Abstract
The biological activity of farnesol (FN) and geranylgeraniol (GG) and their isoprenyl groups is related to membrane-associated processes. We have studied the interactions of FN and GG with 1,2-dielaidoyl-sn-glycero-3-phosphoethanolamine (DEPE) membranes using DSC and X-ray diffraction. Storage of samples at low temperature for a long time favors a multidomain system formed by a lamellar crystalline (Lc) phase and isoprenoids (ISPs) aggregates. We demonstrate that ISPs alter the thermotropic behavior of DEPE, thereby promoting a HII growth in a lamellar Lc phase with a reduced degree of hydration. The HII phase occurs with the same repeat distance (dHII=5.4 nm) as the Lc phase and upon heating it expands considerably (δd/δT≈0.22 nm/°C). The dimensional stabilization of this HII phase coincides with the transition temperature of the Lc to Lα phase. Thereafter, the system DEPE/ISP will progress by increasing the nonlamellar-forming propensity and reaching a single HII phase at high temperature. The cooling scan followed a similar structural path, except that the system went into a stable gel phase Lβ with a repeat distance, dLβ=6.5 nm, in co-existence with a HII phase. The formation of ISP microdomains in model PE membranes substantiates the importance of the isoprenyl group in the binding of isoprenylated proteins to membranes and in lipid–lipid interactions through modulation of the membrane structure.
Abbreviations | ||
FN | = | farnesol |
GG | = | geranylgeraniol |
ISP | = | isoprenoid |
PL | = | phospholipid |
PC | = | phosphocholine |
PE | = | phosphoethanolamine |
DEPE | = | 1,2-dielaidoyl-sn-glycero-3-phosphoethanolamine |
Lβ | = | gel lamellar phase |
Lc | = | lamellar-crystalline phase |
Lα | = | liquid-crystalline lamellar phase |
HII | = | inverted hexagonal phase |
Tm | = | gel-to-liquid lamellar phase transition temperature |
TH | = | lamellar-to-hexagonal phase transition temperatures |
Introduction
The isoprenoid (ISP) lipids, farnesol (FN) and geranylgeraniol (GG), are hydrophobic compounds with one double bond and a methyl side chain every four carbon atoms along the main chain and a single hydroxide group at the headgroup (). The biological activity of FN and GG is well-recognized Citation[1–6]. One interesting aspect is that both compounds are implicated in numerous membrane-related biological processes Citation[1], Citation[2], Citation[4]. On the other hand, the prenyl groups, farnesyl (C15) and geranylgeranyl (C20) are attached to proteins at C-terminal cysteine-rich signal sequences, in a post-translational protein modification process Citation[7]. Protein isoprenylation occurs on certain cytoplasmic proteins such as G proteins Citation[8], Rab proteins Citation[9] and Ras proteins Citation[10]. Such proteins are membrane-associated and they act as signal transducers participating in cell regulatory functions Citation[11], Citation[12]. The isoprenyl group of these proteins is known to be crucial for their function Citation[9], Citation[12–16]. The hydrophobicity of the isoprenyl group attached to the protein makes it a good candidate for insertion into the cellular membrane when protein membrane attachment is necessary. It has been reported that prenylated peptides can interact with model phospholipid bilayers Citation[14], Citation[17–19]. Isoprenylation seems to be crucial for the membrane binding of certain proteins Citation[16], Citation[20], Citation[21]. Some studies support the idea that protein-bound prenyl groups act as a membrane anchor in cells and intercalate directly into the cellular membrane Citation[22]. Interestingly, it has been demonstrated that the heterotrimeric Gi protein (Gαβγ) and the Gβγ dimer can bind to PC and PE model membranes. Indeed, the Gβγ dimer drives the interaction of Gi proteins with nonlamellar (hexagonal HII) membrane structures Citation[16].
Although one important mode of the biological activity of FN and GG and their isoprenyl groups is through the membrane-associated events, there have been few studies of how ISPs interact with the lipid bilayer. Insertion of ISPs into PC bilayers has been demonstrated, and also the corresponding effect on the biophysical properties Citation[23–26]. One interesting conclusion is that FN stabilizes the fluid phase and promotes fluid-gel phase co-existence Citation[25], Citation[26]. However, a main question, not yet resolved concerns the ISP role on the lipid polymorphism. We focus our study on the capacity of FN and GG to modulate the thermotropic phase behavior of model PE membranes. By means of differential scanning calorimetry and X-ray scattering analysis, we demonstrate the formation of structural microdomains rich in ISP in a PE surrounding.
