Abstract
Membrane protein-protein interactions are important for regulation, targeting, and activity of proteins in membranes but are difficult to detect and analyse. This review covers current approaches to studying membrane protein interactions. In addition to standard biochemical and genetic techniques, the classic yeast nuclear two-hybrid system has been highly successful in identification and characterization of soluble protein-protein interactions. However, classic yeast two-hybrid assays do not work for membrane proteins because such yeast-based interactions must occur in the nucleus. Here, we highlight recent advances in yeast systems for the detection and characterization of eukaryote membrane protein-protein interactions. We discuss these implications for drug screening and discovery.
Introduction
Membrane protein-protein interactions are essential for cellular and animal responses to the surrounding environment. For example, receptor interaction with soluble ligands can trigger assembly of functional signalling complexes that regulates gene expression and cell proliferation. The identification and characterization of such membrane protein-protein interactions is an essential prerequisite for understanding cell function and is likely to provide the basis for new therapeutics in many human diseases. A bottleneck in this area of research is in rapidly screening and identifying interactions between membrane proteins that occur within a biological membrane.
The different approaches used to currently study membrane protein-protein interactions are based on genetic, biochemical or cellular techniques. Identification of genetic mutations that delineate one or more gene products associated with a physiological response is usually followed by biochemical analyses to characterize protein function. The intracellular protein location as determined using light, confocal and electron microscopy may give further clues to protein function. Functional characterization of membrane protein complexes using biochemical procedures based on purification is technically difficult, requiring relatively large amounts of starting materials and detergent-based isolation which can be prone to artefacts. The isolation of functionally active membrane protein complexes that are relatively large (> 1 MDa) is also problematical with current techniques Citation[1].
Various biochemical techniques are available for the detailed analysis of protein-protein interactions. These include denaturing and non-denaturing gel electrophoresis, blue native polyacrylamide gel electrophoresis Citation[2], CAT gel Citation[3], immunological or ligand-based affinity purification Citation[4], mass spectrometry Citation[5], protein microarrays Citation[6] and surface plasmon resonance Citation[4]. Such techniques are in vitro in concept and approach: readouts are affected by temperature, ionic strength and co-factor availability thus requiring extensive optimization for each type of protein-protein interaction. Additionally, such techniques are usually not sensitive enough to detect weak or transient interactions, e.g. micromolar affinities. The use of detergent lysis to purify membrane protein complexes can also be problematical for subsequent mass spectrometry analyses Citation[1].
Yeast 2-hybrid (Y2H) screens and assays have been increasingly used over the past 20 years to detect and characterize protein-protein interactions based upon manipulation of auxotrophic and bacterial β-galactosidase gene expression in the budding yeast Saccharomyces cerevisiae. This approach enables analysis of mammalian protein-protein interactions such that the environment resembles higher eukaryotes e.g. human cells. Key advantages include the short life cycle (∼ 90 min) of S. cerevisiae, amenability to a wide range of techniques, genetic tractability and relative low cost. Yeast-based genetic screens can also detect weak and transient interactions unlikely to be revealed by other techniques. Such assays and reagents are now commercially widely available with comprehensive detailed protocols. Currently, estimates reveal that >50% of the database of protein-protein interactions is derived from different Y2H screens Citation[7], Citation[8].
The ‘classic’ yeast 2-hybid system
The original Y2H system was devised by Fields and Song in 1989 Citation[9]. This was based upon the S. cerevisiae GAL4 transcriptional activator which regulates transcription of genes encoding enzymes required for galactose utilization. GAL4 has an N-terminal DNA-binding domain (BD) enabling binding to upstream activating sequences (UAS) and a C-terminal activation domain (AD) which recruits yeast RNA polymerase II and stimulates the initiation of mRNA synthesis. Fusion of the GAL4 UAS upstream of a reporter gene such as Escherichia coli β-galactosidase (LacZ) allows controlled expression of this useful enzyme as a marker (A). Hybrid proteins containing either the GAL4 BD or AD alone cannot activate transcription of reporter gene (B, C). However, when the relevant domains from two known interacting proteins (e.g. X, Y) are fused to the corresponding domains of GAL4, a functional transcriptional activator complex can be assembled resulting in transcription of downstream target genes (D).
Figure 1. The ‘classic’ yeast 2-hybrid system. (A) A yeast S. cerevisiae transcriptional activator consisting of both AD (activating domain) and BD (binding domain) can induce reporter gene transcription. (B) A hybrid of protein X fused to BD (BD-X) alone is incapable of inducing gene transcription. (C) A hybrid of protein Y fused to AD (Y-AD) alone is incapable of inducing gene transcription. (D) Interaction of protein X-BD with Y-AD brings the AD into close proximity to the promoter of the gene, thus recruiting RNA polymerase II to transcribe the reporter gene. This Figure is reproduced in colour in Molecular Membrane Biology online.
