Abstract
It has been shown that a preinjection of diC14-amidine cationic liposomes decreased TNF-α secretion induced by lipoplexes intravenous injection. We showed here that free cationic liposomes inhibit CpG sequences- or lipopolysaccharides- induced TNF-α secretion by macrophages. Surprisingly, this effect was strictly dependent on serum. Free cationic liposomes alone did not reveal any anti-inflammatory activity. Low-density lipoproteins and triglyceride-rich lipoproteins were identified as the serum components that confer to the liposomes an anti-inflammatory activity. Lipid fractions of these lipoproteins were able to reproduce the effect of the total lipoproteins and could inhibit, in association with diC14-amidine liposomes, the CpG-induced TNF-α secretion. Serum components confer to cationic liposomes new properties that can be used to modulate the inflammatory response directed against CpG sequences and lipopolysaccharides.
Introduction
Cationic lipids have been widely used as vectors to deliver DNA into mammalian cells, both in vitro and in vivo, and may represent a safe alternative to viral vectors for gene therapy applications Citation[1–5]. Intravenous administration of cationic lipid-DNA lipoplexes results in efficient reporter gene expression in several organs, including lung, liver, heart, kidney and spleen Citation[2–4]. It has been reported that cationic lipid-DNA lipoplexes injected intravenously induce an acute inflammatory response characterized by cytokine release in the serum that limits the use of these vectors Citation[6], Citation[7]. This inflammatory response was attributed to the presence of unmethylated CpG motifs in the plasmid DNA since depletion or methylation of these CpG sequences resulted in reduced cytokine levels Citation[7–9]. Intravenous injection of cationic liposomes or DNA alone failed to induce an inflammatory response suggesting that complexation of DNA with cationic liposomes strongly potentiated the immunostimulatory effect of the CpG sequences Citation[7], Citation[10], Citation[11]. It has been recently reported that preinjection of free liposomes made of the cationic lipid diC14-amidine decreased the secretion of cytokine induced by lipoplexes into mouse serum, thereby improving the transfection efficiency Citation[10]. This result suggested that diC14-amidine liposomes modulated the inflammatory response induced by diC14-amidine/DNA lipoplexes. Several studies provided evidence that liver and spleen macrophages are among the main target cells of cationic liposomes injected intravenously and are the main source of secreted proinflammatory cytokines after lipoplexes injection Citation[5], Citation[12–14]. In the present work, we provide evidence that the inhibitory effect of diC14-amidine liposomes on TNF-α secretion by macrophage required the presence of serum. Lipids of lipoproteins were identified as the serum components that confer the anti-inflammatory activity to the liposomes.
Materials and methods
CpG and LPS
The CpG oligodeoxynucleotides (CpG ODN) used in this study was 1826 (5′ tcc atg acg ttc ctg acg tt 3′) (InvivoGen), which was shown previously to have potent immunostimulatory effects on the murine immune system Citation[15]. Lipopolysaccharides from E.Coli serotype 011.B4 (LPS) were purshased from Sigma.
Liposomes preparation
DiC14-amidine (3-tetradecylamino-N-tert-butyl-N′-tetradecyl-propionamidine) was synthesized as described Citation[16]. Cationic liposomes were prepared in HBS-20 Buffer (20 mM HEPES, 150 mM NaCl, pH 7.3) and extruded 7 times through 0.4 µm polycarbonate filter at 55°C as described previously Citation[11].