Materials and methods
Materials
Farnesol (all-trans-3,7,11-trimethyl-2,6,10-dodecatrien-1-ol) (FN), geranylgeraniol (all-trans-3,7,11,15-tetramethyl-2,6,10,14-hexadecatetraen-1-ol) (GG) and N-(2-hydroxy ethyl) piperazine-N′-(2-ethanesulfonic acid) sodium salt (Hepes) were obtained from Sigma Chemicals Co. (Madrid, Spain). 1,2-dielaidoyl-sn-glycero-3-phosphoethanolamine (DEPE) was purchased from Avanti Polar Lipids, Inc. (Alabaster, USA). Lipids were stored under argon at −80°C, until use. Differential scanning calorimetry (DSC) of multilamellar liposomes was used to evaluate the phospholipid purity.
Model membrane preparation
Multilamellar lipid vesicles (MLV; 15% w/w) were prepared in 10 mM Hepes, 100 mM NaCl, 1 mM EDTA, pH 7.4 (Hepes buffer). For X-ray scattering, the lipid mixtures were hydrated in the presence or absence of farnesol (FN) or geranylgeraniol (GG) at the indicated molar ratios, homogenized with a pestle-type mini-homogenizer (Sigma), and vortexed until a suspension was obtained. The suspensions were then submitted to five heating and cooling cycles (heating to 70°C and cooling to 4°C) as previously reported Citation[27] and stored at −20°C until use. For DSC measurements, the lipid mixtures (5 mg) were dissolved in chloroform, evaporated under argon and vacuum-dried. A known weight of the samples was transferred to an aluminium pan and hydrated by adding the Hepes buffer. The pans were sealed and submitted to ten heating and cooling cycles (to 75°C and to −20°C), to ensure complete homogenization before measurements.
Differential scanning calorimetry (DSC)
Experiments were run in a DSC 2920 scanning calorimeter (TA instruments). The samples were run at a scan rate of 5, 2 and 1°C/min after their preparation. To ensure the reproducibility of the results, three scan cycles were recorded for each sample. Since each scan yielded a similar thermogram, but with the transitions in the cooling curves shifted to lower temperatures, the second heating scan was used to evaluate the thermotropic transitions. The lipid phase transition temperatures (Tm and TH) were determined from the maximum of the excess heat flow vs. temperature curves and the transition enthalpies were obtained from the area under the peaks. To check the reproducibility of DSC measurements, two to three samples of each DEPE:ISP molar ratio were prepared. The deconvolution analysis of the calorimetric peaks was done using mathematical fitting curves.
X-ray scattering
Small and Wide-Angle (SAXS and WAXS) synchrotron radiation X-ray scattering data were collected simultaneously on the Soft Condensed Matter beamline A2 of Hasylab at the storage ring DORIS III of the Deutsches Elektronen Synchrotron (DESY). Data collection was performed as described previously Citation[27]. Samples were heated from 25 to 75°C at a scan rate of 1°C/min; afterwards they were kept at the highest temperature for 5 min and finally cooled down to the lowest temperature at the same scan rate. Positions of the observed peaks were converted into distances, d, after calibration with the standards rat tendon tail and poly-(ethylene therephtalate) for the SAXS and WAXS regions respectively. Interplanar distances, dhkl, were calculated according to Equation 1:Eq. 1 where s is the scattering vector, 2θ is the scattering angle, λ (0.154 nm) is the X-ray wavelength and hkl are the Miller Indexes of the scattering planes.
