Abstract
Transient Receptor Potential (TRP) channels are mostly Ca2+ permeable cation channels. Transient Receptor Potential Melastatin-like 2 (TRPM2) is expressed in neurological tissues such as brain, dorsal root ganglia (DRG) neurons, hippocampus and also liver, heart and kidney. The SH-SY5Y cells are mostly used as a cellular model of neurodegenerative diseases, Alzheimer's and Parkinson's diseases. Curcumin, shows phenolic structure, synthesized by Curcuma longa L. (turmeric), has powerful non-enzymatically antioxidant effects compared with Vitamin E. Hence, we aimed to investigate that effects of curcumin on TRPM2 cation channel currents using the whole-cell Patch-Clamp method, Ca2+ signaling, apoptosis and cell viability (MTT) assays, reactive oxygen species (ROS) production, mitochondrial membrane potential levels, caspase 3 and caspase 9 activities in TRPM2 transfected SH-SY5Y neuroblastoma cells. For this aim, we designed four experimental groups named; control, curcumin, transfected and transfected + curcumin groups. Cytosolic free calcium concentrations were higher in transfected group compared with curcumin and transfected + curcumin group. Moreover, these data examined with whole-cell Patch-Clamp recordings of single cells in all groups. ROS levels were significantly higher in transfected group than in transfected + curcumin group. Apoptosis levels in transfected + curcumin group were lower than in transfected group. Procaspase 9 and procaspase 3 levels measured by western blotting and caspase 3 and caspase 9 levels by spectrophotometric methods show that TRPM2 transfected cells are more tended to apoptosis. In conclusion, curcumin strongly induces modulator effects on TRPM2-mediated Ca2+ influx caused by ROS and caspase 3 and 9 processes in SH-SY5Y neuroblastoma cells.
Introduction
Oxidative stress is known as destructive effects of abnormally increased reactive oxygen species (ROS) on cellular metabolism and organic essential substances such as carbohydrates, proteins, nucleic acids and lipids. Due to the nervous system vulnerability, oxidative stress plays an important role in the pathogenesis of neurodegenerative diseases. To prevent these harmful effects of ROS and keep under control the oxidative–antioxidative balance, cells use different intracellular and extracellular originated mechanisms. Cells integrate metabolic processes and use non-enzymatic antioxidants with foods such as vitamins, flavonoids and carotenoids (Halliwell & Gutteridge, Citation2015). Curcumin, [1,7-Bis-(4-hydroxy-3-methoxyphenyl)-hepta-1,6-diene-3,5-dione], is an active polyphenolic compound of Curcuma longa L. (turmeric), and has more potent antioxidant properties compared with vitamin E (Ak & Gülçin, Citation2008; S et al., Citation1999). Several studies have been shown powerful antioxidant effects of curcumin on intracellular antioxidant defense system with in vitro experiments (Jayaprakasha et al., Citation2006; Uğuz et al., 2016). Transient Receptor Potential (TRP) channel superfamily has 28 members in mammals and mostly non-selective cation channels, divided into six different subfamilies according to similarity of amino acid sequences which named; Ankyrin (TRPA; 1), Canonical (TRPC; 1-7), Melastatin (TRPM; 1-8), Mucolipin (TRPML; 1-3), Policystin (TRPP; 2, 3, 5), Vanilloid (TRPV; 1-6). TRPM2 is a Ca2+ permeable non-selective cation channel that can be activated by adenosine diphosphate ribose (ADPR), cyclic adenosine diphosphate ribose (cADPR) and nicotinamide adenine dinucleotide (β-NAD+) on unique ADPR pyrophosphatase activity region in the C-terminal Nudix domain (Clapham, Citation2003; Wehage et al., Citation2002). Numerous studies showed that it could act as a cellular oxidant sensor and also independently activated by experimental oxidative stress agent, H2O2 (Hara et al., Citation2002; Kaneko et al., Citation2006; Nazıroğlu & Lückhoff, Citation2008a, Citation2008b). However, we have less data about whether antioxidants have inhibitor or modulator effects on TRPM2 and whether they can gate TRPM2 channels (Çelik & Nazıroğlu, Citation2012; Nazıroğlu et al., Citation2013). SH-SY5Y human neuroblastoma cell line is widely used for in vitro model of Alzheimer's and Parkinson's diseases (Kovalevich & Langford, Citation2013). Calcium ion (Ca2+) has important roles of neuronal cells such as excitation, neurotransmitter secretion, cellular signal transduction and amplification processes. The concentration difference of Ca2+ between intracellular and extracellular media is under controlled by different kind of ion channels. Excessive increase of intracellular free Ca2+ concentration ([Ca2+]i) has been related with neurological diseases and also activation of cellular apoptosis signal pathways via caspases (Naziroglu, Citation2011). To our knowledge, there has been no prior study on interactions of curcumin with Ca2+ signaling, based on TRPM2 cation channels in neuronal cells. The main objective of the current study is to investigate the molecular effects of curcumin on TRPM2 channels in transfected SH-SH5Y neuroblastoma cells. Hence, we evaluated the effect of curcumin on TRPM2 channel activation/inhibition manner by whole-cell electrophysiology recordings as well as intracellular calcium signaling analysis in SH-SY5Y cells. Moreover, we aimed to reveal that curcumin administration how affects the TRPM2, poly-ADP-ribose polymerase 1 (PARP1), procaspase 3 and 9 protein expression levels in the study groups by western blotting. We also examined that other potential intracellular signaling cascades such as caspase activities, cell survival, apoptosis, intracellular ROS production and mitochondrial membrane potential levels by using spectrophotometric techniques.
Materials and methods
Cell culture and human TRPM2 pcDNA transfection
SH-SY5Y human neuroblastoma cell line (CRL-2266) was purchased from ATCC (Manassas, VA). Cells were cultured in Dulbecco's Modified Eagle Medium (DMEM, Sigma Aldrich, St. Louis, MO) and HAM'S F12 (Biochrom, Berlin, Germany) cell culture medium 1:1 ratio containing 10% fetal bovine serum (FBS, Life Technologies) and 100 U/ml penicillin +100 μg/ml streptomycin (Biochrom, Berlin, Germany) at 37 °C in 5% CO2 humidified incubator (Heal Force HF90, Smart Cell, Japan). After cells have reached 80–85% confluence, washed with fresh 1× Phosphate Buffered Saline (PBS, Biochrom, Berlin, Germany), detached with %0.25 Trypsin–EDTA (Sigma Aldrich, St. Louis, MO) and split into the new culture flasks. Cells were used at passages 5–9.