Cell culture and incubation with diC14-amidine liposomes
RAW 264.7 macrophage cell line was cultured in DMEM (Invitrogen) supplemented by 5% FBS (Biowhittaker), 1 mM sodium pyruvate, 1 mM glutamine and antibiotics (Invitrogen) (Complete Medium). RAW 264.7 cells were plated on 24-well plates at a density of 5.105 cells/well for 24 h before experiments. DiC14-amidine/serum was prepared by adding mouse serum (Serotec) to diC14-amidine liposomes (125 µg/ml diC14-amidine in DMEM with 50% serum) and was incubated for 15 min at 37°C, before addition of DMEM to obtain the final concentration of diC14-amidine (generally 50 µg/ml). Final concentration in serum was adjusted to obtain 20% mouse serum. After incubation with diC14-amidine/serum, cells were washed 2 times with DMEM and stimulated with CpG ODN (0.5 µg/ml) or LPS (100 ng/ml) for 4 h. Culture supernatants were collected and stored at −20°C before TNF-α was quantified using ELISA assay (R&D Systems).
Isolation of serum lipoproteins and incubation with diC14-amidine liposomes
Lipoproteins were isolated from mouse serum (Serotec) or human serum (from heathly volunteer) by KBr density gradient ultracentrifugation as described Citation[17]. Briefly, a discontinuous KBr density gradient was prepared in ultracentrifugation tubes using KBr solutions as follow (from bottom to top): 2.5 ml of d = 1.24 g/ml, 7.5 ml of d = 1.21 g/ml containing serum, 10 ml of d = 1.125 g/ml, 10 ml of d = 1.063 g/ml, 2.5 ml of d = 1.019 g/ml, 2.5 ml of d = 1.006 g/ml and 2.5 ml of pure water. Samples were then centrifuged at 105,000×g for 16 h at 4°C. The fractions corresponding to triglycerides-rich lipoproteins (TRL, d < 1.019 g/ml), low density lipoproteins (LDL, d = 1.028–1.050 g/ml), high-density lipoproteins (HDL, d = 1.066–1.153 g/ml) and lipoprotein-depleted serum (d > 1.21 g/ml) were sampled. KBr was removed from lipoproteins using gel filtration chromatography on PD-10 columns (Amersham Biosciences) and volumes of lipoprotein fractions were adjusted in HBS-20 buffer to obtain the serum concentration.
DiC14-amidine/lipoprotein complex was prepared by adding lipoproteins to an equal volume of diC14-amidine liposomes (125 µg/ml in DMEM) and was incubated for 30 min before dilution by DMEM to obtain the final concentration of diC14-amidine (50 µg/ml). Final concentration in serum lipoproteins was adjusted to obtain a concentration equivalent as 20% mouse serum. Cells were plated and treated as described above.
Lipid extraction from lipoproteins
The extraction procedure was described in Citation[18]. Briefly, 250 µl of human serum lipoprotein solution were added to 3 ml of methanol, vortexed before addition of 7 ml of diethyl ether. The mixture was allowed to stand on ice for 10 min then centrifuged at 100×g for 5 min. The supernatant, containing lipids, was collected. 10 ml of diethyl ether was added to the pellet, incubated 10 min on ice, then centrifuged at 100×g for 10 min. The supernatant was collected. All supernatants were washed once by adding 3 ml of methanol, and centrifugated as described before. The solvents were evaporated under a nitrogen stream and the resulting lipidic film was hydrated with 250 µl of HBS-20 buffer.
Statistics
Unpaired two-tails t-Test was used for statistical analysis.
Results
DiC14-amidine liposomes inhibit CpG-induced TNF-α secretion in cultured murine macrophages but this effect requires the presence of serum
RAW 264.7 cells were pretreated with culture medium or with diC14-amidine liposomes in the presence or absence of serum before stimulation with CpG oligonucleotides (CpG ODN). TNF-α level was quantified in the culture supernatants. Treatment of macrophages with CpG ODN significantly increased TNF-α secretion in the culture supernatant. Pretreatment of the cells with diC14-amidine liposomes alone did not inhibit the secretion of TNF-α induced by CpG DNA (see A). On the contrary, pretreatment of the cells by cationic liposomes that have been incubated in the presence of mouse serum resulted in a significant decrease in the release of TNF-α triggered by CpG ODN. This result indicated that the interaction between diC14-amidine liposomes and one or more components of the serum is a prerequisite to the inhibition of TNF-α secretion induced by CpG ODN. For further experiments, the diC14-amidine liposomes incubated with serum will be referred to as diC14-amidine/serum.
