Abstract
Notch receptor signalling plays a central role in development and its misfunction has been linked to a number of diseases. In the cannonical Notch signalling pathway, ligand binding to Notch activates a series of proteolytic cleavages that release the Notch intracellular domain for trafficking to the nucleus, where it activates the transcription factor, Suppressor of Hairless (Su(H)). A number of recent papers have demonstrated the importance of endocytic trafficking of Notch and its ligands for both the activation and the down-regulation of the Notch receptor. These reports highlight uncertainty regarding the whereabouts in the cell where Notch activation occurs, and the form of the ligand that can induce signalling. In this review we speculate that, decision points between alternative trafficking pathways represent important regulatory nodes that may allow Notch signalling levels to be modulated by other developmental signals, providing context-dependency to Notch activation. We also review data that suggest that key proteolytic events, associated with Notch activation, may occur within the endocytic pathway or require prior endocytosis and recycling of Notch and its ligands to the cell surface. Sorting within the endocytic pathway, regulated by several different ubiquitin ligase proteins, may be involved in ensuring whether ligand and receptor are competent to signal. Furthermore, the utilisation of an alternative mechanism of Notch signalling, independent of Su(H), may depend on driving endocytic Notch into a specific compartment, in response to the activity of the ring finger domain protein, Deltex.
Introduction
The Notch receptor regulates many aspects of cell differentiation and developmental patterning in multicellular organisms (reviewed by Lai [Citation2004]). In addition to these developmental roles, Notch also regulates certain stem cell populations in the adult organism (Conboy et al. [Citation2003]; Ohishi et al. [Citation2003]; Alvarez-Buylla & Lim [Citation2004]; Dontu et al. [Citation2004]) and, at least in Drosophila, is involved with long-term memory formation associated with neuronal plasticity (Presente et al. [Citation2004]). Furthermore, loss of Notch signalling capacity leads to an aging-related reduction in the ability to repair muscle damage (Conboy et al. [Citation2003]). Misregulation of Notch activity has also been linked to various forms of cancer. This may be through loss of function mutations in tissues where Notch has been shown to act as a tumour suppressor, or through misactivation of Notch in tissues where increased cell signalling holds cells in an undifferentiated state (reviewed by Weng & Aster [Citation2004]). Gain of function mutations in Notch have been particularly linked to T-cell acute lymphoblastic leukaemia (T-ALL) with as many as 50% of T-ALL patients showing mutations in Notch (Weng et al. [Citation2004]). Elevated Notch signalling has also been shown to be an important downstream response mediating the oncogenic effects of the constitutively active RasV12 mutation that is commonly found in human cancers (Weijzen et al. [Citation2002]). Other known pathological consequences of Notch mutations are the neurological disorder, CADASIL and a number of developmental abnormalities (reviewed by Gridley [Citation2003]). This central role of Notch in development and disease illustrates the importance of understanding the mechanisms by which Notch activation and down-regulation occur.
The signalling pathway of Notch () is activated by its binding to the membrane-bound ligands, Serrate or Delta. This initiates a cleavage of Notch that removes the bulk of its extracellular domain (NECD). The remaining membrane-tethered moiety then becomes a substrate for the Presenilin-containing γ-secretase complex, which cleaves within the Notch transmembrane domain to release the soluble Notch intracellular domain (NICD). The latter translocates to the nucleus to activate a transcription factor, Suppressor of Hairless (Su(H)), or the homologous CBF-1 in mammals (reviewed by Baron et al. [Citation2002]). However, the apparent simplicity of this pathway is misleading. In the nucleus, numerous factors co-assemble with NICD and Su(H) in a multiprotein complex. Entry of NICD into this complex causes an exchange of proteins that facilitates a switch in the activity of Su(H) from a negative regulator of gene transcription to a positive one. This involves the replacement of Histone deacetylases with Histone acetyl transferases, the latter modifying adjacent chromatin to facilitate gene transcription (reviewed by Lai [Citation2002]). The transcriptional activator protein, Mastermind, is also recruited into the NICD/Su(H) complex (Nam et al. [Citation2003]). Upstream of these nuclear events, numerous regulatory factors impinge on the pathway to regulate its activity, including glycosyl transferases and various ubiquitin ligases and other proteins that bind to the intracellular or extracellular domain of Notch (reviewed by Baron et al. [Citation2002]). In addition, an alternative mechanism of Notch signalling appears to be independent of Su(H) and also of the Notch ligands, Delta and Serrate, while being dependent on a cytoplasmically associated protein called Deltex (reviewed by Brennan & Gardener [Citation2002]; Martinez-Arias et al. [Citation2002]).
Figure 1. Overview of the Notch signalling pathway. (1) The functional Notch receptor is expressed on the cell surface as a processed heterodimer due to a Furin-dependent cleavage (S1 cleavage) in the extracellular domain (NECD). S1 cleavage may not occur in Drosophila Notch (Kidd & Lieber [Citation2002]). The Notch receptor on the signal-receiving cell binds to its ligand Delta or Serrate on the signal-donor cell. This triggers the shedding of NECD by an ADAM metalloprotease (S2 cleavage) followed by a cleavage within the transmembrane domain by a protease complex containing Presenilin (S3 cleavage). The domain structure and cleavage sites of Notch have been previously reviewed (Baron et al. [Citation2002]; Baron [Citation2003]) and are omitted in this simplified figure. (2) The released Notch intracellular domain (NICD) translocates to the nucleus. (3) In the nucleus, NICD binds to the DNA binding protein Su(H) and dissociates bound Histone deacetlyase (HDAC) from an Su(H) complex that normally represses gene transcription. The NICD further recruits Histone Acetyltransferase (HAT) as well as Mastermind (Mam) that serves to open up the chromatin structure and allow for gene transcription.
![Figure 1. Overview of the Notch signalling pathway. (1) The functional Notch receptor is expressed on the cell surface as a processed heterodimer due to a Furin-dependent cleavage (S1 cleavage) in the extracellular domain (NECD). S1 cleavage may not occur in Drosophila Notch (Kidd & Lieber [Citation2002]). The Notch receptor on the signal-receiving cell binds to its ligand Delta or Serrate on the signal-donor cell. This triggers the shedding of NECD by an ADAM metalloprotease (S2 cleavage) followed by a cleavage within the transmembrane domain by a protease complex containing Presenilin (S3 cleavage). The domain structure and cleavage sites of Notch have been previously reviewed (Baron et al. [Citation2002]; Baron [Citation2003]) and are omitted in this simplified figure. (2) The released Notch intracellular domain (NICD) translocates to the nucleus. (3) In the nucleus, NICD binds to the DNA binding protein Su(H) and dissociates bound Histone deacetlyase (HDAC) from an Su(H) complex that normally represses gene transcription. The NICD further recruits Histone Acetyltransferase (HAT) as well as Mastermind (Mam) that serves to open up the chromatin structure and allow for gene transcription.](/cms/asset/a0895fb2-0c6f-454e-b64f-fab7ee4ee6e4/imbc_a_112960_uf0001_b.jpg)
The endocytic pathway has long been suspected of being involved in Notch signalling. Shibire, the Drosophila Dynamin homologue, is involved in the pinching-off of Clathrin-coated vesicles and is required both in the signal-donor cell and the signal-receiving cell for Notch activity (Seugnet et al. [Citation1997]). This review will focus on recent developments which highlight the central role of the endocytic pathway in regulating both Notch activation and its down-regulation.
