Abstract
The interactions of a series of histidine-containing peptides with biological model membranes have been investigated by attenuated total reflection Fourier transform infra red (ATR-FTIR) spectroscopy. Related peptides have previously been shown to exhibit antibiotic and DNA transfection activities. The 26-residue LAH4X4 peptides were designed in such a manner to form amphipathic helical structures in membrane environments. Four histidines and four variable amino acids X constitute one face of the helix whereas leucines and alanines characterize the opposite hydrophobic surface. The dichroic ratio of ATR-FTIR spectra has been used to follow the pH-dependent transition from in-plane to transmembrane alignments upon increase in pH. A theoretical model of the topological modulations is presented and the experimental transition curves analysed in order to reveal the Gibbs free energy of transition. The novel concept provides access to the free energy changes associated with the amino acids X incorporated into an extended α-helix and in the context of phospholipid bilayers. For the peptides of the series the Gibbs free energies associated with the transition from the membrane interface to the bilayer interior follow the sequence of amino acids: L < A≈I < S≈F < T≈G < V≈W < <Y.
Introduction
The identification of membrane spanning segments by prediction and computational methods remains an essential step for the development of first topological models of membrane proteins. To this purpose a number of hydrophobicity scales as well as algorithms for the identification of membrane-spanning segments have been developed Citation[1], Citation[2]. The identification of membrane-spanning polypeptide sequences requires good knowledge of the free energies (or a parameter that is correlated to that) involved in moving amino acyl residues from the aqueous phase to the hydrophobic interior of the membrane. The most commonly used ‘hydrophobicity scales’ are based on experimental data Citation[3], mixed experimental and educated guesses Citation[4], or knowledge-based statistical scales Citation[5]. Clearly due to the different chemical nature of the membrane interior and interface Citation[6] the corresponding regions of membrane-spanning helices should be treated separately. In this manner the preference of some amino acids for the membrane interface provides additional information which allows one to differentiate between hydrophobic sequences that are buried inside a globular protein and those in a transmembrane sequence Citation[7], Citation[8]. Distinguishing between interfacial and core localizations is also essential when the alignment of membrane-associated polypeptides parallel or perpendicular to the bilayer surface is of interest Citation[9]. A recent study using an in vivo test system to monitor transmembrane insertion of designed 19-residue sequences has indeed shown that very general thermodynamic principles apply when the endoplasmatic reticulum translocon is at work Citation[2]
During the prediction of protein topologies all existing possibilities, including locations exposed to aqueous buffer, within protein interiors, in the membrane core as well as at bilayer interfaces, have to be compared to each other. This requires that the energetic differences between all these sites can be evaluated. Whereas the transition from aqueous buffer to the membrane interface has been investigated using small peptides (≤6 residues) Citation[3], the energies involved in the transfer of amino acids from the interface to the membrane interior has so far not been analysed experimentally.
Here we present a novel approach which measures directly the relative transition energies of amino acids within extended α-helical peptides when transferred from the membrane interface into the interior of phospholipid bilayers. To this purpose a series of histidine-containing peptides was synthesized that follow the design of LAH4Citation[10]. This peptide is composed in such a manner that hydrophobic interactions and the polar properties of histidine side chains are in a fine balance. The membrane interactions of the histidines are conveniently manipulated by alterations of the pH of the environment. This allows us to switch the alignment of the peptides in a pH-dependent manner. Furthermore, the LAH4 peptide exhibits pronounced antimicrobial activity Citation[11] and acts as a potent DNA transfectant Citation[12]. Notably, this latter activity is strongly correlated to the capacity of the peptide to change its alignment relative to the membrane normal Citation[10]. A transition pH of the peptide close to 6 has been found essential for the efficient DNA transfection activity Citation[12]. In the work presented here we have investigated in a systematic manner how the amino acid composition of LAH4-related peptides can be used to modulate their topological transitions.
All sequences of the LAH4 family of peptides are characterized by a hydrophobic core composed of alanines and leucines that is interrupted by four histidines and flanked by two lysines at each terminus. Whereas the inner core of the peptide sequence is sufficiently long and hydrophobic to be able to span the lipid bilayer the histidines are arranged in such a manner to allow the formation of an amphipathic α-helix (). The lysines act as membrane anchors, improve the handling of the peptides and prevent aggregation in membranes as tested by circular dichroism (CD) line shape – Citation[13], 2H solid-state rotational diffusion – Citation[14] and SDS-PAGE analysis (not shown). In the presence of detergent micelles the histidine pK values of LAH4 and related peptides range between 5.4 and 6.1 Citation[10], Citation[15]. The histidine side chains are thus positively charged at acidic pH, and polar but uncharged at neutral conditions. A related approach has recently been used to control and study the membrane-association of the model peptide TMX-3 Citation[16].
Figure 1. Sequence and helical wheel diagram of LAH4X4 peptides. Whereas the hydrophobic side B consists of leucines and alanines, face A is assembled from the amino acids X and flanked by two histidines on each side.
