC3T3 cells by the observation of PCR amplification products of 279, 952, 269, 814 and 525 bp, respectively. In contrast to human osteoblastic cells, the expression of TRPM3 was not observed. The gene expression of TRPM2 and TRPM5 was not revealed using total or messenger RNA.
Figure 2. Gene expression of the TRPM channels in osteoblast-like cells. Total RNA isolated from human (A) or murine (B) cells was used to synthesize complementary DNA for PCR amplifications using specific primers for each human TRPM (hTRPM) or murine TRPM (mTRPM) channels. Representative data are shown from RNA isolations of at least three independent cultures. Left lane: 100 bp ladder.
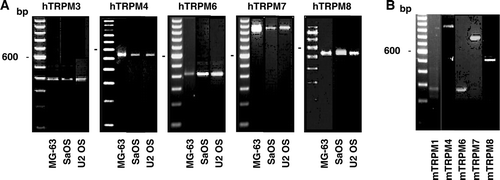
Gene expression of the TRPV channels in osteoblastic cells
The gene expression of TRPV channels was investigated in human and murine osteoblastic cells. Transcripts for the TRPV2 were revealed in the human osteoblast-like cells with PCR products of 433 bp (A) and in the murine cells with PCR product of 522 bp (B). Also, the gene expression of TRPV4 channels were shown in human osteoblast-like SaOS and U2 OS cells with PCR products of 866 and 686 bp, respectively, (A) suggesting two splice variants while no PCR product was observed with the RNA of MG-63 cells. In addition, the presence of transcripts for TRPV4 was revealed in the murine MC3T3 cells with PCR products of 403 bp (B).
Figure 3. Gene expression of the TRPV channels in osteoblast-like cells. Complementary DNA synthesised with total RNA isolated from human (A) or murine (B) cells was used for PCR amplifications using specific primers for human TRPV (hTRPV) or murine TRPV (mTRPV) channels. Representative data are shown from RNA isolations of at least three independent cultures. Left lane: 100 bp ladder.
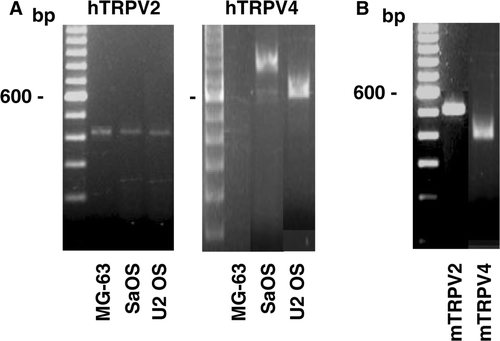
Involvement of TRPC channels in the capacitative calcium entry and the proliferation of osteoblastic cells
Since members of the TRPC channel family have been associated with the induction of CCE following depletion of Ca2+ from intracellular stores in human osteoblastic MG-63 cells Citation[6], we investigated the effect a known TRPC channel inhibitor SKF96365 on the induction of CCE in the murine MC3T3 cells, upon thapsigargine (Tg)-induced endoplasmic reticulum Ca2+ depletion by performing Fluo-3 fluorescence measurements of intracellular Ca2+. In the absence of external Ca2+, 5 µM Tg induced a transient increase of the intracellular Ca2+ concentration ([Ca2+]i) in MC3T3 cells (), which corresponds to the classical depletion of intracellular Ca2+ stores. When extracellular Ca2+ was reintroduced, a rapid rise in [Ca2+]i was evoked, which is typical of CCE activation. In contrast, the cells showed no response to the addition of Ca2+ in the buffer without prior stimulation with Tg (data not shown) suggesting no significant Ca2+ influx following the addition of calcium under basal condition. Following Ca2+ store depletion with 5 µM Tg in the absence of extracellular Ca2+, the addition of extracellular Ca2+ to the incubation media of cells pre-incubated with the known TRPC inhibitors SKF96365 resulted in the observation of minimal CCE (reduction by 94.3 ± 8.3% in MC3T3 cells; Dunnett's p < 0.01) (). Similar inhibition by SKF96365 was obtained for the CCE activated by platelet-derived growth factor (PDGF) and serum (data not shown). No significant difference was noticed for the percentage of cells that responded to Tg in the presence of channel blocker. Similar results were obtained with U2 OS and SaOS cells (data not shown). Altogether, these results indicate the presence of functional TRPCs in osteoblastic cells which are responsible for the establishment of CCE upon depletion of intracellular Ca2+ stores.
Figure 4. Induction of capacitative calcium entry in osteoblastic MC3T3 cells. Fluo3-loaded MC3T3 cells without or with pre-incubation with 30 µM SKF96365 for 15 min were treated with 5 µM thapsigargine (Tg) in Ca2+-free HEPES-buffered saline solution. After Tg-mediated intracellular Ca2+ release, Ca2+ was added (right arrow, final concentration of 1.8 mM) to the buffer alone. Each response is expressed as the mean ± SEM of the relative fluorescence intensity from at least three experiments with cumulating analysis of between 30 and 40 cells per field.