Decision points in the endocytic trafficking pathway
The spatial distributions and levels of membrane proteins at different cellular locations are regulated by trafficking routes between the different internal compartments and the cell surface (reviewed by Maxfield & McGraw [Citation2004]). Different steps in the trafficking pathways provide decision points enabling the flow of protein to be controlled (). The first decision point in the endocytic pathway is whether or not to remove the receptor from the plasma membrane. The best understood mechanism for this is mediated via Clathrin-coated vesicles, although the importance of other endocytic routes is becoming increasingly apparent (Conner & Schmid [Citation2003]). Many transmembrane proteins contain sorting signals within their cytoplasmic domains that allow them to bind directly to the AP-2 adaptor complex, which recruits Clathrin to the plasma membrane. However, not all Clathrin-internalised proteins rely on a direct binding to AP-2. They may use different adaptor proteins to link them indirectly to AP-2, or utilise another adaptor that can bind Clathrin (Bonifacino & Traub [Citation2003]; Motley et al. [Citation2003]). This may allow their endocytosis to be more carefully regulated by cellular factors. An important endocytic label is ubiquitin, which can be covalently attached to certain proteins via the activity of ubiquitin ligase proteins. Monoubiquitination may serve as an endocytic tag by enabling binding to ubiquitin-interacting proteins such as EPS15 and Epsin that can also associate with AP-2 (Bonifacino & Traub [Citation2003]).
Figure 2. An overview of the endocytic pathway illustrating sites of sorting between trafficking routes. Transmembrane proteins can be removed from the plasma membrane (PM) by endocytosis which may be Clathrin-mediated (1). The Clathrin-coated vesicles (CV) lose their Clathrin coat after endocytosis and uncoated vesicles subsequently fuse to the early endosome (EE) a major sorting organelle of the cell that is subcompartmentalised (2). Initially proteins will enter into a region of the early endosome that is rich in the monomeric GTPase protein Rab5. From here, proteins can be sorted on a down-regulatory pathway (3) to a region of the early endosome, rich in Hrs, where vesicles internalise from the limiting membrane. (4) From this region, multivesicular bodies/late endosomes (MVB/LE) are formed. These may mature and then fuse to the lysosome (LYSO) which contains a mixture of different proteases. The contents of the hybrid organelle may then be digested (5). There are recovery routes from this down-regulatory pathway. For example, there is a flow (6) from the MVB/LE back to the trans-Golgi network (TGN). Proteins can also be sorted within the early endosome towards a recycling route (7). This may occur directly back to the plasma membrane (8) or by a slower route to the cell surface via the recycling endosomes (RE) (9, 10). There is also bi-directional transport between the recycling endosome and the trans-Golgi network (11) from where proteins can also be sent to the cell surface (12), as well as to other endocytic compartments including the late endosome (13).
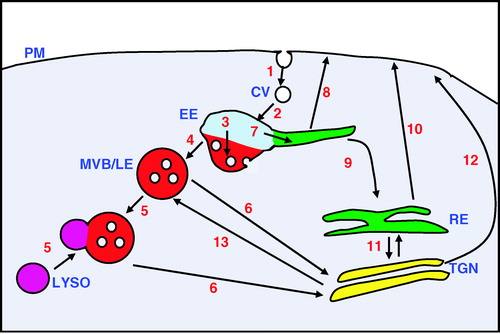
Transmembrane proteins endocytosed via Clathrin will be targeted to the early endosomes, a major sorting organelle, and one that has been found critical in determining the outcomes of several key signalling pathways. It is here in the early endosome, where a choice is made between recycling of proteins back to the cell surface, and their trafficking for degradation by the lysosome. The early endosome is a non-uniform organelle. It comprises both globular and tubular structures and its subcompartmentalisation can be visualised by the non-overlapping distribution of different, small, monomeric Rab GTPases on its membrane surface (Sonnichsen et al. [Citation2000]). Clathrin-endocytosed transmembrane proteins initially enter into a Rab5 positive compartment and are subsequently either directed towards the down-regulatory route, or recycled back to the plasma membrane. The selection between these two pathways may depend on covalent modifications, or protein-binding partners. Ubiquitinated proteins are often sorted to an Hrs-rich domain of the early endosome (Raiborg et al. [Citation2002]). Hrs recruits ubiquitinated proteins through a ubiquitin binding motif to a compartment of the early endosome where the membrane begins to invaginate to form internal vesicles. Ubiquitinated proteins are sorted into these internal vesicles. The structure containing the internal vesicles probably detaches from the early endosome and is often referred to as a multivesicular body (MVB). These MVBs may further mature into late endosomes (Gruenberg & Stenmark [Citation2004]). Late endosomes can then fuse with lysosomes, which contain many hydrolytic enzymes. The resultant hybrid organelle, then digests the endocytosed material (Luzio et al. [Citation2000]).
The alternative to the degradative route is the recycling pathway involving Rab4 and Rab11 GTPases and this is thought to occur either directly from distinct subcompartments of the early endosome, or via specialised recycling endosomes (Sonnichsen et al. [Citation2000]). There is also a possibility of retrieving the protein from the late endosome by retrograde transport between this compartment and the trans-Golgi network (Barbero et al. [Citation2002]). Movement of membrane proteins through alternative endocytic pathways can determine whether or not the protein is degraded, or in some cases, whether it is modified in such a way that it is now in an active or inactive form. For example, following agonist stimulation, many G protein-coupled receptors are desensitised by first being phosphorylated and then endocytosed. In the case of the B2-Adrenergic receptor, it can be resensitised and recycled to the plasma membrane. The agonist may be removed in the more acidic environment of the early endosome, and the receptor de-phosphorlyated, probably in transit between the Rab5 and Rab4 positive compartments, before being recycled to the cell surface (Seachrist et al. [Citation2000]). Alternatively, endocytic trafficking can bring signalling components together. For example, endocytosis of the TGFβ receptor enables the latter to interact with SARA, a protein required for its signal activation, which is highly enriched within the Rab5 positive endosomal compartment (Di Guglielmo et al. [Citation2003]). The involvement of the above-described endosomal sorting points is becoming apparent now for the regulation of Notch receptor signalling.