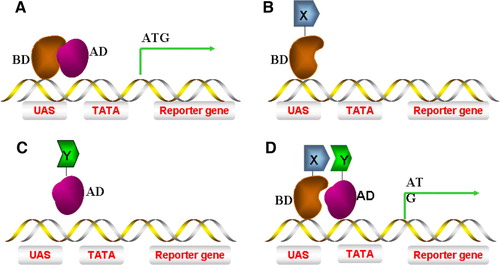
The S. cerevisiae strains used in such assays are genetically engineered to contain auxotrophic marker genes whose expression is controlled by the reconstituted hybrid GAL4 protein activity. Here, genetic markers are engineered for GAL4-regulated gene transcription of an essential enzyme required for amino acid or nucleotide biosynthesis thus controlling yeast growth where such nutrients are missing. Thus functional protein-protein interactions must be reconstituted to stimulate gene expression of the necessary enzyme in such auxotrophic yeast strains. Alternatively, a reporter gene such as LacZ can be controlled to produce β-galactosidase whose levels can be monitored using a simple colorimetric assay. Independent yeast colonies can be screened for β-galactosidase activity either on plates or in culture as a qualitative or quantitative measure of a protein-protein interaction under study. This has enabled identification and characterization of different protein-protein interactions important in different physiological processes including gene expression, intracellular signalling and trafficking Citation[10] but this has been limited to those interactions between soluble domains or subunits. Such an approach can be important in the initial screening of cDNA libraries searching for interacting protein partners (‘prey’) for a protein of interest Citation[11].
There are limitations in the classic Y2H system. Firstly, hybrid proteins need to be targeted to the nucleus. However, integral membrane proteins and membrane-associated proteins retaining the native membrane-linked properties cannot be detected by this approach. Secondly, interactions with proteins within the secretory pathway cannot be detected. Thirdly, if functional protein–protein interactions are dependent on free amino or carboxyl termini, steric hindrance of such domains within hybrid proteins may give a negative readout.
Nearly 30% of proteins encoded by the human genome are likely to be membrane-associated Citation[12]. These proteins have essential cellular and physiological functions such as selective transport across a biological membrane either by acting as channels or active transporters. Other membrane proteins such as receptors bind hormones or growth factors and the receptor-ligand complex can transmit signals to the cell interior. Membrane proteins can also have enzymatic activity which is regulated by extracellular ligands Citation[13]. Of the current drugs commercially available for human disease therapy, > 50% target such membrane receptors and ion channels Citation[14]. Similar to key regulators in other cellular pathways, membrane proteins recruit a host of accessory proteins to initiate biochemical reactions that have profound effects on physiological responses. Many membrane proteins are insoluble in aqueous buffers and form aggregates due to the strong hydrophobic properties of membrane-associated or transmembrane domains. As classic Y2H assays require the hybrid protein ‘bait’ and ‘prey’ to be present within the nucleus, membrane protein analyses require alternative strategies for yeast-based genetic screens. Thus a strong requirement of membrane protein analysis is functional reconstitution within or close to a biological membrane.
Membrane-based yeast 2-hybrid (Y2H) screens
Sos Y2H screen
Membrane yeast 2-hybrid screens are being increasingly used based on complex bait-prey strategies for auxotrophic growth Citation[15]. To reproduce a yeast genetic screen where bait and prey are reconstituted in close proximity to the yeast plasma membrane, Aronheim and co-workers Citation[16] developed a novel Sos-based Y2H system. Here, human Ras GDP exchange factor Sos is used to functionally replace the S. cerevisiae Ras exchange factor (Cdc25) homologue which is essential for cell survival and growth. There are also other variants of this screen: a Ras recruitment system (RRS) Citation[17] and reverse RRS Citation[18–20]. Briefly, the bait is a plasma membrane-localized protein whereas cDNA library-derived prey proteins are fused to human Sos, cdc25, or activated Ras. When a candidate fusion protein associates with a bait and recruits Sos, cdc25, or activated Ras to the plasma membrane, MAPK signalling occurs promoting yeast survival and growth (A, 2B). Aronheim et al. (1997) used this Sos Y2H screen and identified proteins that interacted with c-Jun, a subunit of the AP-1 transcriptional activator Citation[21]. One such protein is JDP2 which forms heterodimers with c-Jun and represses AP-1-mediated activation. Using this membrane-associated Y2H screen, new pleckstrin homology (PH) domain-containing proteins that bind different phosphoinositides have been identified including Gab1, Dos, myosin X and Sbf1 Citation[20]. A disadvantage of this method is that yeast survival signals can be activated independently of bait binding if the prey already has plasma membrane localization properties. Thus this system cannot be used to identify protein-protein interactions between two integral membrane proteins or membrane-linked domains.
Figure 2. The Sos Y2H system. (A) Grb2 binds to a phosphotyrosine epitope in the cytoplasmic domain of an activated growth factor receptor and mediates recruitment of Sos (Cdc25), which in turn leads to Ras activation. (B) A temperature-sensitive mutation in the Ras guanyl nucleotide exchange factor Cdc25–2, blocks yeast growth at 36°C. A cytosolic protein domain Y is fused to Sos that allows interaction with an integral membrane protein X, leading to Ras activation and cell growth at 36°C. This Figure is reproduced in colour in Molecular Membrane Biology online.
