Abstract
Metastases, responsible for most of the solid tumor associated deaths, require angiogenesis and changes in endothelial cells. In this work, the effect of the secretomes of three breast tumor cell lines (MCF-7, MDA-MB-231 and ZR-75-30) on human umbilical vein endothelial cells (HUVEC) morphology was investigated. HUVEC treated with secretomes from breast cells were analyzed by confocal and time-lapse microscopy. Secretomes from ZR-75-30 and MDA-MB-231 cells modify the morphology and adhesion of HUVEC. These changes may provoke the loss of endothelial monolayer integrity. In consequence, tumor cells could have an increased access to circulation, which would then enhance metastasis.
Introduction
Angiogenesis is the mechanism by which new blood vessels are assembled from pre-existing vasculature. This physiological process proves to be indispensable for malignant growth (Weidner et al., Citation1991). A tumor distant from blood vessels cannot exceed a few millimeters in diameter, because, for growth, malignant cells require a vascular network that supplies both nutrients and oxygen (Gupta & Qin, Citation2003). On the other hand, the ability of malignant tumors to metastasize, their most lethal characteristic (Yoshida et al., Citation2000), most times depends on angiogenic or lymphangiogenic processes (Folkman, Citation1992). New vessels augment the opportunity of tumor cells to incorporate into the systemic circulation and to colonize distant organs; consequently, tumor vascularity correlates with poor prognosis (Matsuda et al., Citation2003).
Tumor cells located more than 100–200 μm away from the vascular network become hypoxic (Baronzio et al., Citation2000). This circumstance produces an angiogenic switch that activates the gene transcription of several proteins, some of which will be secreted (Sáinz-Jaspeado et al., Citation2013). The switch to the angiogenic phenotype involves a change in the local equilibrium between positive and negative regulators of the growth of microvessels (Vujasinović et al., Citation2006). That means that tumor cells send signals to the surrounding cells and modify their environments; this transformation results in an advantageous background for their growth and spread. Hypoxia, through the hypoxia-inducible factor (HIF) family of transcription factors, induces the secretion of pro-angiogenic factors including vascular endothelial growth factor (VEGF), angiopoietin 2 (Ang-2), platelet-derived growth factor (PDGF) and fibroblast growth factor (FGF) (Finger & Giaccia, Citation2010).
Other molecules that tumor cells secrete are metalloproteases as MMP-2 (Sims et al., Citation2011) and pro-inflammatory molecules, such as interleukin 8 (IL-8) (Li et al., Citation2013) and interleukin 6 (IL-6) (Kurebayashi, Citation2000). Lymphatic and blood endothelia are target tissues of these angiogenic and pro-inflammatory molecules (Dwyer et al., Citation2012; Lassoued et al., Citation2010; Veikkola et al., Citation2001); endothelial cells therefore respond to these molecular signals with cell division, migration and subsequent vessel formation in the tumor.
The complex angiogenic response comprises finely orchestrated sequential steps (i.e. activation of quiescent endothelial cells, formation of blood vessels and maturation of them) (Rivas et al., Citation2013). Angiogenesis requires that interendothelial junctions are broken down and endothelial cells undergo morphological changes which permit migration (Dvorak et al., Citation1999; Lamalice et al., Citation2007). These actions are achieved by proteins secreted in a tumor environment.
Previously, several groups had examined the effects of some of these proteins separately on blood endothelial cells. However, only few works have examined the effects of these soluble factors in combination, such as are secreted by tumoral cells. The necessity of studying the mixture of factors secreted by cells (secretomes) is reinforced by the fact that antiangiogenic therapies aimed at inhibiting the function of individual molecules (e.g. VEGF) have not been successful in many cases (Pàez-Ribes et al., Citation2009).
Breast cancer is one of the most metastatic malignancies. Despite recent progress in survival rates, many patients relapse, and the majority of them die from disseminated metastatic disease (Almendro et al., Citation2014). In consequence, cell lines derived from breast cancers are a good model for studying the effect of secretomes on vascular endothelium. In this work, we searched for the effect of conditioned media derived from breast cancer cell lines on HUVEC actin cytoskeleton reorganization, necessary for controlling the dynamics of cell shape and the mobilization of these cells. Additionally, we started the characterization of secretome components derived from breast cancer cell lines utilized in this study.
