Abstract
Amphotericin B (AmB) is one of the main antibiotics applied in treatment of deep-seated mycotic infections. Tensiometric technique has been applied to monitor binding of AmB, from the water subphase, to the lipid monomolecular layers, formed with dipalmitoylphosphatidylcholine at the air-water interface. Time dependencies of surface pressure in the monolayers demonstrate strong enhancement of AmB binding to monolayers brought about by sterols present in the membranes. The monolayers have been deposited to a solid support and examined by means of FTIR spectroscopy. FTIR measurements show that majority of the AmB molecules which bind to the membranes are localized in the polar headgroup region. The results of the linear dichroism-FTIR measurements are consistent with the microscopic picture according to which the molecules of the membrane-bound AmB are distributed among two orientational fractions: one horizontal and one vertical with respect to the plane of the membrane (59% versus 41% respectively, in the case of the membrane formed with the pure lipid without sterols). The presence of cholesterol in the membranes (50 mol% with respect to lipid) slightly affects such a distribution (53% horizontal versus 47% vertical) but the presence of ergosterol has a pronounced effect in the increase in population of the fraction of horizontally bound AmB (85% horizontal vs. 15% vertical). The results of the measurements indicate that mode of action of the AmB consists in disruption of the polar headgroup region of biomembranes, brought about by the AmB molecules bound horizontally with respect to the plane of the membrane.
Introduction
Amphotericin B (AmB) is a polyene antibiotic used in medical treatment of deep-seated fungal infections Citation[1–3]. According to the general conviction, the pharmacological action of the drug is based on the formation of membrane pores that considerably affect physiological ion transport Citation[4–8]. It is postulated that such membrane channels are permeable to monovalent ions, water, and small molecules Citation[9], Citation[10]. Sterols are postulated to facilitate formation of transmembrane channels across lipid membranes Citation[5], Citation[6], including cholesterol present in the cells of mammals and ergosterol present in the cells of fungi (see ).
The pharmacological effect of AmB is based on selectivity in the interaction of the drug towards ergosterol vs. cholesterol but severe toxic side effects of AmB to patients have been reported Citation[2]. The effect of AmB on the transmembrane ion transport has also been observed in the lipid membrane system containing the drug without any sterol components Citation[11]. It has been postulated that not only formation of porous molecular structures, but also modification of the physical properties of the lipid bilayers modulates membrane permeability to ions Citation[12]. Such mechanisms can be particularly effective at low concentrations of AmB, promoting monomeric organization of the drug within the lipid phase Citation[8], Citation[13]. On the other hand, owing to the amphiphilic nature of AmB (), its binding to the hydrophobic core of lipid membranes is not favourable for energetic reasons Citation[14]. In most studies on molecular organization of AmB in the lipid membranes, including those carried out in our laboratory Citation[8], Citation[12], Citation[13], Citation[15], the drug was incorporated into the membrane system from the organic phase, during liposome or lipid multibilayer preparation. It seems that the approach that consists in examining the interaction of the drug introduced into the water phase with the membrane system is closer to the natural physiological conditions. The monomolecular layer technique has been also applied to investigate binding of AmB, dispersed in the water phase, to the lipid films Citation[16], Citation[17]. In the present work we apply this approach combined to FTIR-linear dichroism to address the problem of interaction of AmB with lipid membranes.
Materials and methods
Chemicals
Dipalmitoylphosphatidylcholine (DPPC), cholesterol, ergosterol and crystalline amphotericin B (AmB) were purchased from Sigma Chem. Co. (USA). Egg yolk phosphatidylethanolamine (EYPE) and diphytanoylphosphatidylcholine (DPhPC) were purchased from Avanti Polar Lipids Inc. (USA). Chemicals were stored under argon in a deep-freezer.
Monomolecular layers
AmB was dissolved in DMSO or in deionized (mQ) water alkalized to pH 12 with KOH and then centrifuged for 15 min at 5000×g in order to remove microcrystals of the drug, still remained in the sample. A stock solution of AmB was adjusted to 1 mg/ml. Lipids (DPPC, DPhPC, EYPE, cholesterol, ergosterol) were dissolved in chloroform. Monomolecular layers of pure lipids and two-component monolayers of lipids and sterols (typically 1:1 molar ratio) were formed at the air-water interface. Monolayers were formed at the air-water interface and the water subphase was buffered with 10 mM Hepes, pH 7.2. Monomolecular layers were formed in a Teflon trough (282 mm×75 mm) and were compressed along the long side with a speed of 15 mm/min. Surface pressure was monitored by a computer-controlled tensiometer, model KSV, Helsinki, Finland. Monomolecular layers were deposited to a solid support by means of the Langmuir-Blodgett technique (L-B films), with a speed of lift 5 mm/min at a constant, computer-controlled surface pressure. In order to remove residuals of water, a thin L-B films were placed in a vacuum for 1 h. Monolayer compression and deposition was carried out at 26±1°C.