Results
Differential scanning calorimetry (DSC)
illustrates the DSC thermograms of DEPE alone and in the presence of FN and GG. In the heating mode, DEPE showed two endothermic phase transitions with high cooperativity ( and ), in agreement with earlier reports Citation[28], Citation[29]. The first one corresponds to the gel to liquid-crystalline (Lβ–Lα) phase transition centered at 37.1°C with an enthalpy of 7.2 kcal/mol, while the second one, corresponds to the lamellar to inverted hexagonal (Lα–HII) phase transition centered around 65°C with an enthalpy of 0.6 kcal/mol. An important effect of FN or GG on the thermotropic behavior of DEPE is a concentration-dependent decrease in the TH value and a broadening of the Lα–HII transition peak (a reduction of the thermal transition cooperativity) ( and ). Indeed, the gel-to-liquid crystalline phase transition splits into two main peaks in presence of ISPs (, and ). The shift in the maximum excess heat capacity and the relative area of the two peaks was also dependent on the DEPE:ISP molar ratio (). However, the transition enthalpy of Lβ–Lα and Lα–HII curves was scarcely affected by the presence of ISP in the range of the molar ratio studied (up to 10:1, DEPE:ISP, mol:mol) (). DSC scans of DEPE:ISP mixtures proved to be sensitive to the thermal history of the sample. A sample pre-incubated at −20°C for a long time, showed a transition composed of two peaks with a higher combined enthalpy (11.9 Kcal/mol for DEPE:FN, 20:1 molar ratio) (data not shown). However, when this sample was re-scanned without removing it from the calorimeter, the calorimetric curve also exhibited two peaks but the transition enthalpy decreased (7.2 Kcal/mol). The later scan was identical to the calorimetric profile of DEPE:ISP samples run immediately after their preparation without storage at −20°C (). DSC experiments were performed at different scan rates between 1 and 5°C/min (data not shown). The dependency of the transition temperature on the scan rate was small (≅1°C for both the heating and cooling scans) compared to the shift in the Lα-to-HII phase transition induced by FN and GG ( and ).
Figure 2. DSC heating (a, c) and cooling (b, d) thermograms of aqueous dispersions of 1,2-dielaidoyl-sn-glycero-3-phosphoethanolamine (DEPE) in absence and in presence of farnesol (FN) and geranygeraniol (GG) at the molar ratio indicated. The arrows (panel c) indicate the endothermic peak of the Lα-to-HII phase transition. The scan rate was 2°C/min. (e) Deconvolution of the calorimetric peak of DEPE:FN (20:1, mol:mol) corresponding to the Lβ-to-Lα transition (heating scan): experimental data (solid line), fitted overall (broken line) and individual components (dotted lines).
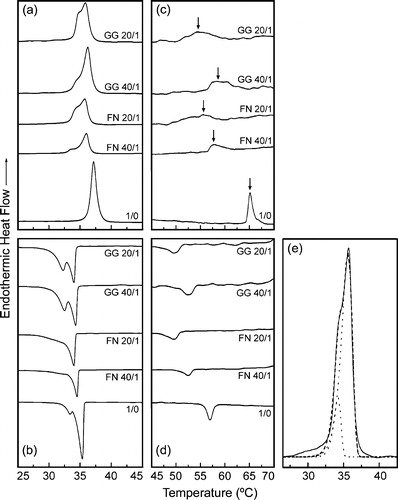
Table I. Calorimetric data of the effect of FN and GN in DEPE mixtures.
X-ray scattering studies
DSC data gave limited information about the gel-to-liquid lamellar and lamellar-to-nonlamellar phase transitions. Therefore, we also studied the structure of DEPE:ISP samples by X-ray scattering ( and ). DEPE:ISP mixtures showed a particularly interesting structural behavior, depending on the time and storage conditions to which they were submitted (). We will focus the analysis mainly on the thermotropic phase behaviour of DEPE:FN at the highest FN concentration studied with this technique (20:1, mol:mol) (). At the beginning of the scan, DEPE:FN (20:1, mol:mol) mixtures stored at −20°C for a long time (days), showed scattering patterns associated with a lamellar-crystalline LcFootnote1 phase (, and ) and a lattice parameter (dLc=5.4 nm) invariant with the temperature and mixture composition. Although the WAXS peaks were not sharp, looking at the scan and comparing it with other phases, one can see the differences and identify the Lc phase. Furthermore, the SAXS patterns also showed high scattering intensity at very small angles which decreased as the temperature increased (up to ≈39°C) (). These properties are characteristics of micellar scattering and suggest a two-phase system. We note a very weak signal at smaller s-values that the peak associated with the Lc phase. It matches the s-value measured for the Lβ phase seen on cooling at the end of the temperature scan. However, no measurable signal from Lβ phase can be seen on the WAXS range of the scattering pattern (, and ). Therefore, even though there is evidence of Lβ phase co-existing with Lc at low temperatures, its proportion is so small that its presence can be neglected. More interestingly, we also observed the appearance (at ca. 34°C) of weak signals (, and ) that were unequivocally associated with a HII phase (dHII=5.4 nm and δd/δT=0.22 nm/°C). The system went into a Lc + HII two-phase region during a short range temperature (ΔTLc–HII=34–38°C). The Lc phase completely melted at 38°C, going into a Lα phase (dLα=5.5 nm), still co-existing with an HII phase (ΔTLα–HII=39–59°C). Finally, a single HII phase was reached at 60°C (dHII=6.3 nm at 72°C and δd/δT= − 0.024 nm/°C). It is worth noting that the co-existence of Lc and HII phases are not commonly seen in phospholipids, however phase transitions from Lc-to-HII have been observed in mixtures of saturated short-chain phosphatidylcholines and the respective fatty acids Citation[30], glycoglycerolipids Citation[31–33] and DSPE dispersed in glycerol/water (>70wt% glycerol) Citation[34].