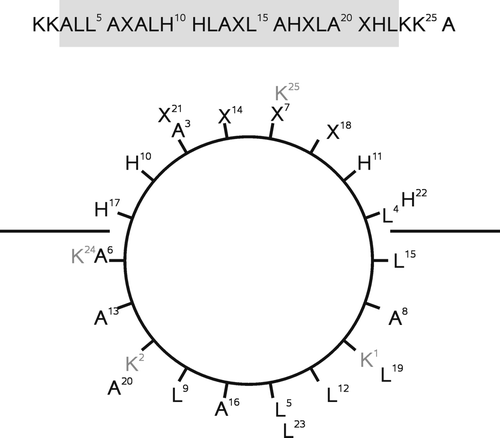
The helical wheel diagram of LAH4X4, a peptide derived from the original LAH4 sequence and used during the investigations presented here, is shown in . A hydrophobic face and a surface opposite which, depending on pH, is polar or charged define the amphipathic character of the helix. Four amino acids of variable composition (labelled X) are interspersed between the histidines. This design opens avenues to test for the propensity of the X amino acids to translocate from the bilayer interface to the membrane interior. When the X amino acids are hydrophobic they provide a strong driving force for membrane insertion which is counteracted by the pH-dependent preference of the histidine side chains to remain in the water phase. Therefore the transmembrane insertion of the peptides is dependent on both the environmental pH and the ‘hydrophobicity’ of the X-amino acids. The pH of the in-plane to transmembrane transitions can thus be used to test for the ‘hydrophobicity’ of the amino acids X.
Attenuated total reflection Fourier transform infra red (ATR-FTIR) spectroscopy of oriented membrane samples has been established previously to monitor the average peptide alignment and conformation by measurement of the dichroic ratio Citation[17], Citation[18] and the frequencies of the amide bands, respectively Citation[19]. Whereas α-helical peptides exhibit wave numbers in the 1662–1648 cm−1 region, β-sheet conformations resonate at 1640–1620 cm−1 with a shoulder between 1689 and 1682 cm−1. Random coil sequences are found between 1655 and 1640 cm−1 with a band width much broader than for the helical structures Citation[19]. Furthermore, the dichroic ratio of the amide I band is a direct indicator of the average tilt angle of helical polypeptides Citation[17]. Whereas in-plane oriented peptides exhibit R-values around 1.3 this parameter can augment up to about 4 in the presence of transmembrane orientations. Here we have used this approach to investigate several peptides of the LAH4X4 series.
Theory
In order to describe the bilayer insertion of membrane-associated LAH4X4 a sequence of equilibria connecting subsequent states is considered: IPch↔IP0↔TM. Here IP indicates the in-plane oriented peptide helices, with the histidines charged (ch) or neutral (o), respectively, and TM the transmembrane inserted peptides (). This model can easily be extended by also considering peptides that are dissolved in the aqueous phase when bulk water is present.
The energy contributions that govern the in-plane→transmembrane reorientation process have already been discussed in some detail in earlier work Citation[10]. According to the model only LAH4-type peptides that carry uncharged histidines are able to adopt the transmembrane configuration, whereas peptides carrying charged histidine side chains adopt in-plane configurations Citation[10], Citation[17].
However, even at pH values smaller or around the pK the histidine side chains can be neutralized. The required energy of discharge must derive from other driving forces that are associated with changes in the peptide's conformation and topology, such as ‘hydrophobic’ energies. Therefore, for predominantly hydrophobic peptides transmembrane-insertion and discharge are tightly connected processes and a direct equilibrium between the transmembrane uncharged peptide and the in-planar charged peptide is established (IPch↔TM).
On the other hand, in cases where the X-residues exhibit a low degree of hydrophobicity the in-planar state is populated even when the histidines are not charged (IP0). When the pH of the sample is augmented the four histidines of such peptides are discharged in a gradual manner. As a consequence, close to the pKa values of the histidines, only a fraction of these side chains are charged. Therefore, in this region a transmembrane uncharged state (TM), an uncharged in-planar state (IP0) and differently charged in-planar states are taken into consideration (IP1 + , IP2 + , IP3 + , IP4 + ).
The equilibrium constant for the IP to TM transition is related to the free energy ΔG gained by moving the peptide from the interface into the membrane interior. Whereas the in-planar orientation can be made accessible on both sides of the lipid bilayer two possible orientations exist for the transmembrane peptide. The equilibrium constant is therefore defined as:1
Furthermore, the uncharged in-planar configuration (IP0) is in equilibrium exchange with the series of charged in-planar states (IPch). If we assume that none of these charged states is able to insert into the membrane, they can be combined: [IPch]=[IP1 + ]+[IP2 + ]+[IP3 + ]+[IP4 + ]. The reciprocal of the constant (kCh) for the transition IP0↔IPch can be expressed as a sum of the reciprocals of the individual constants:2 kj depends on the free energy difference by charging j histidines, which can be realized in
different ways. The constants are given by:
3 Combining Equations 2 and 3 results in
4
The experimentally accessible ratio of the transmembrane over total peptide concentration, pTM, is given by:5
This function (5) is graphically represented in . The slope of the transition is thus a function of ΔG, being steeper for very negative ΔG (). For close-to-zero or positive ΔG the transmembrane state is only partly populated even at elevated pH values. also illustrates that for ΔG near and >0, the transition mid-point is shifted towards pH values higher than the pKa values of the histidines. This shift is due to the degeneracy of the in-planar states where the configurations carrying one to three charges can be realized in εj ways.
Figure 3. The fraction of transmembrane aligned peptide, pTM, as a function of pH at different transfer energies ΔG/RT. The titration curves were simulated with pKa values 6.0 for the histidine side chains. The simulations shown represent the following values of ΔG/RT: -8 (solid line), -2 (hatched line), 0 (dotted line), and 2 (hatched-dotted line).
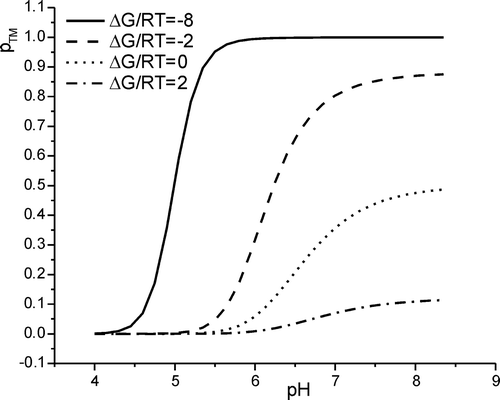
For very hydrophobic peptides ▵G < < 0 and the transition takes place at pH < pK. In this case the fraction of transmembrane peptide and can be approximated by:6
The resulting curve is sigmoidal with the transition at .