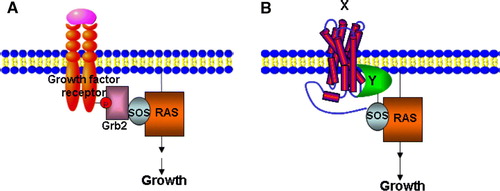
G-protein coupled receptor Y2H screen
An alternative membrane-associated Y2H screen utilizes the endogenous heterotrimeric G-protein receptor (GPCR) signalling pathway as a readout to select for protein-protein interactions Citation[15], Citation[22]. GPCRs can respond to diverse stimuli including hormones, neurotransmitters, odours and light. GPCR activation triggers a conformational change in the G-protein α-subunit, exchange of GDP for GTP, and dissociation of Gα from the G-protein βγ subunits (A). Depending on the system of choice, either Gα or Gβγ can activate downstream effectors until GTP is hydrolyzed and the protein reverts to the inactive conformation. In B, one binding partner is an integral membrane protein (X) whereas the other is a soluble protein domain (Y) fused to a G-protein γ subunit. If X and Y interact, G-protein signalling via Gβγ is disrupted. This system has been used to demonstrate known interactions between syntaxin 1a with neuronal Sec1 (nSec1) and fibroblast-derived growth factor receptor 3 (FGFR3) with SNT-1Citation[22]. In this assay, yeast survival and growth is only feasible when an integral membrane protein (X) interacts with a soluble cytoplasmic protein or domain (Y).
Figure 3. A G-protein coupled receptor-based Y2H system. (A) The activation of a G-protein coupled receptor can lead to dissociation of Gβγ from Gα and then to the activation of downstream effectors. (B) In the Gγ-deficient yeast mutant stain, a hybrid cytosolic protein Y interacts with Gβγ and membrane-bound protein X simultaneously and prevents interaction of Gβγ to activated Gα, thus inhibiting downstream signalling and gene expression. This Figure is reproduced in colour in Molecular Membrane Biology online.
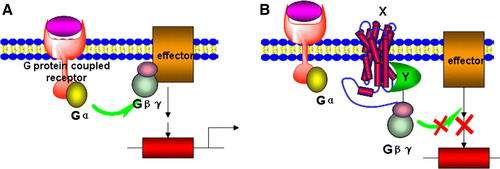
The split-ubiquitin Y2H screen
Integral membrane protein interaction Y2H studies are now feasible using the split-ubiquitin assay developed by Johnsson and Varshavsky Citation[15],Citation[23]. Here, ubiquitin is used as a sensor: this small polypeptide (76 residues) is a highly conserved eukaryote module that is recognized by proteolytic degradation and cleavage machinery from yeast to man. Ubiquitin can be covalently attached to target proteins for recognition and degradation by the 26S proteasome. Ubiquitin-regulated degradation regulates many cellular processes including membrane trafficking, intracellular signalling, gene expression, apoptosis, DNA repair and the cell cycle. Ubiquitin is protected from proteasome degradation by ubiquitin-specific proteases (UBPs) which cleave ubiquitin from the target protein and allow ubiquitin recycling; this enzymatic reaction is again conserved from yeast to man.
The three-dimensional structure of ubiquitin shows it can be divided into an N-terminal fragment (Nub; residues 1–37) and a C-terminal fragment (Cub; residues 38–76) Citation[23]. Co-expression of Nub and Cub as separate cytosolic polypeptides allows functional reconstitution of a stable folded ubiquitin domain, i.e. the ‘split-ubiquitin’ module. UBPs can still recognize and cleave this split-ubiquitin from a target protein but cannot recognize the Nub or Cub portions alone (A). The I13G substitution within the Nub moiety (NubG) strongly reduces affinity for the Cub moiety. Co-expression of NubG and Cub cannot reconstitute the split-ubiquitin module and UBP-mediated cleavage cannot occur (B). However, the split-ubiquitin Y2H system brings NubG and Cub together via functional interaction of bait and prey. Here, hybrid proteins are created by fusing a bait protein (X) to Cub and a prey protein (Y) to NubG. Interaction of the two proteins brings Cub and NubG into close proximity, forcing reconstitution of the folded split-ubiquitin module. This triggers UBP-mediated cleavage and release of a LexA-VP16 transcriptional activator attached on the C-proximal side of the Cub moiety. Thus interaction between bait and prey is detected by LexA-VP16-regulated expression of auxotrophic markers and the lacZ reporter (C).
Figure 4. The split-ubiquitin Y2H system. (A) Native ubiquitin can be divided into two parts: Cub and Nub. Fusion of Cub to the transcription activator LexA-VP16 followed by co-expressed with Nub, leads to spontaneous assembly of a native ubiquitin molecule and recognition by a UBP, cleavage and liberation of LexA-VP16. (B) Introduction of the I13G mutation into Nub (Nub-G) causes reduced affinity for Cub, lack of ubiquitin assembly and no cleavage. (C) The interaction of integral membrane protein X with either the cytosolic protein Y or an integral membrane protein Z can bring NubG and Cub into close proximity, followed by UBP-mediated cleavage which releases the LexA-VP16 transcription factor, allowing it to enter into the nucleus and stimulate reporter gene transcription.