Figure 3. Sequence of SAXS (left) and WAXS (right) scattering patterns of (a, b) DEPE, (c, d) DEPE:FN (20:1, mol:mol) and (e, f) DEPE:GG (20:1, mol:mol). The scan rate was 1°C/min. Successive diffraction patterns were collected for 15 s every minute. The thermal sequence of the transition from (Lc + HII) to Lα and Lα-to-HII phases was clearly observed in the heating scan. A Lβ phase occur at the end of the cooling scan. The Lc and Lβ phases were identified by the reflections in the WAXS region. The Lα phase was identified by a single peak of reflection in the SAXS region with a very good signal-to-noise ratio. The HII phase was characterised by the appearance of three diffraction orders in the SAXS region with a d-spacing ratio of 1:1/√3:1/√4.
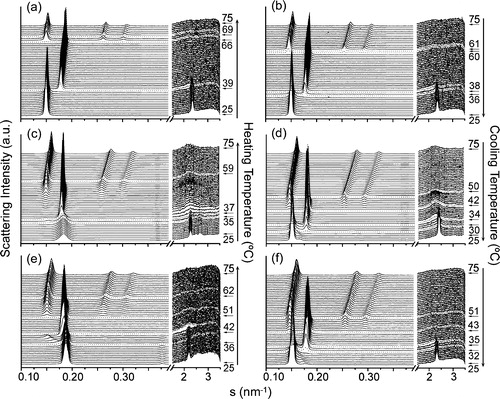
Figure 4. Sequence of scattering patterns from DEPE:FN (20:1, mol:mol). (a) In the heating scan, the transition from Lc to Lα is seen on the WAXS part of the scattering. Also the transition from Lc into HII (associated peaks marked by arrows) and Lα (sharp peaks) is evidenced. (b) In the cooling sequence, the transition from HII+Lc to Lα and the re-entrant HII co-existing with Lβ is shown.
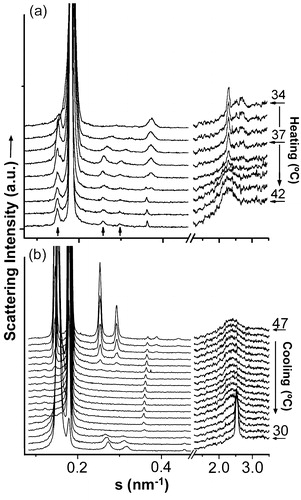
Figure 5. Dependence of the interplanar repeat distance (d) on temperature for (a) DEPE heating run, (b) DEPE:FN (40:1, mol:mol) heating run, (c) DEPE:FN (20:1, mol:mol) heating and (d) cooling run, (e) DEPE:GG (40:1, mol:mol) heating run and (f) DEPE:GG (20:1, mol:mol) heating run. The different phases represented are: Lc, Lβ, Lα and HII. The co-existence of the Lc + HII and Lα+HII phases is indicated by the interval temperature range covered by the vertical lines. The spacing corresponding to the first order of the re-entrant HII phase is not plotted for simplicity of the figure.
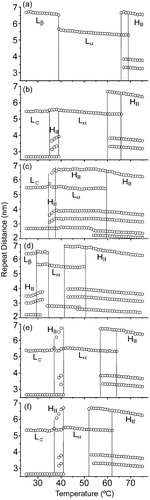
Table II. Summary of the structural properties.