Figure 1. Treatment of RAW 264.7 cells with diC14-amidine liposomes in the presence of serum inhibits the CpG-induced TNF-α secretion. (A) 5.105 RAW 264.7 cells were pretreated for 1 h with medium or diC14-amidine liposomes 20 µg/ml in DMEM, with or w/o mouse serum (20%), then washed two times and stimulated with CpG oligonucleotides (0.5 µg/ml) for 4 h. TNF-α level in the culture supernatant was quantified by ELISA. Data represent means±SD (n=3). **p<0.01 (versus CpG ODN w/o pretreatment). (B) 5.105 RAW 264.7 cells were pretreated for 1 h with medium or diC14-amidine liposomes at different doses with 20% serum, then washed two times and stimulated with CpG oligonucleotides (0.5 µg/ml) for 4 h. TNF-α level in the culture supernatant was then quantified by ELISA. Data represent means±SD (n=3). *p<0.05 (versus CpG ODN w/o pretreatment).
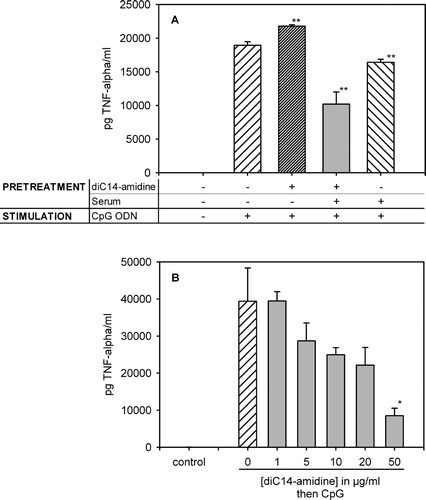
Inhibition of TNF-α secretion by diC14-amidine/serum was dependent on the cationic lipid concentration, and maximum inhibition was observed at 50 µg/ml (see B). Trypan blue exclusion assay showed that viability of cells treated by diC14-amidine/serum, at these concentrations, was 90–95%, which was comparable to that of non-pretreated cells (data not shown). This suggested that the inhibitory effect of diC14-amidine/serum was not due to a possible toxicity of the liposomes.
The choice of a one-hour period of preincubation of cells with diC14-amidine/serum was based on the observation that this interval between free liposome preinjection and lipoplex injection allowed an optimal TNF-α inhibition in vivo Citation[10]. In order to verify whether this time interval was required in vitro, macrophages were pretreated with diC14-amidine/serum for various periods of time. Thereafter they were treated with CpG ODN and TNF-α secretion was measured in the culture supernatant (see ). Inhibition was time-dependent and was optimal for an incubation period of 60 min.
Figure 2. Inhibition of TNF-α secretion by diC14-amidine/serum is time dependent. 5.105 RAW 264.7 cells were incubated for indicated periods with diC14-amidine liposomes (50 µg/ml) and mouse serum (or medium for control), then washed two times and stimulated with CpG oligonucleotides (0.5 µg/ml) for 4 h. TNF-α level in the culture supernatant was quantified by ELISA. Data represent means±SD (n=3). **p<0.01 (versus CpG ODN w/o pretreatment).