Notably, this result is also obtained by considering a simple model of a direct IP4 + ↔TM equilibrium. The free energy difference of this process has been discussed previously Citation[10].
The midpoint of transition, pH50, can be defined by the pH-value, where 50% of the molecules are in transmembrane state (pTM=1/2). Using Equation 5 one obtains:7
For peptides of moderate or low hydrophobicity it is more appropriate to measure p1/2, which indicates the pH where 50% of the maximal pTM is reached (p1/2=1/2 pTMmax, cf. ). With:8 pH1/2 becomes:
9
A graphical representation of the variation of pH50 and of pH1/2 as a function of ΔG/RT is shown in . Notably, the two parameters are identical for very negative ΔG (ΔG/RT < − 10) but differ significantly for moderately hydrophobic or hydrophilic peptides. When the transition is characterized by highly negative ΔG a linear correlation is obtained:10
Figure 4. The calculated shifts for the in-plane to transmembrane transition pH as compared to the pKa value common to all histidines is shown as a function of changes in hydrophobicity of the X-amino acids, ΔG/RT. Whereas the solid line corresponds to the pH where half the maximum of pTM is reached, the dotted line indicates the pH value where half the peptides are in a transmembrane state, pTM=50%. The dashed line represents the linear approximation obtained for very hydrophobic peptides (large negative ΔG). See text for details.
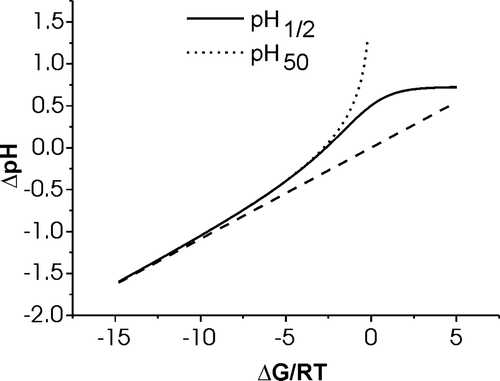
The local pH close to the membrane surface or in the proximity of other charges and dipoles exhibits significant differences when compared to the proton activity in bulk solution. Therefore, when the apparent pK values of LAH4 and related peptides is investigated in the presence of dodecyl phosphocholine (DPC) detergent micelles the titration curves are less steep than expected from the Henderson-Hasselbach equation 10, Citation[15]. To take these deviations into account a ‘steepness’ factor c is introduced, that takes into account attractive or repulsive interactions at the biomolecular surfaces. By fitting the pH-dependent 1H chemical shifts of the indol 4H positions of several related LAH4X-peptides the pK values obtained range between 5.8 and 6.1, with average values of c = 0.8 and pKa=6.0 Citation[15]. Therefore, the following modification of Equation 5 is introduced:11
To quantitatively analyse the dichroic ratio obtained by ATR-FTIR measurements the value calculated for PTM is inserted into the following equation Citation[17].12 Here
and
are the maximal and minimal dichroic ratio, respectively.
Materials and methods
Peptides were prepared by solid-phase peptide synthesis and purified as described before Citation[14]. The identity and the purity of the products were confirmed by matrix-assisted laser-desorption ionization (MALDI) mass spectrometry.
CD spectroscopy
For CD spectroscopic analysis 1-palmitoyl-2-oleoyl-sn-glycero-3-phosphocholine (POPC) (Avanti Polar Lipids, Birmingham AL, USA) was suspended in 10 mM phosphate buffer at concentrations of 10 mM. This ‘stock solution’ was left to equilibrate for several hours and vortexed at regular intervals. Small unilamellar vesicles (SUV) were prepared by sonification using a tip sonicator (soniprep 150, MSE [SANYO], Tokyo, Japan). The sample was cooled with an ice bath during the application of pulsed ultrasound and titanium was removed by centrifugation afterwards. The peptides were added to 10 mM phosphate buffer of the indicated pH value from a 1 mM stock solution in order to obtain a 10 µM peptide solution. Lipid was added from the 10 mM stock solution to obtain the indicated lipid concentration. These peptide solutions were directly analysed by CD spectroscopy. The CD spectra were recorded on a Jasco J-810 CD Spectrometer using a∼300 µl cell with a path-length of 1 mm. Eight scans covering the spectral range between 190 mn and 260 nm were accumulated.
The CD spectra were evaluated using the software package CDPro (N. Sreerama and R.W. Woody, Colorado State University, USA, Citation[20]) using the reference set SP37A and SPD48 and the CONIN/LL fitting algorithm. In addition, the CD spectra were evaluated by calculating the factor:
, where [θ]222is the molar ellipticity at 222 nm and [θ]min, the minimal molar ellipticityin the range 195–210 nm Citation[21]. When the peptide conformation follows a two-state equilibrium involving helical and random coil secondary structures the factor R varies between 0 (absence of helical structure) and ≥1 (100% α-helix) Citation[21]. This approach also works when uncertainties about the peptide concentration exist, e.g., due to adsorption to the walls of vials and cuvettes.