The cooling process also followed an interesting structural path (, and ). First, we observed the Lα phase occurring as a single phase between 42 and 35°C and then, a re-entrant HII phase. Second, the system reached a stable gel Lβ phase (dLβ=6.5 nm) instead of the crystalline Lc phase co-existing with the HII phase. The gel phase was stable over a period of days. Only a weak signal associated with the Lc phase could be seen at the end of the cooling scan. Taking the relative proportions of the scattering peaks as indicative of the amount of each phase present, we could estimate that there were only traces of the Lc phase at the end of the scan. However, DEPE:FN mixtures pre-heated to 45°C and then cooled to 25°C (the starting point of the temperature scan) showed only the Lβ phase in the heating scan (data not shown).
DEPE:GG mixtures showed similar diffraction patterns and sequence of phases (, , and ). Comparatively, GG increased the temperature for the range of co-existence between the Lc + HII phases (36–42°C). Nevertheless, the main differential effect was on the transition temperature of the Lα-to-HII phase (). GG was more efficient to lower both the onset and the end of the transition temperature (ΔTLα–HII=51–62°C for DEPE:GG at 20:1, mol:mol).
Discussion
The isoprenoids, FN and GG, affected the thermotropic behavior of the nonlamellar-prone phospholipid DEPE by altering the gel-to-liquid lamellar phase transition and promoting the nonllamelar phase, HII (). DEPE:ISP mixtures showed scattering patterns that allowed characterization of the Lc, Lβ, Lα and HII phases. Interestingly, the two ISP molecules exhibited similar trends and promoted an HII phase in co-existence with the Lc or the Lβ phase (, and ).
DSC profiles show asymmetric peaks in the (Lc + Lβ)-to-Lα phase transition, indicating that DEPE:ISP mixtures exhibit non-ideal mixing (). These data suggest that ISP induced the appearance of domains in the (Lc + Lβ)-to-Lα phase transition. However, the main argument comes from the X-ray data that demonstrate unequivocally the presence of co-existing domains (). Considering the X-ray scattering pattern of samples previously heated (), we can conclude: (i) the first calorimetric peak (Tm1) is associated with a co-existence of Lc/Lβ and HII phases and (ii) the second peak (Tm2) matches with the range temperature of co-existence of Lβ and Lα phases. In addition, the Lα phase alone appears on cooling at a temperature (≈42°C) and was scarcely affected by the presence of the ISP molecules.
It should be noted that the form of the DEPE:ISP thermograms differs from the ones previously reported with the lamellar-prone phospholipid DMPC:FN mixtures Citation[19], Citation[25], Citation[26]. From DSC and 2H-NMR, it was concluded that FN promotes the Lβ–Lα phase co-existence and induces a marked reduction in the Tm value and the cooperativity of the phase transition Citation[19], Citation[25], Citation[26]. These studies support a model in which FN partitions preferentially into the liquid Lα phase, without affecting the lipid chain order. However, our data showed that ISPs induce a HII phase in co-existence with either Lc or Lβ phases at physiological temperature (i.e., near 37°C). Indeed, the transition of DEPE:ISP to the Lα phase does not show the common characteristics of a DEPE temperature-induced Lα phase formation (compare and ). These differences between DEPE:ISP and DMPC:FN systems should be due to the differences in the molecular geometry of PE compared to PC phospholipids, which facilitate the aggregation of the ISP in the former class of lipids.
The thermotropic behavior of DEPE:ISP samples in relation to the formation of the Lc or Lβ phase at a low temperature (below 34°C) (, ), should be interpreted in terms of a balance between the thermodynamic and the kinetic parameters to reach each phase formation. Thus, the more stable crystalline Lc phase requires a longer time (days) for its formation at low temperatures. Considering intuitively, that the transition from Lc-to-Lα phases increases the entropy of the system and that the transition enthalpy is similar to the Lβ-to-Lα phase transition, we conclude that the energy levels of the phases Lc and Lβ should be similar. Moreover, because only one phase, crystalline or gel, was seen at once in considerable amounts, at the beginning of the heating or at the end of the cooling scans, we suggest that the activation energy of the Lc/Lβ transition should be significantly high. This is not surprising, because the Lc and Lβ structures have different molecular arrangements, making the transition between them difficult to occur, and therefore slow.