Infrared absorption measurements (FTIR) and linear dichroism measurements
Infrared absorption spectra were recorded with the Fourier-transform infrared (FTIR) spectrometer, model Bio-Rad FTS 185, equipped with a MCT detector and KBr beamsplitter. Before measurements the instrument was purged with CO2-free dry air for 40 min. The attenuated total reflection (ATR) configuration was used with a 10-reflections Ge crystal (45° cut). Typically 200 interferograms were collected, Fourier transformed and averaged. Absorption spectra in the region between 4000 and 600 cm−1, at a resolution of one data point every 2 cm−1, were obtained using a clean crystal as the background. ATR crystals were cleaned with organic solvents and for 30 min by ‘Harric’ Plasma Cleaner. Glan-Taylor polarizer was used in the IR polarization experiments. Spectral analysis was performed with Grams 32 software from Galactic Industries (USA).
Interpretation of FTIR linear dichroism measurements
FTIR technique with polarization of the IR beam was used to obtain information on molecular order and orientation. This is based on the fact that the absorbance (A) is proportional to the square of the product of the transition dipole moment (µ) and the projection of the electric field (E) of the polarized IR beam on the direction of the transition dipole:1 where φ is the angle between the directions of µ and E.
The dichroic ratio (R) is defined as a ratio of the integrated absorption bands or the absorbance values corresponding to the selected vibrational modes in the parallel, AII and perpendicular A⊥ configuration:2 In practice, because of orientational fluctuations of the molecules in lipid bilayers, an ensemble of molecular orientations contribute to the measured dichroic ratio. Therefore, it is convenient and customary in membrane spectroscopy to analyse polarized IR spectra in terms of the order parameters (S) defined as:
3 where θ is the average tilt angle of the molecular axis with respect to the axis perpendicular to the plane of the membrane (z axis). On the other side S is related to the dichroic ratio by:
4 where α is the angle between the transition moment of the investigated vibration and the longest axis of the molecule Citation[18], Citation[19]. In the case of the lipid chains, for example, α is 90° for the symmetric and antisymmetric CH2 stretching and 0° for the symmetric CH3 stretching. Typical value found for the phospholipid vesicles in the liquid crystalline state is R = 1.3 for the band at 2851 cm−1. The corresponding order parameter is S = 0.52 and the orientation angle θ = 34.5° Citation[20]. Ex, Ey and Ez are the components of the electric field of the evanescent wave in the three directions and can be expressed as follows:
5
6
7 Where γ is the angle of incidence of the IR beam (45°), n1,n2,n3 are the refractive indexes of the internal reflection Ge plate (4.0), of the supported lipid membrane (1.40), and of the air (1.0) respectively. In the Equations 5–7 n32=n3/n2 and n31=n3/n1. The values of E2x=1.99, E2y=2.13, E2z=0.59 Citation[18]. The nominal penetration depth of the electromagnetic field into the sample for Ge crystals is between 1.1 and 1.5 µm Citation[21].
Results and discussion
Monomolecular layers at the air-water interface
Monomolecular layer technique has been applied in the present study to address the problem of incorporation of AmB into lipid membranes. A similar approach has been already applied in the precise studies carried out by Barwicz and Tancrede Citation[17] and by Dynarowicz-Latka et al. Citation[16]. presents the effect of the injection of AmB solution into the subphase on the surface pressure at the air-water interface.
Figure 2. Surface pressure increase after the injection of AmB beneath the air-water interface. Each arrow indicates the injection of 10 µl of AmB solution into 12 ml of buffer. After single injection the AmB concentration in the subphase was increased by 0.9 µM. The solution of AmB was prepared either in DMSO (1) or in water alkalized with KOH to pH 12 (3). Trace No. 2 represents the time-dependency of the surface pressure after injection of the same volume of pure DMSO. Inset shows comparison of the effects of AmB injected in the form of solution in DMSO and water pH 12 on surface pressure changes.