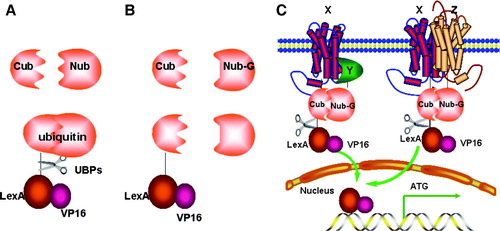
A major advantage of this system is that formation of the split-ubiquitin complex does not require unique cellular localization apart from reconstitution within the yeast cytoplasm for recognition by endogenous UBPs. Thus both membrane bound and cytosolic proteins can be used for analysis. In addition, Nub and Cub are relatively small modules that have minimal steric hindrance on bait-prey interactions. Such a protein-protein interaction directly corresponds to affinity for the split-ubiquitin by UBP, proteolytic cleavage and levels of free LexA-VP16. This is in turn proportional to transcriptional activation of downstream auxotrophic marker and the lacZ reporter gene.
This method has been used for the detection of interactions between soluble proteins Citation[23], transcriptional activators Citation[24] and membrane proteins. In this latter category, Stagljar and co-workers have used this approach to define protein-protein interactions between two ER resident proteins, Ost1 and Wbp1 Citation[25]. Similarly, this has been used to show that the N-terminus of SUT2 interacts functionally with the sucrose transporter, SUT1 Citation[26]. This system has also been used to study interactions between endoplasmic reticulum α1,2-mannosidase Mns1p with Rer1p Citation[27] and the yeast multiple transmembrane domain ABC transporter Citation[28].
This technique can be used to screen interacting proteins from a cDNA library using a membrane protein bait fused to Cub-LexA-VP16. In one study, human ErbB3 (a member of the epidermal growth factor receptor tyrosine kinase family) was used as bait to screen a human brain cDNA library. Of three novel prey proteins identified, interaction of one such protein (RGS4) with ErbB3 was confirmed by co-precipitation experiments in cultured human cells Citation[29]. Plasma membrane integral membrane proteins such as the amyloid precursor protein (APP) and the low-density lipoprotein receptor-related protein (LRP) have been used to identify known interacting proteins (Fe65) and a new interacting proteins (Bri2); such interactions were again confirmed by co-precipitation in cultured mammalian cells Citation[30], Citation[31]. One problem with large scale screens using the split-ubiquitin system are false positive or negative signals. To solve this problem, a mating-based split-ubiquitin system (Mb-SUS) used a regulated promoter Met to control the expression of a bait and distinguish between specific and non-specific NubG-x interactions. This approach allowed a systematic analysis of plant potassium channel membrane protein-protein interactions Citation[32]. Another optimized split-ubiquitin cDNA-library screening system based on the URA3 auxotrophic marker used 5-fluoroorotic acid (5-FOA) to increase the reproducibility of protein-protein interactions and decrease non-specific background growth: several novel proteins that interacted with the Frizzled membrane protein were identified Citation[33].
The yeast three-hybrid (Y3H) system
Some signal transduction proteins do not directly interact with a downstream target but require a partner to mediate functional interaction. For example, EGF binds and activates EGFR leading to receptor homodimerization and tyrosine autophosphorylation, creating high-affinity phosphotyrosine sites that are recognized by proteins containing SH2 (c-src homology domain 2) or phosphotyrosine-binding (PTB) domains. The Grb2 SH2 domain binds to autophosphorylated EGFR; the Grb2 SH3 domains bind to proline-rich motifs in the Sos exchange factor. Plasma membrane recruitment of Sos stimulates Ras-GTP formation, activation and MAPK signalling Citation[34]. Protein-protein interaction domains resembling receptor/Grb2/Sos are found in other receptor tyrosine kinase receptor signalling pathways Citation[35]. In this case, the more complex Y3H system can be used to reconstitute a tripartite complex and reveal hierarchies of protein-protein interactions. Z-protein-mediated X and Y interactions (A) are exemplified by Grb2 formation of a bridge between the EGFR C-terminus and Sos Citation[36]. This method has also been used to detect extracellular interactions such as growth hormone-induced receptor dimerization and ligand-induced vascular endothelial growth factor receptor dimerization in which the hormone or ligand was co-expressed as a secreted protein Citation[37]. The Y3H system can also be used to detect interactions between protein X and protein Y dependent on modification by a third protein, Z (B). For example, using FcεRI as bait and Syk as prey, protein tyrosine kinase Lck was also expressed. Lck could phosphorylate FcεRI to promote phospho-FcεRI binding to the Syk SH2 domain Citation[38]. Using Gab2 as a bait protein, co-expression of Lck promoted Gab2 tyrosine phosphorylation enabling phospho-Gab2 binding to prey proteins containing SH2 domains Citation[39].