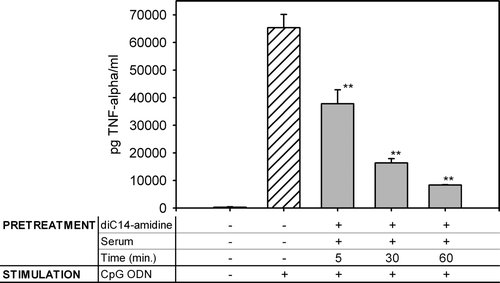
DiC14-amidine/serum inhibits the LPS-induced TNF-α secretion by macrophages
It was important to verify whether the inhibitory effect of diC14-amidine/serum was specific to CpG sequences or could be extended to other proinflammatory-stimuli like lipopolysaccharides (LPS). In contrast to CpG, which binds to Toll-like receptor 9 (TLR9) likely inside endosomes, LPS is recognized by TLR4, which is located at the cell surface. Both receptors share common downstream signaling pathways Citation[19], Citation[20]. Macrophages were pretreated 1 h with diC14-amidine/serum or medium then stimulated with LPS and TNF-α was quantified in the supernatant (see ). DiC14-amidine/serum at 50 µg/ml also inhibited the inflammatory response induced by LPS although the inhibitory effect was less pronounced as compared to that observed when CpG was used. This may indicate that the diC14-amidine/serum exert its inhibitory effect on signaling steps common to CpG and LPS.
Figure 3. DiC14-amidine/serum inhibits LPS-induced TNF secretion. 5.105 RAW 264.7 cells were incubated for 1 h with medium or diC14-amidine liposomes (50 µg/ml) and mouse serum, then washed two times and stimulated with lipopolysaccharides (100 ng/ml) for 4 h. TNF-α level in the culture supernatant was quantified by ELISA. Data represent means±SD (n=3). **p<0.01 (versus LPS w/o pretreatment).
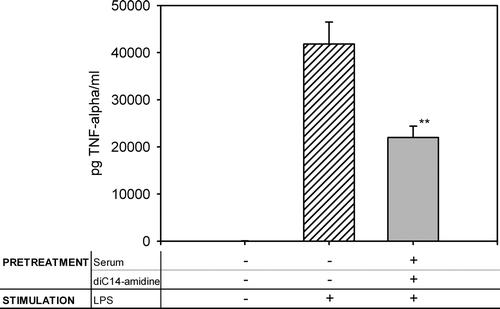
Low-density (LDL) and triglycerides-rich lipoproteins (TRL) are the main component responsible for diC14-amidine/serum inhibitory effect on TNF-α secretion
The data presented above showed that incubation with serum was essential for the anti-inflammatory property of diC14-amidine liposomes. In order to identify which component of serum conferred to diC14-amidine this new property, mouse serum was fractionated on a KBr density gradient. DiC14-amidine liposomes were incubated 30 min with mouse serum, lipoprotein-depleted serum, HDL, LDL or TRL, then incubated 1 h with RAW 264.7 cells. Cells were then washed and stimulated for 4 hours with CpG ODN, and TNF-α was quantified in culture supernatant. Preincubation of diC14-amidine liposomes with low-density lipoproteins (LDL) or triglycerides-rich lipoproteins (TRL) led to an inhibition of TNF-α secretion (see ). In contrast, HDL or lipoprotein-depleted serum, in association with diC14-amidine liposomes, were unable to inhibit TNF-α secretion.
Figure 4. Identification of the serum components involved in diC14-amidine mediated inhibition of TNF-α secretion. 5.105 RAW 264.7 cells were incubated for 1 h with medium or with diC14-amidine (50 µg/ml) and mouse serum, LDL, TRL, HDL or lipoprotein-depleted serum, then washed two times and stimulated with CpG oligonucleotides (0.5 µg/ml) for 4 h. The final lipoprotein concentrations were equivalent to concentration existing in 20% serum. TNF-α level in the culture supernatant was quantified by ELISA. Data represent means±SD (n=3). *p<0.05, **p<0.01 (versus CpG ODN w/o pretreatment).