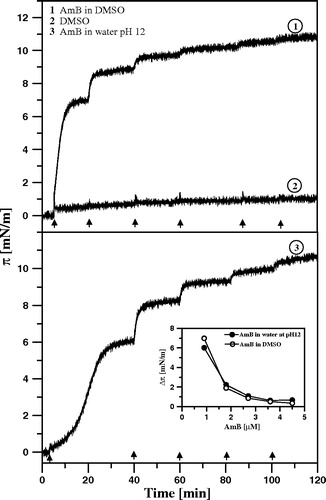
As can be seen, the surface pressure approaches a steady-state level close to 11 mN/m in the consequence of injection of AmB solution, independently of the actual method of AmB solubilization (DMSO versus H2O at high pH, see inset to ). The surface pressure of 11 mN/m represents the adsorption status of AmB at the air-water interface corresponding to the microscopic organization of the interfacial layer with horizontally oriented molecules of the drug Citation[7].
presents the isotherm of compression of pure AmB monolayer formed at the air-water interface. The semi-plateau portion of the isotherm, visible in the surface pressure region 11–15 mN/m has been interpreted as related to the compression-induced reorientation of AmB molecules in the monolayer and separates two different phases of the monolayer, characterized by horizontal (below 11 mN/m) and roughly vertical (above 15 mN/m) orientations of the molecules Citation[7], Citation[12], Citation[22]. These two distinct orientations of AmB molecules in the monolayer at the air-water interface has been characterized by two specific molecular area values: 136 Å2 and 36 Å2, corresponding very well to the crystallographic dimensions of the drug Citation[7]. The vertical orientation is most probably stabilized by the hydrogen bond interaction of the macrolide ring-bound sugar mycosamine with the subphase and the horizontal orientation by the same kind of interaction with polar groups (mostly hydroxyls) located along the longer molecular axis of AmB (see ). presents also the isotherm of compression of the monomolecular layer formed with pure DPPC. As can be seen, the monolayer remains in the so-called solid state film at surface pressures higher than 15 mN/m. A surface pressure of 22 mN/m has been selected for further experiments on incorporation of AmB into DPPC monomolecular membranes in order to mimic compact lipid layer and conditions at which the membrane may be penetrated by the drug present in the water phase.
Figure 3. Isotherms of compression of monomolecular layers formed with pure AmB (solid line) and pure DPPC (dotted line).
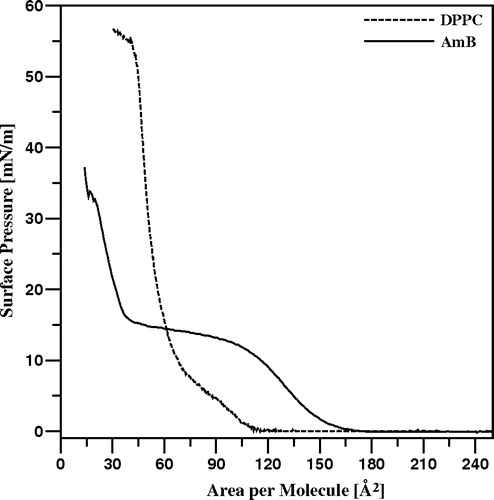
presents the effects of the injection of AmB solution into the subphase, beneath the lipid film formed at the air-water interface. It is expected that relatively small fractions of AmB would penetrate a monolayer from the subphase. On the other hand, it has been shown that just in the low molar fractions range of AmB in DPPC (below 10 mol%), the average molecular areas of the two-component monolayers display pronounced overadditivity Citation[12]. It is therefore highly probable that at those concentrations, the AmB molecules remain in the monomeric form (unlike in the case of one-component monolayer Citation[7]) and the drug molecules are horizontally oriented, even at high surface pressure values. Three kinds of films were studied, the mono-component DPPC films, the two-component films composed of DPPC and cholesterol and the two-component films composed of DPPC and ergosterol. As can be seen, both sterols influence considerably incorporation of AmB into the lipid monolayers, as demonstrated by changes in the surface pressure of the membranes. In the case of ergosterol the effect of AmB-induced increase in the surface pressure is exceptionally strong (more than 16 mN/m). On the other hand, the surface pressure tends to decrease following the initial increase, in the ergosterol-containing membranes. This effect can be observed very distinctly after single injection of AmB solution beneath the DPPC monolayer (C). One of the possible interpretations of this effect is that the molecules of membrane-bound AmB aggregate in course of the experiment Citation[8], Citation[15], Citation[23]. It is also probable that the molecules of membrane-bound AmB influence orientation and/or localization of ergosterol, which result in the decrease in the surface pressure, following the initial increase, directly related to the penetration of AmB into the monolayers. The collapse pressure of the two-component monolayers formed with DPPC:ergosterol (1:1) is close to 50 mN/m Citation[16], far above the surface pressure at which the effect is observed (in the region of 40 mN/m). On the other hand, incorporation of relatively small fractions of AmB into the DPPC monolayers decreases effectively the apparent collapse pressure (for example to 40 mN/m, at 6 mol% AmB, Citation[12]). It is therefore highly probable that the effect of decrease in the surface pressure observed reflects the dynamic and structural properties of the monolayer under conditions close to the collapse of the film. The structural differences between cholesterol and ergosterol (see ), that influence both the sterol-AmB molecular interactions and the effect of the sterols on the physical properties of DPPC membranes, (including fluidity and penetration barrier to AmB) can be assigned as responsible for the particularly strong effect of ergosterol in enhancement of binding AmB to DPPC membranes as compared to the effect of cholesterol Citation[17]. It is probable that the additional methyl group in the mobile fragment of the ergosterol molecule, as compared to the cholesterol molecule (), affects the tight molecular packing within the hydrocarbon zone and facilitates penetration of AmB. In fact, the penetration of AmB into a lipid monolayer formed with DPhPC, the derivative of DPPC with additional four methyl groups substituted to each acyl hydrocarbon chain, was found to be much more efficient as comparing to the pure DPPC membranes, (see ).