Figure 5. Yeast three-hybrid (Y3H) systems. (A) Interaction between protein X and protein Y must be mediated by protein Z and assembly of this protein complex stimulates reporter gene transcription. (B) Protein X can only interact with protein Y (and stimulate gene transcription) after being modified by protein Z, e.g. phosphorylation. This Figure is reproduced in colour in Molecular Membrane Biology online.
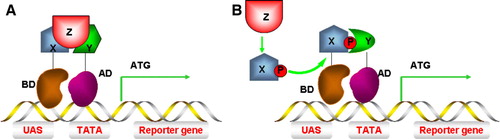
Yeast genetic screens in drug discovery
Protein-protein interactions integral to signalling pathways are ideal targets for disruption using small organic molecules that interfere or modulate such interactions. For example, the p53 tumour suppressor interacts with an E3-like ubiquitin ligase MDM2, and this interaction is a key target for cancer therapy. Small organic molecules called nutlins have been discovered and can specially inhibit the interaction between MDM2 and p53 Citation[40–42]. Thus such yeast hybrid screens and assays could be used for identifying new molecules for drug discovery.
Y3H screens for inhibitors of protein-protein interactions
Here, the yeast URA3 gene is used as a reporter Citation[43] and the interaction between protein X and protein Y leads to production of the reporter enzyme (URA3p). This enzyme now converts synthetic 5′-fluoroorotic acid (FOA) in the selective medium into a toxic metabolite and kills yeast cells. Addition of compounds which inhibit protein-protein interactions prevent expression of this toxic product and restore yeast growth () Citation[44]. This assay can be used to directly screen a chemical library for drugs that disrupt ternary complex formation.
Figure 6. Y3H screen for compounds that inhibit protein-protein interactions. (A) The association of protein X and protein Y induces transcription of URA3, leading to URA3p production and conversion of 5-FOA into a toxic metabolite and cell death. (B) A small chemical compound c can inhibit the interaction between protein X and protein Y, protecting against cell death causing cell survival instead. This Figure is reproduced in colour in Molecular Membrane Biology online.
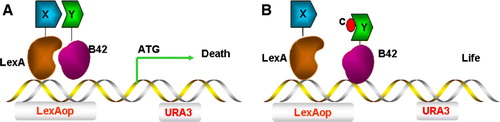
Y3H screens for detecting small molecules
The identification of a membrane protein or receptor associated with a human disease invariably leads to a quest for small molecules or compounds that modulate receptor function. Several Y3H screens have been developed based on the nuclear Y2H system. In one such system, a binding site for LexA (a DNA binding protein) is placed upstream of the LacZ reporter. A dexamethasone-methotrexate hybrid compound facilitates interaction of LexA-dihydrofolate reductase (LexA-DHFR) and a B42-glucocorticoid receptor (B42-GR) fusion protein. Thus, the Dex-Mtx small molecule effectively reconstitutes the LexA-B42 transcriptional activator, stimulating transcription of the LacZ reporter gene and production of β-galactosidase (). Similar methods were designed by varying one of the ligand and receptor pairs such as FK506 and FK506 binding protein 12 (FKB12), methotrexate (MTX) or dihydrofolate reductase (DHFR) Citation[45]. A method with split-ubiquitin as sensor has also been successfully used to identify a small-molecular inhibitor of human PCTAIRE protein kinase. This has opened up new possibilities: studying protein-protein interactions that cannot be reconstituted in the nucleus and identifying small molecules that perturb protein-protein interactions between membrane proteins which became key drug targets Citation[46].
Figure 7. Y3H system for the detection of small molecules and protein interactions. Interaction between dihydrofolate reductase (DHFR) and glucocorticoid receptor (GR) is mediated by a dexamethasone-methotrexate (Mtx-Dex) heterodimer, thus inducing transcription of a reporter gene. This Figure is reproduced in colour in Molecular Membrane Biology online.
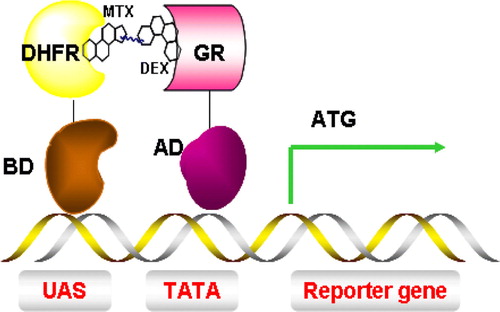
Conclusions and future perspectives
Understanding protein function is dependent on the identification of other proteins and factors recruited to a functionally active complex. Over the past 20 years, yeast 2-hybid systems and its derivatives have been shown to be powerful for identification and analysis of protein-protein interactions Citation[47]. Much effort has now shifted towards the determination of large-scale and complex protein-protein interactions linked to mapping whole pathways and physiological responses. The yeast system is advantageous in its genetic tractability, and relative ease of use for robotic platform-based screens to build genomic protein-protein interaction maps. Such approaches have also been used to build protein-protein interaction maps for viruses Citation[48], prokaryotes Citation[49] and yeasts Citation[50].