ATR-FTIR spectroscopy
In order to test the pH-dependent topology of LAH4X4 oriented membrane samples were prepared for ATR-FTIR measurements using the procedure described in Citation[15]. In short, the peptide-lipid mixtures in TFE/water were applied on a 5×1 cm surface of a plasma cleaned trapezoidal germanium ATR plate (ACM, Villiers St Frédéric, France) with an aperture angle of 45°. The organic solvent was evaporated under a stream of nitrogen. Oriented lipid bilayers spontaneously form along the surface of the crystal Citation[18]. In order to adjust the pH values the samples were overlaid with 200 µl of 66 mM phosphate buffer of the appropriate pH, carefully washed with MilliQ-water (Millipore) and dried under a stream of nitrogen. A fresh sample was prepared for every pH value.
ATR-FTIR measurements were performed on a Bruker IFS55 FTIR spectrophotometer (Ettlingen, Germany) equipped with a MCT detector at a resolution of 2 cm−1. For each spectrum 64 scans were accumulated. The spectrometer was continuously purged with dry air.
All the spectra showed a well-resolved lipid C≈O peak at about 1750 cm−1. The amide I peak was located at about 1650 cm−1. The spectral intensity at 1657 cm−1 was analysed for the parallel and perpendicular polarization of the light beam. The dichroic ratio was calculated by division of the intensity of the parallel by the intensity of the perpendicular spectrum. Thus the amide I band exhibits dichroic ratios between 1.2 and >3, these values reflecting the in-plane and transmembrane helical orientations.
Results
The peptides of the LAH4X4 series were designed to form α-helical secondary structures in membrane environments as has been observed for many other sequences of related composition Citation[10], Citation[17], Citation[22]. The helical design of the LAH4X4 peptides is confirmed in oriented lipid bilayers by the characteristic absorption at 1657 cm−1 and 1545 cm−1 in ATR-FTIR spectra. A detailed analysis indicates that in all membrane samples investigated the peptides investigated adopt about 75% α-helix secondary structure in membrane environments, with the remainder corresponding to turn structures (). The standard variations are typically around 10%. This is confirmed by CD spectroscopic experiments which consistently indicate a very high degree of helix formation in the presence of small unilamellar vesicles (). In this conformation the overall hydrophobic face made of leucines and alanines covers a hydrophobic angle of 200° (side B in ). The remaining part (side A) contains four amino acids X which are flanked by the histidines.
Figure 5. The amide region of ATR-FTIR spectra of LAH4F4 reconstituted into oriented POPC membranes is shown at pH 8 (A) and at pH 3 (B). The incident light was polarized in a parallel (dotted lines) or in a perpendicular orientation (hatched lines). The difference spectra (solid lines) are obtained by subtracting the perpendicular polarized spectrum from the parallel polarized spectrum. For this Figure the latter was multiplied by a factor 1.35 before subtraction, which corrects for the difference of strength of the evanescent power for the parallel and perpendicular polarisations as discussed in detail in reference Citation[17].
![Figure 5. The amide region of ATR-FTIR spectra of LAH4F4 reconstituted into oriented POPC membranes is shown at pH 8 (A) and at pH 3 (B). The incident light was polarized in a parallel (dotted lines) or in a perpendicular orientation (hatched lines). The difference spectra (solid lines) are obtained by subtracting the perpendicular polarized spectrum from the parallel polarized spectrum. For this Figure the latter was multiplied by a factor 1.35 before subtraction, which corrects for the difference of strength of the evanescent power for the parallel and perpendicular polarisations as discussed in detail in reference Citation[17].](/cms/asset/3b153f25-1433-4ec9-8e77-90cf701ee322/imbc_a_173837_f0005_b.gif)
Figure 6. CD spectra of 10 µM LAH4L4 in the presence of 1000 µM small unilamellar POPC vesicles at pH 4.0 (dotted line) pH 5.0 (dash-dot line), pH 6.0 (dashed line) and pH 7 (solid line)
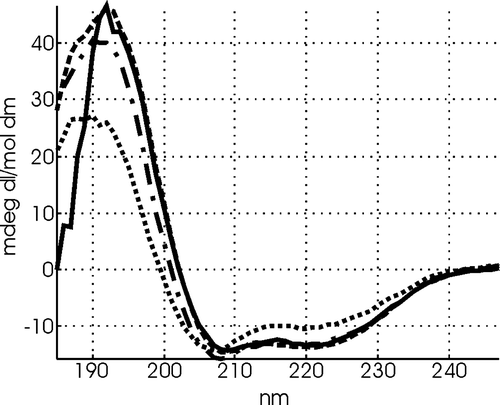
The ATR FTIR spectra of LAH4F4 when reconstituted into oriented POPC membranes exhibits maxima at 1657 cm−1 (amide I) and at 1545 cm−1 (amide II, ) indicative of α-helical conformations Citation[19]. Some of the spectra show an additional small resonance at 1688 cm−1 (B). The in-plane to transmembrane transition curve is well defined between pH 5 and pH 6 (A). With pKa=6.0 and c=0.8 the data analysis (Equations 11 and 12) provides and
. The results from other peptides are shown in and the analysis of these data is summarized in .
Figure 7. The dichroic ratio RATR of LAH4X4 peptides reconstituted into oriented POPC phospholipids bilayers are shown as a function of pH. A. LAH4F4, B. LAH4L4, C. LAH4V4, D. LAH4W4, E. LAH4I4., F. LAH4A3L. The experiments were performed in duplicate.
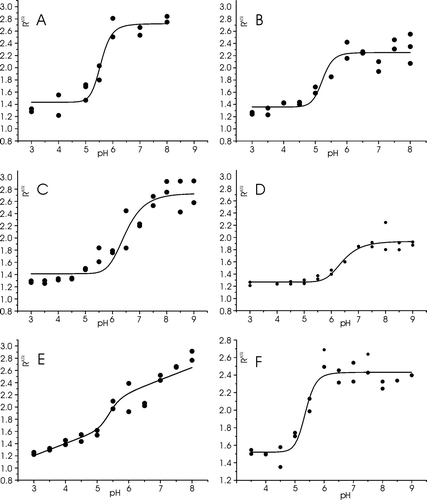
Table I. Analysis of the pH-dependent dichroic ratio of LAH4X4 peptides as observed in oriented ATR-FTIR experiments. A fit of the experimental data was obtained using Equations 11 and 12.