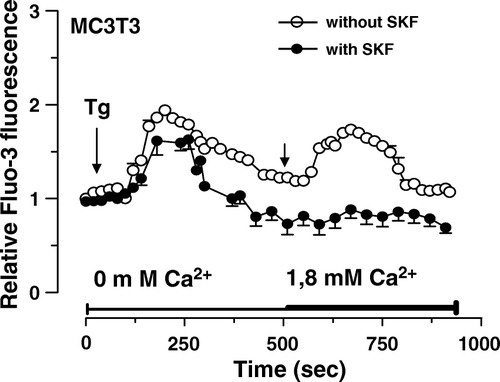
In order to address the role of channels of the TRPC family in the functions of osteoblastic cells, we investigated the effects of the SKF96365 on the proliferation of osteoblastic cells. As shown in , cell proliferation promoted by 10% FBS was reduced by SKF96365 in a dose-dependent manner in all human osteoblast-like cell lines. Similar results were observed for the murine MC3T3 cells (data not shown). Also, PDGF-stimulated proliferation was inhibited by SKF96365 in a dose-dependent manner in MG-63 and U2 OS cells, while the inhibitor was without effect on PDGF-unresponsive SaOS cells indicating that this inhibitor acted specifically on the stimulated cell proliferation.
Figure 5. Effect of the TRPC inhibitor SKF96365 on osteoblastic cell proliferation. MG-63 (A), SaOS (B) and U2 OS (C) osteoblasts were cultured for 48 h in DMEM-F12 supplemented with 10% FBS or 25 ng/ml PDGF and increasing concentrations of SKF96365. Cell proliferation was determined after 48 h by the MTT assay and data are the mean ± SEM of the cell proliferation compared to control condition in culture medium from 3–6 independent experiments. Statistical analyses were performed by ANOVA with Dunnett's post-test (p < 0.001 from 2 µM SKF96365 for MG-63 with either PDGF or FBS; p < 0.001 from 5 µM SKF96365 for SaOS with FBS; p < 0.001 from 2 µM SKF96365 for U2 OS with either PDGF or FBS)
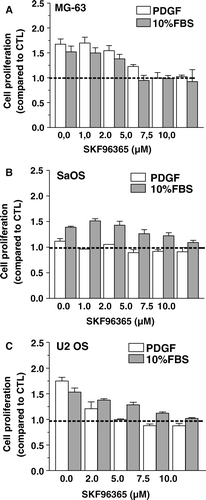
It should be noted that although SKF96365 has been used as TRP channel blockers, it also blocks chloride channels, ICRAC and second-messenger-activated non-selective currents Citation[11]. Nevertheless as our results suggest that one or numerous channels of the TRPC family are involved in the proliferation of osteoblastic cells, we further investigated the involvement of specific TRPC channels in osteoblast functions.
Involvement of TRPC1 and TRPC3 in the CCE and osteoblast proliferation
Of the TRPC family, TRPC1 and TRPC3 have been often considered as responsible for CCE. As murine MC3T3 cells did not express these channels, we therefore investigated the involvement of TRPC1 and TRPC3 in the CCE of human MG-63 cells. As TRPC3 channels have been shown to be responsible of the CCE induced upon 1alpha,25(OH)2D3- or thapsigargin-induced depletion of intracellular Ca2+ stores in the rat osteoblast-like ROS17/2.8 cells Citation[3], we investigated its involvement in our conditions. As shown in A, treatment of MG-63 cells with siRNA against TRPC3 led to a specific reduction of the expression of TRPC3. However, intracellular Ca2+ measurements showed that CCE was still observed upon depletion of intracellular Ca2+ stores by PDGF in the cells where TRPC3 expression has been abrogated (B).
Figure 6. Effect of reducing TRPC3 expression on the capacitative calcium entry induced by PDGF. MG-63 cells were transfected with specific siRNAs against human TRPC3 (si-hTRPC3) or with nontargeting control siRNA (Mock). (A) The expression of human TRPC1 and TRPC3 was determined after 48 h by RT-PCR and normalized according to the expression of GAPDH for three experiments. Student's t-test: *p < 0.05 compared to Mock condition. (B) Fluo3-loaded MG-63 cells transfected for 48 h with si-hTRPC3 or non-targeting control siRNA (Mock) were treated with 25 ng/ml PDGF in Ca2+-free HEPES-buffered saline solution. After PDGF-mediated intracellular Ca2+ release, Ca2+ was added (right arrow, final concentration of 1.8 mM) to the buffer alone. Each response is expressed as the mean ± SEM of the relative fluorescence intensity from at least three experiments with cumulating analysis of between 30 and 40 cells per field.
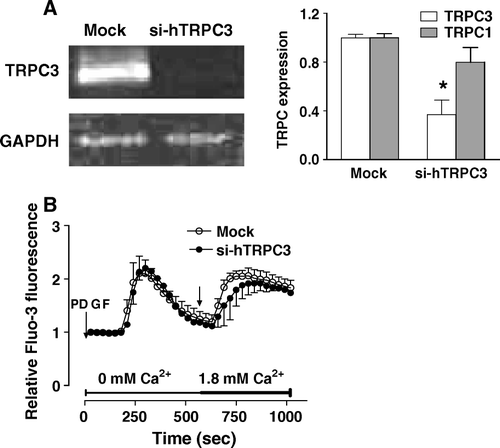
Moreover, transfection with siRNA against TRPC3 was without effect on the stimulation of MC3T3 proliferation by PDGF (1.49 ± 0.03 vs. 1.45 ± 0.05 for mock and si-TRPC3 conditions, respectively). As our results showed that TRPC3 channels were not responsible of CCE under our condition, we speculated that TRPC1 channels may account for the establishment of CCE. Under conditions where the expression of TRPC1 is reduced by 80% using interfering RNA (A), the CCE is nearly abolished (B). Moreover, PDGF did not stimulate cell proliferation in the osteoblasts transfected with the siRNA against TRPC1 while the basal cell proliferation was not affected (C).