Transfection process applied to both transfected and transfected + curcumin groups described according to the manufacturer procedure. One day before transfection, TransFast Transfection Reagent (Promega, Madison, WI) was prepared and stored at −30 °C freezer. After 24 h, reagent was waited at room temperature to melting and transfection process was initiated. For every 80% confluence T25 sized flasks, 5 μg of plasmid construct enhanced green fluorescent protein encoding TRPM2/TRPM2 splice variant (pcDNA3-EGFP-TRPM2/TRPM2ΔC) plasmids were used as described elsewhere (Nazıroğlu & Lückhoff, Citation2008b; Wehage et al., Citation2002). Plasmids were diluted in 2 ml fresh medium, and reagent was added three fold of used DNA, mixed up and incubated during 15 min in the laminar flow (Cryste, South Korea) sterile conditions at room temperature. All growth medium was removed, and the mixture was gently transferred into the flasks. After 1 h incubation, 4 ml fresh medium was added and incubated during 48 h at 37 °C and 5% CO2. At the end of time, the medium was renewed in transfected group and again incubated for 24 h at 37 °C and 5% CO2. Curcumin dissolved in dimethyl sulphoxide (DMSO) and cells were treated with curcumin at concentration of 5 μM. Final concentration of DMSO in the media was less than 0.1% in curcumin and transfected + curcumin groups. Curcumin including medium used for incubation in curcumin and transfected + curcumin groups for 24 h at 37 °C and 5% CO2. Transfection efficiency checked by EGFP shining (excitation 488 nm and emission 509 nm) under the fluorescent attached inverted microscope (Zeiss, AxioVert 40 CFL, Germany), and cells were ready to the experiments.
Study groups
Numerous studies can be found showing the effective dose of curcumin between 1 and 10 μM in literature. In this study, similarly our pilot study (unpublished data), it was elected that 5 μM curcumin incubation is optimal dose for cell survival (Morabito et al., Citation2015; Qian et al., Citation2015; Wang et al., Citation2009; Uguz et al., Citation2016).
Group I was control group; SH-SY5Y cells were seeded, and incubated their medium for 48 h (37 °C and 5% CO2). Medium was renewed 24 h before the experiment day.
Group II was curcumin group; SH-SY5Y cells were seeded, and incubated their medium for 48 h and after then incubated with fresh medium including 5 μM curcumin for 24 h (37 °C and 5% CO2).
Group III was transfected group; SH-SY5Y cells were transfected by using transfection kit and pcDNA during 48 h incubated and after then with fresh medium for 24 h (37 °C and 5% CO2). For the transfection procedure, SH-SY5Y cells were seeded, and incubated in their medium for 48 h by using transfection kit and pcDNA. Incubated with fresh medium (curcumin free) for 24 h (37 °C and 5% CO2) after transfection.
Group IV was transfected + curcumin group; SH-SY5Y cells were seeded, and incubated in their medium for 48 h by using transfection kit and pcDNA. Incubated with fresh medium including 5 μM curcumin for 24 h (37 °C and 5% CO2) after transfection.
Electrophysiology
Patch-Clamp experiments have been described in detail elsewhere (Nazıroğlu & Lückhoff, Citation2008a, Citation2008b). SH-SY5Y cells were studied in the whole-cell configuration, using an EPC 10 USB amplifier equipped with a personal computer with PatchMaster software (HEKA, Lamprecht, Germany). The standard extracellular bath solution contained; 145 mM NaCl, 1 mM MgCl2, 1 mM CaCl2, 5 mM KCl, 10 mM HEPES [4- (2-hydroxyethyl)21-piperazine ethane sulfonic acid] and 10 mM D-glucose, pH adjusted to 7.4 with KOH. For Na+ free solutions, Na+ was replaced by 150 mM N-methyl-d-glucamine (NMDG+), and the pH was adjusted to 7.35 with HCl. The osmolarity of the solution was 300 mOsmol/l. The pipette solution contained; 145 mM L-glutamic acid, 8 mM NaCl, 8 mM ethylene-glycol tetraacetic acid (EGTA), 1 mM MgCl2, 1 mM CaCl2, 10 mM HEPES and the pH was adjusted to 7.2 with CsOH. It is well known that TRPM2 channels are activated in the presence of high intracellular Ca2+ concentration (Starkus et al., Citation2007). The Ca2+ concentration of intracellular solution in TRPM2 experiments was set to 1 μM (0.886 mM Ca2+, 1 mM Cs-EGTA). The Ca2+ concentrations of the intracellular and extracellular solutions were calculated by using the MAXC-program (http://maxchelator.stanford.edu/downloads.htm).
Cells were held at a potential of −60 mV, and current–voltage (I–V) relations were obtained from voltage ramps from −90 to +60 mV applied for >400 ms. To produce patch pipettes, borosilicate glass tubes with filament (outer diameter 1.5 mm, inner diameter 0.86 mm and 10 cm in length, Sutter Instruments Co., Novato, CA) were used. The access resistance of the whole-cell recording electrodes was between 3 and 7 MΩ. The serial resistance was less than 20 MΩ, and leak currents were compensated. In TRPM2 whole-cell patch-clamp experiments, the cells were stimulated by a H2O2 (10 mM). As an inhibitor agent we used N-(p-amylcinnamoyl) anthranilic acid (ACA, 25 μM) because of 2-aminoethoxydiphenyl borate (2-APB) can react with curcumin (Priyadarsini, Citation2014). Stock ACA solution was solved in DMSO, before the each experiment, in extracellular bath solution, ACA (25 μM) and H2O2 (10 mM) were diluted to reach the required final concentrations, and the pH of these solutions were adjusted to 7.4 with KOH. All experiments were carried out at room temperature (approx. 23 °C). H2O2 and ACA and all other chemicals were obtained from Sigma Aldrich (St. Louis, MO). Whole-cell experiments applied for all groups and current changes were recorded.