The main disadvantages of yeast-based methods are the false positives caused by promoter mutations or bait protein overexpression. The inclusion of comprehensive controls linked to expression of more reporter genes can minimize false positives in such assays Citation[51], Citation[52]. Some protein fusions cause yeast toxicity which can be partially circumvented by the use of inducible expression systems. Thus independent verification of putative protein-protein interactions is essential using biochemical assays, e.g. immunoprecipitation of protein complexes from cell lysates and pull-down assays using functionally active and/or recombinant proteins. In addition to biochemical and gene-based assays, post-translational assays using fluorescence and bioluminescence as readouts including FRET (fluorescence resonance energy transfer), BRET (bioluminescence resonance energy transfer), self-splicing split inteins (DnaE) and split YFP (yellow fluorescence protein) have been devised for the detection, screening or reconstitution of interactions by both soluble and membrane proteins in the cytosol, mitochondria and nucleus Citation[53–57].
How can we best use such yeast genetic methods to evaluate complex membrane protein function, e.g. receptor tyrosine kinases (RTKs)? These proteins are not only membrane receptors but also enzymes and signalling modules that regulate intracellular communication, cell migration, proliferation and differentiation. Deregulation of RTKs via specific mutations, gene rearrangements, gene amplification and overexpression are implicated in the development and progression of numerous human malignancies Citation[58]. RTK signalling pathways have become promising targets for development of new and more selective anti-cancer drugs.
For example, a RTK cytoplasmic domain could be used as a soluble bait to isolate factors using the classic nuclear Y2H system. However, the newer membrane-based Y2H split-ubiquitin system now allows screening for cytosolic or membrane interactions from a cDNA library, using an RTK integral membrane protein as bait. ErbB3 has successfully studied with the Y2H split-ubiquitin system Citation[29]. The split-ubiquitin system also has flexibility in allowing modification to all types of yeast hybrid systems to screen for interacting proteins, small molecule inhibitors of RTK dimerization or higher order protein complex assembly and functionality. Such RTK-specific inhibitors could be new cancer therapeutics. The information derived from the use of such yeast genetic methods will enable a better understanding of intracellular protein-protein interaction networks and aid better drug discovery.
Acknowledgements
This work was supported by grants from the British Heart Foundation (SP and JHW), Wellcome Trust (JHW and SP), and European Union (JBCF). LB is recipient of an Overseas Research PhD Scholarship and Tetley and Lupton Scholarship. Declaration of interest: The authors report no conflicts of interest. The authors alone are responsible for the content and writing of the paper.
References
- Gavin AC, Bosche M, Krause R, Grandi P, Marzioch M, Bauer A, Schultz J, Rick JM, Michon AM, Cruciat CM, Remor M, Hofert C, Schelder M, Brajenovic M, Ruffner H, Merino A, Klein K, Hudak M, Dickson D, Rudi T, Gnau V, Bauch A, Bastuck S, Huhse B, Leutwein C, Heurtier MA, Copley RR, Edelmann A, Querfurth E, Rybin V, Drewes G, Raida M, Bouwmeester T, Bork P, Seraphin B, Kuster B, Neubauer G, Superti-Furga G. Functional organization of the yeast proteome by systematic analysis of protein complexes. Nature 2002; 415: 141–147
- Wittig I, Braun HP, Schägger H. Blue native PAGE. Nature Protocols 2006; 1: 418–428
- Akins RE, Levin P, Tuan RS. Cetyltrimethylammonium bromide discontinuous gel electrophoresis: Mr-based separation of proteins with retention of enzymatic activity. Anal Biochem 1992; 202: 172–178
- Drewes G, Bouwmeester T. Global approaches to protein-protein interactions. Curr Opin Cell Biol 2003; 15: 199–205
- Vasilescu J, Figeys D. Mapping protein-protein interactions by mass spectrometry. Curr Opin Biotechnol 2006; 17: 394–399
- Hultschig C, Kreutzberger J, Seitz H, Konthur Z, Bussow K, Lehrach H. Recent advances of protein microarrays. Curr Opin Chem Biol 2006; 10: 4–10
- Brachmann RK, Boeke JD. Tag games in yeast: the two-hybrid system and beyond. Curr Opin Biotechnol 1997; 8: 561–568