Methods
Materials
RPMI 1640 and M199 media, antibiotic-antimycotic 100× solution, collagenase type II and 0.25% trypsin-EDTA were purchased from GIBCO/BRL (Grand Island, NY); fetal bovine serum (FBS) was from Biowest (Nuaille, France). Sterile plastic material for tissue culture was acquired from Corning (Corning, NY). Neutralizing antibodies against human IL-8 and VEGF as human recombinant VEGF were from R&D Systems (Minneapolis, MN). Human recombinant IL-8 was from Biolegend (San Diego, CA) and human recombinant insulin was from Pisa (Guadalajara, Jalisco, Mexico). Endothelial mitogen was purchased from Biomedical Technologies and all other chemicals were from Sigma Aldrich (St. Louis, MO).
Cell cultures
Breast cancer cell lines (ZR-75-30, MDA-MDB-231 and MCF-7) were obtained from ATCC and they were cultured in RPMI medium supplemented with 10% FBS. Breast epithelial normal cell line MCF10A was also obtained from ATCC and was cultured in DMEM-F12K medium supplemented with 10% FBS, 100 mU/ml insulin, 1 μg/ml hydrocortisone and 20 ng/ml epithelial growth factor (EGF). All cells were grown under an atmosphere of 95% humidity and 5% CO2 at 37 °C.
Primary HUVEC cultures were obtained by proteolytic dissociation of the umbilical cord veins from normal deliveries, treated with collagenase type II (0.2 mg/ml), and cultured in M199 supplemented with 10% FBS, glutamine (2 mM), heparin (1 mg/ml), and endothelial mitogen (20 μg/ml), as previously described (Montiel-Dávalos et al., Citation2010).
Production of conditioned media (secretomes)
Breast cell lines were grown to confluence in 175 cm2 flasks. Then cell monolayers were washed six times with HEPES buffer (8.8 g/l), pH 7.4, in order to remove serum components. Next, cultures were incubated in 20 ml of serum free RPMI medium without phenol red for 48 h. Conditioned media were collected, centrifuged at 3000 rpm for 10 min and lyophilized. The resulting powders were dissolved in HEPES buffer (1/10 of the original volume) and dialyzed using a PM-12 ultra-filtration membrane (Millipore, Bedford, MA) for 48 h with a change of buffer at 24 h; the buffer volume was 200-fold that of the sample. To sterilize, the media were filtered using a 0.2 μm membrane. Control medium, which was not in contact with cells, was also produced, and no phenol red RPMI was lyophilized, dissolved, dialyzed and sterilized as conditioned media.
Detection of polymerized actin
HUVEC were seeded on glass slides in 12-well plates and grown to confluence. The culture medium was removed and then was added fresh M199 medium plus 10% of FBS: control or conditioned medium (1:1). After 24 h, cells were washed with buffer PBS 1X (NaCl 8 g/l, KCl 0.2 g/l, Na2HPO4 1.8 g/l, KH2PO4 0.2 g/l) pH 7.4, fixed with 4% paraformaldehyde and washed twice with PBS buffer. Cells were permeabilized with 1% Triton X-100 in PBS, washed twice with PBS 1X, incubated with 1% serum albumin in PBS and washed twice with PBS 1X. Then, they were incubated with fluorescent phalloidin (which binds to polymerized actin) 1 h at 37 °C and observed by confocal microscopy.
Image analysis
After actin immunolocalization, slides were used for image analysis and pictures were taken using Leica confocal microscopy (DM IRB TCS SP-2 Germany) with images of 1260× magnification and 2800ab pixels.
Afterwards, images were processed with the image J analysis software 1.47m (NIH; 2012), analyzed and segmented using color threshold (24 bits) RGB. Then, they were converted to grayscale (8 bits) images and binarized to measure area (A), which was carried out by means of the software as the sum of the individual areas of the pixels composing the object. Perimeter (P) was obtained by adding the sizes of the pixels forming the image contour. Circularity (Ci) was measured as the ratio area/perimeter.
Time lapse microscopy
Endothelial cells were cultured until they were confluent; the initial culture medium was removed and then, fresh M199 medium plus 10% FBS was added: control or ZR-75-30 conditioned medium (1:1). HUVEC were recorded with an inverse Axiovert 200M microscope equipped with an incubation chamber, an Axiocam MRm camera and an AxioVison software (all from Zeiss) taking one frame every 3 min for up to 24 h.