Determination of intracellular free calcium concentration ([Ca2+]i)
To determination of [Ca2+]i in all groups of cells were loaded with 4 μM of Fura-2 AM (Invitrogen, Carlsbad, CA), the cells were washed and gently resuspended in loading buffer (Na-HEPES solution (pH 7.4) containing; NaCl 140 mM, KCl 4.7 mM, CaCl2 1.2 mM, MgCl2 1.1 mM, d-glucose 10 mM and HEPES 10 mM) with 2 × 106 cells/ml for 45 min at 37 °C shaker bath in the dark (Pariente et al., Citation2001; Uğuz et al., Citation2009). The four groups were exposed to cumene hydroperoxide (CHPx) to stimulate of Ca2+ signals. Fluorescence was recorded from 2 ml aliquots of magnetically stirred cellular suspension at 37 °C using a spectrofluorometer (Varian Cary Eclipse, Sydney, Australia) with excitation wavelengths of 340 and 380 nm and emission at 505 nm. Changes in [Ca2+]i were monitored using the Fura-2 340/380 nm fluorescence ratio and were calibrated according to the method of Grynkiewicz et al. (Citation1985). Concentrations of Ca2+ in the SH-SY5Y cells were estimated using the integral of the rise in [Ca2+]i for 150 s after addition of CHPx (0.1 mM). Ca2+ concentrations are expressed in nM quantities, taking a sample every second as previously described (Uğuz et al., Citation2009).
Western blotting
For all groups, western blot analyses were performed using the standard procedure. To detection of TRPM2, procaspase 3, procaspase 9 and PARP1 expression levels, SH-SY5Y cells were homogenized in lysis buffer, the supernatants removed and conserved after centrifuge at 16,000 g for 20 min. The total protein assessed using Bradford reagent at 595 nm. For immunoblotting step, equal amounts of proteins (30 μg of each sample) were loaded into 6–13% sodium dodecyl sulfate-polyacrylamide gel, and after electrophoresis, they transferred to a nitrocellulose membrane. The blot was blocked for 1 h at room temperature with 5% non-fat dry milk in Tris Buffered Saline (TBS) with 0.1% Tween 20 (TBST). After that the membranes were incubated with primary antibodies, TRPM2 (Anti-TRPM2 antibody, Thermo Scientific Fischer, Waltham, MA) and others (Caspase 9/p35/p10 Polyclonal Antibody; Caspase 3, p17-specific Polyclonal Antibody; beta Actin Polyclonal Antibody; PARP1 Polyclonal Antibody Proteintech) and secondary antibody (Rabbit IgG, HRP-linked whole Ab (from donkey), GE Healthcare, Amersham, UK). Bands were visualized using ECL Western HRP Substrate (Millipore Luminate Forte), and imaging was achieved with (G:Box, Syngene, UK) and normalized against β-actin protein (Kahya et al., Citation2017).
Measurement of intracellular ROS production
To determine ROS activity, approximately 106 cells were loaded with 2 μM dihydrorhodamine-123 (DHR-123, Molecular Probes) by incubation at 37 °C for 30 min as previously described (Bejarano et al., Citation2011). This non-fluorescent cell permeable compound once enter the cell, it turns fluorescent upon oxidation to yield rhodamine-123 (Rh-123), fluorescence being proportional to ROS generation. The fluorescence intensity of Rh-123 was measured in a fluorescence multiplate reader (Tecan Infinite 200 Pro, Austria). Excitation was set at 488 nm and emission at 543 nm. Treatments were carried out in triplicate. Data were calculated as fold increase experimental to control.
Measurement of mitochondrial membrane potential (MMP)
SH-SY5Y cells were incubated with 1 μM concentration of 5,5′,6,6′-tetrachloro-1,1′,3,3′-tetraethylbenzimi-dazolylcarbocyanine iodide (JC-1, Santa Cruz Biotechnology) for 15 min at 37 °C as previously described (Uguz & Naziroglu, Citation2012; Uguz et al., Citation2012). The lipophilic cationic dye, JC-1, exhibits potential-dependent accumulation in mitochondria. It indicates mitochondrial depolarization by a decrease in the red-to-green fluorescence intensity ratio. After incubation with JC-1, the dye was removed, and the cells were washed in PBS. The green JC-1 signal was measured at the excitation wavelength of 485 nm and the emission wavelength of 535 nm, and the red signal, at the excitation wavelength of 540 nm and the emission wavelength of 590 nm. Fluorescence changes were analyzed using a fluorescence spectrophotometer (Tecan Infinite 200 Pro, Austria). Treatments were carried out in triplicate. Data are presented as emission ratios (590/535). Changes in mitochondrial membrane potential were quantified as the integral of the decrease in JC-1 fluorescence ratio of experimental/control.
Assay for caspase 3 and 9 activities
To determine caspase 3 and 9 activities, SH-SY5Y cells were sonicated, and cell lysates were incubated with 2 ml of substrate solution (20 mM HEPES [pH 7.4], 2 mM EDTA, 0.1% CHAPS, 5 mM DTT and 8.25 μM of caspase substrate) for 1 h at 37 °C as previously described (Uguz & Naziroglu, Citation2012). The activities of caspase 3 and 9 were calculated from the cleavage of the respective specific fluorogenic substrate (AC-DEVD-AMC for caspase 3 and AC-LEHDAMC for caspase 9, were purchased from Bachem, Switzerland). Substrate cleavage was measured with a fluorescence multiplate reader (Tecan Infinite 200 Pro, Austria) with an excitation wavelength of 360 nm and an emission wavelength of 460 nm. Preliminary experiments confirmed that caspase 3 and 9 substrate cleavage was not detected in the presence of DEVD-CMK or z-LEHDFMK, which inhibit caspase 3 and 9, respectively. The data were calculated as fluorescence units per milligram of protein (Uğuz et al., Citation2009).
Cell viability (MTT) assay
Cell viability was evaluated by the MTT assay based on the ability of viable cells to convert a water-soluble, yellow tetrazolium salt into a water-insoluble, purple formazan product. The enzymatic reduction of the tetrazolium salt happens only in living, metabolically active cells, not in dead ones. After the treatments, the cells were detached and MTT dye was added to each tube and then cells were incubated for 90 min at 37 °C in a shaking water bath. The supernatant was discarded, and 150 μl DMSO was added to dissolve the formazan crystals. Experiments were carried out in duplicate. Optical density was measured in an automatic multiplate reader (Tecan Infinite 200 Pro) at 490 and 650 nm (as reference wavelength) and presented as the fold experimental/control as described elsewhere (Uğuz et al., Citation2009).
Apoptosis assay
The APOPercentageTM assay (Biocolor Ltd., Belfast, Northern Ireland) was performed according to the manufacturer instruction. The APOPercentageTM assay is a dye-uptake assay, which stains only the apoptotic cells with a red dye. When the membrane of apoptotic cell lost its asymmetry, the APOPercentage dye is bonded phosphatidyl serine lipids actively and transported into cells, staining apoptotic cells red, thus allowing the detection of apoptosis by a multiplate reader (Tecan Infinite 200 Pro, Austria) as previously described, elsewhere (Uguz & Naziroglu, Citation2012). Data were calculated as fold increase experimental to control.