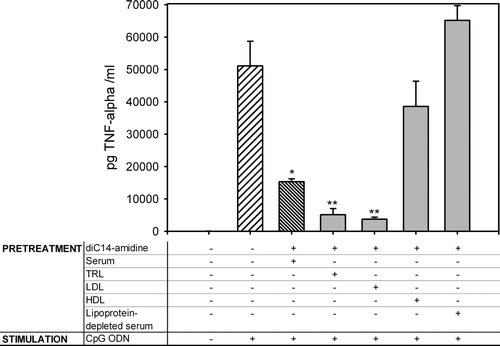
Lipids of lipoproteins, in combination with diC14-amidine liposomes, are able to inhibit TNF-α secretion induced by CpG ODN
Lipids were extracted from lipoprotein fractions as described under materials and methods. Silver stained electrophoresis gel did not reveal any protein associated to the lipid fractions suggesting that the effect was not due to the residual proteins (data not shown). DiC14-amidine liposomes were incubated 30 min with total or lipid fraction of HDL, LDL or TRL, then incubated 1 h with RAW 264.7 cells. After 4 h of stimulation with CpG ODN, TNF-α was quantified in culture supernatant. All lipid fractions were able to inhibit TNF-α secretion, in association with diC14-amidine (see ). The corresponding lipoproteins particles presented the same inhibitory profile as in See , i.e., only LDL and TRL, but not HDL, were able to inhibit TNF-α secretion.
Figure 5. Lipoprotein lipids and diC14-amidine liposomes are able to inhibit TNF-a secretion. 5.105 RAW 264.7 cells were pretreated for 1 hour with medium or with diC14-amidine (50 µg/ml) incubated first with total or lipidic fractions of LDL, TRL and HDL, then washed two times and stimulated with CpG oligonucleotides (0.5 µg/ml) for 4 h. The final lipoprotein or lipid concentrations were equivalent to concentration existing in 20% serum. TNF-α level in the culture supernatant was quantified by ELISA. Data represent means±SD (n=3). *p<0.05, **p<0.01 (versus CpG ODN w/o pretreatment).
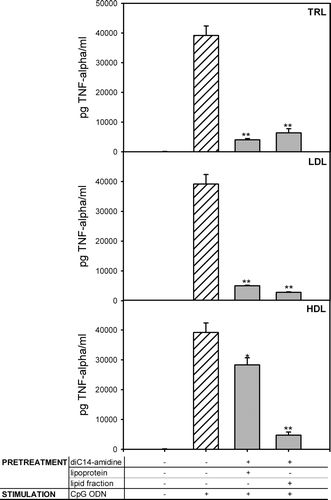
Discussion
Lipoplexes injected intravenously induce a strong inflammatory response characterized by secretion of proinflammatory cytokines including TNF-α in the serum Citation[6], Citation[7], Citation[10]. Free IV injected-diC14-amidine liposomes have been shown to partially inhibit this inflammatory response Citation[10]. In this work, preinjection of up to 200 µg of free diC14-amidine liposomes into mice did not change viability and did not cause any major physiological changes.
In the present work, we show in vitro that free cationic liposomes alone are unable to inhibit TNF-α secretion by RAW 264.7 cells after stimulation with CpG ODN. Free liposomes acquire their anti-inflammatory activity when preincubated with serum. Low density lipoproteins and triglyceride-rich lipoproteins were identified as the serum component that confers the anti-inflammatory activity to the cationic liposomes. Further separation indicated that lipids of lipoproteins were able, in the absence of any protein, to reproduce the anti-inflammatory effect.
While the exact mechanism of the inhibition of TNF-α secretion is still unclear, several data suggest that diC14-amidine/lipoprotein mixture act on the cell signaling pathways that leads to macrophage activation. Indeed, both CpG and LPS-triggered TNF-α secretion by macrophages were inhibited by diC14-amidine/serum complex (see and ). CpG and LPS interact with two different receptors, i.e. TLR9 and TLR4, which are localized at the endosomal and plasma membranes respectively Citation[19], Citation[20]. This suggests that diC14-amidine/lipoprotein lipids complex may act on a process that leads to cell activation at a stage downstream to the stimulus/receptor interaction.