Notably, the RATR-pH diagram of LAH4W4 shows a well-defined transition (D), albeit the dichroic ratio of the transmembrane peptide 2.2 is comparatively low when compared to the values of the other peptides. On the other hand the pH-dependent transition of RATR of LAH4I4 is less well defined. To first approximation the curve is a superposition of a linear
and a sigmoidal contribution (E), the latter forming the basis for the calculation of ΔG ().
Discussion
The LAH4X4-peptides were designed to adopt α-helical conformations in membrane environments. Both FTIR and CD spectroscopies confirm that the peptides consistently adopt a high degree of helical secondary structures in the presence of membranes and at all pH values tested (). In particular the ATR-FTIR spectra indicate that under all conditions tested the peptides exhibit amide II and amide I bands in the range 1657 cm−1 and 1545 cm−1 (±3 cm−1), respectively, which are indicative of a high degree of α-helix conformation (). Deconvolution of the spectral FTIR spectral line shapes indicates about 75% regular helices and the remainder ‘turn structures’, which includes irregular α-helical conformations.
The design of the LAH4X4-series allows for four amino acids X to be exchanged and tested for their propensity to move from the membrane interface to the membrane interior. The large number of X residues allows one to increase the differences in the transition pH, thereby also reducing the statistical errors associated with the per-residue-analysis of ΔG/RT. In previous experiments we have also tested polypeptides of an LAH4X6-series Citation[15], where the hydrophobic angle was considerably reduced when compared to the present peptides. Although the differences in transition pH between the polypeptides of the LAH4X4-series are less pronounced, unwanted side effects such as exceedingly high solubility in the aqueous phase or distortions of the α-helical structure due to many like-residues next to each other are less likely.
At low pH all the peptides investigated exhibit a dichroic ratio around 1.35±0.1, indicative of in-plane oriented helices. In contrast the maximal dichroic ratios observed at high pH vary between 2.17 (tryptophan peptide) and 3.37 (valine derivative, ). The values are within the variations previously observed for transmembrane peptides of different compositions Citation[17]. The low maximum dichroic ratio measured for the LAH4W4 peptide could indicate a slight tilt of the peptide with respect to the membrane normal. As the high values of the dichroic ratio are very sensitive to the tilt a 5–10° deviation is sufficient to explain this difference Citation[23]. Alternatively, a distortion of the α-helix geometry in the presence of bulky side chains could deviate the transition dipole. However, the main conclusions of the present work are independent of the absolute values of the dichroic ratios.
The free energy difference between the in-planar and the transmembrane configuration is directly obtained from the pH dependence of the dichroic ratio measured in oriented ATR-FTIR spectra ( and ). A prerequisite for an equilibrium thermodynamic analysis (cf. theory section) is the reversibility if the in-plane to transmembrane transition which has previously been demonstrated for LAH4 and its derivatives Citation[17]. of this previous paper Citation[17] shows a titration curve of LAH4, which was obtained from a single oriented sample. The pH was changed in a stepwise manner by overlying the sample with buffer of the desired pH, equilibration for a few minutes, drying and recording the spectra, incubation with buffer etc. The curve was recorded from low to high and back to low pH. In of reference Citation[17] two data points at a given pH represent the measurements that have been recorded before and after the highest pH has been reached, respectively. Other peptides of the family have shown reversibility as well. Interestingly, the reversibility of the transition implies that the terminal lysines pass to the opposite site of the membrane thereby confirming earlier data where lysine-containing peptides can adopt transmembrane alignments provided that the large energy associated with lysine-insertion in the hydrophobic environment is compensated by other favourable contributions Citation[24].
The free energy of reorienting helical peptides from in-plane to transmembrane alignments has previously been shown to be a composition of energetic contributions from the peptide as well as the lipid bilayer Citation[10], Citation[25], Citation[26]. The absolute value of ΔG includes many different contributions including discharge and membrane insertion of the histidines, the hydrophobic character of the amino acids X as well as of other residues changing their environment during the transition. Furthermore, changes in lipid packing or entropic effects have also to be considered. However, when two peptides of the same series are directly compared to each other the differences reflect to first approximation the contributions of the four X amino acids.
and summarize free energy data obtained from the LAH4X4 peptides. The comparison of e.g., LAH4L4 and LAH4F4, provides the difference for the exchange of four leucines by four phenylalanines in a direct manner. When other more hydrophilic amino acids were tested less leucines were exchanged. The leucines thus remaining at the X-positions ensure a high enough driving force into the transmembrane configuration also in the presence of hydrophilic residues at the A-face (). The per-residue errors obtained in these latter experiments, therefore, scale by a correspondingly lower factor ().
Figure 8. Summary of the relative contributions of the amino acid X to ΔG of transfer from in-plane to transmembrane alignments (cf. text for details). The values are scaled to take into account the influence of one X amino acid side chain within the peptide. The LAH4L4 peptide is taken as a reference. The boxes indicate the experimental errors obtained during the line fit analyis of ΔG/RT (). The top scale indicates values obtained when calculating the difference ΔG(aqueous→octanol) - ΔG(aqueous→membraneinterface) as published in Citation[6].