Statistical analyses
All results were expressed as mean ± standard deviation (SD). The significance of differences among 3 groups was assessed with a Mann–Whitney U test. Data were analyzed using the SPSS, version 9.05 (SPSS, Inc., Chicago, IL). P < 0.05 was considered significant.
Results
Curcumin acts as an inhibitor on TRPM2 currents
Effects of transfection and curcumin incubation on TRPM2 currents are shown in and . TRPM2 currents were triggered by extracellularly using H2O2 (10 mM). The currents were non-selective cationic currents with a reversal potential close to 0 mV, as previously found to be typical of TRPM2 currents and were readily blocked in the inside direction. When the Na+ in the bath was replaced by the large impermanent cation NMDG+ no such currents were observed (). When the cells were studied that were transfected with the vector only, without TRPM2, such negative control data were obtained on every experimental day of studying TRPM2 (). Currents density was significantly (p < 0.001) higher in the transfected group than in control or transfected + curcumin groups (. When H2O2 was added to the chamber, there was a typical delay of 2–4 min before TRPM2 cation currents initiation within 5 min, the currents reached a clear plateau in many experiments (). Curcumin incubation inhibited H2O2-mediated activation of TRPM2 channels. The antioxidant curcumin caused completely inhibition of TRPM2 currents in transfected + curcumin group. The results of these experiments are presented in . The data with curcumin would support the idea that H2O2 directly activates the TRPM2 channels or indirectly acts by initiating a metabolic cascade resulting in the production of cytosolic factors such as oxidative stress and ADPR that are responsible for the activation of TRPM2 channel activity. We also tested effects of TRPM2 antagonist ACA on SH-SY5Y cells. We tested whether extracellular ACA (25 μM) would prevent or attenuate the induction of TRPM2 currents induced by oxidative stress. ACA inhibited H2O2-induced TRPM2 currents in transfected group shown in . However, current densities were significantly (p < 0.001) higher in the ACA administered transfected group than in the transfected + curcumin group (). Hence, ACA induces less blockage of H2O2-induced TRPM2 currents in compared to curcumin.
Figure 1. Effects of curcumin on TRPM2 channel activity in SH-SY5Y neuroblastoma cells. (A) Control record: original recordings from SH-SY5Y cells. (B) Non-transfected control group, was tested whether H2O2 cause on the cellular cationic currents or not. (C) Non-transfected but curcumin incubated records of cells were to test any relationship between curcumin incubation and H2O2 induction on the currents. (D) TRPM2 currents in the transfected SH-SY5Y cells were stimulated by H2O2 (10 mM) in the chamber (bath) solution and they were inhibited by ACA (25 μM) in the bath. (E) I–V curve of panel D: current-voltage relationships of whole-cell currents in the presence of various extracellular cations, activators and inhibitors as indicated (same experiments as in panel D). (F) TRPM2 currents inhibited by curcumin incubation in the transfected + curcumin group. The cells were incubated with curcumin (5 μM) after transfection for 24 h and then they were not gated by H2O2 administration (in the patch chamber). The holding potential was -60 mV. NMDG+ time is shown where the normal bath solution (140 nM Na+) was exchanged to a solution with NMDG+ as main cation (150 mM, Ca2+ free solution). W.C.: Whole cell.
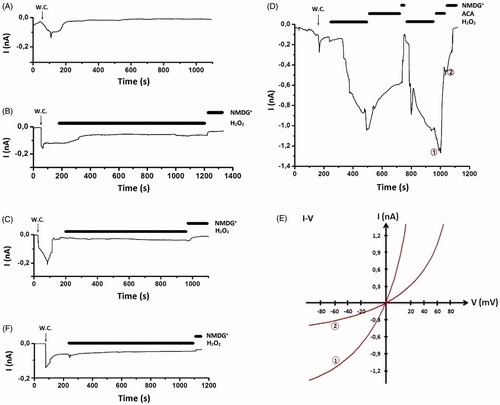
Figure 2. Effects of curcumin on TRPM2 cation channel current densities in SH-SY5Y neuroblastoma cells. The cells were in vitro treated with H2O2 (10 mM) and ACA (25 μM). For each of the experiments, the initial current density (divided to the cell capacitance, which is a measure of cell size) was calculated after administration of H2O2 (mean ± SD). The numbers in parentheses indicate n number of groups. Significant stimulation and inhibition of currents were indicated with letters a; p < 0.001 vs control group, b; p < 0.001 vs curcumin group, c; p < 0.001 vs transfected group. Cur: curcumin; Trans: transfected; Trans + Cur: express transfected + curcumin group.
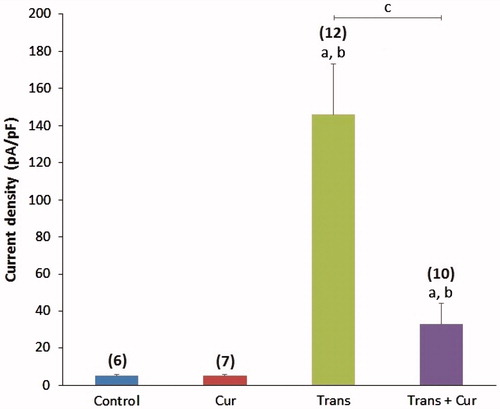
Effects of curcumin on intracellular free calcium concentration ([Ca2+]i)
The [Ca2+]i results shown as line plots and columns are presented in , respectively. SH-SY5Y cells are not natively express oxidative stress sensitive TRPM2 cation channels (see western blot results). In the TRPM2 transfected SH-SY5Y cells, TRPM2 channels were activated by CHPx but not curcumin incubated group. [Ca2+]i levels were significantly (p < 0.05) higher in the transfected group compared with the control group. 24 h incubation with curcumin induced further TRPM2 channel blocking effects and the [Ca2+]i was also significantly (p < 0.05) lower in the transfected + curcumin group than in the transfected group.
Figure 3. A. Calcium release from four groups of SH-SY5Y cells following stimulation with 25 μM cumene hydroperoxide (CHPx). Original time course chart recordings showing [Ca2+]i transients in SH-SY5Y cells. B. Bar charts showing mean ± SD data for [Ca2+]i from H2O2 stimulated groups of SH-SY5Y cells (n = 6 for each). Note that the significant elevation in [Ca2+]i for SH-SY5Y cells compared to control group. a; p < 0.05 vs control group, b; p < 0.05 vs transfected group. Cur: curcumin; Trans: transfected; Trans + Cur: express transfected + curcumin group.