Ligand endocytosis drives Notch activation
Evidence that ligand endocytosis in the signal-donor cell is required for Notch activation in the adjacent cell, came from the identification of endocytosis defective mutations of DrosophilaDelta which were incapable of activating Notch signalling (Parks et al. [Citation2000]). Subsequent work in Drosophila and vertebrate models showed that two ring finger E3 ubiquitin ligases, Neuralized and Mindbomb, are required in some developmental contexts to ubiquitinate Delta and promote both the latter's endocytosis and ability to activate Notch in adjacent cells (Pavlopolous et al. [Citation2001]; Le Borgne & Schweisguth [Citation2003]; Itoh et al. [Citation2003]; Li & Baker [Citation2004]). The requirement for Delta endocytosis in the signal-donor cell has further been supported by recent papers that show that Epsin, which in Drosophila, is encoded by the liquid facets (lqf) gene, is required in the signal-donor cell for Notch activation and for a proportion, although not all, of Delta endocytosis (Overstreet et al. [Citation2004]; Tian et al. [Citation2004]; Wang & Struhl [Citation2004]). Interestingly, the activities of both Lqf and Neuralized proteins can themselves be regulated. Lqf is down-regulated by ubiquitination and the latter is relieved by the activity of a deubiquitinating protein called Fat facets. Neuralized, on the other hand, has been shown to be regulated during Drosophila sensory organ development by cytoskeletal-dependent interactions that lead to its asymmetric distribution between the two daughter cells of the sensory organ precursor (Le Borgne & Schweisguth [Citation2003]). The higher level of Neuralized in one daughter cell biases that cell to become dominant as a Notch signal-donor. This contributes in part to an asymmetric adoption of cell fates because of the neural fate-repressing activity of Notch in the sister cell. Thus, stimulation of endocytic trafficking provides the means to regulate the ability of a cell to send a signal to its neighbours, allowing for a fine control which can be superimposed on the simple transcriptional regulation of ligand expression.
How can Delta endocytosis in the signal-donor cell be linked to Notch activation in an adjacent cell? One possibility is that engagement of the endocytic machinery with the Delta cytoplasmic domain places mechanical strain on the Notch protein bound to the extracellular domain of Delta in a trans-complex, exposing a cleavage site targeted by metalloprotease activity (A). Evidence for this model comes from a study in the Drosophila eye, which showed trafficking of NECD but not NICD from the signal-receiving cell into the signal-donor cell, co-localised with Delta, a process termed transendocytosis (Parks et al. [Citation2000]). Preventing endocytosis using a mutation of Dynamin/Shibire caused an accumulation of both NECD and NICD epitopes, co-localised with Delta at the cell surface. It was also found that trafficking-defective mutations of Delta were unable to support Notch transendocytosis, and this was correlated with an inability of the mutant ligand to activate Notch. These data have been further supported by an analysis of Delta and Notch trafficking during Drosophila embryonic mesoectoderm formation (Morel et al. [Citation2003]). Together, these observations suggested that in vivo, the cleavage and activation of Notch cannot occur without endocytosis of Delta. However, a requirement for ligand endocytosis to act directly as part of the biochemical mechanism that cleaves Notch, is not proven and it is possible that internalisation of NECD simply reflects the clearance of shed ectodomains following the S2 cleavage step. It is also notable that the dissociation of NECD and NICD epitopes that was observed in vivo does not mimic the situation in vitro in S2 cell culture, when transendocytosis of Notch into Delta-expressing cells appears to reflect transport of the entire Notch protein from one cell to another (Klueg & Muskavitch [Citation1999]). Similarly, in another study of sensory organ development both extra and intracellular epitopes of Notch were found to be co-localised with Delta in both the signal-donor cell and the signal-receiving cell, suggesting that trafficking of full-length receptor was being observed in these cases (Le Borgne & Schweisguth [Citation2003]).
Figure 3. Models to explain the endocytic requirement of Delta in the signal-donor cell for signal activation. (A) A model in which the Notch extracellular domain (NECD) is transendocytosed into the signal-donor cell. Here Delta (Dl), which is bound to Notch on the opposing cell, first engages the endocytic machinery (1). This exerts a mechanical force on the Notch receptor causing a metalloprotease site in NECD to be exposed and S2 cleavage to occur (2). Full-length Delta, bound to the cleaved fragment of NECD is subsequently endocytosed into the signal-donor cell (Parks et al. [Citation2000]) (3). The membrane-tethered Notch intracellular domain (NICD) on the signal-receiving cell is now a target for the Presenilin S3 cleavage (4). This results in the release of soluble NICD, which translocates to the nucleus to modulate gene transcription (5). (B) A model in which endocytic trafficking of the Delta ligand is required to generate an active ligand at the cell surface of the signal donor cell. Clathrin mediated endocytosis of Delta that had been ubiquitinated by Neuralized is targeted to the early endosome (EE) (1). From here, it is sorted for recycling (2), either directly back to the plasma membrane (PM) (3), or via the recycling endosome (RE) (4–5). During this endocytic recycling route, Delta may be modified in some manner in order to become an active receptor, possibly by proteolytic cleavage (Wang & Struhl [Citation2004]). This Figure is reproduced in colour in Molecular Membrane Biology online.