![Figure 8. Summary of the relative contributions of the amino acid X to ΔG of transfer from in-plane to transmembrane alignments (cf. text for details). The values are scaled to take into account the influence of one X amino acid side chain within the peptide. The LAH4L4 peptide is taken as a reference. The boxes indicate the experimental errors obtained during the line fit analyis of ΔG/RT (Table I). The top scale indicates values obtained when calculating the difference ΔG(aqueous→octanol) - ΔG(aqueous→membraneinterface) as published in Citation[6].](/cms/asset/b6dd037f-89a5-4012-a9ac-7bf54b6fc906/imbc_a_173837_f0008_b.gif)
The experimental data (, ) thereby provide a measure of the differences in ΔG for the transfer from the membrane interface to its interior when the amino acid composition represented by X4 is modified (). During the design of the series of peptides the rather hydrophobic LAH4L4 provided the starting point. Successively 2–4 leucines were exchanged with other amino acids and the resulting peptides investigated. In an arbitrary manner the transition of leucine thus provides a reference ().
However, the membrane insertion of polyalanine peptides (≥18 residues) were measured using proton-decoupled 15N solid-state nuclear magnetic resonance (NMR) spectroscopy Citation[27] or in vivo by glycosylation mapping of the topology of the integral membrane protein leader peptidase carrying an additional H-domain Citation[2]. The data show that for these peptides in-plane and transmembrane alignments co-exist, thereby indicating that the transfer of alanine, in the context of a helical peptide and a phospholipid bilayer, partitions equally likely in the membrane interface or the membrane interior. The free energy of this transition was therefore found ≥ − 0.2 kJ/mole Citation[27]. A close to zero propensity of alanine to enter the membrane interior was also observed in other experiments such as those described by references Citation[2], Citation[5], Citation[28–30].
In the past the ‘hydrophobicity’ of the common amino acids has been analysed and tabulated using different model systems. These include measurements of the transfer of amino acid side chains from liquid water into a condensed vapour phase Citation[31] or the solubility of the amino acids in water/organic solvent mixtures of different composition Citation[32]. This latter approach makes accessible the free energy associated with the transfer of amino acids from the water into organic solvent phases. Using this data a wide variety of mixed scales based on experimental data and educated guesses have been established, which have in common that the transition of amino acid side chains from an aqueous phase to a hydrophobic environment (mimicking the membrane interior) are compared to each other (e.g., Citation[2], Citation[4], Citation[33–36]). Due to differences in the systems considered already between these commonly used scales considerable variations are observed. Glycine, for example, is considered ‘hydrophobic’ in one table Citation[4] or hydrophilic in another Citation[2].
In contrast, in a more recent investigation the transition of small peptides from the water to the membrane interface has been studied Citation[3]. In another series of experiments the amino-acid dependent ‘hydrophobicity’ of extended peptide chains has been tested by analysing their high performance liquid chromatography (HPLC) retention times Citation[37]. Furthermore, statistical methods have become more elaborate by analysing the composition of transmembrane sub-segments Citation[5], Citation[38], Citation[39]. In the work presented in this paper the transfer of amino acid residues from the interface to the membrane interior has been analysed, thereby complementing these latter studies.
To our knowledge, the data presented here are the first experimental measure of the transition free energies from the membrane interface to the membrane interior. In order to be able to compare our data to previous experiments we have therefore established a calculated scale using the data of Citation[3]. These authors have published whole-residue energies of transfer from the aqueous medium to the membrane interface using a series of small peptides (≤6 residues), which they later tested on larger peptides Citation[16]. By taking the difference between the energies published for the transfer water→octanol and that of the partitioning water→interface, an energy associated with the transfer interface→octanol is obtained (). Although the energies calculated in that manner are of the same order of magnitude as those measured in this paper, considerable differences exist when individual amino acids are compared (, ). These probably reflect the differences in peptide and ‘solvent’ systems investigated. Notably the interfacial properties can vary as well and depend on the phospholipid system investigated Citation[40]. The calculated and measured scales shown in also confirm the design of our peptides () as at acidic pH the ‘hydrophobic’ energies are significantly lower that the energy of discharge of two or more histidines Citation[10].
Our results indicate that, in the context of helical peptides and in membrane environments, of the amino acids tested only leucine exhibits a clear preference to transfer from the interface into the hydrophobic interior. Furthermore phenylalanine and serine exhibit a preference similar to that of alanine. The ‘hydrophilic’ amino acid side chains such as glycine, threonine or tyrosine, but also tryptophan and valine, exhibit a preference for a localization in the membrane interface, which is associated with in-plane alignments of the peptides. The interfacial preference of valine seems surprising although in good agreement with the calculated scale using the data of Citation[6]. Possibly, the steric constraints imposed by placing four bulky side chains next to each other on one face of the helix might have an effect on the energy of transition of the LAH4V4 peptide.
Furthermore one might have expected a more pronounced preference of phenylalanine and tryptophan for the membrane interface due to specific interactions at the level of the phospholipids carbonyls. However, in the context of the in-plane helical peptides the aromatic side chains of the LAH4W4 and LAH4F4 peptides () can probably not all penetrate sufficiently into the membrane and/or orient in such a manner to efficiently interact with the lipid carbonyls Citation[41]. Therefore, the values measured in this context should reflect their hydrophobic/hydrophilic properties rather than specific interactions with the lipids. It also remains possible that selected residues of the transmembrane peptide specifically interact with the lipid interface (e.g., H-bonding), thereby stabilizing this configuration. More work is needed to clarify such details, this, however, is beyond the scope of the present paper. Notably in recent work considerable differences have also been observed when the energetic contributions of amino acids have been studied as a function of position within transmembrane oriented helices Citation[2], Citation[8].