![Figure 3. A. Calcium release from four groups of SH-SY5Y cells following stimulation with 25 μM cumene hydroperoxide (CHPx). Original time course chart recordings showing [Ca2+]i transients in SH-SY5Y cells. B. Bar charts showing mean ± SD data for [Ca2+]i from H2O2 stimulated groups of SH-SY5Y cells (n = 6 for each). Note that the significant elevation in [Ca2+]i for SH-SY5Y cells compared to control group. a; p < 0.05 vs control group, b; p < 0.05 vs transfected group. Cur: curcumin; Trans: transfected; Trans + Cur: express transfected + curcumin group.](/cms/asset/efce4925-000c-4aa8-989f-dd2d00ef21e3/imbc_a_1318224_f0003_c.jpg)
Effects of transfection and curcumin administration on TRPM2 channel protein, PARP1, procaspase 3 and 9 expression levels
TRPM2 expression was higher in transfected group as expected, and lower in transfected + curcumin group, but we could not conclude that the curcumin whether altered the expression levels of TRPM2 or not (). PARP1 is an indicator enzyme on the breaks of DNA strands, it initiates repair mechanisms after binding the damaged DNA (Zorn & Annunziata, Citation2016). PARP1 level was higher in transfected group compared with transfected + curcumin group but not found any significant difference between curcumin and control groups (). Procaspase 3 and procaspase 9 are precursor enzymes of apoptosis, they are reduced by apoptotic processes when they are converted to their active forms, caspase 3 and 9, in the apoptotic pathways (Liu et al., Citation1996). We assayed procaspase 3 and 9 activities as indicator of apoptosis. The procaspase 3 and 9 activities were markedly (p < 0.05) lower in transfected group than in the control. The procaspase 3 and 9 activities were decreased in the transfected and transfected + curcumin groups as compared to control group ().
Figure 4. Effects of TRPM2 transfection and/or curcumin incubation on the TRPM2 channel protein (A), PARP1 enzyme (B), procaspase 9 and procaspase 3 enzymes expression levels (C) relative densities represented of these protein levels respectively in SH-SY5Y cells. Cur: curcumin; Trans: transfected; Trans + Cur: express transfected + curcumin group.
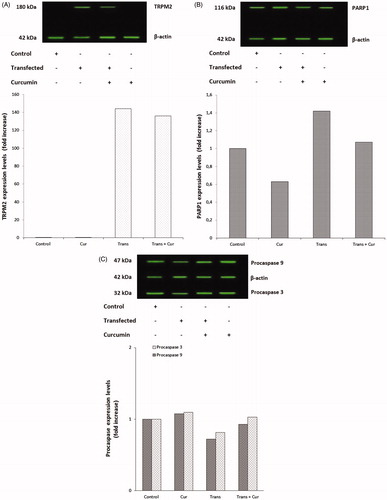
Effects of curcumin administration on reactive oxygen species (ROS) production
ROS production levels were determined by dihydrorhodamine 123 (DHR-123) non-fluorescent dye. The ROS levels obtained from the study groups were shown in . The results indicated that ROS levels were significantly (p < 0.001) lower in curcumin group compared with the control group samples. Moreover, curcumin incubation markedly (p < 0.001) decreased the ROS level in the transfected + curcumin group compared with the transfected group.
Figure 5. Effects of curcumin administration on ROS production levels in SH-SY5Y cells (mean ± SD; n = 8). The values were expressed as fold increase over the control level (experimental/control). a; p < 0.001 vs control group, b; p < 0.001 vs curcumin group, c; p < 0.001 vs transfected group. Cur: curcumin; Trans: transfected; Trans + Cur: express transfected + curcumin group.
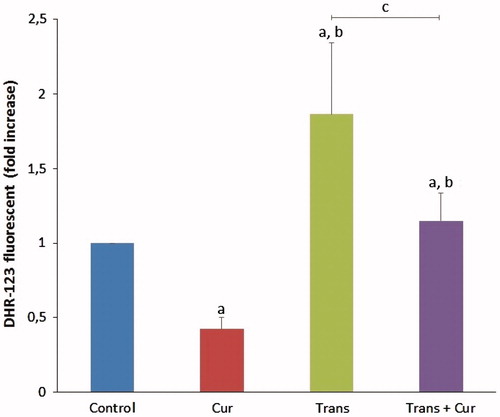
Effects of curcumin on mitochondrial membrane potential (MMP) levels
The mitochondrial membrane potential levels were given in . The MMP levels in transfected group were significantly higher than in control and curcumin groups (p < 0.05). Curcumin incubation significantly decreased MMP levels in transfected + curcumin group compared with transfected group (p < 0.05).
Figure 6. Effects of curcumin on mitochondrial membrane depolarization levels in transfected and non-transfected SH-SY5Y cells (mean ± SD; n = 8). The values were expressed as fold increase over the control level (experimental/control). a; p < 0.05 vs control group, b; p < 0.05 vs curcumin group, c; p < 0.05 vs transfected group. Cur: curcumin; Trans: transfected; Trans + Cur: express transfected + curcumin group.
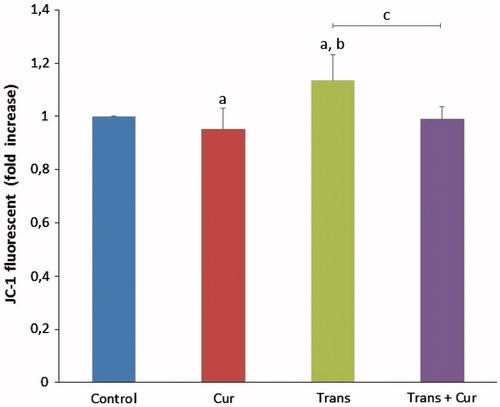
Effects of curcumin on caspase 3 and 9 activities
The caspase 3 levels in transfected group were significantly higher than in control group (p < 0.05). It was indicated that both enzyme activities were highest levels in the transfected group and curcumin incubation after transfection decreased caspase 3 and 9 activation in transfected + curcumin group compared with transfected group (p < 0.05) ().
Figure 7. Effects of curcumin on caspase 3 (A) and caspase 9 enzyme activity values (B) in SH-SY5Y neuroblastoma cells (mean ± SD; n = 8). The values were expressed as fold increase over the control level (experimental/control). a; p < 0.05 vs control group, b; p < 0.05 vs transfected group. Cur: curcumin; Trans: transfected; Trans + Cur: express transfected + curcumin group.