Our previous results revealed that a one hour period of time between preinjection of diC14-amidine liposomes and lipoplexes was required to optimize the inhibitory effect of diC14-amidine on TNF-α secretion in vivo Citation[10]. In this report, TNF-α secretion in vitro was drastically reduced when diC14-amidine liposomes/serum were incubated with macrophages one hour before the stimulation (see ). This suggests that the capture of liposomes by cells in vivo and in vitro has a comparable kinetics and that access of liposomes to the immune cells through the blood stream in vivo is not the restrictive step. We could hypothesize that the limiting step is the entry and/or subcellular localization of the diC14-amidine/serum in the cell.
We could ask if other cationic lipids in association with lipids of lipoproteins could also inhibit TNF-α secretion, suggesting that the positive charge is sufficient to obtain this anti-inflammatory effect. Our preliminary results show that DOTAP liposomes, preincubated with lipoproteins, have no significant effect on CpG-induced TNF-α secretion, at the same molar concentration that diC14-amidine liposomes (data not shown). As it has been shown, lipid mixing between lipids of lipoproteins and diC14-amidine liposomes occurred within 30 min of incubation at 37°C whereas lipid mixing between lipoproteins and DOTAP liposomes did not occur Citation[21]. It is highly probable that this lipid mixing is essential to the inhibitory activity since DOTAP liposomes were inefficient to inhibit TNF-α secretion. The fact that lipids alone, in association with diC14-amidine liposomes, were able to inhibit TNF-α secretion induced by CpG sequences supports this possibility.
The fact that HDL did not inhibit TNF-α secretion but lipids from HDL did (see ), suggests that the presence of proteins decreases the efficiency of lipids in the regulation of inflammatory process. We could expect a different cellular uptake of lipids when proteins are present in the particule. To support this conclusion, several works indicate that apolipoproteins modulate the selective lipid uptake from lipoprotein particles by monocytes after interaction with the scavenger receptor BI Citation[22–25]. In our case, this step is bypassed and the lipid extract is active on cells after complexation with cationic liposomes.
A trivial explanation of the TNF-α level decrease would be that lipoprotein could strongly enhance the uptake of cationic lipids, thus inhibiting secretion due to their possible cytotoxicity. Cell uptake experiments (unpublished results, Lonez C., Legat A., Vandenbranden M., Ruysschaert J.-M.) have shown that there is clearly no correlation between the amount taken up by macrophages and the inhibition of TNF-α secretion.
Based on these results we propose the following mechanism to account for the previously observed cationic liposome-mediated inhibition of inflammation in vivo Citation[10]. Intravenously preinjected free cationic liposomes interact rapidly with serum lipoproteins and lipid mixing occurs between the two components Citation[21], Citation[26]. It can be suggested that the presence of cationic lipids in the resulting complexes facilitates the capture of lipoprotein-derived lipids by macrophages, allowing them to interact with key regulatory components of the cell.
The lipid composition is characteristic of the different types of lipoproteins and can be correlated with different pathological situations Citation[27], Citation[28]. The possibility that some lipid components of lipoproteins may exert a specific effect on the signalling cascade leading to inflammation remains obscure, despite some indications that are suggestive of such a role Citation[29–32]. These lipids have been reported as anti-inflammatory components without the presence of any adjuvant but at high concentrations. In our case, the presence of the cationic lipid is essential for the inhibition of TNF-α but diC14-amidine liposomes could promote a more efficient or different uptake of these lipids, increasing their effect at lower concentration.
In summary, we provided evidence that interaction of free cationic liposomes with lipids of lipoproteins inhibit the TNF-α secretion induced by cationic lipids/DNA lipoplexes. Association between free cationic liposomes and lipids of lipoproteins, generates a complex that, upon internalization into macrophages, modulates the capacity of macrophages to respond to inflammatory stimuli such as CpG or LPS.
Caroline Lonez is a FRIA fellow (Fonds de la Recherche Scientifique pour l'Industrie et l'Agriculture) and Michel Vandenbranden is Research Associate of the Belgian National Fund for Scientific Research. This work was supported by ARC (Action de Recherche Concertée).