Although we have initially made the approximation that the transition curve is predominantly affected by the propensity of the four amino acids X () to be located either in the interface or the membrane interior this assumption should be considered in more detail. Notably, when different peptides of the LAH4X4 series are compared to each other the initial states (IP) might not always be exactly the same even though the initial alignment of the peptide is in all cases parallel to the membrane surface. For example, the hydrophobicity of the amino acid side chain might have an effect on the insertion depth of the peptide when aligned parallel to the membrane surface. Similarly, small differences of the transmembrane helix conformation and tilt angle might be related to the amino acid composition. Such effects compensate for some of the differences in ‘hydrophobicity’ between the X amino acids. The environmental changes experienced during the in-plane to transmembrane transition might therefore be less pronounced in case of the leucine side chain when compared to alanine or other hydrophilic residues.
In this paper a novel concept is presented where the pH-dependent alignment of histidine containing amphipathic helices Citation[10] is used to study the energies involved in the transfer between in-plane and transmembrane alignments Citation[9]. Notably, the alignment of peptides in an in-plane or in a transmembrane fashion has a profound effect on their acitivity and can have implications in their regulation Citation[11], Citation[12]. The quantitative biophysical data presented in this paper can thus be used to design peptides that are active in a selected pH range or in a specific organelle Citation[12]. Here a theoretical model has been developed and applied to directly evaluate the free energy of transition from the mid-point and the shape of the transition curve. By using a series of peptides where a well-defined number of amino acids is systematically exchanged () the relative propensities of these residues for a location at the membrane-water interface or in the membrane interior is obtained (). The data thus obtained also have an impact on our understanding of the amino acid propensities for different locations within the lipid bilayer.
The question of how polypeptide sequences orient relative to the membrane surface is of considerable importance during the investigations of peptides but also during predictions of the topology of larger membrane proteins. Whereas an in-plane oriented helix leaves the neighbouring hydrophilic domains on the same side of the bilayer, a change of cellular compartments is associated with a transmembrane built. Notably, the structural data that exists from membrane proteins have been obtained from well-folded compact proteins that pass the bilayer one or several times in a well-defined fashion. However, biophysical studies indicate that some membrane-inserted proteins exhibit a more loosely folded structure, thereby resembling an assembly of individual helices such as the colicin channel domains Citation[42]. The interactions of these proteins with the membrane should, therefore, be well described by a scale such as the one developed in this paper.
This paper was first published online on prEview on 31 May 2006.
We are grateful to the Agence Nationale pour la Recherche contre le SIDA, Vaincre la Mucoviscidose, the CNRS, the Université Louis Pasteur, the Reseau RMN Grand Est and the French Ministry of Research for financial support. We thank Josefine März, Monika Zabowa and Gérard Nullans for the preparation and MALDI mass spectrometry of the peptide synthetic products. Peter Chen has provided helpful information on the up-to-date use of hydrophobicity scales and statistical methods.
References
- Chen CP, Rost B. State-of-the-art in membrane protein prediction. Appl Bioinform 2002; 1: 1–15
- Hessa T, Kim H, Bihlmaier K, Lundin C, Boekel J, Andersson H, Nilsson I, White SH, von Heijne G. Recognition of transmembrane helices by the endoplasmic reticulum translocon. Nature 2005; 433: 377–381
- Wimley WC, White SH. Experimentally determined hydrophobicity scale for proteins at membrane interfaces. Nature Struct Biol 1996; 3: 842–848
- Engelman DM, Steitz TA, Goldman A. Identifying nonpolar transbilayer helices in amino acid sequences of membrane proteins. Ann Rev Biophys Biophys Chem 1986; 15: 321–353
- Punta M, Maritan A. A knowledge-based scale for amino acid membrane propensity. Proteins 2003; 50: 114–121
- White SH, Wimley WC. Membrane protein folding and stability: Physical principles. Ann Rev Biophys Biomol Struct 1999; 28: 319–365
- Tusnady GE, Simon I. The HMMTOP transmembrane topology prediction server. Bioinform 2001; 17: 849–850
- Hessa T, White SH, von Heijne G. Membrane insertion of a potassium-channel voltage sensor. Science 2005; 307: 1427
- Bechinger B. Solid-state NMR investigations of interaction contributions that determine the alignment of helical polypeptides in biological membranes. FEBS Lett 2001; 504: 161–165
- Bechinger B. Towards membrane protein design: pH dependent topology of histidine-containing polypeptides. J Mol Biol 1996; 263: 768–775
- Vogt TCB, Bechinger B. The interactions of histidine-containing amphipathic helical peptide antibiotics with lipid bilayers: The effects of charges and pH. J Biol Chem 1999; 274: 29115–29121
- Kichler A, Leborgne C, März J, Danos O, Bechinger B. Histidine-rich amphipathic peptide antibiotics promote efficient delivery of DNA into mammalian cells. Proc Natl Acad Sci USA 2003; 100: 1564–1568