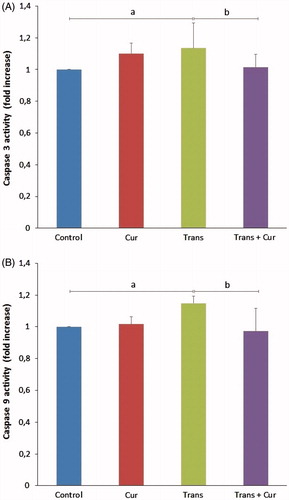
Effects of curcumin on cell viability
MTT assay results showed that the cell viability of the transfected group was significantly decreased (p < 0.05) compared with control and curcumin groups, there were several significant differences in the transfected and transfected + curcumin groups (p < 0.05). It was clearly demonstrated that curcumin incubation has positive effects on cell viability via decreasing apoptosis levels ().
Figure 8. Effects of curcumin on cell viability levels in SH-SY5Y cells (mean ± SD; n = 8). The values were expressed as fold increase over the control level (experimental/control). a; p < 0.05 vs control group, b; p < 0.05 vs curcumin group, c; p < 0.05 vs transfected group. Cur: curcumin; Trans: transfected; Trans + Cur: express transfected + curcumin group.
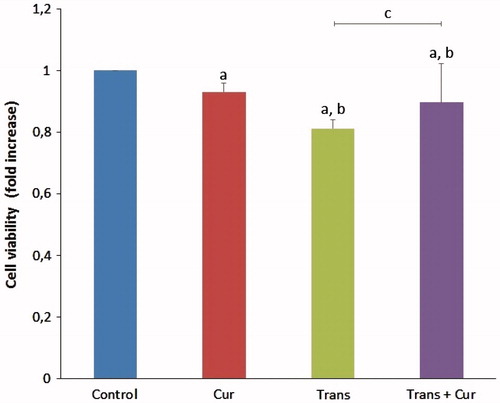
Effects of curcumin on apoptosis levels
The effects of curcumin on apoptosis levels in SHSY5Y cells are shown in . The mean apoptosis levels determined as fold increase in curcumin, transfected and transfected + curcumin groups compared with control group. The apoptosis levels were significantly lower in the curcumin group (p < 0.05), compared with the transfected group samples (p < 0.001). However, apoptosis levels were significantly decreased in transfected + curcumin group (p < 0.001). Hence, we observed the protective effects of curcumin on apoptosis levels in SH-SY5Y neuroblastoma cells.
Figure 9. Effects of curcumin on apoptosis levels in SH-SY5Y cells (mean ± SD; n = 8). The values were expressed as fold increase over the control level (experimental/control). a; p < 0.05 vs control group, b; p < 0.05 vs curcumin group, c; p < 0.001 vs transfected group. Cur: curcumin; Trans: transfected; Trans + Cur: express transfected + curcumin group.
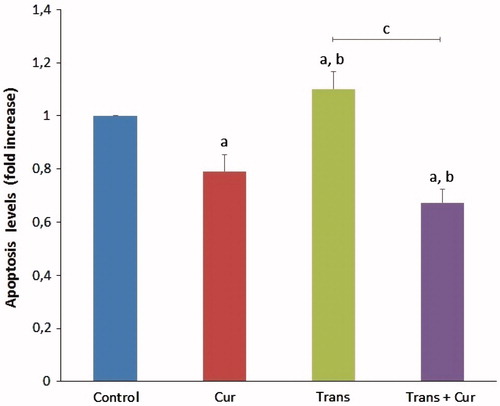
Discussion
Oxidative/antioxidative balance in the cells is involved in normal cellular and metabolic functions but impairments of redox status can induce apoptotic processes, ischemia and neurodegeneration. Regulation of [Ca2+]i has also critical importance in both routine and pathological cellular responses. In the present study, the dissipation of MMP was significantly inhibited, and SHSY-5Y cell survival was continued by curcumin treatment in contrast with our findings some reports showed that curcumin incubation might help the PC-12 cells to survive by inducing the overexpression of Bcl-2, but also reducing the loss of MMP (Chen et al., Citation2006). In the current study, TRPM2 channels were constitutively activated by an experimental ROS, CHPx, and a H2O2 analog. Different strategies have been used to inhibit oxidative stress-induced TRPM2 channel gating, but in this study we showed that for the first time curcumin incubation almost totally inhibited channel currents and related Ca+2 entry in SH-SY5Y neuronal cells. Generation of ROS including H2O2 by consumption of molecular oxygen is enhanced by oxidative stress-induced mitochondrial depolarization and excessive influx of Ca2+ through the cells. TRPM2 channels are calcium permeable non-specific cation channels, widely expressed in brain and nervous system tissues including dorsal root ganglia (DRG) and hippocampus (Fonfria et al., Citation2006). First year of the millennium, Perraud et al. showed that TRPM2 channels can be activated by intracellular ADPR and it regulated Ca2+ entry in natively TRPM2 expressing U937 monocyte cell line. TRPM2 gating by ADPR directly or by ROS indirectly, is highly unique because it contains both ion channel and enzymatic activity in the C-terminal of the channel, namely ADPR pyrophosphatase domain (Perraud et al., Citation2001). ADPR production enhances by ROS, however, Hara et al. and Naziroğlu and Lückhoff remarked that the same C-terminal Nudix homology region of TRPM2 channels also might be activated by ROS (H2O2) independently of the ADPR (Hara et al., Citation2002; Nazıroğlu & Lückhoff, Citation2008a). Recent years, so many studies have been aimed to reveal indirectly inhibitor effects of antioxidants and trace elements on TRPM2 channel cationic currents by scavenging the ROS generation, because of these channels play an oxidant sensor role in cellular physiology of neuronal cells and tissues. Although vitamin E, C and reduced glutathione (GSH) had not inhibitor effects (Nazıroğlu & Lückhoff, Citation2008b), it has been shown that selenium and melatonin act as inhibitor on TRPM2 channel currents in transfected Chinese hamster ovary (CHO) cells (Çelik & Nazıroğlu, Citation2012; Nazıroğlu et al., Citation2013). We have less data about the effects of curcumin on anionic channels, most of the studies having been focused on the interaction between curcumin and cationic channels such as several kinds of ion channels (Liu et al., Citation2006; Shin et al., Citation2011). Curcumin has nearly eightfold more antioxidant capacity than vitamin E and its effect on TRPM2 currents has not been experimented in neuronal like cells before. Latest findings have showed that non-selective TRPM2 cationic channel currents can be inhibited by extracellular curcumin administration in a dose dependent manner in cultured rat hepatocytes (Kheradpezhouh et al., Citation2016). The importance of the current study is that one of the novel findings explains an interaction between curcumin and TRPM2 channels in neuronal cells that is different from findings in similar studies. In our work, calcium signaling results demonstrated that the curcumin incubation inhibits Ca2+ release in curcumin administrated cells.