Figure 4. Surface pressure increase after the injections (marked with arrows) of 10 µl of AmB solution in DMSO (panel A) or in water pH 12 (panel B) into the air-water subphase (12 ml), beneath the monomolecular layers at the surface pressure 22 mN/m, formed with: DPPC (1), DPPC + Cholesterol 1:1 (2) and DPPC + Ergosterol 1:1 (3). Each injection of AmB increased its concentration by 0.9 µM. Panel C presents the effect of the single injection. Temperature 26°C.
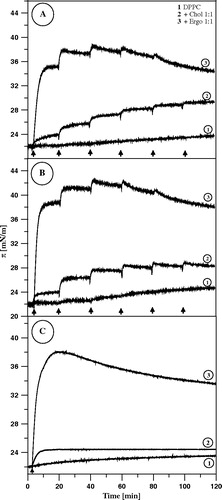
Figure 5. Surface pressure increase after the injection (marked with an arrow) of 10 µl of AmB solution in DMSO into the air-water subphase (12 ml), beneath the monomolecular layers at the surface pressure 22 mN/m, formed with: DPhPC, DPPC and EYPE (indicated). The final concentration of AmB was 0.9 µM. Temperature 26°C.
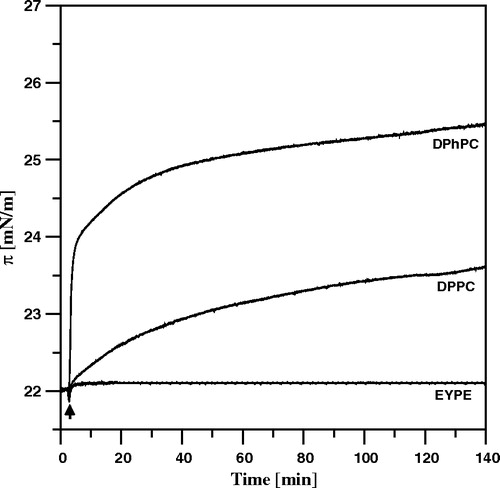
On the other hand the effect of AmB on the surface pressure was not as strong as the effect associated with the presence of sterols. This difference indicates also that other molecular mechanisms are responsible for the strong effect of sterols on binding AmB to lipid membranes. The polar headgroup region of the lipid monolayer is a first barrier to the drug molecule and therefore an indirect effect of the organization of the hydrocarbon chains on organization of the polar head groups can be also important in the process of penetration of AmB into the lipid membranes. It is interesting that binding of AmB is almost totally ineffective in the case of the monolayer formed with phosphatidylethanolamine, the lipid characterized by relatively small polar head cross-section (see ). Despite very clear demonstration of the penetration mechanism of AmB into lipid membranes and relatively high surface pressures applied it is not clear whether AmB molecules bind into the hydrocarbon core of the membranes vertically or rather penetrate only the polar zone and remain in the horizontal orientation as discussed in the case of the lipid bilayer-bound AmB Citation[8], Citation[15], Citation[24]. In order to address this problem we performed linear dichroism-FTIR experiments as described below.
FTIR and FTIR-linear dichroism experiments
presents the IR absorption spectra of single AmB monolayer deposited to the ATR Ge crystal, recorded with radiation polarized parallel and perpendicular with respect to the plane of incidence.
Figure 6. Polarized ATR-FTIR absorption spectra recorded from Langmuir-Blodgett monomolecular films of AmB deposited at two sides of a Ge crystal. The films were deposited from the monomolecular layer of AmB at the air-water interface at the surface pressure 10 mN/m. The spectrum recorded with parallel polarisation of the electric vector of radiation with respect to the plane of incidence is plotted with the solid line and perpendicular polarisation with the dotted line. The inset drawing presents orientation of the AmB molecule deposited at the plate of crystal, that corresponds to the tilt angle of the molecular axis with respect to the axis normal to the plane of the crystal (z axis) θ = 90°. The assignment of the main spectral bands and the integration limits of the spectral band corresponding to the C = C stretching vibrations are shown.