- Loudet C, Khemtemourian L, Aussenac F, Gineste S, Achard MF, Dufourc EJ. Bicelle membranes and their use for hydrophobic peptide studies by circular dichroism and solid state NMR. Biochim Biophys Acta 2005; 1724: 315–323
- Aisenbrey C, Bechinger B. Investigations of peptide rotational diffusion in aligned membranes by 2H and 15N solid-state NMR spectroscopy. J Am Chem Soc 2004; 126: 16676–16683
- Aisenbrey C, Kinder R, Goormaghtigh E, Ruysschaert JM, Bechinger B. Interactions involved in the realignment of membrane-associated helices: An investigation using oriented solid-state NMR and ATR-FTIR spectroscopies topologies. J Biol Chem 2006; 281: 7708–7716
- Ladokhin AS, White SH. Interfacial folding and membrane insertion of a designed helical peptide. Biochemistry 2004; 43: 5782–5791
- Bechinger B, Ruysschaert JM, Goormaghtigh E. Membrane helix orientation from linear dichroism of infrared attenuated total reflection spectra. Biophys J 1999; 76: 552–563
- Ivanov D, Dubreuil N, Raussens V, Ruysschaert JM, Goormaghtigh E. Evaluation of the ordering of membranes in multilayer stacks built on an ATR-FTIR germanium crystal with atomic force microscopy: the case of the H(+),K(+)-ATPase-containing gastric tubulovesicle membranes. Biophys J 2004; 87: 1307–1315
- Goormaghtigh E, Cabiaux V, Ruysschaert JM. Determination of soluble and membrane protein structure by Fourier transform infrared spectroscopy. III. Secondary structures. Sub-Cellular Biochem 1994; 23: 405–450
- Sreerama N, Woody RW. A self-consistent method for the analysis of protein secondary structure from circular dichroism. Anal Biochem 1993; 209: 32–44
- Bruch MD, Dhingra MM, Gierasch LM. Side chain-backbone hydrogen bonding contributes to helix stability in peptides derived from an α-helical region of carboxypeptidase A. Proteins: Struct. Funct Genetics 1991; 10: 130–139
- Zhang YP, Lewis RN, Hodges RS, McElhaney RN. Interaction of a peptide model of a hydrophobic transmembrane alpha-helical segment of a membrane protein with phosphatidylethanolamine bilayers: Differential scanning calorimetric and Fourier transform infrared spectroscopic studies. Biophys J 1995; 68: 847–857
- Goormaghtigh, E, Ruysschaert, JM. Polarized attenuated total reflection infrared spectroscopy as a tool to investigate the conformation and orientation of membrane components, In: R Brasseur, editor, Molecular description of biological membranes by computer aided conformational analysis. Boca Raton, FL: CRC Press; 1990. pp 285–329.
- Vogt TCB, Ducarme P, Schinzel S, Brasseur R, Bechinger B. The topology of lysine-containing amphipathic peptides in bilayers by CD, solid-state NMR and molecular modelling. Biophys J 2000; 79: 2644–2656
- Chatelier RC, Minton AP. Adsorption of globular proteins on locally planar surfaces: models for the effect of excluded surface area and aggregation of adsorbed protein on adsorption equilibria. Biophys J 1996; 71: 2367–2374
- Zuckermann MJ, Heimburg T. Insertion and pore formation driven by adsorption of proteins onto lipid bilayer membrane-water interfaces. Biophys J 2001; 81: 2458–2472
- Bechinger B. Membrane insertion and orientation of polyalanine peptides: A 15N solid-state NMR spectroscopy investigation. Biophys J 2001; 82: 2251–2256
- Lewis RN, Zhang YP, Hodges RS, Subczynski WK, Kusumi A, Flach CR, Mendelsohn R, McElhaney RN. A polyalanine-based peptide cannot form a stable transmembrane alpha-helix in fully hydrated phospholipid bilayers. Biochemistry 2001; 40: 12103–12111
- Liu LP, Li SC, Goto NK, Deber CM. Threshold hydrophobicity dictates helical conformations of peptides in membrane environments. Biopolymers 1996; 39: 465–470
- Ben-Tal N, Ben-Shaul A, Nicholls A, Honig B. Free-energy determinants of α-helix insertion into lipid bilayers. Biophys J 1996; 70: 1803–1812
- Wolfenden R, Andersson M, Cullis PR, Souvignier G. ffinities of amino acid sidechains for solvent water. Biochemistry 1981; 20: 849–855
- Nozaki Y, Tanford C. he solubility of amino acids and two glycine peptides in aqueous ethanol and dioxane solution. J Biologic Chem 1971; 246: 2211–2217
- von Heijne G. Membrane Proteins: The amino acid composition of membrane penetrating segements. Eur J Biochem 1981; 120: 275–278
- Kyte J, Doolittle RF. A simple method for displying the hydropathic character of a protein. J Mol Biol 1982; 157: 105–132
- Rees DC, DeAntonio L, Eisenberg D. Hydrophobic organization of membrane proteins. Science 1989; 245: 510–513
- White, SH. Hydropathy plots and the prediction of membrane protein topology, In: White SH, editor. Membrane protein structure: Experimental approaches. New York: Oxford University Press; 1994. pp 97–124.
- Deber CM, Wang C, Liu LP, Prior AS, Agrawal S, Muskat BL, Cuticchia AJ. TM Finder: A prediction program for transmembrane protein segments using a combination of hydrophobicity and nonpolar phase helicity scales. Protein Sci 2001; 10: 212–219
- Jones DT, Taylor WR, Thornton JM. A model recognition approach to the prediction of all-helical membrane protein structure and topology. Biochemistry 1994; 33: 3038–3049
- Pilpel Y, Ben-Tal N, Lancet D. kPROT: A knowledge-based scale for the propensity of residue orientation in transmembrane segments. Application to membrane protein structure prediction. J Mol Biol 1999; 294: 921–935
- Ladokhin AS, White SH. Protein chemistry at membrane interfaces: Non-additivity of electrostatic and hydrophobic interactions. J Mol Biol 2001; 309: 543–552
- Killian JA, von Heijne G. How proteins adapt to a membrane-water interface. Trends Biochem Sci 2000; 25: 429–434
- Zakharov SD, Lindeberg M, Griko Y, Salamon Z, Tollin G, Prendergast FG, Cramer WA. Membrane-bound state of the colicin E1 channel domain as an extended two-dimensional helical array. Proc Natl Acad Sci USA 1998; 95: 4282–4287