In a concentration-dependent manner curcumin both show activator and inhibitor effect on intracellular calcium signaling and cell proliferation. It has been proved that curcumin appears elevating intracellular calcium concentration-dependent MMP increase, may have cytotoxic effects over 40–50 μM doses on lung cancer cells (Xu et al., Citation2015). Additionally, it was shown that increasing of [Ca2+]i is associated with curcumin treatment via InsP3R related intracellular calcium stores. Unlike the other researches, we focused on the effect of low dose (5 μM) of curcumin on [Ca2+]i via TRPM2-mediated influx from extracellular media. Whole-cell Patch-Clamp recordings demonstrated that curcumin incubation after transfection almost completely inhibits TRPM2-mediated cationic currents induced by H2O2. Kheradpezhouh et al. (Citation2016) determined that 5 μM curcumin incubation may inhibit paracetamol- and H2O2-induced Ca2+ rise in rat hepatocytes. They also showed that TRPM2 cationic currents induced by intracellularly ADPR (1 mM), completely inhibited by after 15 min curcumin incubation in both human TRPM2 transfected HEK 293 T cells and cultured rat hepatocytes (Kheradpezhouh et al., Citation2016). However, we focused on the indirect inactivation of the TRPM2 non-selective currents induced by extracellularly H2O2 (10 mM) administration. When these two different researches assessed together, it can be postulated that two different inducers of the TRPM2 cation channel currents can be completely inhibited by acute curcumin incubation in transfected cells. On the other hand, it is concluded that curcumin blocks both intracellular and extracellular originated activation pathways of TRPM2. Because of the curcumin is a potent ROS scavenger, it has already been nominated by a number of studies as a neuroprotective and target therapeutic chemical for neurodegenerative diseases (Yang et al., Citation2005, Wang et al., Citation2005, Wang et al., Citation2009). Decreased intracellular ROS production levels and western blotting results of PARP1 expression, and increased procaspase 3 and procaspase 9 expression levels in the curcumin incubated group seems to curcumin targets the ROS related apoptotic processes in SH-SY5Y neuroblastoma cells. Curcumin can cause apoptosis by activating caspases in HL-60 cancer cell lines. It was also reported that caspase 3 and 9 activities may show alterations according to PARP1 expression level in the cells. These reports show that various doses of curcumin have different effects on cancer cell lines. Apoptosis related proteins including caspase 3 expression levels can change by administration of various doses of curcumin in glioblastoma cells. (Su et al., Citation2010). Taking into account all of these and together with our results confirmed that curcumin plays a dual role on cell survival and apoptotic processes in cancer cell lines (Zhang et al., Citation2012). Furthermore, the accuracy of our hypothesis was confirmed by spectrophotometrically observed assays that revealing reduced apoptosis, reduced cell viability (MTT) and reduced caspase 3 and 9 activity in the transfected + curcumin group.
In conclusion, the possible inhibitor effect of curcumin incubation on TRPM2 channel currents is that due to its ROS scavenger and oxidative-stress preventer ability. However we previously observed that effect of curcumin incubation MMP levels that this could be due to time dependent manner (Uğuz et al., 2016). If incubation time period was prolonged we might observe that curcumin pays a regulatory role on MMP levels in TRPM2 transfected SH-SY5Y neuronal cells.
Abbreviations | ||
[Ca2+]i | = | intracellular free calcium ion concentration |
ACA | = | N-(p-amylcinnamoyl) anthranilic acid |
Caspases | = | cysteine-dependent aspartate directed proteases |
CHPx | = | cumene hydroperoxide |
H2O2 | = | hydrogen peroxide |
InsP3R | = | inositol triphosphate receptor |
NMDG+ | = | N-methyl-d-glucamine |
PARP1 | = | Poly-ADP-ribose polymerase 1 |
ROS | = | reactive oxygen species |
TRP | = | Transient Receptor Potential |
TRPM2 | = | Transient Receptor Potential Melastatin-like 2 |
W.C. | = | whole cell |
Disclosure statement
The authors Ahmi ÖZ and Ömer ÇELİK declare that they have no conflicts of interest.
Additional information
Funding
References
- Ak T, Gülçin İ. 2008. Antioxidant and radical scavenging properties of curcumin. Chem Biol Interact 174:27–37.
- Bejarano I, Espino J, Barriga C, Reiter RJ, Pariente JA, Rodriguez AB. 2011. Pro-oxidant effect of melatonin in tumour leucocytes: relation with its cytotoxic and pro-apoptotic effects. Basic Clin Pharmacol Toxicol 108:14–20.
- Chen J, Tang XQ, Zhi JL, Cui Y, Yu HM, Tang EH, et al. 2006. Curcumin protects PC12 cells against 1-methyl-4-phenylpyridinium ion-induced apoptosis by bcl-2-mitochondria-ROS-iNOS pathway. Apoptosis 11:943–953.
- Clapham DE. 2003. TRP channels as cellular sensors. Nature 426:517–524.
- Çelik Ö, Naziroğlu M. 2012. Melatonin modulates apoptosis and TRPM2 channels in transfected cells activated by oxidative stress. Physiol Behav 107:458–465.
- Fonfria E, Murdock PR, Cusdin FS, Benham CD, Kelsell RE, Mcnulty S. 2006. Tissue distribution profiles of the human TRPM cation channel family. J Recept Signal Transduct Res 26:159–178.
- Grynkiewicz G, Poenie M, Tsien RY. 1985. A new generation of Ca2+ indicators with greatly improved fluorescence properties. J Biol Chem 260:3440–3450.
- Halliwell B, Gutteridge JM. 2015. Free Radicals in Biology and Medicine. USA: Oxford University Press.
- Hara Y, Wakamori M, Ishii M, Maeno E, Nishida M, Yoshida T, et al. 2002. LTRPC2 Ca2+-permeable channel activated by changes in redox status confers susceptibility to cell death. Mol Cell 9:163–173.
- Jayaprakasha G, Rao LJ, Sakariah K. 2006. Antioxidant activities of curcumin, demethoxycurcumin and bisdemethoxycurcumin. Food Chem 98:720–724.
- Kahya MC, Naz?roğlu M, Övey İS. 2017. Modulation of diabetes-induced oxidative stress, apoptosis, and Ca(2+) Entry Through TRPM2 and TRPV1 channels in dorsal root ganglion and hippocampus of diabetic rats by melatonin and selenium. Mol Neurobiol 54:2345–2360.