Thermotropic transitions from multicomponent systems were observed in lipid–lipid and lipid–peptide mixtures Citation[35–38]. The molecular shape of the lipids and the packing characteristics of hydrocarbon side chains were reported as the main cause to induce lateral heterogeneity. However, we propose the following model to interpret the particular structural behaviour of DEPE:ISP mixtures: Storage of samples at a low temperature for a long time favours a system formed by a layered crystalline Lc phase with a rather reduced hydration. Due to the low miscibility between ISPs and lipid molecules, ISPs would aggregate to form ISP-rich domains in the Lc or the Lβ phase of DEPE. ISP lipids are characterized by a small headgroup and a large hydrocarbon cross-sectional area due to the methyl branches along the chain (inverted molecular geometry). Therefore, ISP aggregates will strongly promote the formation of HII phases in water-poor conditions. The HII phase expands until 38°C, when the Lc-to-Lα phase transition temperature is reached. Then, the increased fluidity enhances diffusion and miscibility of ISP into the DEPE matrix forming ISP-poor domains into the fluid phase. Thereafter, the DEPE:ISP system will progress by increasing the nonlamellar-forming propensity and the formation of a HII phase, as observed by calorimetry and X-ray diffraction. The Lα-to-HII phase transition follows a similar trend as the systems formed by DEPE and unsaturated fatty acids previously reported Citation[27], Citation[29].
This model is supported by the observation that (1) the initial HII phase occurs with the same repeat distance (dHII=5.4 nm) as the Lc phase, i.e., it fits well within the Lc bilayer, and upon heating it expands considerably (δd/δT≈0.22 nm/°C); and (2) the lattice parameter stabilization of the HII phase coincides with the transition temperature of the Lc-to-Lα phase. Further support for such organization of DEPE:ISP components into Lc/HII domains could be found by estimating the molecule sizes and the lattice parameters of the phases involved. A simple estimation can be done by comparing the lattice parameter of the Lc phase with the molecular length of DEPE. A PE bilayer containing 18 all-trans carbons on each side would be 5.6 nm thick (assuming 0.5 nm for the lipid headgroup on each side). This value agrees with the experimental lattice parameter for the Lc phase (dLc=5.4 nm) and the initial d-value of the HII phase. Although the experimental data do not give unequivocal evidence for such model, they are in line with the molecular mechanism for the lamellar/inverse hexagonal phase transition described by Rappolt et al. Citation[39]. Interestingly, it has been shown that POPE undergoes a Lβ-to-HII phase transition in a water-poor system Citation[40]. Thus, DEPE:ISP systems constitute a new example where an HII domain grows in a multilamellar matrix with a reduced degree of hydration.
Biological significance
The reported data are related to the context of (i) lipid microdomains in a membrane-rich in PE lipids and (ii) the role that isoprenyl groups could play in the isoprenyl protein membrane interactions. Our experimental concentrations of ISP used in vitro are high, compared to those of ISP or isoprenyl groups protein-bound in a cellular system. However, the presence of structural microdomains rich in ISP, observed within the temperature range of the gel/fluid phases in DEPE membranes, could substantiate the role of lipid-driven protein targeting to cellular membranes. If this is the case, it is likely that the isoprenyl group could be a protein anchoring to enhance the clustering of prenylated proteins in a PE surrounding and through it modulate the membrane structure. We have recently demonstrated that the presence or propensity to form inverted hexagonal (HII) phases favors the membrane association of Gαβγ heterotrimer and Gβγ dimer proteins, whereas Gα monomers prefer ordered lamellar structures Citation[16]. In this context, the isoprenyl group on Gγ subunits could favor recruitment of G protein heterotrimers to nonlamellar-prone phases and facilitate the subsequent exit of activated Gα monomers to lamellar-prone regions, e.g., membrane rafts. This possibility represents a new point to be considered in biophysical studies of the cellular signaling process, in addition to the importance of rafts. This paper was first published online on prEview on 29 June 2005.
This work was supported by grants SAF2001-0839, SAF2003-00232, SAF2004-05249 (Ministerio de Educación y Ciencia, Spain), PRDIB-2002GC2-11 (Govern Balear), and I-02-066EC from Deutsches Elektronen-Synchrotron DESY, HASYLAB (Hamburg, Germany) from the IHP-Contract HPRI-CT-2001-00140 of the European Commission. We are grateful to Dr J. Cifre for his laboratory assistance.
Notes
1A similar phase was analysed by DSC and 31P NMR and it was called “subgel” phase (Lewis R, Mannock DA, McElhaney RN 1989). Effect of fatty acyl chain length and structure on the lamellar gel to liquid-crystalline and lamellar to reversed hexagonal phase transitions of aqueous phosphatidylethanolamine dispersions. Biochemistry 28: 541–548).