- Xenarios I, Fernandez E, Salwinski L, Duan XJ, Thompson MJ, Marcotte EM, Eisenberg D. DIP: the database of interacting proteins: 2001 update. Nucleic Acids Res 2001; 29: 239–241
- Fields S, Song OK. A novel genetic system to detect protein-protein interactions. Nature 1989; 340: 245–246
- Wallach D, Boldin MP, Kovalenko AV, Malinin NL, Mett IL, Camonis JH. The yeast two-hybrid screening technique and its use in the study of protein-protein interactions in apoptosis. Curr Opin Immunol 1998; 10: 131–136
- Chien CT, Bartel PL, Sternglanz R, Fields S. The two-hybrid system: a method to identify and clone genes for proteins that interact with a protein of interest. Proc Natl Acad Sci USA 1991; 88: 9578–9582
- Wallin E, von Heijne G. Genome-wide analysis of integral membrane proteins from eubacterial, archaean, and eukaryotic organisms. Protein Sci 1998; 7: 1029–1038
- Muller DJ, Wu N, Palczewski K. Vertebrate membrane proteins: structure, function, and insights from biophysical approaches. Pharmacol Rev 2008; 60: 43–78
- Reiss T. Drug discovery of the future: the implications of the human genome project. Trends Biotechnol 2001; 19: 496–499
- Stagljar I, Fields S. Analysis of membrane protein interactions using yeast-based technologies. Trends Biochem Sci 2002; 27: 559–563
- Aronheim A, Engelberg D, Li NX, Alalawi N, Schlessinger J, Karin M. Membrane targeting of the nucleotide exchange factor Sos is sufficient for activating the Ras signaling pathway. Cell 1994; 78: 949–961
- Broder YC, Katz S, Aronheim A. The Ras recruitment system, a novel approach to the study of protein-protein interactions. Curr Biol 1998; 8: 1121–1124
- Aronheim A. Protein recruitment systems for the analysis of protein +/( protein interactions. Methods 2001; 24: 29–34
- Hubsman M, Yudkovsky G, Aronheim A. A novel approach for the identification of protein-protein interaction with integral membrane proteins. Nucl Acids Res 2001; 29: e18
- Isakoff SJ, Cardozo T, Andreev J, Li Z, Ferguson KM, Abagyan R, Lemmon MA, Aronheim A, Skolnik EY. Identification and analysis of PH domain-containing targets of phosphatidylinositol 3-kinase using a novel in vivo assay in yeast. EMBO J 1998; 17: 5374–5387
- Aronheim A, Zandi E, Hennemann H, Elledge SJ, Karin M. Isolation of an AP-1 repressor by a novel method for detecting protein-protein interactions. Mol Cell Biol 1997; 17: 3094–3102
- Ehrhard KN, Jacoby JJ, Fu XY, Jahn R, Dohlman HG. Use of G-protein fusions to monitor integral membrane protein-protein interactions in yeast. Nat Biotechnol 2000; 18: 1075–1079
- Johnsson N, Varshavsky A. Split ubiquitin as a sensor of protein of protein interactions in-vivo. Proc Natl Acad Sci USA 1994; 91: 10340–10344
- Mockli N, Deplazes A, Hassa PO, Zhang ZL, Peter M, Hottiger MO, Stagljar I, Auerbach D. Yeast split-ubiquitin-based cytosolic screening system to detect interactions between transcriptionally active proteins. Biotechniques 2007; 42: 725–730
- Stagljar I, Korostensky C, Johnsson N, Heesen S. A genetic system based on split-ubiquitin for the analysis of interactions between membrane proteins in vivo. Proc Natl Acad Sci USA 1998; 95: 5187–5192
- Reinders A, Schulze W, Thaminy S, Stagljar I, Frommer WB, Ward JM. Intra- and intermolecular interactions in sucrose transporters at the plasma membrane detected by the split-ubiquitin system and functional assays. Structure 2002; 10: 763–772
- Massaad MJ, Herscovics A. Interaction of the endoplasmic reticulum alpha 1,2-mannosidase Mns1p with Rer1p using the split-ubiquitin system. J Cell Sci 2001; 114: 4629–4635
- Paumi CM, Menendez J, Arnoldo A, Engels K, Iyer KR, Thaminy S, Georgiev O, Barral Y, Michaelis S, Stagljar I. Mapping protein-protein interactions for the yeast ABC transporter Ycf1p by integrated split-ubiquitin membrane yeast two-hybrid analysis. Mol Cell 2007; 26: 15–25
- Thaminy S, Auerbach D, Arnoldo A, Stagljar I. Identification of novel ErbB3-interacting factors using the split-ubiquitin membrane yeast two-hybrid system. Genome Res 2003; 13: 1744–1753
- Vitale R, Buxbaum JD. Use of the split-ubiquitin two-hybrid system to identify proteins interacting with the Alzheimer proteins APP and LRP. Biol Bull 2004; 207: 167
- Matsuda S, Giliberto L, Matsuda Y, Davies P, McGowan E, Pickford F, Ghiso J, Frangione B, D'Adamio L. The familial dementia BRI2 gene binds the Alzheimer gene amyloid-beta precursor protein and inhibits amyloid-beta production. J Biol Chem 2005; 280: 28912–28916