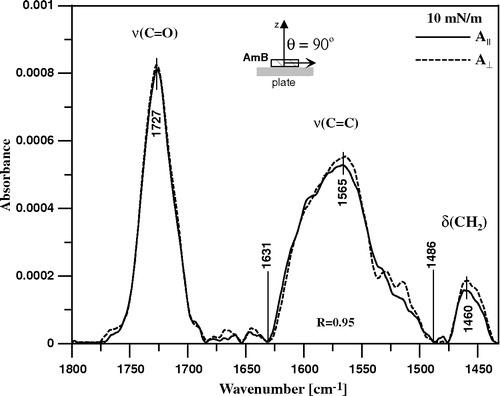
The spectral window is shown, covering the region characteristic of the three principal vibrations of the AmB molecule: the C = O group stretching vibration (with maximum at 1727 cm−1), the CH2 scissoring vibrations (the band centered at 1460 cm−1) and the C = C stretching vibration, represented by a relatively broad band (between 1486 and 1631 cm−1) with the maximum at 1565 cm−1. Such a broad band is an indication of an aggregation of AmB molecules in the monolayer, stabilized by van der Waals interactions between the neighbouring polyene chains. The analysis of the electronic absorption spectra of AmB in the compressed monomolecular layers has led to the same conclusion Citation[7]. The monolayer has been deposited at the surface pressure 10 mN/m in order to assure horizontal orientation of the AmB molecules at the support, as discussed above (see also discussion in Citation[7]). Assuming that the long molecular axis of AmB is oriented along the conjugated double bond system one arrives to a value of θ = 90° (see Equation 3). The dichroic ratio, determined for the band corresponding to the C = C stretching vibrations (R = 0.95, see ), let calculate the angle between the molecular director and the transition moment of this particular vibration α = 24° (see Equation 4). The linear dichroism FTIR studies show that the direction of the transition moment of the C = C stretching with respect to the long axis of polyene depends on the extent of vibrational mixing and the particular modes which contribute to the absorption band Citation[25].
presents the FTIR spectrum of single DPPC monolayer deposited to the ATR crystal superimposed to the spectrum of the DPPC film deposited after the injection of AmB into the subphase and time required for equilibration of the system. A very distinct band with the maximum at 1562 cm−1 in the latter spectrum, assigned to the C = C vibrational stretching of AmB demonstrates unequivocally binding of the drug to the DPPC membranes, owing to the fact that the lipid does not contain any C = C bonds. Both the carbonyl groups of DPPC and AmB give rise to the band centered at 1738 cm−1 and interestingly, the intensity of this band decreases after binding of AmB to the membrane. Instead, the new band appears at 1645 cm−1, in the spectral region of the carbonyl group vibrations. It is very difficult to attribute this band to a single component of the membrane (neither DPPC nor AmB) without falling into speculations. On the other hand, this band is localized in the spectral region shifted towards lower wavenumbers, with respect to the principal band at 1738 cm−1, and therefore indicates involvement of carbonyl groups in binding of AmB to the lipid membrane, presumably by a hydrogen bonding network. Such an observation points additionally to the polar headgroup region of the membrane as a localization place of the molecules of membrane-bound AmB.
Figure 7. ATR-FTIR absorption spectra recorded from the Langmuir-Blodgett monomolecular films deposited at two sides of a Ge crystal from lipid monomolecular layer of DPPC formed at the air-water interface, compressed and stabilized at the surface pressure 22 mN/m (dashed line) and the DPPC film deposited to the crystal after the injection of 10 µl of water solution of AmB (pH 12) into 12 ml of the subphase (solid line). Final concentration of AmB in the subphase 0.9 µM. The central part of the spectra is also presented on the 10-fold expanded absorption scale.
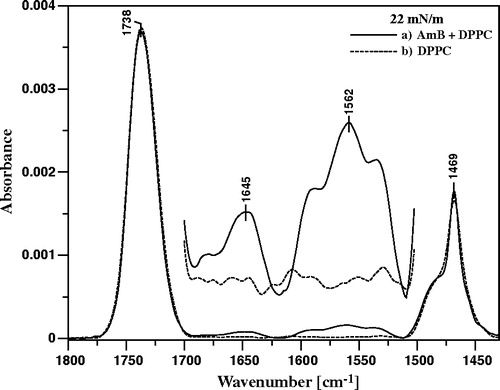
presents the polarized IR absorption spectra of monomolecular layers, deposited on the ATR support, composed of DPPC and DPPC with sterols (cholesterol-B or ergosterol-C) deposited from the same monolayers formed at the air-water interface after injection of AmB into the subphase and time required for equilibration of the system. The band corresponding to the C = C vibrational stretching (indicated in ), attributed to the membrane-bound AmB, was integrated and the dichroic ratio R was calculated according to Equation 2 (presented in ).