Determination of media constituents
In order to evaluate media constituents, the Bio-Plex suspension array system (Bio-Rad, Hercules, CA) was used. This is a microsphere-based immunoassay, which utilizes Luminex™ beads coupled to specific antibodies, as an analyte capture platform. In total, 25 μl of each secretome were used for detecting the secreted factors. The samples were added in duplicate to 96-well plates containing polystyrene beads from the 27-plex assay kit, and the beads were filter-washed twice with Bio-Plex wash buffer using a vacuum manifold (Millipore, Bedford, MA). Human cytokine standards were added to the antibody-conjugated beads, and incubated in the dark on a platform shaker for 30 min. After incubation, the samples and standards were removed by vacuum, and the beads were filter-washed three times with Bio-Plex wash buffer. A 1:50 dilution of biotinylated detection antibody was added to the beads, followed by incubation in the dark on a platform shaker for 30 min. Subsequently, the beads were washed three times and reacted with a 1:100 dilution of streptavidin-phycoerythrin (PE) for 10 min. The beads were washed three times as described above, resuspended in Bio-Plex assay buffer, and analyzed on a Bio-Plex plate reader.
Neutralization of IL-8 and VEGF in secretomes
To block the function of IL-8 and VEGF in secretomes, neutralizing antibodies were used. To this, HUVEC were seeded at confluence and simultaneously secretomes and neutralizing antibodies against IL-8 (400 ng/ml) and VEGF (150 ng/ml) were added for 24 h. After this time, cells were fixed with glutaraldehyde (1.1%) and stained with crystal violet. Morphology was observed and photographed in an inverted microscopy (Olympus, model CKX41) using a camera (Moticam 1000). To test the activity of neutralizing antibodies, positive controls were performed. To this, human recombinant IL-8 (100 ng/ml) was used to induce migration of MDA-MB-231 cells (Li et al., Citation2014b) as it was previously described (López-Marure et al., Citation2011). Migrating cells were photographed at 0, 24, 48 and 96 h, then the percentage of open wound area was calculated using TScratch software (Gebäck et al., Citation2009). Likewise, HUVEC were treated with human recombinant VEGF (10 ng/ml) using M199 medium without endothelial mitogen and proliferation was determined by crystal violet staining.
Crystal violet staining
HUVEC were seeded in 96-well plates (3 × 103/well) and treated with conditioned media (1:1) for 72 h. Next, cells were fixed with 1.1% glutaraldehyde and then stained with 0.1% violet crystal in 200 mM formic acid buffer solution pH 6.0. Stain was solved in 10% acetic acid and was read at 595 nm.
Results
Secretome from breast cancer cell lines alters morphology and actin cytoskeletal organization of HUVEC
To explore if conditioned media derived from breast cancer cell lines have an effect on HUVEC morphology, we selected three cell lines with different tumorigenicity and metastatic capabilities (Li et al., Citation2014a; Xia et al., Citation2012). HUVEC monolayers were treated with conditioned media of cell lines MCF-7 (low invasive), MDA-MB-231 (high invasive) and ZR-75-30 (high invasive). HUVEC treated with ZR-75-30 or MDA-MB-231 cell secretomes for 24 h showed a more elongated shape; endothelial cells treated with ZR-75-30 cells secretome exhibited an evidently different form, compared with endothelial cells treated with control medium (, top panel). Confocal images of fixed cells stained with fluorescent phalloidin corroborated this morphological change. In HUVEC treated with the media obtained from the three breast cancer cells, both a rearrangement of polymerized actin and a formation of stress fibers were clearly visible (, bottom panel).
Figure 1. Effect of breast tumor cells conditioned media on morphology of endothelial cells. (A) Upper light microscopy micrographs were taken 24 h after treatments. Lower micrographs are confocal microscope images. Cells were incubated with fluorescent phalloidin (24 h after treatment) to detect polymerized actin. Analysis of area (B) and circularity (C) of endothelial cells after their exposure to conditioned media. This is a representative experiment of three performed independently. Statistical analysis was performed using one-way analysis of variance (ANOVA-test) with GraphPad Prism software version 5.01. *A p-value of <.05 was considered statistically significant.