- Obrdlik P, El-Bakkoury M, Hamacher T, Cappellaro C, Vilarino C, Fleischerf C, Ellerbrok H, Kamuzinzih R, Ledenth V, Blaudezi D, Sandersi D, Revuelta JL, Boles E, André B, Frommer WB. K+ channel interactions detected by a genetic system optimized for systematic studies of membrane protein interactions. Proc Natl Acad Sci USA 2004; 101: 12242–12247
- Dirnberger D, Messerschmid M, Baumeister R. An optimized split-ubiquitin cDNA-library screening system to identify novel interactors of the human Frizzled 1 receptor. Nucleic Acids Res 2008; 36: e37
- Yarden Y, Shilo B. SnapShot: EGFR signaling pathway. Cell 2007; 131: 1018
- Birge RB, Knudsen BS, Besser D, Hanafusa H. SH2 and SH3-containing adaptor proteins: redundant or independent mediators of intracellular signal transduction. Genes Cells 1996; 1: 595–613
- Zhang J, Lautar S. A yeast three-hybrid method to clone ternary protein complex components. Anal Biochem 1996; 242: 68–72
- Ozenberger BA, Young KH. Functional interaction of ligands and receptors of the hematopoietic superfamily in yeast. Mol Endocrinol 1995; 9: 1321–1329
- Crouin C, Arnaud M, Gesbert F, Camonis J, Bertoglio J. A yeast two-hybrid study of human p97/Gab2 interactions with its SH2 domain-containing binding partners. FEBS Lett 2001; 495: 148–153
- Osborne MA, Dalton S, Kochan JP. The yeast tribrid system – genetic detection of trans-phosphorylated ITAM-SH2-interactions. Biotechnology 1995; 13: 1474–1478
- Dudkina AS, Lindsley CW. Small molecule protein-protein inhibitors for the p53-MDM2 interaction. Curr Top Med Chem 2007; 7: 952–960
- Vassilev LT. MDM2 inhibitors for cancer therapy. Trends Mol Med 2007; 13: 23–31
- Ishi K, Sugawara F. A facile method to screen inhibitors of protein-protein interactions including MDM2-p53 displayed on T7 phage. Biochem Pharmacol 2008; 75: 1743–1750
- Vidal M, Brachmann RK, Fattaey A, Harlow E, Boeke JD. Reverse two-hybrid and one-hybrid systems to detect dissociation of protein-protein and DNA-protein interactions. Proc Natl Acad Sci USA 1996; 93: 10315–10320
- Huang J, Schreiber SL. A yeast genetic system for selecting small molecule inhibitors of protein-protein interactions in nanodroplets. Proc Natl Acad Sci USA 1997; 94: 13396–13401
- Henthorn DC, Jaxa-Chamiec AA, Meldrum E. A GAL4-based yeast three-hybrid system for the identification of small molecule-target protein interactions. Biochem Pharmacol 2002; 63: 1619–1628
- Dirnberger D, Unsin G, Schlenker S, Reichel C. A small-molecule-protein interaction system with split-ubiquitin as sensor. Chembiochem 2006; 7: 936–942
- Auerbach D, Thaminy S, Hottiger MO, Stagljar I. The post-genomic era of interactive proteomics: facts and perspectives. Proteomics 2002; 2: 611–623
- McCraith S, Holtzman T, Moss B, Fields S. Genome-wide analysis of vaccinia virus protein-protein interactions. Proc Natl Acad Sci USA 2000; 97: 4879–4884
- Rain JC, Selig L, De Reuse H, Battaglia V, Reverdy C, Simon S, Lenzen G, Petel F, Wojcik J, Schachter V, Chemama Y, Labigne A, Legrain P. The protein-protein interaction map of Helicobacter pylori. Nature 2001; 409: 211–215
- Miller JP, Lo RS, Ben-Hur A, Desmarais C, Stagljar I, Noble WS, Fields S. Large-scale identification of yeast integral membrane protein interactions. Proc Natl Acad Sci USA 2005; 102: 12123–12128
- Golemis EA, Serebriiskii I, Law SF. The yeast two-hybrid system: criteria for detecting physiologically significant protein-protein interactions. Curr Issues Mol Biol 1999; 1: 31–45
- Serebriiskii I, Estojak J, Berman M, Golemis EA. Approaches to detecting false positives in yeast two-hybrid systems. Biotechniques 2000; 28: 328–330
- Piehler J. New methodologies for measuring protein interactions in vivo and in vitro. Curr Opin Struct Biol 2005; 15: 4–14
- Villalobos V, Naik S, Piwnica-Worms D. Current state of imaging protein-protein interactions in vivo with genetically encoded reporters. Annu Rev Biomed Eng 2007; 9: 321–349
- Nyfeler B, Michnick SW, Hauri H-P. Capturing protein interactions in the secretory pathway of living cells. Proc Natl Acad Sci USA 2005; 102: 6350–6355
- Lim RP, Misra A, Wu Z, Thanabalu T. Analysis of conformational changes in WASP using a split YFP. Biochem Biophys Res Commun 2007; 362: 1085–1089
- Barnard E, McFerran NV, Trudgett A, Nelson J, Timson DJ. Development and implementation of split-GFP-based bimolecular fluorescence complementation (BiFC) assays in yeast. Biochem Soc Trans 2008; 36: 479–482
- Blume-Jensen P, Hunter T. Oncogenic kinase signalling. Nature 2001; 411: 355–365