![Figure 3. Models to explain the endocytic requirement of Delta in the signal-donor cell for signal activation. (A) A model in which the Notch extracellular domain (NECD) is transendocytosed into the signal-donor cell. Here Delta (Dl), which is bound to Notch on the opposing cell, first engages the endocytic machinery (1). This exerts a mechanical force on the Notch receptor causing a metalloprotease site in NECD to be exposed and S2 cleavage to occur (2). Full-length Delta, bound to the cleaved fragment of NECD is subsequently endocytosed into the signal-donor cell (Parks et al. [Citation2000]) (3). The membrane-tethered Notch intracellular domain (NICD) on the signal-receiving cell is now a target for the Presenilin S3 cleavage (4). This results in the release of soluble NICD, which translocates to the nucleus to modulate gene transcription (5). (B) A model in which endocytic trafficking of the Delta ligand is required to generate an active ligand at the cell surface of the signal donor cell. Clathrin mediated endocytosis of Delta that had been ubiquitinated by Neuralized is targeted to the early endosome (EE) (1). From here, it is sorted for recycling (2), either directly back to the plasma membrane (PM) (3), or via the recycling endosome (RE) (4–5). During this endocytic recycling route, Delta may be modified in some manner in order to become an active receptor, possibly by proteolytic cleavage (Wang & Struhl [Citation2004]). This Figure is reproduced in colour in Molecular Membrane Biology online.](/cms/asset/17bc2d8e-0d1e-4f40-b607-16fea1372b17/imbc_a_112960_uf0003_b.jpg)
An attractive, alternative explanation for the endocytic requirement for Delta is that the latter must first be trafficked through a compartment in which it becomes, in some way, competent to generate a signal (Wang & Struhl [Citation2004]). Epsins contain ubiquitin-interacting motifs and are thought to act as selective adaptors that recruit monoubiquitinated membrane proteins into endocytic complexes. It has been proposed that the Drosophila Epsin/Lqf recruits Delta into a recycling endosomal pathway, somehow allowing its conversion from an inactive ligand into an active ligand, which is then redisplayed at the cell surface (Wang & Struhl [Citation2004]). Thus, the choice between alternative trafficking pathways may determine the proportion of Delta proteins that are able to signal (B). This model would also go some way to resolve the paradox that, in vitro, the requirement for ligand endocytosis can be bypassed by artificial manipulation of the way the ligand is presented to Notch i.e., if the ligand is immobilised on a surface or forced into an oligomeric state (Varnum-Finney et al. [Citation2000]; Hicks et al. [Citation2002]). In support of the endocytic/recycling model, the in vivo requirement for Lqf was partly overcome by introducing into the cytoplasmic domain of Delta, a peptide sequence that normally allows the internalisation and recycling of the LDL receptor. The nature of the proposed modification of Delta that transforms it into a signalling competent ligand is unknown. It is possible that recycling fosters ligand clustering, mimicking the experimentally induced oligomeric ligands that have been found to be effective in vitro. However, it is intriguing that the requirement for Lqf for signalling was correlated with its requirement for a proteolytic cleavage of Delta (Wang & Struhl [Citation2004]). The latter was predicted to release a soluble N-terminal fragment that contains the Notch interacting domain. It is interesting therefore to speculate as to whether it is this cleavage that generates an active form of the ligand. As previously reviewed (Baron et al. [Citation2002]), there is conflicting evidence regarding the ability of soluble fragments of Notch ligands to activate the receptor, as both agonistic and antagonistic properties have been attributed to such fragments. The precise nature of the soluble ligand fragment to which Notch is exposed may therefore be crucial in determining whether it activates the receptor or not. Alternatively, the observed proteolytic cleavage of Delta may be linked to other regulatory processes. For example, both the metalloproteases, Kuzbanian and Kuzbanian-like, generate soluble proteolytic fragments of Delta, but this has been attributed to a mechanism to remove Delta and prevent its cis-inhibition of Notch in the signal-receiving cell (Mishra-Gorur et al. [Citation2002]; Sapir et al. [Citation2005]).
Down-regulation of Notch by endocytosis
In epithelial cells, Notch is localised both at the adherens junction and in endocytic vesicles. There appears to be a steady state flux of continual delivery and endocytic removal of Notch to and from the cell surface (Wilkin et al. [Citation2004]). A number of studies have implicated endocytic pathways in down-regulating Notch. A Drosophila mutation in phosphocholine cytidylyltransferase 1 (CCT1) affects membrane lipid composition, and thereby results in increased Notch endocytosis which is correlated with decreased signalling (Weber et al. [Citation2003]). In C. elegans, it was found that Ras pathway activation down-regulated Lin-12, a C. elegans homologue of Notch, by stimulating Lin-12 endocytosis (Shaye & Greenwald [Citation2002]). The latter example illustrates the potential of cross-talk with other signals to modulate Notch receptor and/or ligand activity by regulation of their trafficking. Another example of Notch down-regulation, which is probably linked to endocytosis, is that mediated by the Notch-binding protein, Numb. The latter down-regulates Notch in a subset of Notch-dependent developmental contexts, for example, the adoption of asymmetric cell fates during sensory organ differentiation. Numb is asymmetrically distributed between the daughter cells arising from the division of the sensory organ precursor, and down-regulates Notch cell-autonomously (Guo et al. [Citation1996]). It has been proposed that the latter activity depends on the interaction of Numb with the endocytic machinery of the cell. Numb binds both to the intracellular domain of Notch and to the endocytic protein, alpha-adaptin, thus potentially linking Notch to the AP-2 complex and hence its recruitment into Clathrin-coated vesicles (Berdnik et al. [Citation2002]). In the latter study however, no actual difference in Notch protein levels was identified between the two daughter cells of the asymmetric cell division, hinting that the mechanism may be more complicated. Other studies have suggested different mechanisms for Numb. For example, a recent study has suggested a role for Numb in the recycling of membrane receptors to the cell surface (Smith et al. [Citation2004]). In mammalian cells, Numb also recruits a Nedd4 family ubiquitin ligase called Itch, which ubiquitinates Notch and promotes its degradation (McGill & McGlade [Citation2003]). In Drosophila, two Nedd4 family proteins have also been shown to down-regulate Notch, but in developmental contexts that do not normally involve Numb activity. DNedd4 promotes Notch ubiquitination and dominant negative forms of DNedd4 block endocytic trafficking of Notch (Sakata et al. [Citation2004]). It was suggested, that in cell culture, DNedd4 prevents Notch signalling, independent of its ligands, but in vivo, its over-expression can also down-regulate endogenous ligand-dependent Notch signalling, for example, at the dorsal-ventral boundary of the wing imaginal disc (Sakata et al. [Citation2004]; Wilkin et al. [Citation2004]). The related Suppressor of deltex protein (Su(dx)) also down-regulates Notch signalling and, in vivo, promotes Notch accumulation in an Hrs and ubiquitin-enriched subcompartment of the early endosome (Wilkin et al. [Citation2004]), from where it can be trafficked to the MVB/late endosome. There may therefore be a degree of functional redundancy between these Nedd4 family proteins in Drosophila, although their mechanism of action is not necessarily identical.
Where in the cell is Notch activated?