- Kaneko S, Kawakami S, Hara Y, Wakamori M, Itoh E, Minami T, et al. 2006. A critical role of TRPM2 in neuronal cell death by hydrogen peroxide. J Pharmacol Sci 101:66–76.
- Kheradpezhouh E, Barritt GJ, Rychkov GY. 2016. Curcumin inhibits activation of TRPM2 channels in rat hepatocytes. Redox Biol 7:1–7.
- Kovalevich J, Langford D. 2013. Considerations for the use of SH-SY5Y neuroblastoma cells in neurobiology. Methods Mol Biol 1078:9–21.
- Liu H, Danthi SJ, Enyeart JJ. 2006. Curcumin potently blocks Kv1.4 potassium channels. Biochem Biophys Res Commun 344:1161–1165.
- Liu X, Kim CN, Yang J, Jemmerson R, Wang X. 1996. Induction of apoptotic program in cell-free extracts: requirement for dATP and cytochrome c. Cell 86:147–157.
- Morabito R, Falliti G, Geraci A, Spada GL, Marino A. 2015. Curcumin protects -SH groups and sulphate transport after oxidative damage in human erythrocytes. Cell Physiol Biochem 36:345–357.
- Naziroglu M. 2011. TRPM2 cation channels, oxidative stress and neurological diseases: where are we now? Neurochem Res 36:355–366.
- Naziroğlu M, Lückhoff A. 2008a. A calcium influx pathway regulated separately by oxidative stress and ADP-Ribose in TRPM2 channels: single channel events. Neurochem Res 33:1256–1262.
- Naziroğlu M, Lückhoff A. 2008b. Effects of antioxidants on calcium influx through TRPM2 channels in transfected cells activated by hydrogen peroxide. J Neurol Sci 270:152–158.
- Naziroğlu M, Özgül C, Küçükayaz M, Çiğ B, Hebeisen S, Bal R. 2013. Selenium modulates oxidative stress‐induced TRPM2 cation channel currents in transfected Chinese hamster ovary cells. Basic Clin Pharmacol Toxicol 112:96–102.
- Pariente JA, Camello C, Camello PJ, Salido GM. 2001. Release of calcium from mitochondrial and nonmitochondrial intracellular stores in mouse pancreatic acinar cells by hydrogen peroxide. J Membr Biol 179:27–35.
- Perraud AL, Fleig A, Dunn CA, Bagley LA, Launay P, Schmitz C, et al. 2001. ADP-ribose gating of the calcium-permeable LTRPC2 channel revealed by Nudix motif homology. Nature 411:595–599.
- Priyadarsini KI. 2014. The chemistry of curcumin: from extraction to therapeutic agent. Molecules 19:20091–20112.
- Qian Y, Ma J, Guo X, Sun J, Yu Y, Cao B, et al. 2015. Curcumin enhances the radiosensitivity of U87 cells by inducing DUSP-2 up-regulation. Cell Physiol Biochem 35:1381–1393.
- S MK, Priyadarsini KI, Venkatesan P, Rao MN. 1999. Free radical scavenging ability and antioxidant efficiency of curcumin and its substituted analogue. Biophys Chem 80:85–91.
- Shin DH, Seo EY, Pang B, Nam JH, Kim HS, Kim WK, Kim SJ. 2011. Inhibition of Ca2+-release-activated Ca2+ channel (CRAC) and K + channels by curcumin in Jurkat-T cells. J Pharmacol Sci 115:144–154.
- Starkus J, Beck A, Fleig A, Penner R. 2007. Regulation of TRPM2 by extra- and intracellular calcium. J Gen Physiol 130:427–440.
- Su CC, Wang MJ, Chiu TL. 2010. The anti-cancer efficacy of curcumin scrutinized through core signaling pathways in glioblastoma. Int J Mol Med 26:217–224.
- Uguz AC, Cig B, Espino J, Bejarano I, Naziroglu M, Rodriguez AB, Pariente JA. 2012. Melatonin potentiates chemotherapy-induced cytotoxicity and apoptosis in rat pancreatic tumor cells. J Pineal Res 53:91–98.
- Uguz AC, Naziroglu M. 2012. Effects of selenium on calcium signaling and apoptosis in rat dorsal root ganglion neurons induced by oxidative stress. Neurochem Res 37:1631–1638.
- Uguz AC, Oz A, Naziroglu M. 2016. Curcumin inhibits apoptosis by regulating intracellular calcium release, reactive oxygen species and mitochondrial depolarization levels in SH-SY5Y neuronal cells. J Recept Signal Transduct Res 36:395–401.
- Uğuz AC, Naziroğlu M, Espino J, Bejarano I, González D, Rodríguez AB, Pariente JA. 2009. Selenium modulates oxidative stress-induced cell apoptosis in human myeloid HL-60 cells through regulation of calcium release and caspase-3 and-9 activities. J Memb Biol 232:15–23.
- Wang J, Du XX, Jiang H, Xie JX. 2009. Curcumin attenuates 6-hydroxydopamine-induced cytotoxicity by anti-oxidation and nuclear factor-kappa B modulation in MES23.5 cells. Biochem Pharmacol 78:178–183.
- Wang Q, Sun AY, Simonyi A, Jensen MD, Shelat PB, Rottinghaus GE, et al. 2005. Neuroprotective mechanisms of curcumin against cerebral ischemia-induced neuronal apoptosis and behavioral deficits. J Neurosci Res 82:138–148.
- Wehage E, Eisfeld J, Heiner I, Jungling E, Zitt C, Luckhoff A. 2002. Activation of the cation channel long transient receptor potential channel 2 (LTRPC2) by hydrogen peroxide. A splice variant reveals a mode of activation independent of ADP-ribose. J Biol Chem 277:23150–23156.
- Xu X, Chen D, Ye B, Zhong F, Chen G. 2015. Curcumin induces the apoptosis of non-small cell lung cancer cells through a calcium signaling pathway. Int J Mol Med 35:1610–1616.
- Yang F, Lim GP, Begum AN, Ubeda OJ, Simmons MR, Ambegaokar SS, et al. 2005. Curcumin inhibits formation of amyloid beta oligomers and fibrils, binds plaques, and reduces amyloid in vivo. J Biol Chem 280:5892–5901.
- Zhang F, Lau SS, Monks TJ. 2012. A dual role for poly(ADP-ribose) polymerase-1 during caspase-dependent apoptosis. Toxicol Sci 128:103–114.
- Zorn KK, Annunziata CM. 2016. Editorial: inhibiting PARP as a strategic target in cancer. Front Oncol 6:91.