Figure 8. ATR-FTIR absorption spectra recorded from the AmB-containing Langmuir-Blodgett monomolecular films deposited at two sides of a Ge crystal from the lipid monomolecular layers at the air-water interface compressed to the surface pressure 22 mN/m composed of: (A) pure DPPC, (B) DPPC + Cholesterol 1:1 (by mole) and (C) DPPC + Ergosterol 1:1 (by mole). The electric vector of the IR radiation was polarized parallel (dashed line) and perpendicular (solid line) with respect to the plain of incidence. The Langmuir-Blodgett monomolecular films deposited from the lipid monolayers 20 min after the injection of 10 µl of the water solution of AmB (pH 12) into 12 ml of the subphase. Final concentration of AmB in the subphase was 0.9 µM.
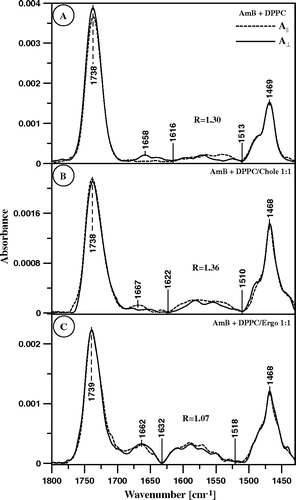
Table I. Orientation angle (θ) of the long molecular axis of AmB bound to monomolecular layers formed with DPPC, DPPC and cholesterol or DPPC and ergosterol, with respect to the axis normal to the plane of the film, determined on the basis of linear dichroism measurements (see ) and Equation 4.
Generally, the orientation angles of AmB with respect to the normal to the membrane are in the range 50°–67°. This is an average orientation angle that corresponds unequivocally to the order parameter S (Equation 3, ). On the other hand, the calculated orientation angle of AmB with respect to the normal to the plane of the layer, in the lipid environment (50°, ) corresponds to the particular orientation that is highly unfavourable for energetic reasons, owing to the interactions with lipids (highly tilted AmB molecule). This average orientation angle is close to the ‘magic angle’ (54.7°) and in the worst case it would represent the totally homogenic angular distribution of the AmB molecules with respect to the axis normal to the film. Such a distribution seems highly improbable both due to the organization and deposition of the film to the Ge support and due to the presence of lipids. Another possibility, discussed also elsewhere Citation[15], Citation[26], is that, owing to the chemical structure of AmB, the molecules adopt two distinct orientations with respect to the membrane: horizontal (θ1=90°) and vertical (θ2=0°). The rationale of such an approach is that the average orientation angle θ corresponds to two orthogonal distributions: parallel with respect to the membrane (θ1=90°, fraction k1) and perpendicular to the membrane (θ2=90°, fraction k2). Such a distribution is represented by the following equation:8 where k1+k2=1
This equation allows the calculation of the fractions of both forms (k1=41% and k2=59% in the case of θ = 50°). Two distributional fractions, parallel and perpendicular with respect to the plane of the membrane, has been also determined in the precise linear dichroism studies of AmB incorporated to the lipid multibilayers Citation[24] but under such experimental conditions the vertical fraction was much more populated than the horizontal one. The presence of cholesterol in the lipid multilayers resulted in narrower orientation of AmB Citation[24]. Also in the case of the present study, orientation of AmB bound to the cholesterol-containing DPPC monolayer was slightly different θ = 47° which corresponds to the following distribution: 53% horizontal and 47% vertical. Interestingly, essentially different distribution has been found in the case of AmB incorporated to the membrane formed with DPPC and ergosterol. The orientation angle θ = 67°, determined in this case, corresponds to 15% molecules oriented vertically and as much as 85% oriented horizontally. The distribution of AmB in the lipid membrane among two orthogonal pools seems to be very likely, taking into consideration molecular structure of AmB (). Vertically-oriented AmB would penetrate the hydrophobic zone of the membrane in the aggregated form Citation[7]. In the case of horizontally-oriented molecules, most probable localization is the membrane polar zone or the hydrophobic-hydrophilic interface.
The very distinct effect of ergosterol on orientation of AmB bound to the DPPC membranes corresponds to the very strong effect of this particular sterol on binding of the membranes, as demonstrated by the surface pressure measurements, presented in this work and in the literature Citation[17]. Such a correspondence suggests that the membrane-bound ergosterol facilitates binding of AmB to lipid membranes and that the drug adopts horizontal orientation in the membrane polar headgroup region. Such orientation of AmB would also explain strong effect of the drug on the membrane surface pressure. Interference with the compact membrane structure, in particular in the headgroup region, is a factor that can be expected to influence considerably a transmembrane transport of ions and small molecules.
Binding of AmB to the DPPC membrane does not influence significantly the hydrophobic core of the membrane, as can be seen from the analysis of the vibrational spectra of deposited monolayers, in the region characteristic of the symmetric and antisymmetric stretching vibrations of the CH3 and CH2 groups of alkyl chains ().