Two proteolytic steps are involved in ligand-dependent activation of Notch. The so-called S2 cleavage occurs in response to ligand activation and leads to the shedding of the bulk of the extracellular domain. It is mediated by an ADAM family metalloprotease enzyme, possibly TACE (ADAM17) and/or Kuzbanian (ADAM10) (Brou et al. [Citation2000]; Klein [Citation2002]; Lieber et al. [Citation2002]). The membrane-tethered NICD is then a substrate for γ-secretase-dependent cleavage (the S3 cleavage) requiring the aspartate protease Presenilin (reviewed by Baron [Citation2003]). The simplest model of Notch activation would be that both proteolytic events occur at the cell surface, as depicted in . However, it has been shown earlier that Dynamin-dependent endocytosis is required for Notch signalling in the signal-receiving cell as well as in the signal-donor cell (Seugnet et al. [Citation1997]). Recent data also supports a requirement for Notch endocytosis in signal activation. It has been shown for human Notch1, that the Presenilin-dependent cleavage of membrane-tethered NICD, requires the latter's monoubiquitination and endocytosis, dependent on an unknown ubiquitin ligase (Gupta-Rossi et al. [Citation2004]). Work in our group also supports a model in which endocytosis of Notch is an intermediate step in its activation. In vivo, co-expression of Notch with either wild type or ubiquitin ligase-deficient forms of Su(dx) promotes Notch accumulation in early endosomes, but the former is associated with a down-regulation of Notch, while the latter promotes Notch signalling (Wilkin et al. [Citation2004]). In each case, however, Notch accumulated in a different endosomal sub-compartment. Wild type Su(dx) activity promoted Notch accumulation in an Hrs and ubiquitin positive early endosomal domain, while the dominant negative Su(dx) promoted accumulation of Notch in an adjacent early endosomal domain, partly overlapping with Rab11, a recycling endosomal marker. Importantly, it was not possible to explain away the increased Notch activity, induced by dominant negative Su(dx), by an increased availability of Notch at the cell surface. The implication therefore is that Notch may have to be diverted to a particular endosomal compartment to allow its activation to occur. Furthermore, both extra and intracellular epitopes of Notch co-localised in the enlarged endosomal compartment implying that the endocytosis of full-length Notch occurs prior to its ectodomain cleavage. One possibility is that S2 cleavage may actually occur in an endosomal compartment following a ligand-stimulated endocytic step (A). A recent study using mammalian cell culture has indicated that TACE, which has been implicated in Notch activation, is present in endosomes and may be able to target substrates at this location (Schantl et al. [Citation2004]). This model is however difficult to reconcile with the observation of transendocytosis of NECD along with ligand into the signal-donor cell (Parks et al. [Citation2000]). It is possible that the latter process may be a byproduct following Notch activation, when shed NECD could be recycled and secreted into the extracellular space. Alternatively, it is possible that, like Delta, Notch also needs to be first endocytosed and then recycled via a specific endosomal compartment back to the cell surface, in order to be activated, thus releasing NECD. The choice between entering a recycling compartment or transfer to the late endosome may therefore be a rate limiting step and potentially, a site of cross-talk with other signalling pathways.
Figure 4. Models to explain the endocytic requirement of Notch in the signal-receiving cell for signal activation. A) Following ligand activation, full-length Notch at the plasma membrane (PM) may be endocytosed (1) and targeted to the early endosome (EE) where it may be sorted on a down-regulatory lysosomal degradative pathway, limiting signal activation (2) (Wilkin et al. [Citation2004]). Alternatively, it may be directed to the recycling region of the early endosome (3). Notch may then be recycled back to the cell surface in a modified form (*) competent for S2 cleavage and activation (4). Alternatively, full-length Notch may encounter and be a target of the TACE metalloprotease and undergo S2 cleavage within the endosomal compartment (5). The membrane-tethered NICD may then be recycled back to the plasma membrane prior to S3 cleavage (see B). Alternatively, it may encounter Presenilin and undergo S3 cleavage within the endocytic compartment (6). The released soluble Notch intracellular domain then translocates to the nucleus and activates transcription. B) Membrane-tethered Notch intracellular domain may be located at the plasma membrane, either following S2 cleavage at the plasma membrane, or, as described above, following S2 cleavage in an endosomal compartment and recycling to the cell surface. S3 cleavage by Presenilin may then occur at the cell surface (1). However, a recent report (Gupta-Rossi et al. [Citation2004]) indicates that membrane tethered NICD requires endocytosis for activation (2). Endocytosed, membrane-tethered NICD may then be sorted to and activated in the recycling endosome (See A). Alternatively, it may be sorted to the late endosome (LE) (3) and, following vesicle fusion (4), may be delivered to the lysosome (LYSO). In the latter case, depending on its ubiquitination status, it may either be sorted to the internal lumens of the LE and subsequently be degraded by lysosomal proteases (5), or, remain on the limiting membrane of the LEs and be delivered to the outer limiting membrane of the lysosome (6). It may then be cleaved by the Presenilin present in the limiting membrane of the lysosome (Pasternak et al. [Citation2003]) to release soluble NICD for transport to the nucleus.
![Figure 4. Models to explain the endocytic requirement of Notch in the signal-receiving cell for signal activation. A) Following ligand activation, full-length Notch at the plasma membrane (PM) may be endocytosed (1) and targeted to the early endosome (EE) where it may be sorted on a down-regulatory lysosomal degradative pathway, limiting signal activation (2) (Wilkin et al. [Citation2004]). Alternatively, it may be directed to the recycling region of the early endosome (3). Notch may then be recycled back to the cell surface in a modified form (*) competent for S2 cleavage and activation (4). Alternatively, full-length Notch may encounter and be a target of the TACE metalloprotease and undergo S2 cleavage within the endosomal compartment (5). The membrane-tethered NICD may then be recycled back to the plasma membrane prior to S3 cleavage (see Figure 4B). Alternatively, it may encounter Presenilin and undergo S3 cleavage within the endocytic compartment (6). The released soluble Notch intracellular domain then translocates to the nucleus and activates transcription. B) Membrane-tethered Notch intracellular domain may be located at the plasma membrane, either following S2 cleavage at the plasma membrane, or, as described above, following S2 cleavage in an endosomal compartment and recycling to the cell surface. S3 cleavage by Presenilin may then occur at the cell surface (1). However, a recent report (Gupta-Rossi et al. [Citation2004]) indicates that membrane tethered NICD requires endocytosis for activation (2). Endocytosed, membrane-tethered NICD may then be sorted to and activated in the recycling endosome (See Figure 4A). Alternatively, it may be sorted to the late endosome (LE) (3) and, following vesicle fusion (4), may be delivered to the lysosome (LYSO). In the latter case, depending on its ubiquitination status, it may either be sorted to the internal lumens of the LE and subsequently be degraded by lysosomal proteases (5), or, remain on the limiting membrane of the LEs and be delivered to the outer limiting membrane of the lysosome (6). It may then be cleaved by the Presenilin present in the limiting membrane of the lysosome (Pasternak et al. [Citation2003]) to release soluble NICD for transport to the nucleus.](/cms/asset/c468bc51-0afe-4ff4-acd8-59d71e7214f1/imbc_a_112960_uf0004_b.jpg)
Similarly, there is controversy surrounding the actual location in the cell where Presenilin-dependent S3 cleavage occurs. Evidence from cell culture studies, using cell surface biotinylation of chilled cells (the latter suppresses endocytosis), show that Presenilin can associate with Notch at the cell surface (Ray et al. [Citation1999]). However, as discussed above, it has recently been reported that activation of membrane-tethered NICD by Presenilin requires an endocytic step (Gupta-Rossi et al. [Citation2004]). This may mean that membrane-tethered NICD also needs to be recycled back to the cell surface before it can be activated by Presenilin cleavage (A). However, other work has shown that Presenilin is located and is active in the endosomal/lysosomal pathway and requires the acidic environment of this compartment for optimal activity (Pasternak et al. [Citation2003]). The latter study reported Presenilin to reside in the limiting membrane of the lysosome. Interestingly a second consequence of the over-expression of the dominant negative form of Su(dx) is the retention of Notch in the limiting membrane of late endosomes (Wilkin et al. [Citation2004]). This may be significant, because following late endosome/lysosome fusion, proteins in this location will be delivered to the lysosomal-limiting membrane rather than being degraded in the lysosomal lumen (B). If active Presenilin does reside in the lysosomal-limiting membrane, then this may contribute to the stimulation of Notch signalling that is induced by the mutant Su(dx) protein.