References
- Felgner PL, Gadek TR, Holm M, Roman R, Chan HW, Wenz M, Northrop JP, Ringold GM, Danielsen M. Lipofection: a highly efficient, lipid-mediated DNA-transfection procedure. Proc Natl Acad Sci USA 1987; 84: 7413–7417
- Zhu N, Liggitt D, Liu Y, Debs R. Systemic gene expression after intravenous DNA delivery into adult mice. Science 261:209–211.
- Mahato RI, Anwer K, Tagliaferri F, Meaney C, Leonard P, Wadhwa MS, Logan M, French M, Rolland A. Biodistribution and gene expression of lipid/plasmid complexes after systemic administration. Hum Gene Ther 1998; 9: 2083–2099
- Templeton NS, Lasic DD, Frederik PM, Strey HH, Roberts DD, Pavlakis GN. Improved DNA: liposome complexes for increased systemic delivery and gene expression. Nat Biotechnol 1997; 15: 647–652
- McLean JW, Fox EA, Baluk P, Bolton PB, Haskell A, Pearlman R, Thurston G, Umemoto EY, McDonald DM. Organ-specific endothelial cell uptake of cationic liposome-DNA complexes in mice. Am J Physiol 1997; 273: H387–404
- Dow SW, Fradkin LG, Liggitt DH, Willson AP, Heath TD, Potter TA. Lipid-DNA complexes induce potent activation of innate immune responses and antitumor activity when administered intravenously. J Immunol 1999; 163: 1552–1561
- Li S, Wu SP, Whitmore M, Loeffert EJ, Wang L, Watkins SC, Pitt BR, Huang L. Effect of immune response on gene transfer to the lung via systemic administration of cationic lipidic vectors. Am J Physiol 1999; 276: L796–804
- Tan Y, Li S, Pitt BR, Huang L. The inhibitory role of CpG immunostimulatory motifs in cationic lipid vector-mediated transgene expression in vivo. Hum Gene Ther 1999; 10: 2153–2161
- Yew NS, Zhao H, Wu IH, Song A, Tousignant JD, Przybylska M, Cheng SH. Reduced inflammatory response to plasmid DNA vectors by elimination and inhibition of immunostimulatory CpG motifs. Mol Ther 2000; 1: 255–262
- Elouahabi A, Flamand V, Ozkan S, Paulart F, Vandenbranden M, Goldman M, Ruysschaert JM. Free cationic liposomes inhibit the inflammatory response to cationic lipid-DNA complex injected intravenously and enhance its transfection efficiency. Mol Ther 2003; 7: 81–88
- Tousignant JD, Zhao H, Yew NS, Cheng SH, Eastman SJ, Scheule RK. DNA sequences in cationic lipid:pDNA-mediated systemic toxicities. Hum Gene Ther 2003; 14: 203–214
- Mahato RI, Kawabata K, Nomura T, Takakura Y, Hashida M. Physicochemical and pharmacokinetic characteristics of plasmid DNA/cationic liposome complexes. J Pharm Sci 1995; 84: 1267–1271
- Osaka G, Carey K, Cuthbertson A, Godowski P, Patapoff T, Ryan A, Gadek T, Mordenti J. Pharmacokinetics, tissue distribution, and expression efficiency of plasmid [33P]DNA following intravenous administration of DNA/cationic lipid complexes in mice: use of a novel radionuclide approach. J Pharm Sci 1996; 85: 612–618
- Sakurai F, Terada T, Yasuda K, Yamashita F, Takakura Y, Hashida M. The role of tissue macrophages in the induction of proinflammatory cytokine production following intravenous injection of lipoplexes. Gene Ther 2002; 9: 1120–1126
- Kwon HJ, Lee KW, Yu SH, Han JH, Kim DS. NF-kappaB-dependent regulation of tumor necrosis factor-alpha gene expression by CpG-oligodeoxynucleotides. Biochem Biophys Res Commun 2003; 311: 129–138