Figure 7. Effect of reducing TRPC1 expression on the capacitative calcium entry and cell proliferation induced by PDGF. MG-63 cells were transfected with specific siRNAs against human TRPC1 (si-hTRPC1) or with nontargeting control siRNA (Mock). (A) The expression of human TRPC1 was determined after 48 h by RT-PCR and normalized according to the expression of GAPDH for three experiments. Student's t-test: *p < 0.05 compared to Mock condition. (B) Fluo3-loaded MG-63 cells transfected for 48 h with si-hTRPC1 or nontargeting control siRNA (Mock) were treated with 25 ng/ml PDGF in Ca2+-free HEPES-buffered saline solution. After PDGF-mediated intracellular Ca2+ release, Ca2+ was added (right arrow, final concentration of 1.8 mM) to the buffer alone. Each response is expressed as the mean ± SEM of the relative fluorescence intensity from at least three experiments with cumulating analysis of between 30 and 40 cells per field. Statistical analyses were performed by ANOVA with Dunnett's post-test p < 0.001. (C) The cell proliferation was determined by MTT assays on 48-h transfected cells with si-hTRPC1, non-trageting control (Mock) or in culture medium (CTL) following subsequent treatments of 48 h without or with 25 ng/ml PDGF. Data are the mean ± SEM of the cell proliferation compared to CTL without PDGF from 3–6 independent experiments. Student's t-test: ***p < 0.001 compared to CTL without PDGF, £££p < 0.001 compared to Mock with PDGF.
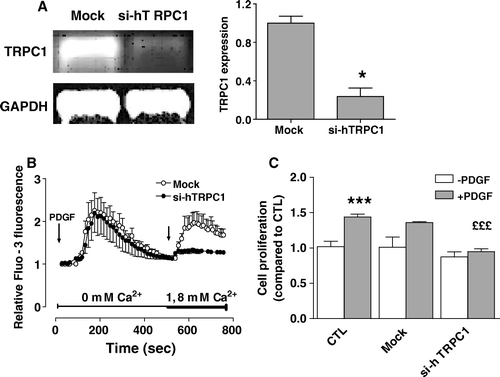
Involvement of TRPM7 channels in the proliferation of osteoblastic cells
One of the most characterized members of the TRPM channel family is TRPM7, a calcium and magnesium channel, which has been involved in cell proliferation Citation[12], Citation[13]. In order to evaluate the role of TRPM7 in osteoblastic cells, we determined the effects of RNA interference against TRPM7 on cell proliferation. The relative expression of TRPM7 evaluated by RT-PCR two days after the transfection showed a reduction of 80% (A). Abrogation of TRPM7 expression led to reduction of basal cell proliferation in the U2 OS and MG-63 and cells (B). Moreover, the stimulation of cell proliferation by PDGF was abolished by the siRNA against TRPM7. Similar results were obtained with the MC3T3 cells (data not shown). The silencing of TRPM7 expression had no effect on the depletion of intracellular Ca2+ stores and the activation of CCE (data not shown). Therefore, our results indicate that TRPM7 channels are involved in the basal and stimulated proliferation of osteoblastic cells.
Figure 8. Effect of reducing TRPM7 expression on the proliferation of osteoblasts. Cells were transfected with specific siRNAs against human TRPM7 (si-hTRPM7) or with nontargeting control siRNA (Mock). (A) The expression of human TRPM7 in U2 OS cells was determined after 48 h by RT-PCR and normalized according to the expression of GAPDH for three independent experiments. Student's t-test: ***p < 0.001 compared to Mock. (B) Cell proliferation was determined after 48 h for control cells in the culture medium alone (CTL) or for cells following 48-h transfection with nontargeting control siRNA (Mock) or with si-hTRPM7 in the absence or the presence of 25 ng/ml PDGF. Results of initial MTT values (absorbance values from cells at the initial day of treatment) are also shown. Data are expressed as the mean ± SEM of the cell proliferation compared to CTL of three independent experiments. Student's t-test analysis for U2 OS cells: *p < 0.05 compared to Mock condition, ££p < 0.001 compared to Mock condition with PDGF; MG-63 cells: ***p < 0.001 compared to Mock condition, £££p < 0.001 compared to Mock condition with PDGF.