References
- Bard M, Albrecht MR, Gupta N, Guynn CJ, Stillwell W. Geraniol interferes with membrane functions in strains of Candida and Saccharomyces. Lipids 1988; 23: 534–538
- Bringmann A, Skatchkov SN, Faude F, Enzmann V, Reichenbach A. Farnesol modulates membrane currents in human retinal glial cells. J Neurosci Res 2000; 62: 396–402
- Correll CC, Ng L, Edwards PA. Identification of farnesol as the non-sterol derivative of mevalonic acid required for the accelerated degradation of 3-hydroxy-3-methylglutaryl-coenzyme A reductase. J Biol Chem 1994; 269: 17390–17393
- Haug JS, Goldner CM, Yazlovitskaya EM, Voziyan PA, Melnykovych G. Directed cell killing (apoptosis) in human lymphoblastoid cells incubated in the presence of farnesol: effect of phosphatidylcholine. Biochim Biophys Acta 1994; 1223: 133–140
- Rioja A, Pizzey AR, Marson CM, Thomas NS. Preferential induction of apoptosis of leukaemic cells by farnesol. FEBS Lett 2000; 467: 291–295
- Takahashi N, Kawada T, Goto T, Yamamoto T, Taimatsu A, Matsui N, Kimura K, Saito M, Hosokawa M, Miyashita K, Fushiki T. Dual action of isoprenols from herbal medicines on both PPARgamma and PPARalpha in 3T3-L1 adipocytes and HepG2 hepatocytes. FEBS Lett 2002; 514: 315–322
- Glomset JA, Farnsworth CC. Role of protein modification reactions in programming interactions between ras-related GTPases and cell membranes. Annu Rev Cell Biol 1994; 10: 181–205
- Dietrich A, Scheer A, Illenberger D, Kloog Y, Henis YI, Gierschik P. Studies on G-protein alpha.beta gamma heterotrimer formation reveal a putative S-prenyl-binding site in the alpha subunit. Biochem J 2003; 376: 449–456
- Pereira-Leal JB, Hume AN, Seabra MC. Prenylation of Rab GTPases: molecular mechanisms and involvement in genetic disease. FEBS Lett 2001; 498: 197–200
- Leventis R, Silvius JR. Lipid-binding characteristics of the polybasic carboxy-terminal sequence of K-ras4B. Biochemistry 1998; 37: 7640–7648
- Casey PJ. Protein lipidation in cell signaling. Science 1995; 268: 221–225
- Sinensky M. Functional aspects of polyisoprenoid protein substituents: roles in protein-protein interaction and trafficking. Biochim Biophys Acta 2000; 1529: 203–209
- Niv H, Gutman O, Henis YI, Kloog Y. Membrane interactions of a constitutively active GFP-Ki-Ras 4B and their role in signaling. Evidence from lateral mobility studies. J Biol Chem 1999; 274: 1606–1613
- Moffett S, Brown DA, Linder ME. Lipid-dependent targeting of G proteins into rafts. J Biol Chem 2000; 275: 2191–2198
- Paz A, Haklai R, Elad-Sfadia G, Ballan E, Kloog Y. Galectin-1 binds oncogenic H-Ras to mediate Ras membrane anchorage and cell transformation. Oncogene 2001; 20: 7486–7493
- Vogler O, Casas J, Capo D, Nagy T, Borchert G, Martorell G, Escriba PV. The Gbeta gamma -dimer drives the interaction of heterotrimeric Gi proteins with nonlamellar membrane structures. J Biol Chem 2004; 279: 36540–36545
- Ghomashchi F, Zhang X, Liu L, Gelb MH. Binding of prenylated and polybasic peptides to membranes: affinities and intervesicle exchange. Biochemistry 1995; 34: 11910–11918
- Silvius JR. Peptide–Lipid Interactions. Currents Topics in Membranes, S Simon, T McIntosh. Academic Press, New York 2002; 52: 371–395
- Rowat AC, Brask J, Sparrman T, Jensen KJ, Lindblom G, Ipsen JH. Farnesylated peptides in model membranes: A biophysical investigation. Eur Biophys J 2004; 33: 300–309
- Spiegel AM, Backlund PS, Jr, Butrynski JE, Jones TL, Simonds WF. The G protein connection: molecular basis of membrane association. Trends Biochem Sci 1991; 16: 338–341
- Hagiwara K, Wada A, Katadae M, Ito M, Ohya Y, Casey PJ, Fukada Y. Analysis of the molecular interaction of the farnesyl moiety of transducin through the use of a photoreactive farnesyl analogue. Biochemistry 2004; 43: 300–309