Figure 9. ATR-FTIR absorption spectra recorded from the Langmuir-Blodgett monomolecular films deposited at two sides of a Ge crystal from the lipid monomolecular layer of DPPC formed at the air-water interface, compressed to the surface pressure 22 mN/m (dashed line) and the DPPC film deposited to the crystal after the injection of 10 µl of water solution of AmB (pH 12) into 12 ml of the subphase (solid line). Final concentration of AmB in the subphase 0.9 µM. The spectral region presented corresponds to the stretching vibrations of CH3 groups (νs 2881 cm−1, νas 2959 cm−1) and CH2 groups (νs 2850 cm−1, νas 2919 cm−1).
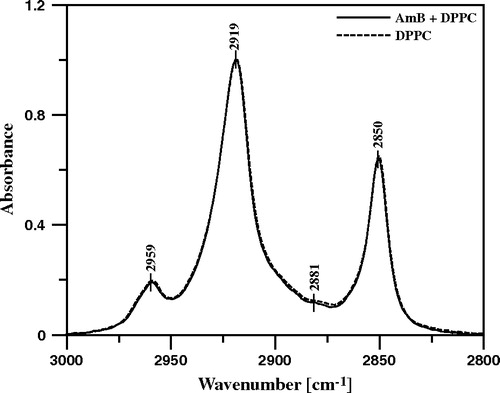
presents the results of the IR linear dichroism determinations, based on this spectral region (CH2 stretching vibrations). The relatively small orientation angle θ, determined as 27° in the case of the membrane formed with pure DPPC, corresponds to the order parameter 0.68 (see Equation 3) and reflects rather restricted segmental motional freedom of lipids in the hydrophobic core of the membrane. The order parameter is even lower under the presence of both cholesterol and ergosterol in the membrane. The decreased molecular packing within the membrane, demonstrated by this particular result, corresponds to the decreased penetration barrier to AmB, under the presence of cholesterol and ergosterol, as demonstrated by means of the surface pressure experiments. Very interestingly, the binding of AmB to the membrane restores the order parameter of alkyl chains observed in the membrane without any sterols (). Such a result shows directly abolition of the membrane modifying effect of sterols, brought about by incorporation of AmB.
Table II. Mean orientation angle (θ) of the molecular axis along the CH2 alkyl chains of DPPC in monomolecular layers formed with DPPC, DPPC and cholesterol or DPPC and ergosterol, with respect to the axis normal to the plane of the film, determined on the basis of linear dichroism measurements (Equation 3). The Table also presents the orientation angle in the case of the lipid films deposited from monolayers after injection of 0.9 M AmB into the subphase and deposited after time required for equilibration of the system. The angle α has been taken arbitrary as 90o according to the literature data Citation[25].
Conclusion
The results of spectroscopic measurements and monomolecular layer technique experiments show that:
AmB binds to the lipid membranes from aqueous phase and it is localized mostly in the polar headgroup region.
Sterols, in particular ergosterol, facilitate penetration of AmB into the membranes.
The mean orientation angle of the membrane-bound AmB corresponds to the distribution of the antibiotic among two pools: one horizontal and one vertical with respect to the plane of the membrane.
The presence of ergosterol in the lipid membrane facilitates horizontal orientation of AmB.
The results of the measurements indicate that the mode of action of the AmB consists in disruption of the polar headgroup region of biomembranes, brought about by the AmB molecules bound horizontally with respect to the plane of the membrane.
This paper was first published online on prEview on 23 September 2005.
This research was financed by the Committee for Scientific Research of Poland in the years 2004–2007 within the research project 2P05F04327. M. Gagos thanks the EMBO foundation for the short-term fellowship to carry out research in the ITC-CNR Institute of Biophysics, Unit at Trento, Via Sommarive 18, 38050 Povo (Trento) Italy (ASTF 118.00-04).