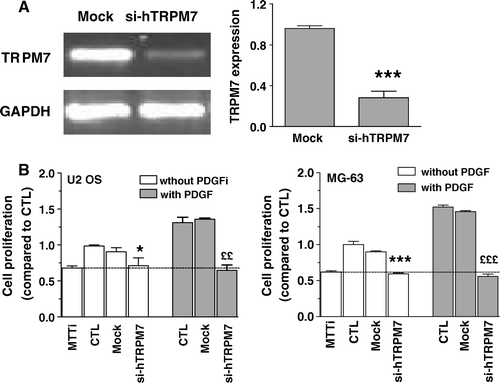
Discussion
TRPC channels in osteoblastic cells
On the basis of sequence homology and functional similarities, members of the mammalian TRPC family have been divided into four subfamilies: TRPC1, TRPC2, TRPC3/6/7 and TRPC4/5, where TRPC2 is a pseudogene in human. It should be noted that the expression of at least one member of each subfamily was shown in human osteoblastic cell lines, namely TRPC1, TRPC4 and TRPC3/6 with the exception of TRPC4 in U2 OS cells (). On the other hand, the pattern of expression of TRPCs in murine MC3T3 cells was remarkably distinct from human osteoblast-like cells. Although the expression of TRPC4 and TRPC6 was also observed as in human osteoblastic cells, no transcript for TRPC1 was revealed. Instead, the specific expression of TRPC2 and TRPC7 was found in murine cells. Differences in the pattern of TRPC expression could be related to species. On the other hand, the level of osteoblastic maturity may influence the pattern of TRPC expression since MG-63, U2OS are rather preosteoblasts and SaOS mature osteoblasts, whereas MC3T3 cells were maintained in the current study as osteoprogenitors. Further investigation of TRPC expression along osteoblastic differentiation would be of interest.
Table II. Gene expression of the TRPC channels in human and murine osteoblastic cells.
The existence of numerous splice variants has increased the functional multiplicity of TRPC channels. All members of the TRPC family are believed to share a common topology Citation[14], Citation[15]. The cytoplasmic N- and C-termini are separated by six predicted transmembrane domains (TM1–TM6), in addition of a putative pore region between TM5 and TM6. TRPs oligomerized as hetero or homotetramers to form ion channels. The N-terminus of TRPCs is composed of three to four ankyrin repeats, a predicted coiled coil region and a putative caveolin binding region. The cytoplasmic C-terminus includes the TRP signature motif (EWKFAR), a highly conserved proline rich motif, the CIRB (calmodulin/IP3 receptor binding) region and a predicted coiled-coil region. An extended C-terminus containing a PDZ binding motif is unique to TRPC4 and TRPC5. In view of the TRPCs expressed in osteoblastic cells, short (NM_003305) and long (TRPC3a, AY865574) variants for human TRPC3 have been reported with an extension of 73 amino acids in the N-terminus segment of the protein which has been proposed to influence the activation property of the channel from receptor-operated to store-operated channel Citation[16]. However, the identification of latter variants for TRPC3 was not possible with the primer set that we used. Splice variants for hTRPC6 (called delta316-431, AJ271067 and delta377-413, AJ271068) lacking the first out of six hydrophobic regions predicted to span the plasma membrane have been reported, although the protein expression and the functional meaning are still unknown. However, the identification of latter variants for TRPC6 was not possible with the primer set that we used. Numerous splice variants have been reported for human TRPC4. The three major bands revealed by RT-PCR on human osteoblast-like MG-63 and SaOS cells may correspond to transcripts previously reported as TRPC4 and variants α, β, ε, δ (PCR product of 1210 bp; NM_016179, AF421358, AF421359, AF421360, AF063824), TRPC4η (PCR product of 873 bp; AF421361) and TRPC4κ (PCR product of 691 bp; AF421362). Further investigations are warranted in order to identify the exact variant(s) of TRPC4 expressed in osteoblastic cells. Although the primer set for murine TRPC2 did not permit to reveal splice variants, isoforms with a shorter N-terminus (AF111107, AF230802 and AF230803) and with differences in the sequence for the C-terminus segment (AY465411) have been reported. Moreover, splice variants leading to shorter N-terminus segments have been identified for murine TRPC6 (AF057748) and C-terminus domain of TRPC4 (U50921 and AF019663).
There is evidence that TRPC channels can be activated, following phospholipase C activation, by DAG (as receptor-operated Ca2+ channel, ROCC), by direct interaction with the Ca2+ release channels of the endoplasmatic reticulum, the IP3 receptor and the ryanodine receptors, by translocation to the plasma membrane via an exocytotic process and by store-operated mechanisms (as store-operated Ca2+ channel, SOCC) Citation[17], Citation[18]. Although some debates persist about the exact mode of activation, our results indicate that the human TRPC1 account for capacitative calcium current (CCE) in osteoblastic cells. In contrast, TRPC3 has been shown in rat osteoblast-like ROS 17/2.8 cells to mediate capacitative calcium entry induced by thapsigargine and 1,25(OH)2D3 Citation[3]. It should be noted that our results indicated differences on the expression of TRPC channels with the expression of TRPC1 and TRPC3 in human and solely TRPC2 expression in murine osteoblast cells. Therefore similar functions to TRPC1 and TRPC3 may be ensured by the murine TRPC2. With the exception of TRPC3, the expression of other TRPC channels is currently unknown in rat osteoblast cells and differences in the expression of TRPC members between human and rat osteoblasts may underlie opposite results. Moreover, the prevailing mode of channel operation, ROCC or SOCC, has been shown to depend on the differential degree of TRPC3 expression levels which influence the final tetraoligomeric composition Citation[19]. Again, the levels of TRPC3 expression and of other TRPC members are unknown in rat osteoblasts. On the other hand, human and murine TRPC6 as well as murine TRPC7 may account for DAG-activated cation entry. It is generally accepted that CCE is triggered in order to ensure proper Ca2+ refilling of intracellular stores Citation[17] and it is thought to be an essential component of the long-term responses of the cell, including proliferation. In accordance, our results indicate that TRPC1 channels are involved in the stimulated osteoblast proliferation by PDGF. Interestingly, TRPC1 has been shown to form stretch-activated cation channels Citation[20] which may be related to the increase in bone formation upon mechanical loading.