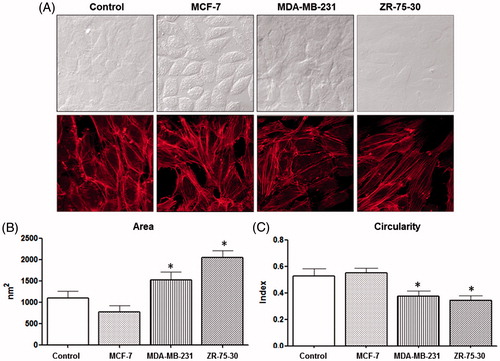
To quantify this morphological change, we utilized a software that measures cell circularity. HUVEC treated with ZR-75-30-conditioned medium showed a greater loss of roundness in comparison with MCF-7 medium-treated cells, which did not present any diminution (). On the other hand, MDA-MB-231 and ZR-75-30 media-treated cells displayed an area increase compared with other treatments ().
In order to evaluate if the secretome derived from normal breast cells can induce changes on morphology of HUVEC, MCF10A cells secretome was tested on endothelial monolayer. Results did not show a change on morphology after 24 h of treatment (see Supplementary material, Figure S1, available online).
Secretome of ZR-75-30 cells diminished the attachment of HUVEC
With the purpose of studying in more detail the morphologic and motility changes in respect to time, we utilized the Time Lapse method and micrographed both the cells treated with control medium and those treated with ZR-75-30 secretome every 3 min. As observed in , besides the change in cell shape, ZR-75-30 media provoked a diminution of adhered endothelial cells. When endothelial monolayer was treated with ZR-75-30 cells secretome, cell detachment from glass surface and cell-cell contacts rupture took place, as revealed by videos 1 and 2 in the Supplementary material. To investigate if conditioned media of breast tumor cells caused a decrease in the number of endothelial cells on the plate, we stained HUVEC with violet crystal after 72 h of treatment with conditioned media. As exhibited in , only the ZR-75-30 cells medium provoked a reduction in the cell numbers of HUVEC attached to the well. The decrease of the number of adhered cells to the surface culture (glass in time lapse microscopy and actin detection assay, and plastic in crystal violet assay) was also observed when surface culture were covered with gelatin.
Figure 2. Effect of breast tumor cells conditioned media on morphology and attachment of endothelial cells. (A) Endothelial cells treated with control medium (see video 1, Supplementary material) and (B) Endothelial cells treated with ZR-75-30 cells conditioned medium (see video 2, Supplementary material). Micrographs were obtained at 0, 6, 12, 18 and 24 h of treatment by time lapse microscopy. This assay is representative of the three independent experiments performed.
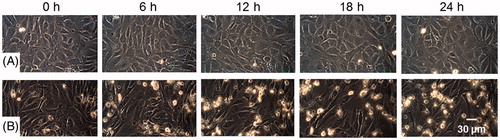
Figure 3. Effect of breast tumor cells conditioned media on the cell number. Cells were treated with the corresponding conditioned media for 72 h and cell proliferation was evaluated by crystal violet staining. Each value was estimated as a percentage of control (cells without any treatment). At least, three independent trials of the experiments were performed, and the data are expressed as the means ± standard deviation (SD). Statistical analysis was performed using one-way analysis of variance (ANOVA-test) with GraphPad Prism software version 5.01. *A p-value of <.05 was considered statistically significant.
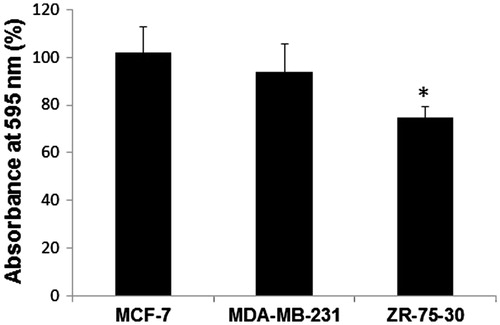
ZR-75-30 cell secretome has a greater concentration of pro-inflammatory and chemoattracting proteins compared with the other media
For secretome characterization, we used a multiplex kit to determine the presence or absence of 27 proteins involved in angiogenesis and metastasis processes. We could detected only 14 of the 27 pro-inflammatory interleukins and chemoattracting molecules evaluated. In the medium produced by ZR-75-30 cells, we identified a higher expression of these molecules, compared with the MCF-7 and MDA-MB-231 secretomes (). It is important to mention that VEGF, a good candidate to account for morphologic changes, is present in a major concentration in the MCF-7 cells secretome. Interestingly, the only molecule secreted by both MDA-MB-231 and ZR-75-30 cells was IL-8.