An alternative Notch signalling pathway is linked to Notch endocytosis
Studies in Drosophila and mammals have suggested that not all of the activities of Notch can be accounted for by the Su(H)-dependent signalling pathway (Reviewed by Brennan & Gardener [Citation2002]; Martinez-Arias et al. [Citation2002]). It is claimed that a subset of Notch activities is independent of a number of components of the canonical signalling pathway, including the ligands Delta and Serrate, the transcription factor, Su(H), and the downstream Notch target genes within the Enhancer of split gene complex (Ramain et al. [Citation2001]). At least, in Drosophila, however, the existence of a Su(H)-independent form of Notch signalling has been controversial. A number of examples of apparent Su(H)-independence have turned out to be so, because of the fact that earlier studies used Su(H) mutant alleles which retained some activity (Morel and Schweisguth. [Citation2000]; Li & Baker [Citation2001]). The fact that Su(H) can act as a repressor as well as an activator of transcription has also complicated the analysis of its role. However, a recent study, using a null mutation of Su(H), is not easily explained without invoking an Su(H)-independent signal (Hori et al. [Citation2004]), although, until a mechanism for this proposed signalling pathway is firmly established, this area is likely to remain controversial.
One component of the proposed alternative mode of signalling has been identified as Deltex (Ramain et al. [Citation2001]), a ring zinc finger E3 ubiquitin ligase protein, which is known to bind to the intracellular domain of Notch (Matsuno et al. [Citation1995]). In mammalian cells, the membrane-bound Contactin and the Contactin-like protein, NB-3, have been found to be ligands for a Deltex-dependent Notch signal (Hu et al. [Citation2003]; Cui et al. [Citation2004]). There is little consensus, however, on the role of Deltex in this pathway. Studies in mammalian cells, utilising cell fractionation experiments, have suggested that Deltex acts in the nucleus, possibly interacting in a complex with NICD and the co-activator p300 (Yamamoto et al. [Citation2001]), and may antagonise the canonical Notch pathway by preventing the recruitment by NICD of other transcriptional co-activators (Izon et al. [Citation2002]). However, recent data from in vivo studies in Drosophila show that Deltex acts to regulate Notch trafficking with a proportion of Notch endocytosis requiring Deltex function (Hori et al. [Citation2004]). Increasing the expression of Deltex activates a Su(H)-independent form of Notch signalling correlated with a depletion of Notch from the cell surface and promotion of its endocytosis. Notch was required for Deltex to activate the signal and the expression of a mutant form of Rab5, which interferes with early endosomal trafficking, suppressed this signal induction. It seems therefore that endocytic trafficking of Notch through particular endosomal compartments or subcompartments not only determines whether or not Notch signals, but also which mode of signalling is selected. The mechanism of signal initiation in this pathway and the subsequent events in the nucleus are not fully understood. However, recent work in mammalian cells indicates that Presenilin-dependent cleavage and nuclear localisation of Notch is involved (Hu et al. 2004). The role of Deltex in Notch trafficking is not necessarily mutually exclusive to the model that Deltex acts in the nucleus as it is possible that Deltex remains associated with the Notch intracellular domain after the latter translocates to the nucleus, possibly preventing the latter's association with Su(H).
Conclusions
In this review, we have proposed that trafficking of Notch and its ligands plays a central role in regulating Notch signal activation. Further exploration of this area may enable the many disparate strands of intracellular and extracellular Notch modulatory factors to be brought together within a more unified model of signal regulation. We suggest that nodes between trafficking routes may be points of regulation, determining signalling level and also selecting alternative mechanisms of Notch signalling. It is likely that other signalling pathways impinge on such decision points fostering regulatory cross-talk, and that disruption of normal ligand and receptor trafficking routes may be linked to Notch-associated pathology. In this regard, it is interesting that recent work indicates that the loss of Numb-dependent Notch down-regulation contributes to around half of all human breast cancers (Pece et al. [Citation2004]). The emerging importance of Notch in cancer, the immune system, stem cell regulation and aging-associated processes will make it increasingly important to develop ways of therapeutically manipulating Notch signalling levels. Understanding in detail the mechanisms of Notch regulation will aid the development of such agents. This paper was first published online on prEview on 29 June 2005.