TRPM channels in osteoblastic cells
On the basis of the proposed four groups (TRPM1/3, TRPM2/8, TRPM4/5 and TRPM6/7), again at least one member of each TRPM subfamily was shown to be expressed in all our osteoblastic cell lines, namely TRPM3, TRPM4, TRPM6/7 and TRPM8 for human cells, and TRPM1, TRPM4, TRPM6/7 and TRPM8 in murine cells (). The main difference was the expression of TRPM1 by murine osteoblastic cells compared to the expression of TRPM3 by human cells. Again, the differences in the pattern of TRPM expression could be related to species or the level of osteoblastic maturity and further investigation is warranted.
Table III. Gene expression of the TRPM channels in human and murine osteoblastic cells.
TRPM proteins lack ankyrin repeats in the N-terminus but contain the TRP domain in the C-terminus Citation[21]. The N-terminal part of TRPM proteins is considerably longer than the corresponding regions in TRPC, contains a large, 700 amino acids ‘TRPM homology region’. The C-terminus of TRPM proteins is considerably longer than the corresponding region of other TRP and some members of the TRPM family (TRPM2, TRPM6 and TRPM7) contain enzyme domains. These TRPM proteins are thus called “chanzymes”. Among all nine variants identified to date for TRPM3 and in view of our PCR product of 395 bp obtained for TRPM3 in human osteoblast-like cells, splice variants 1 (NM_020952), 2 (NM_024971), 3 (NM_206944), 4 (NM_206945), 7 (NM_206948) and 9 (NM_001007471) are possible. On the other hand variants 5 (NM_206946), 6 (NM_206947) and 8 (NM_001007470) are excluded since PCR product would be of 470 bp. Former variants show deletion of exons 10 to 12. Variants B and C of TRPM4 are likely according to PCR product of 541 bp whereas splice variant A for TRPM4 are excluded since PCR product would be of 184 bp. Expression of TRPM6 was revealed by RT-PCR (amplicon of 441 bp). Since M-6-Kinase variants were reported, we performed RT-PCR with specific primers for M-6-kinase 1 to 3 (AY333286, AY333287, AY333288 respectively). No PCR product was obtained (data not shown) confirming the expression of TRPM6 channel.
TRPM channels exhibit highly varying permeability to Ca2+ and Mg2+, from Ca2+-impermeable (TRPM4 and 5) to highly Ca2+ and Mg2+ permeable (TRPM6 and 7) Citation[22]. In view of the TRPM channels shown to be expressed in osteoblastic cells, little is known about the functional properties and cellular functions of TRPM1. This channel was identified and named melastatin because of the absence of TRPM1 mRNA in malignant transformed melanoma cell lines, suggesting a tumor suppressor function of the channel protein Citation[23], Citation[24]. TRPM3 channels have been shown to be expressed primarily in kidney and, at lesser levels in several tissues such as, in brain, testis, and spinal cord Citation[22], Citation[25–27]. TRPM3 channels showed a constitutive Ca2+ entry characterized by activation at positive potentials and deactivation at negative potentials, suggesting a voltage-dependent gating of TRPM3 Citation[28]. Depletion of intracellular Ca2+ stores further augmented TRPM3-mediated Ca2+ entry Citation[27]. Moreover, the Ca2+ entry in TRPM3-expressing HEK293 cells increased during treatment with hypotonic extracellular solution Citation[26] suggesting volume-regulated activity of TRPM3. Physiological function in the kidney was therefore attributed to Ca2+ homeostasis. TRPM4 forms calcium-activated sodium channels impermeable for calcium and have been suggested to mediate depolarization of the plasma membrane Citation[22], Citation[25]. Furthermore, Nilius et al Citation[29] reported that phosphatidylinositol 4,5-biphosphate (PIP2) may constitute a physiologically important brake on TRPM4 activity since activation of the phospholipase C (PLC)-coupled receptors potently inhibited currents through TRPM4. Cheng et al. Citation[30] reported that in insulin-secreting cells of the rat pancreatic beta-cell line INS-1, a dominant negative TRPM4 construct result in significantly decreased insulin secretion in response to a glucose stimulus.