Table 1. Proteins detected in the secretome of breast tumor cell lines. Secretome was obtained according to methods. Expression of proteins was determined by Bio-Plex suspension array system.
Considering that IL-8 is present in both the secretomes of ZR-75-30 and MDA-MB-231 cells and that it correlates with the effect of endothelial cell elongation, a neutralizing antibody was used and cell morphology was observed. IL-8 blocking did not abolish the morphologic change induced by ZR-75-30 cells secretome on HUVEC (). We corroborated the neutralizing activity of anti-IL-8 antibody with a migration assay of MDA-MB-231 cells using wounds ( and Supplementary material, Figure S2).
Figure 4. Effect of neutralizing antibody anti-IL-8 on morphology of endothelial cells treated with ZR-75-30 cells secretome. (A) HUVEC treated with control medium, conditioned medium of ZR-75-30 cells and conditioned medium of ZR-75-30 cells plus neutralizing antibody anti IL-8 (0.4 μg/ml). Cells were treated for 24 h and then stained with crystal violet. (B) Positive control to test neutralizing activity of anti-IL-8. Cells were treated with IL-8 (100 ng/ml) and IL-8 plus neutralizing antibody against IL-8 (2 μg/ml) for 24, 48 and 96 h, then migration of MDA-MB-231 cells was evaluated mediating a wound assay. Analysis of the percentage of open wound area was performed with TScratch software. Data are shown as the percentage of open wound area in comparison with IL-8-treated cells. Results are expressed as the mean ± SD from three independent experiments. *p < .05 compared with IL-8-treated cells.
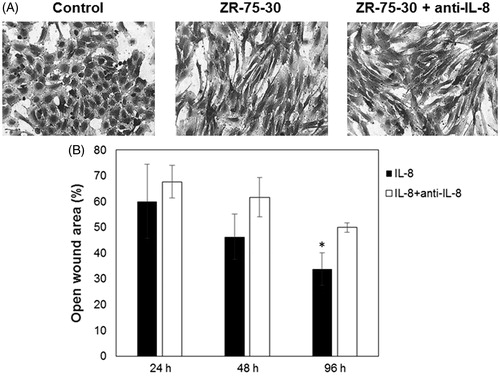
On the other hand, in spite of VEGF concentration was low in ZR-75-30 cells secretome, we performed an experiment with neutralizing VEGF antibody and HUVEC morphology was observed. Similar to IL-8 experiments, anti-VEGF did not abolish the morphologic change induced by ZR-75-30 cells secretome (). Positive control of antibody was achieved with a proliferation assay of HUVEC (data not shown).
Figure 5. Effect of neutralizing antibody anti-VEGF on morphology of endothelial cells treated with ZR-75-30 cells secretome. HUVEC were treated with control medium, secretome of ZR-75-30 cells and secretome of ZR-75-30 cells plus neutralizing antibody anti-VEGF (150 ng/ml) for 24 h, and then stained with crystal violet.
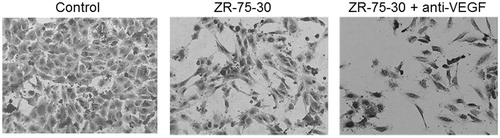
Discussion
Angiogenic switch is a complex process that promotes malignancy growth (Bergers & Benjamin, Citation2001). Tumor neighboring cells, including tumor cells, release several soluble factors which can bind to receptors in vessels. As a consequence, the vascular network augments due to excess of proangiogenic signalling. It is known that angiogenic factors such as VEGF and FGF are involved in tumor angiogenesis; however, it is important to study the orchestrated effect of all secreted factors. The interactions of endothelial cells with the components of tumor cell secretome determine whether endothelial cells will undergo an angiogenic process or not.
Our results showed a morphological effect in HUVEC treated with conditioned media of breast tumor cell lines. Conditioned media from the more invasive tumor cells (ZR-75-30 and MDA-MB-231) provoked an elongation of HUVEC. This morphological change correlates with a reorganization of actin cytoskeleton and formation of stress fibers (). Microvessel leakage are present in tumoral angiogenesis (Nagy et al., Citation2012). This endothelial damage correlates with stress fibers and favors the escape of tumor cells into circulation.