References
- Alvarez-Buylla A, Lim DA. For the long run: maintaining germinal niches in the adult brain. Neuron 2004; 41: 683–686
- Barbero P, Bittova L, Pfeffer SR. Visualization of Rab9-mediated vesicle transport from endosomes to the trans-Golgi in living cells. J Cell Biol 2002; 156: 511–518
- Baron M. An overview of the Notch signalling pathway. Semin Cell Dev Biol 2003; 14: 113–119
- Baron M, Aslam H, Flasza M, Fostier M, Higgs JE, Mazaleyrat SL, Wilkin MB. Multiple levels of Notch signal regulation (review). Mol Membr Biol 2002; 19: 27–38
- Berdnik D, Torok T, Gonzalez-Gaitan M, Knoblich JA. The endocytic protein alpha-Adaptin is required for numb-mediated asymmetric cell division in Drosophila. Dev Cell 2002; 3: 221–231
- Bonifacino JS, Traub LM. Signals for sorting of transmembrane proteins to endosomes and lysosomes. Annu Rev Biochem 2003; 72: 395–447
- Brennan K, Gardner P. Notching up another pathway. Bioessays 2002; 24: 405–410
- Brou C, Logeat F, Gupta N, Bessia C, LeBail O, Doedens JR, Cumano A, Roux P, Black RA, Israel A. A novel proteolytic cleavage involved in Notch signaling: the role of the disintegrin-metalloprotease TACE. Mol Cell 2000; 5: 207–216
- Conboy IM, Conboy MJ, Smythe GM, Rando TA. Notch-mediated restoration of regenerative potential to aged muscle. Science 2003; 302: 1575–1577
- Conner SD, Schmid SL. Regulated portals of entry into the cell. Nature 2003; 422: 37–44
- Cui XY, Hu QD, Tekaya M, Shimoda Y, Ang BT, Nie DY, Sun L, Hu WP, Karsak M, Duka T, et al. NB-3/Notch1 pathway via Deltex1 promotes neural progenitor cell differentiation into oligodendrocytes. J Biol Chem 2004; 279: 25858–25865
- Di Guglielmo GM, Le Roy C, Goodfellow AF, Wrana JL. Distinct endocytic pathways regulate TGF-beta receptor signalling and turnover. Nat Cell Biol 2003; 5: 410–421
- Dontu G, Jackson KW, McNicholas E, Kawamura MJ, Abdallah WM, Wicha MS. Role of Notch signaling in cell-fate determination of human mammary stem/progenitor cells. Breast Cancer Res 2004; 6: R605–615
- Gridley T. Notch signaling and inherited disease syndromes. Hum Mol Genet 2003; 12: R9–R13
- Gruenberg J, Stenmark H. The biogenesis of multivesicular endosomes. Nat Rev Mol Cell Biol 2004; 5: 317–323
- Guo M, Jan LY, Jan YN. Control of daughter cell fates during asymmetric division: interaction of Numb and Notch. Neuron 1996; 17: 27–41
- Gupta-Rossi N, Six E, LeBail O, Logeat F, Chastagner P, Olry A, Israel A, Brou C. Monoubiquitination and endocytosis direct gamma-secretase cleavage of activated Notch receptor. J Cell Biol 2004; 166: 73–83
- Hicks C, Ladi E, Lindsell C, Hsieh JJ, Hayward SD, Collazo A, Weinmaster G. A secreted Delta1-Fc fusion protein functions both as an activator and inhibitor of Notch1 signaling. J Neurosci Res 2002; 68: 655–667
- Hori K, Fostier M, Ito M, Fuwa TJ, Go MJ, Okano H, Baron M, Matsuno K. Drosophila Deltex mediates Suppressor of Hairless-independent and late-endosomal activation of Notch signaling. Development 2004; 131: 5527–5537
- Hu QD, Ang BT, Karsak M, Hu WP, Cui XY, Duka T, Takeda Y, Chia W, Sankar N, Ng YK, et al. F3/contactin acts as a functional ligand for Notch during oligodendrocyte maturation. Cell 2003; 115: 163–175
- Itoh M, Kim CH, Palardy G, Oda T, Jiang YJ, Maust D, Yeo SY, Lorick K, Wright GJ, Ariza-McNaughton L, et al. Mind bomb is a ubiquitin ligase that is essential for efficient activation of Notch signaling by Delta. Dev Cell 2003; 4: 67–82
- Izon DJ, Aster JC, He Y, Weng A, Karnell FG, Patriub V, Xu L, Bakkour S, Rodriguez C, Allman D, Pear WS. Deltex1 redirects lymphoid progenitors to the B cell lineage by antagonizing Notch1. Immunity 2002; 16: 231–243
- Kidd S, Lieber T. Furin cleavage is not a requirement for Drosophila Notch function. Mech Dev 2002; 115: 41–51
- Klein T. Kuzbanian is required cell autonomously during Notch signalling in the Drosophila wing. Dev Genes Evol 2002; 212: 251–255
- Klueg KM, Muskavitch MA. Ligand-receptor interactions and trans-endocytosis of Delta, Serrate and Notch: members of the Notch signalling pathway in Drosophila. J Cell Sci 1999; 112: 3289–3297
- Lai EC. Keeping a good pathway down: transcriptional repression of Notch pathway target genes by CSL proteins. EMBO Rep 2002; 3: 840–845
- Lai EC. Notch signaling: control of cell communication and cell fate. Development 2004; 131: 965–973
- Le Borgne R, Schweisguth F. Unequal segregation of Neuralized biases Notch activation during asymmetric cell division. Dev Cell 2003; 5: 139–148
- Li Y, Baker NE. Proneural enhancement by Notch overcomes Suppressor-of-Hairless repressor function in the developing Drosophila eye. Curr Biol 2001; 11: 330–338
- Li Y, Baker NE. The roles of cis-inactivation by Notch ligands and of Neuralized during eye and bristle patterning in Drosophila. BMC Dev Biol 2004; 4: 5
- Lieber T, Kidd S, Young MW. Kuzbanian-mediated cleavage of Drosophila Notch. Genes Dev 2002; 16: 209–221
- Luzio JP, Rous BA, Bright NA, Pryor PR, Mullock BM, Piper RC. Lysosome-endosome fusion and lysosome biogenesis. J Cell Sci 2000; 113: 1515–1524
- Martinez Arias A, Zecchini V, Brennan K. CSL-independent Notch signalling: a checkpoint in cell fate decisions during development?. Curr Opin Genet Dev 2002; 12: 524–533
- Matsuno K, Diederich RJ, Go MJ, Blaumueller CM, Artavanis-Tsakonas S. Deltex acts as a positive regulator of Notch signaling through interactions with the Notch ankyrin repeats. Development 1995; 121: 2633–2644
- Maxfield FR, McGraw TE. Endocytic recycling. Nat Rev Mol Cell Biol 2004; 5: 121–132
- McGill MA, McGlade CJ. Mammalian numb proteins promote Notch1 receptor ubiquitination and degradation of the Notch1 intracellular domain. J Biol Chem 2003; 278: 23196–23203
- Mishra-Gorur K, Rand MD, Perez-Villamil B, Artavanis-Tsakonas S. Down-regulation of Delta by proteolytic processing. J Cell Biol 2002; 159: 313–324