The TRPM7 channels have been associated with cell proliferation and survival Citation[31], exhibiting spontaneously activated divalent cation (Ca2+, Mg2+, and other trace metals) entry regulated by the cytosolic Mg2+ and ATP levels Citation[32]. Expression of TRPM7 has been reported in neurons Citation[33], lymphocytes Citation[34], cardiac myocytes Citation[35], vascular smooth muscle cells Citation[13], and is thought to be widely expressed. TRPM7 channels have been associated with cell viability Citation[31], cellular Mg homeostasis Citation[36], anoxic neuronal cell death Citation[33], intestinal pacemaking activity Citation[37], cell proliferation of retinoblastoma cells Citation[12], vascular smooth muscle cell proliferation and response to fluid flow Citation[13]. Our previous results have indicated that TRPM7 is involved in the intracellular Mg homeostatis of osteoblastic cells as well as in basal cell proliferation Citation[7]. In the current study, we report that TRPM7 channels are also involved in PDGF stimulated osteoblast proliferation.
The cDNA of TRPM8 was first isolated from prostate cancer cells, and the function of TRPM8 was initially linked to progression of cancer cells Citation[38]. TRPM8 expression was also found in a subset of cold-responsive dorsal root ganglia neurons and in neurons from trigeminal ganglia as a cold receptor of the body Citation[39]. Heterologously expressed TRPM8 channels showed that TRPM8 channels are permeable to Ca2+ and are activated by cooling cells to < 24°C and by application of cooling agents such as menthol, icilin and eucalyptol. However, such thermosensation in osteoblastic cells may not be the main function of TRPM8 and further studies are needed in order to address its physiological roles in bone metabolism.
Expression of TRPV channels in osteoblastic cells
Our results indicate that only TRPV2 and TRPV4 are expressed in osteoblastic cells (). In accordance with our current results, van der Eerden et al. Citation[9] have reported the expression of TRPV5 in osteoclasts but not in primary cultures of human and murine osteoblasts. TRPV2, also called VRL-1 and growth factor-regulated channel (GRC), is a weakly Ca2+-selective cation channel which is a member of the so-called thermo-TRPs (TRPV1-4) that are activated by heating in heterologous expression systems and presumably appeared to function in thermosensation of sensory neurons (for a review Citation[40]). Whereas TRPV1 is activated by temperature higher than 43C, TRPV3 responds to a lower temperature range of 32–40°C Citation[41]. TRPV2 on the other hand was shown to respond to a noxious temperature of > 52°C Citation[42], thereby its physiological role as such remains unsubstantiated. These channels are also expressed in cell and tissues with no known thermoregulatory homeostasis such as lung alveolar macrophages, epithelial cells of various human tissues such as kidney, stomach, intestine and prostate Citation[43], in neutrophil granulocytes Citation[44], in skeletal, vascular and cardiac muscle cells Citation[45], Citation[46], in human hepatoblastoma (HepG2) cells Citation[47]. In osteoblastic cells TRPV2 main role may not be thermosensation. Moreover TRPV1 and TRPV2 have been shown to be not essential to normal heat responses Citation[48]. TRPV2 channels are emerging as multifunctional receptors which respond to growth factors Citation[49], Citation[50], is proposed to play a role in proinflammatory degranulation events in mast cells Citation[51] and in mechanosensation as a stretch-activated channel in vascular smooth muscle Citation[45]. Latter function in mechanosensation is more likely in osteoblastic cells since beneficial effects of mechanical loading on bone structure are known Citation[52]. Recently, the expression of TRPV4 channels has been shown in osteoblasts and osteoclasts Citation[8]. A physiologic role of TRPV4 in bone adaptation to mechanical stress was proposed as TRPV4 deficient mice were protected from the bone loss induced by unloading.
Table IV. Gene expression of the TRPV channels in human and murine osteoblastic cells.
Acknowledgements
This work was supported by the Natural Sciences and Engineering Research Council of Canada (NSERC) and the Fonds Québécois de la Recherche sur la Nature et les Technologies (FQRNT). Declaration of interest: The authors report no conflicts of interest. The authors alone are responsible for the content and writing of the paper.