Experiments shown in and indicate that after treatment with ZR-75-30 cells secretome, the number of endothelial cells adhered to the plate was diminished. Detachment could be a consequence of adherence loss and/or cell death. To investigate if endothelial cells were dying with treatment, we performed Annexin V assays after 72 h of incubation with ZR-75-30-conditioned medium. The results showed the absence of cell death (data not shown). In conclusion, treated endothelial cells detached from glass or plastic, but they were still alive after a 72 h treatment.
It has been established that the presence of stress fibers is related with an augmented endothelial cell contraction and an increase of permeability (García-Ponce et al., Citation2015). In endothelial monolayers, barrier function depends on two factors: adhesion (among neighboring cells and to substrate) and contractile movements (Michel & Curry, Citation1999; Wojciak-Stothard & Ridley, Citation2002). The imbalance between the adhesive and contractile forces results in barrier dysfunction. Both competing forces are intimately linked through the endothelial cytoskeleton.
One of the first steps in the angiogenesis process is the disaggregation of individual endothelial cells and posterior migration to construct the new vessels. Abolished VE-cadherin function causes adhesion loss and culminates in an increase of vascular permeability. This loss of function can be due to several causes; one of them is the reduction of VE-cadherin cell surface expression. By cytometry, we analyzed the cell membrane presence of VE-cadherin, PECAM and JAM-1, adhesion molecules involved in interendothelial adhesion. None of these molecules changed their membrane expression after treatment with breast cancer cell line conditioned media (data not shown). It has been reported that tension and mechanical forces are also other ways to destroy the function of adhesion molecules (Gavard, Citation2008). The augmented contractile forces caused by stress fibers can provoke the disruption of intercellular adhesion and a gain in cell motility. In addition to the loss of interendothelial adhesion, a decrease of substrate adhesion to the glass cover or plastic well could be also involved. In any case, the cytoskeleton plays an important role in both types of interactions. Actin filaments are involved in controlling the dynamics of cell shape, cell polarity, cell-substrate adhesion and cell-cell adhesion (Schnittler et al., Citation2014).
We think that the effect observed on endothelial cells is probably the result of several factors. In angiogenesis, synergistic effects are common (Han et al., Citation2014; Kryczek et al., Citation2005). For this reason, it remains important to characterize the components of tumoral secretomes. Especially in the ZR-75-30 conditioned medium, data obtained by a suspension array system indicated the presence of several molecules related with the angiogenic process (see ).
It is known that VEGF has an effect on endothelial morphology and adhesion (Le Guelte et al., Citation2011). In contrast, results shown in indicate that VEGF is more concentrated in the MCF-7 conditioned medium. That is, VEGF concentration was inversely proportional to the morphologic change and is thus unlikely to be the molecule responsible for the elongation and detachment of endothelial cells. In addition, supporting our conclusion, the neutralizing anti-VEGF antibody was not able to inhibit the mentioned change.
The molecules IL-8, IL-6, MCP-1, MIP-1α and MIP-1β are also of interest, since they are involved in angiogenesis. However, IL-6 is not present in the MDA-MB-231 secretome, which also evokes a morphologic effect on endothelium. This situation also occurs with the MCP-1, MIP-1α and MIP-1β molecules.
Our results with neutralizing anti-IL-8 indicate that this molecule is not responsible for changes induced by ZR-75-30 cells secretome on the morphology of HUVEC, despite reports that IL-8 elicited the retraction of human lung microvascular endothelial cells (HMVECs) and immortalized dermal human microvascular endothelial cells (HMECs) (Schraufstatter et al., Citation2001).
It is important to highlight that the molecules tested in this work are not the only ones that could be responsible of the effect of secretomes on HUVEC; therefore, our group will continue looking for the molecule or molecules responsible not only for endothelial elongation, but also for attachment loss. We consider that these secreted factors prepare endothelium to respond quickly to other factors, which induce more specific pathways, such as proliferation and migration. Additionally to soluble factors, we consider it interesting to explore the effect of extracellular vesicles present in the secretomes on the morphology of HUVEC because they are a relevant mechanism of communication between cells in the tumor microenvironment (Green et al., Citation2015).
IMBC_1229057_Supplementary_Materials.zip
Download Zip (786.2 KB)Disclosure statement
The authors report no conflicts of interest. The authors alone are responsible for the content and writing of the paper.