References
- Hartsel SC, Hatch C, Ayenew W. How does amphotericin B work?: Studies on model membrane systems. J Liposome Res 1993; 3: 377–408
- Hartsel S, Bolard J. Amphotericin B: new life for an old drug. Trends Pharmacol Sci 1996; 17: 445–449
- Sternberg TM, Wright ET, Oura M. A new antifungal antibiotic, amphotericin B. Antibiotics Ann 1956; 3: 566–573
- De Kruijff B, Demel RA. Polyene antibiotic-sterol interaction in membranes of Acholeplasma Laidlawii cells and lecithin liposomes. III. Molecular structure of the polyene antibiotic-cholesterol complex. Biochim Biophys Acta 1974; 339: 57–70
- De Kruijff B, Gerritsen WJ, Oerlemans A, Demel RA, van Deenen LL. Polyene antibiotic-sterol interactions in membranes of Acholeplasma laidlawii cells and lecithin liposomes. I. Specificity of the membrane permeability changes induced by the polyene antibiotics. Biochim Biophys Acta 1974; 339: 30–43
- Baginski M, Resat H, Borowski E. Comparative molecular dynamics simulations of amphotericin B-cholesterol/ergosterol membrane channels. Biochim Biophys Acta 2002; 1567: 63–78
- Gruszecki WI, Gagos M, Kernen P. Polyene antibiotic amphotericin B in monomolecular layers: spectrophotometric and scanning force microscopic analysis. FEBS Lett 2002; 524: 92–96
- Gruszecki WI, Gagos M, Herec M, Kernen P. Organization of antibiotic amphotericin B in model lipid membranes. A mini review. Cell Mol Biol Lett 2003; 8: 161–170
- Khutorsky V. Ion coordination in the amphotericin B channel. Biophys J 1996; 71: 2984–2995
- Baginski M, Resat H, McCammon JA. Molecular properties of amphotericin B membrane channel: A molecular dynamics simulation. Mol Pharmacol 1997; 52: 560–570
- Cotero BV, Rebolledo-Antunez S, Ortega-Blake I. On the role of sterol in the formation of the amphotericin B channel. Biochim Biophys Acta 1998; 1375: 43–51
- Wojtowicz K, Gruszecki WI, Walicka M, Barwicz J. Effect of amphotericin B on dipalmitoylphosphatidylcholine membranes: Calorimetry, ultrasound absorption and monolayer technique studies. Biochim Biophys Acta 1998; 1373: 220–226
- Gagos M, Koper R, Gruszecki WI. Spectrophotometric analysis of organisation of dipalmitoylphosphatylcholine bilayers containing the polyene antibiotic amphotericin B. Biochim Biophys Acta 2001; 1511: 90–98
- Sternal K, Czub J, Baginski M. Molecular aspects of the interaction between amphotericin B and a phospholipid bilayer: Molecular dynamics studies. J Mol Model 2004; 10: 223–232
- Gruszecki WI, Gagos M, Herec M. Dimers of polyene antibiotic amphotericin B detected by means of fluorescence spectroscopy: molecular organization in solution and in lipid membranes. J Photochem Photobiol B: Biol 2003; 69: 49–57
- Dynarowicz-Latka P, Seoane R, Minones J, Jr, Velo M, Minones J. Study of penetration of amphotericin B into cholesterol or ergosterol containing dipalmitoyl phosphatidylcholine Langmuir monolayers. Colloids and Surfaces B: Biointerfaces 2002; 27: 249–263
- Barwicz J, Tancrede P. The effect of aggregation state of amphotericin-B on its interactions with cholesterol- or ergosterol-containing phosphatidylcholine monolayers. Chem Phys Lipids 1997; 85: 145–155
- Tatulian SA, Jones LR, Reddy LG, Stokes DL, Tamm LK. Secondary structure and orientation of phospholamban reconstituted in supported bilayers from polarized attenuated total reflection FTIR spectroscopy. Biochemistry 1995; 34: 4448–4456
- Harrick NJ. Internal Reflection Spectroscopy. Harrick Scientific Corporation, Ossining, NY 1979
- Menestrina G. Use of Fourier-transformed infrared spectroscopy (FTIR) for secondary structure determination of staphylococcal pore-forming toxins. Bacterial toxins, methods and protocols, O Holst. Humana Press, Totowa, NJ 2000; 115–132
- Binder H. The molecular architecture of lipid membranes – new insights from hydration-tuning infrared linear dichroism spectroscopy. Appl Spectr Rev 2003; 38: 15–69
- Minones J, Jr, Carrera C, Dynarowicz-Latka P, Minones J, Conde O, Seoane R, Rodriguez Patino JM. Orientational changes of amphotericin B in Langmuir monolayers observed by Brewster angle microscopy. Langmuir 2001; 17: 1477–1482
- Barwicz J, Gruszecki WI, Gruda I. Spontaneous organization of amphotericin B in aqueous medium. J Colloid Interf Sci 1993; 158: 71–76
- Lopes S, Castanho ARB. Revealing the orientation of nystatin and amphotericin B in lipidic multilayers by UV-Vis linear dichroism. J Phys Chem B 2002; 106: 7278–7282
- Binder H, Gutberlet T, Anikin A. Biaxial ordering of terminal diene groups in lipid membranes: an infrared linear dichroism. J Molec Struct 1999; 510: 113–129
- Lopes S, Castanho MARB. Revealing the orientation of nystatin and amphotericin B in lipidic multilayers by UV-Vis linear dichroism. J Phys Chem B 2002; 106: 7278–7282