References
- Mackie E. Osteoblasts: novel roles in orchestration of skeletal architecture. Int J Biochem Cell Biol 2003; 35: 1301–1305
- Pedersen SF, Owsianik G, Nilius B. TRP channels: an overview. Cell Calcium 2005; 38: 233–252
- Baldi C, Vazquez G, Calvo JC, Boland R. TRPC3-like protein is involved in the capacitative cation entry induced by 1alpha,25-dihydroxy-vitamin D3 in ROS 17/2.8 osteoblastic cells. J Cell Biochem 2003; 90: 197–205
- Santillan G, Baldi C, Katz S, Vazquez G, Boland R. Evidence that TRPC3 is a molecular component of the 1alpha,25(OH)2D3-activated capacitative calcium entry (CCE) in muscle and osteoblast cells. J Steroid Biochem Mol Biol 2004; 89-90: 291–295
- Santillan G, Katz S, Buitrago C, Boland R. 1alpha,25(OH)2D3 induces capacitative calcium entry involving a TRPC3 protein in skeletal muscle and osteoblastic cells. Biol Res 2004; 37: 647–651
- Labelle D, Jumarie C, Moreau R. Capacitative calcium entry and proliferation of human osteoblast-like MG-63 cells. Cell Prolif 2007; 40: 866–884
- Abed E, Moreau R. Importance of melastatin-like transient receptor potential 7 and cations (magnesium, calcium) in human osteoblast-like cell proliferation. Cell Prolif 2007; 40: 849–865
- Mizoguchi F, Mizuno A, Hayata T, Nakashima K, Heller S, Ushida T, Sokabe M, Miyasaka N, Suzuki M, Ezura Y, Noda M. Transient receptor potential vanilloid 4 deficiency suppresses unloading-induced bone loss. J Cell Physiol 2008; 216: 47–53
- van der Eerden BC, Hoenderop JG, de Vries TJ, Schoenmaker T, Buurman CJ, Uitterlinden AG, Pols HA, Bindels RJ, van Leeuwen JP. The epithelial Ca2+ channel TRPV5 is essential for proper osteoclastic bone resorption. Proc Natl Acad Sci USA 2005; 102: 17507–17512
- Wes PD, Chevesich J, Jeromin A, Rosenberg C, Stetten G, Montell C. TRPC1, a human homolog of a Drosophila store-operated channel. Proc Natl Acad Sci USA 1995; 92: 9652–9656
- Franzius D, Hoth M, Penner R. Non-specific effects of calcium entry antagonists in mast cells. Pflugers Arch 1994; 428: 433–438
- Hanano T, Hara Y, Shi J, Morita H, Umebayashi C, Mori E, Sumimoto H, Ito Y, Mori Y, Inoue R. Involvement of TRPM7 in cell growth as a spontaneously activated Ca2+ entry pathway in human retinoblastoma cells. J Pharmacol Sci 2004; 95: 403–419
- Beech DJ. Emerging functions of 10 types of TRP cationic channel in vascular smooth muscle. Clin Exp Pharmacol Physiol 2005; 32: 597–603
- Vannier B, Zhu X, Brown D, Birnbaumer L. The membrane topology of human transient receptor potential 3 as inferred from glycosylation-scanning mutagenesis and epitope immunocytochemistry. J Biol Chem 1998; 273: 8675–8679
- Vazquez G, Wedel BJ, Aziz O, Trebak M, Putney JW, Jr. The mammalian TRPC cation channels. Biochim Biophys Acta 2004; 1742: 21–36
- Yildirim E, Kawasaki BT, Birnbaumer L. Molecular cloning of TRPC3a, an N-terminally extended, store-operated variant of the human C3 transient receptor potential channel. Proc Natl Acad Sci USA 2005; 102: 3307–3311
- Parekh AB, Putney JW, Jr. Store-operated calcium channels. Physiol Rev 2005; 85: 757–810
- Freichel M, Vennekens R, Olausson J, Stolz S, Philipp SE, Weissgerber P, Flockerzi V. Functional role of TRPC proteins in native systems: implications from knockout and knock-down studies. J Physiol 2005; 567: 59–66
- Trebak M, Vazquez G, Bird GS, Putney JW. The TRPC3/6/7 subfamily of cation channels. Cell Calcium 2003; 33: 451–461
- Maroto R, Raso A, Wood TG, Kurosky A, Martinac B, Hamill OP. TRPC1 forms the stretch-activated cation channel in vertebrate cells. Nat Cell Biol 2005; 7: 179–185
- Huang CL. The transient receptor potential superfamily of ion channels. J Am Soc Nephrol 2004; 15: 1690–1699
- Kraft R, Harteneck C. The mammalian melastatin-related transient receptor potential cation channels: an overview. Pflugers Arch 2005; 451: 204–211
- Duncan LM, Deeds J, Hunter J, Shao J, Holmgren LM, Woolf EA, Tepper RI, Shyjan AW. Down-regulation of the novel gene melastatin correlates with potential for melanoma metastasis. Cancer Res 1998; 58: 1515–1520
- Deeds J, Cronin F, Duncan LM. Patterns of melastatin mRNA expression in melanocytic tumors. Hum Pathol 2000; 31: 1346–1356
- Harteneck C. Function and pharmacology of TRPM cation channels. Naunyn Schmiedebergs Arch Pharmacol 2005; 371: 307–314
- Grimm C, Kraft R, Sauerbruch S, Schultz G, Harteneck C. Molecular and functional characterization of the melastatin-related cation channel TRPM3. J Biol Chem 2003; 278: 21493–21501
- Lee N, Chen J, Sun L, Wu S, Gray KR, Rich A, Huang M, Lin JH, Feder JN, Janovitz EB, Levesque PC, Blanar MA. Expression and characterization of human transient receptor potential melastatin 3 (hTRPM3). J Biol Chem 2003; 278: 20890–20897