References
- Almendro V, Kim HJ, Cheng YK, Gonen M, Itzkovitz S, Argani P, et al. 2014. Genetic and phenotypic diversity in breast tumor metastases. Cancer Res 74:1338–1348.
- Baronzio GF, Gramaglia A, Baronzio A, Freitas I. 2000. Influence of tumor microenvironment on thermoresponse: biologic and clinical implications. In: Madame Curie Bioscience Database [Internet]. Austin, TX: Landes Bioscience. Available from: http://www.ncbi.nlm.nih.gov/books/NBK6245/.
- Bergers G, Benjamin LE. 2001. Tumorigenesis and the angiogenic switch. Nat Rev Cancer 3:401–410.
- Dvorak HF, Nagy JA, Feng D, Brown LF, Dvorak AM. 1999. Vascular permeability factor/vascular endothelial growth factor and the significance of microvascular hyperpermeability in angiogenesis. Curr Top Microbiol Immunol 237:97–132.
- Dwyer J, Hebda JK, Le Guelte A, Galan-Moya EM, Smith SS, Azzi S, Bidere N, Gavard J. 2012. Glioblastoma cell-secreted interleukin-8 induces brain endothelial cell permeability via CXCR2. PLoS One 7:e45562. doi: 10.1371/journal.pone.0045562.
- Finger EC, Giaccia AJ. 2010. Hipoxia, inflammation, and tumor microenvironment in metastatic disease. Cancer Metastasis Rev 29:285–293.
- Folkman J. 1992. The role of angiogenesis in tumor growth. Semin Cancer Biol 3:65–71.
- García-Ponce A, Citalán-Madrid AF, Velázquez-Avila M, Vargas-Robles H, Schnoor M. 2015. The role of actin-binding proteins in the control of endothelial barrier integrity. Thromb Haemost 113:20–36.
- Gavard J. 2008. Breaking the VE-cadherin bonds. FEBS Lett 583:1–6.
- Gebäck T, Schulz MM, Koumoutsakos P, Detmar M. 2009. TScratch: a novel and simple software tool for automated analysis of monolayer wound healing assays. Biotechniques 46:265–274.
- Green TM, Alpaugh ML, Barsky SH, Rappa G, Lorico A. 2015. Breast cancer-derived extracellular vesicles: characterization and contribution to the metastatic phenotype. Biomed Res In 2015:634865. doi: 10.1155/2015/634865.
- Gupta MK, Qin NY. 2003. Mechanism and its regulation of tumor-induced angiogenesis. World J Gastroenterol 9:1144–1155.
- Han EC, Lee J, Ryu SW, Choi C. 2014. Tumor-conditioned Gr-1(+)CD11b(+) myeloid cells induce angiogenesis through the synergistic action of CCL2 and CXCL16 in vitro. Biochem Biophys Res Commun 443:1218–1225.
- Kryczek I, Lange A, Mottram P, Alvarez X, Cheng P, Hogan M, et al. 2005. CXCL12 and vascular endothelial growth factor synergistically induce neoangiogenesis in human ovarian cancers. Cancer Res 65:465–472.
- Kurebayashi J. 2000. Regulation of interleukin-6 secretion from breast cancer cells and its clinical implications. Breast Cancer 7:124–129.
- Lamalice L, Le Boeuf F, Huot J. 2007. Endothelial cell migration during angiogenesis. Circ Res 100:782–794.
- Lassoued W, Murphy D, Tsai J, Oueslati R, Thurston G, Lee WM. 2010. Effect of VEGF and VEGF Trap on vascular endothelial cell signaling in tumors. Cancer Biol Ther 10:1326 –1333.
- Le Guelte A, Dwyer J, Gavard J. 2011. Jumping the barrier: VE- cadherin, VEGF and other angiogenic modifiers in cancer. Biol Cell 103:593–605.
- Li C, Guo S, Shi T. 2013. Role of NF-κB activation in matrix metalloproteinase 9, vascular endothelial growth factor and interleukin 8 expression and secretion in human breast cancer cells. Cell Biochem Funct 31:263–268.
- Li J, Yang XF, Ren XH, Meng XJ, Huang HY, Zhao QH, et al. 2014a. Stable SET knockdown in breast cell carcinoma inhibits cell migration and invasion. Biochem Biophys Res Commun 453:7–12.