- Magee AI, Seabra MC. Are prenyl groups on proteins sticky fingers or greasy handles?. Biochem J 2003; 376: e3–e4
- McCloskey MA, Troy FA. Paramagnetic isoprenoid carrier lipids. 1. Chemical synthesis and incorporation into model membranes. Biochemistry 1980; 19: 2056–2060
- McCloskey MA, Troy FA. Paramagnetic isoprenoid carrier lipids. 2. Dispersion and dynamics in lipid membranes. Biochemistry 1980; 19: 2061–2066
- Bondar OP, Melnykovych G, Rowe ES. Effects of farnesol on the thermotropic behavior of dimyristoylphosphatidylcholine. Chem Phys Lipids 1994; 74: 93–98
- Rowat AC, Davis JH. Farnesol-DMPC phase behavior: A (2)H-NMR study. Biochim Biophys Acta 2004; 1661: 178–187
- Funari SS, Barcelo F, Escriba PV. Effects of oleic acid and its congeners, elaidic and stearic acids, on the structural properties of phosphatidylethanolamine membranes. J Lipid Res 2003; 44: 567–575
- Lewis RNAH, Mannock DA, McElhaney RN. Membrane lipid, molecular structure and polymorphism. Lipid Polymorphism and Membrane Properties, R Epand. Academic Press, San Diego 1997; 25–102
- Barcelo F, Prades J, Frau J, Funari SS, Alemany R, Escriba PV. The hypothensive drug 2-hydroxyoleic acid modifies the structural properties of model membranes. Mol Membr Biol 2004; 21: 261–268
- Koynova R, Tenchov B, Rapp G. Mixing behavior of saturated short-chain phosphatidylcholines and fatty acids. Chem Phys Lipids 1997; 88: 45–61
- Kuttenreich H, Hinz H-J, Inczedy-Marcsek M, Koynova R, Tenchov B, Laggner P. Polymorphism of synthetic 1,2- dialkyl-3-O-β-D-galactosyl-sn-glycerols of different chain lengths. Chem Phys Lipids 1988; 47: 245–260
- Hinz HJ, Kuttenreich H, Meyer R, Renner M, Frund R, Koynova R, Boyanov AI, Tenchov BG. Stereochemistry and size of sugar head groups determine structure and phase behavior of glycolipid membranes: densitometric, calorimetric, and X-ray studies. Biochemistry 1991; 30: 5125–5138
- Tenchova R, Tenchov B, Hinz H-J, Quinn PJ. Lamellar/non-lamellar phase transitions in synthetic glycoglycerolipids studied by time-resolved X-ray diffraction. Liq Crys 1996; 20: 469–482
- Williams WP, Quinn PJ, Tsonev LI, Koynova RD. The effects of glycerol on the phase behavior of hydrated distearoylphosphatidylethanolamine and its possible relation to the mode of action of cryoprotectants. Biochim Biophys Acta 1991; 1062: 123–132
- Latal A, Degovics G, Lohner K. Phase separation of enriched phosphatidylglycerol domains in mixtures with phosphatidylethanolamine. Chem Phys Lipids 1998; 94: 161–170
- McMullen TP, Lewis RN, McElhaney RN. Calorimetric and spectroscopic studies of the effects of cholesterol on the thermotropic phase behavior and organization of a homologous series of linear saturated phosphatidylethanolamine bilayers. Biochim Biophys Acta 1999; 1416: 119–134
- Lohner K, Prenner EJ. Differential scanning calorimetry and X-ray diffraction studies of the specificity of the interaction of antimicrobial peptides with membrane-mimetic systems. Biochim Biophys Acta 1999; 1462: 141–156
- Lohner K, Latal A, Degovics G, Garidel P. Packing characteristics of a model system mimicking cytoplasmic bacterial membranes. Chem Phys Lipids 2001; 111: 177–192
- Rappolt M, Hickel A, Bringezu F, Lohner K. Mechanism of the lamellar/inverse hexagonal phase transition examined by high resolution x-ray diffraction. Biophys J 2003; 84: 3111–3122
- Katsaras J, Jeffrey KR, Yang DS, Epand RM. Direct evidence for the partial dehydration of phosphatidylethanolamine bilayers on approaching the hexagonal phase. Biochemistry 1993; 32: 10700–10707