- Grimm C, Kraft R, Schultz G, Harteneck C. Activation of the melastatin-related cation channel TRPM3 by D-erythro-sphingosine. Mol Pharmacol 2005; 67: 798–805
- Nilius B, Mahieu F, Prenen J, Janssens A, Owsianik G, Vennekens R, Voets T. The Ca2+-activated cation channel TRPM4 is regulated by phosphatidylinositol 4,5-biphosphate. EMBO J 2006; 25: 467–478
- Cheng H, Beck A, Launay P, Gross SA, Stokes AJ, Kinet JP, Fleig A, Penner R. TRPM4 controls insulin secretion in pancreatic beta-cells. Cell Calcium 2007; 41: 51–61
- Nadler MJ, Hermosura MC, Inabe K, Perraud AL, Zhu Q, Stokes AJ, Kurosaki T, Kinet JP, Penner R, Scharenberg AM, Fleig A. LTRPC7 is a Mg.ATP-regulated divalent cation channel required for cell viability. Nature 2001; 411: 590–595
- Fleig A, Penner R. The TRPM ion channel subfamily: molecular, biophysical and functional features. Trends Pharmacol Sci 2004; 25: 633–639
- Aarts M, Iihara K, Wei WL, Xiong ZG, Arundine M, Cerwinski W, MacDonald JF, Tymianski M. A key role for TRPM7 channels in anoxic neuronal death. Cell 2003; 115: 863–877
- Perraud AL, Knowles HM, Schmitz C. Novel aspects of signaling and ion-homeostasis regulation in immunocytes. The TRPM ion channels and their potential role in modulating the immune response. Mol Immunol 2004; 41: 657–673
- Gwanyanya A, Amuzescu B, Zakharov SI, Macianskiene R, Sipido KR, Bolotina VM, Vereecke J, Mubagwa K. Magnesium-inhibited, TRPM6/7-like channel in cardiac myocytes: permeation of divalent cations and pH-mediated regulation. J Physiol 2004; 559: 761–776
- Schmitz C, Perraud AL, Johnson CO, Inabe K, Smith MK, Penner R, Kurosaki T, Fleig A, Scharenberg AM. Regulation of vertebrate cellular Mg2+ homeostasis by TRPM7. Cell 2003; 114: 191–200
- Kim BJ, Lim HH, Yang DK, Jun JY, Chang IY, Park CS, So I, Stanfield PR, Kim KW. Melastatin-type transient receptor potential channel 7 is required for intestinal pacemaking activity. Gastroenterology 2005; 129: 1504–1517
- Tsavaler L, Shapero MH, Morkowski S, Laus R. Trp-p8, a novel prostate-specific gene, is up-regulated in prostate cancer and other malignancies and shares high homology with transient receptor potential calcium channel proteins. Cancer Res 2001; 61: 3760–3769
- McKemy DD, Neuhausser WM, Julius D. Identification of a cold receptor reveals a general role for TRP channels in thermosensation. Nature 2002; 416: 52–58
- Ramsey IS, Delling M, Clapham DE. An introduction to TRP channels. Annu Rev Physiol 2006; 68: 619–647
- Desai BN, Clapham DE. TRP channels and mice deficient in TRP channels. Pflugers Arch 2005; 451: 11–18
- Caterina MJ, Rosen TA, Tominaga M, Brake AJ, Julius D. A capsaicin-receptor homologue with a high threshold for noxious heat. Nature 1999; 398: 436–441
- Kowase T, Nakazato Y, Yoko O, Morikawa A, Kojima I. Immunohistochemical localization of growth factor-regulated channel (GRC) in human tissues. Endocr J 2002; 49: 349–355
- Heiner I, Eisfeld J, Halaszovich CR, Wehage E, Jungling E, Zitt C, Luckhoff A. Expression profile of the transient receptor potential (TRP) family in neutrophil granulocytes: evidence for currents through long TRP channel 2 induced by ADP-ribose and NAD. Biochem. J 2003; 371: 1045–1053
- Muraki K, Iwata Y, Katanosaka Y, Ito T, Ohya S, Shigekawa M, Imaizumi Y. TRPV2 is a component of osmotically sensitive cation channels in murine aortic myocytes. Circ Res 2003; 93: 829–838
- Iwata Y, Katanosaka Y, Arai Y, Komamura K, Miyatake K, Shigekawa M. A novel mechanism of myocyte degeneration involving the Ca2+-permeable growth factor-regulated channel. J Cell Biol 2003; 161: 957–967
- Vriens J, Janssens A, Prenen J, Nilius B, Wondergem R. TRPV channels and modulation by hepatocyte growth factor/scatter factor in human hepatoblastoma (HepG2) cells. Cell Calcium 2004; 36: 19–28
- Woodbury CJ, Zwick M, Wang S, Lawson JJ, Caterina MJ, Koltzenburg M, Albers KM, Koerber HR, Davis BM. Nociceptors lacking TRPV1 and TRPV2 have normal heat responses. J Neurosci 2004; 24: 6410–6415
- Kanzaki M, Zhang YQ, Mashima H, Li L, Shibata H, Kojima I. Translocation of a calcium-permeable cation channel induced by insulin-like growth factor-I. Nat Cell Biol 1999; 1: 165–170
- Boels K, Glassmeier G, Herrmann D, Riedel IB, Hampe W, Kojima I, Schwarz JR, Schaller HC. The neuropeptide head activator induces activation and translocation of the growth-factor-regulated Ca(2+)-permeable channel GRC. J Cell Sci 2001; 114: 3599–3606
- Stokes AJ, Shimoda LM, Koblan-Huberson M, Adra CN, Turner H. A TRPV2-PKA signaling module for transduction of physical stimuli in mast cells. J Exp Med 2004; 200: 137–147
- Pavalko FM, Norvell SM, Burr DB, Turner CH, Duncan RL, Bidwell JP. A Model for mechanotransduction in bone cells: the load-bearing mechanosomes. J Cell Biochem 2003; 88: 104–112