- Li X, Zhao SJ, Shi HL, Qiu SP, Xie JQ, Wu H, et al. 2014b. Berberine hydrochloride IL-8 dependently inhibits invasion and IL-8-independently promotes cell apoptosis in MDA-MB-231 cells. Oncol Rep 32:2777–2788.
- López-Marure R, Contreras PG, Dillon JS. 2011. Effects of dehydroepiandrosterone on proliferation, migration, and death of breast cancer cells. Eur J Pharmacol 660:268–274.
- Matsuda Y, Hagio M, Ishiwata T, Nestin T. 2003. A novel angiogenesis marker and possible target for tumor angiogenesis. World J Gastroenterol 19:42–48.
- Michel CC, Curry FE. 1999. Microvascular permeability. Physiol Rev 79:703–761.
- Montiel-Dávalos A, Ibarra-Sánchez MJ, Ventura-Gallegos JL, Alfaro-Moreno E, López-Marure R. 2010. Oxidative stress and apoptosis are induced in human endothelial cells exposed to urban particulate matter. Toxicol In Vitro 24:135–141.
- Nagy JA, Dvorak AM, Dvorak HF. 2012. Vascular hyperpermeability, angiogenesis, and stroma generation. Cold Spring Harb Perspect Med 2:a006544. doi: 10.1101/cshperspect.a006544.
- Pàez-Ribes M, Allen E, Hudock J, Takeda T, Okuyama H, Viñals F, et al. 2009. Antiangiogenic therapy elicits malignant progression of tumors to increased local invasion and distant metastasis. Cancer Cell 15:220–231.
- Rivas V, Carmona R, Muñoz-Chápuli R, Mendiola M, Nogués L, Reglero C, et al. 2013. Developmental and tumoral vascularization is regulated by G protein-coupled receptor kinase 2. J Clin Invest 123:4714–4730.
- Sáinz-Jaspeado M, Huertas-Martinez J, Lagares-Tena L, Martin Liberal J, Mateo-Lozano S, de Alava E, et al. 2013. EphA2-induced angiogenesis in ewing sarcoma cells works through bFGF production and is dependent on caveolin-1. PLoS One 8:e71449. doi: 10.1371/journal.pone.0071449.
- Schnittler H, Taha M, Schnittler MO, Taha AA, Lindemann N, Seebach J. 2014. Actin filament dynamics and endothelial cell junctions: the Ying and Yang between stabilization and motion. Cell Tissue Res 355:529–543.
- Schraufstatter IU, Chung J, Burger M. 2001. IL-8 activates endothelial cell CXCR1 and CXCR2 through Rho and Rac signaling pathways. Am J Physiol Lung Cell Mol Physiol 280:L1094–1103.
- Sims JD, McCready J, Jay DG. 2011. Extracellular heat shock protein (Hsp)70 and Hsp90α assist in matrix metalloproteinase-2 activation and breast cancer cell migration and invasion. PLoS One 6:e18848. doi: 10.1371/journal.pone.0018848.
- Veikkola T, Jussila L, Makinen T, Karpanen T, Jeltsch M, Petrova TV, et al. 2001. Signalling via vascular endothelial growth factor receptor-3 is sufficient for lymphangiogenesis in transgenic mice. EMBO J 20:1223–1231.
- Vujasinović T, Buta M, Markićević M, Nikolić-Vukosavljević D. 2006. Angiogenesis: bFGF and VEGF in breast carcinoma. Arch Oncol 14:126–130.
- Weidner N, Semple JP, Welch WR, Folkman J. 1991. Tumor angiogenesis and metastasis – correlation in invasive breast carcinoma. N Engl J Med 324:1–8.
- Wojciak-Stothard B, Ridley AJ. 2002. Rho GTPases and the regulation of endothelial permeability. Vascul Pharmacol 39:187–199.
- Xia TS, Wang GZ, Ding Q, Liu XA, Zhou WB, Zhang YF, et al. 2012. Bone metastasis in a novel breast cancer mouse model containing human breast and human bone. Breast Cancer Res Treat 132:471–486.
- Yoshida BA, Sokoloff MM, Welch DR, Rinker-Schaeffer CW. 2000. Metastasis-supressor genes: a review and perspective on an emerging field. J Natl Cancer Inst 92:1717–1730.