- Morel V, Le Borgne R, Schweisguth F. Snail is required for Delta endocytosis and Notch-dependent activation of single-minded expression. Dev Genes Evol 2003; 213: 65–72
- Morel V, Schweisguth F. Repression by Suppressor of hairless and activation by Notch are required to define a single row of single-minded expressing cells in the Drosophila embryo. Genes Dev 2000; 14: 377–388
- Nam Y, Weng AP, Aster JC, Blacklow SC. Structural requirements for assembly of the CSL. intracellular Notch1. Mastermind-like 1 transcriptional activation complex. J Biol Chem 2003; 278: 21232–21239
- Motley A, Bright NA, Seaman MN, Robinson MS. Clathrin-mediated endocytosis in AP-2-depleted cells. J Cell Biol 2003; 162: 909–918
- Ohishi K, Katayama N, Shiku H, Varnum-Finney B, Bernstein ID. Notch signalling in hematopoiesis. Semin Cell Dev Biol 2003; 14: 143–150
- Overstreet E, Fitch E, Fischer JA. Fat facets and Liquid facets promote Delta endocytosis and Delta signaling in the signaling cells. Development 2004; 131: 5355–5366
- Parks AL, Klueg KM, Stout JR, Muskavitch MA. Ligand endocytosis drives receptor dissociation and activation in the Notch pathway. Development 2000; 127: 1373–1385
- Pasternak SH, Bagshaw RD, Guiral M, Zhang S, Ackerley CA, Pak BJ, Callahan JW, Mahuran DJ. Presenilin-1, nicastrin, amyloid precursor protein, and gamma-secretase activity are co-localized in the lysosomal membrane. J Biol Chem 2003; 278: 26687–26694
- Pavlopoulos E, Pitsouli C, Klueg KM, Muskavitch MA, Moschonas NK, Delidakis C. Neuralized encodes a peripheral membrane protein involved in Delta signaling and endocytosis. Dev Cell 2001; 1: 807–816
- Pece S, Serresi M, Santolini E, Capra M, Hulleman E, Galimberti V, Zurrida S, Maisonneuve P, Viale G, Di Fiore PP. Loss of negative regulation by Numb over Notch is relevant to human breast carcinogenesis. J Cell Biol 2004; 167: 215–221
- Presente A, Boyles RS, Serway CN, de Belle JS, Andres AJ. Notch is required for long-term memory in Drosophila. Proc Natl Acad Sci U S A 2004; 101: 1764–1768
- Raiborg C, Bache KG, Gillooly DJ, Madshus IH, Stang E, Stenmark H. Hrs sorts ubiquitinated proteins into clathrin-coated microdomains of early endosomes. Nat Cell Biol 2002; 4: 394–398
- Ramain P, Khechumian K, Seugnet L, Arbogast N, Ackermann C, Heitzler P. Novel Notch alleles reveal a Deltex-dependent pathway repressing neural fate. Curr Biol 2001; 11: 1729–1738
- Ray WJ, Yao M, Mumm J, Schroeter EH, Saftig P, Wolfe M, Selkoe DJ, Kopan R, Goate AM. Cell surface presenilin-1 participates in the gamma-secretase-like proteolysis of Notch. J Biol Chem 1999; 274: 36801–36807
- Sakata T, Sakaguchi H, Tsuda L, Higashitani A, Aigaki T, Matsuno K, Hayashi S. Drosophila nedd4 regulates endocytosis of notch and suppresses its ligand-independent activation. Curr Biol 2004; 14: 2228–2236
- Sapir A, Assa-Kunik E, Tsruya R, Schejter E, Shilo BZ. Unidirectional Notch signaling depends on continuous cleavage of Delta. Development 2005; 132: 123–132
- Schantl JA, Roza M, Van Kerkhof P, Strous GJ. The growth hormone receptor interacts with its sheddase, the tumour necrosis factor-alpha-converting enzyme (TACE). Biochem J 2004; 377: 379–384
- Seachrist JL, Anborgh PH, Ferguson SS. Beta 2-adrenergic receptor internalization, endosomal sorting, and plasma membrane recycling are regulated by rab GTPases. J Biol Chem 2000; 275: 27221–27228
- Seugnet L, Simpson P, Haenlin M. Requirement for dynamin during Notch signaling in Drosophila neurogenesis. Dev Biol 1997; 192: 585–598
- Shaye, DD, Greenwald, I. 2002. Endocytosis-mediated downregulation of LIN-12/Notch upon Ras activation in Caenorhabditis elegans. Nature. 686–690.
- Smith CA, Dho SE, Donaldson J, Tepass U, McGlade CJ. The cell fate determinant numb interacts with EHD/Rme-1 family proteins and has a role in endocytic recycling. Mol Biol Cell 2004; 15: 3698–3708
- Sonnichsen B, De Renzis S, Nielsen E, Rietdorf J, Zerial M. Distinct membrane domains on endosomes in the recycling pathway visualized by multicolor imaging of Rab4, Rab5, and Rab11. J Cell Biol 2000; 149: 901–914
- Tian X, Hansen D, Schedl T, Skeath JB. Epsin potentiates Notch pathway activity in Drosophila and C. elegans. Development 2004; 131: 5807–5815
- Varnum-Finney B, Wu L, Yu M, Brashem-Stein C, Staats S, Flowers D, Griffin JD, Bernstein ID. Immobilization of Notch ligand, Delta-1, is required for induction of Notch signaling. J Cell Sci 2000; 113: 4313–4318
- Wang W, Struhl G. Drosophila Epsin mediates a select endocytic pathway that DSL ligands must enter to activate Notch. Development 2004; 131: 5367–5380
- Weber U, Eroglu C, Mlodzik M. Phospholipid membrane composition affects EGF receptor and Notch signaling through effects on endocytosis during Drosophila development. Dev Cell 2003; 5: 559–570
- Weijzen S, Rizzo P, Braid M, Vaishnav R, Jonkheer SM, Zlobin A, Osborne BA, Gottipati S, Aster JC, Hahn WC, et al. Activation of Notch-1 signaling maintains the neoplastic phenotype in human Ras-transformed cells. Nat Med 2002; 8: 979–986
- Weng AP, Aster JC. Multiple niches for Notch in cancer: context is everything. Curr Opin Genet Dev 2004; 14: 48–54
- Weng AP, Ferrando AA, Lee W, Morris JP 4th, Silverman LB, Sanchez-Irizarry C, Blacklow SC, Look AT, Aster JC. Activating mutations of NOTCH1 in human T cell acute lymphoblastic leukemia. Science 2004; 306: 269–271
- Wilkin MB, Carbery AM, Fostier M, Aslam H, Mazaleyrat SL, Higgs J, Myat A, Evans DA, Cornell M, Baron M. Regulation of notch endosomal sorting and signaling by Drosophila nedd4 family proteins. Curr Biol 2004; 14: 2237–2244
- Yamamoto N, Yamamoto S, Inagaki F, Kawaichi M, Fukamizu A, Kishi N, Matsuno K, Nakamura K, Weinmaster G, Okano H, Nakafuku M. Role of Deltex-1 as a transcriptional regulator downstream of the Notch receptor. J Biol Chem 2001; 276: 45031–45040