- Ruysschaert JM, el Ouahabi A, Willeaume V, Huez G, Fuks R, Vandenbranden M, Di Stefano P. A novel cationic amphiphile for transfection of mammalian cells. Biochem Biophys Res Commun 1994; 203: 1622–1628
- Chapman MJ, Goldstein S, Lagrange D, Laplaud PM. A density gradient ultracentrifugal procedure for the isolation of the major lipoprotein classes from human serum. J Lipid Res 1981; 22: 339–358
- Osborne JC. Delipidation of plasma lipoproteins. Methods Enzymol 1986; 128: 213–222
- Takeda K, Akira S. Toll-like receptors in innate immunity. Int Immunol 2005; 17: 1–14
- Leifer CA, Kennedy MN, Mazzoni A, Lee C, Kruhlak MJ, Segal DM. TLR9 is localized in the endoplasmic reticulum prior to stimulation. J Immunol 2004; 173: 1179–1183
- Tandia BM, Lonez C, Vandenbranden M, Ruysschaert JM, Elouahabi A. Lipid mixing between lipoplexes and plasma lipoproteins is a major barrier for intravenous transfection mediated by cationic lipids. J Biol Chem 2005; 280: 12255–12261
- Thuahnai ST, Lund-Katz S, Williams DL, Phillips MC. Scavenger receptor class B, type I-mediated uptake of various lipids into cells. Influence of the nature of the donor particle interaction with the receptor. J Biol Chem 2001; 276: 43801–43808
- Urban S, Zieseniss S, Werder M, Hauser H, Budzinski R, Engelmann B. Scavenger receptor BI transfers major lipoprotein-associated phospholipids into the cells. J Biol Chem 2000; 275: 33409–33415
- de Beer MC, Castellani LW, Cai L, Stromberg AJ, de Beer FC, van der Westhuyzen DR. ApoA-II modulates the association of HDL with class B scavenger receptors SR-BI and CD36. J Lipid Res 2004; 45: 706–715
- Huard K, Bourgeois P, Rhainds D, Falstrault L, Cohn JS, Brissette L. Apolipoproteins C-II and C-III inhibit selective uptake of low- and high-density lipoprotein cholesteryl esters in HepG2 cells. Int J Biochem Cell Biol 2005; 37: 1308–1318
- Tandia BM, Vandenbranden M, Wattiez R, Lakhdar Z, Ruysschaert JM, Elouahabi A. Identification of human plasma proteins that bind to cationic lipid/DNA complex and analysis of their effects on transfection efficiency: implications for intravenous gene transfer. Mol Ther 2003; 8: 264–273
- Lada AT, Rudel LL. Associations of low density lipoprotein particle composition with atherogenicity. Curr Opin Lipidol 2004; 15: 19–24
- Scheffer PG, Teerlink T, Heine RJ. Clinical significance of the physicochemical properties of LDL in type 2 diabetes. Diabetologia 2005; 48: 808–816
- Lee JY, Plakidas A, Lee WH, Heikkinen A, Chanmugam P, Bray G, Hwang DH. Differential modulation of Toll-like receptors by fatty acids: preferential inhibition by n-3 polyunsaturated fatty acids. J Lipid Res 2003; 44: 479–486
- Ares MP, Stollenwerk M, Olsson A, Kallin B, Jovinge S, Nilsson J. Decreased inducibility of TNF expression in lipid-loaded macrophages. BMC Immunol 2002; 3: 13
- Calder PC. Polyunsaturated fatty acids and inflammation. Biochem Soc Trans 2005; 33: 423–427
- Hamilton TA, Ma GP, Chisolm GM. Oxidized low density lipoprotein suppresses the expression of tumor necrosis factor-alpha mRNA in stimulated murine peritoneal macrophages. J Immunol 1990; 144: 2343–2350