Abstract
BK channels regulate vascular tone by hyperpolarizing smooth muscle in response to fluctuating calcium concentrations. Oestrogen has been reported to lower blood pressure by increasing BK channel open probability through direct binding to the regulatory β1-subunit(s) associated with the channel. The present investigation demonstrates that 17β-oestradiol activates the BK channel complex by increasing the burst duration of channel openings. A subconductance state was observed in 25% of recordings following the addition of 17β-oestradiol and could reflect uncoupling between the pore forming α1-subunit and the regulatory β1-subunit. We also present evidence that more than one β1-subunit is required to facilitate binding of 17β-oestradiol to the channel complex.
Acronyms | ||
BK channel | = | large conductance, Ca2+ and voltage dependent K+ channel |
VDCC | = | Voltage dependent Ca2+ channels |
STOC | = | spontaneous transient outward currents |
Introduction
Large conductance Ca2+- and voltage-sensitive K+ channels (BK or Maxi-K) are activated by cytosolic Ca2+ sparks to produce hyperpolarizing spontaneous transient outward currents (STOC) and relax vascular smooth muscle cells (Brenner et al. [Citation2000b]). The pore forming α1-subunit was first cloned in 1993 by Butler and colleagues and further investigations revealed that the voltage- and Ca2+-regulated channel was formed by a tetramer of subunits (Diaz et al. [Citation1998], Knaus et al. [Citation1994a], Knaus et al. [Citation1994b], Meera et al. [Citation1996], Quirk & Reinhart [Citation2001], Wallner et al. [Citation1996]). A regulatory β-subunit was cloned shortly after the discovery of the channel (Knaus et al. [Citation1994c], Knaus et al. [Citation1994c]) and 3 additional family members, β2-4, were subsequently identified (Brenner et al. [Citation2000a], Uebele et al. [Citation2000], Weiger et al. [Citation2000]). Co-assembly of the pore-forming α1 subunit with these β-subunits revealed the mechanisms underlying the previously unexplained tissue specific behaviour of BK channels (Orio et al. [Citation2002]).
Oestrogen is thought to have a beneficial effect on blood pressure via direct actions on the vasculature. The first indication of vascular tone regulation by oestrogen was reported in a study demonstrating that women were less susceptible to vascular disease than men prior to menopause (Stampfer et al. [Citation1991]). The effects of oestrogen on the vascular tissue are multiple: for example, a 40-min exposure of vascular endothelial cells to 17β-oestradiol can induce the expression of up to 250 genes (Pedram et al. [Citation2002]). It has become increasingly evident that BK channels represent a non-genomic oestrogen target. It was first shown that oestrogen relaxed coronary arteries by opening BK channels through a cGMP-dependent mechanism (White et al. [Citation1995]). A more direct and acute effect was shown where 17β-oestradiol increased whole cell current, accelerated current kinetics and increased open probability of BK channels. Moreover, this effect was purported to be directly attributed to oestrogen interaction with the regulatory β1-subunit (Valverde et al. [Citation1999]). However, not all the effects of oestrogens are β subunit dependent. For example, xenoestrogens such as tamoxifen and ethylbromide tamoxifen, also increased the open probability of BK channels in the presence of the β1-subunit, but these drugs also had a β1-subunit independent effect on the unitary conductance of the channel. This would suggest an additional interaction with the pore-forming α subunit (Dick et al. [Citation2001], Dick et al. [Citation2002]). Another β1-subunit-independent effect appears to be the increased proteosomal degradation of the BK channel complex, specifically induced by oestrogen treatment (Korovkina et al. [Citation2004]).
The physiological importance of the regulation of BK channels by β1 was demonstrated in β1-subunit knockout mice. Vascular smooth muscle cells isolated from the transgenic mice displayed a reduction in functional coupling of calcium sparks to BK channel activation (STOCS) and a consequential increase in blood pressure (Brenner et al. [Citation2000b], Dick & Sanders, [Citation2001]). The β1 knockout mouse has also been used to demonstrate the requirement of the accessory subunit for oestrogen and xenoestrogen activation of BK channels (Brenner et al. [Citation2000b], Dick & Sanders [Citation2001]). Mice lacking the pore-forming α1-subunit entirely, BK(-/-), also display elevated blood pressure (Sausbier et al. [Citation2005]). Furthermore, a gain-of-function mutation in the regulatory β1-subunit, E65K, that results in increased Ca2+-dependent activation of the channel appears to protect against diastolic hypertension in humans (Fernandez-Fernandez et al. [Citation2004]).
The present study investigated the effect of 17β-oestradiol on BK channel activity at the single channel level in isolation from the genomic and cellular effects of oestrogen. Recordings were done in planar lipid bilayers with pore-forming α1-subunits in isolation or complexed with the regulatory β1-subunit(s). The Ca2+ sensitivity of the 17β-oestradiol effects was also investigated.
Materials and methods
Molecular biology and cell culture
The subunit stop codons in constructs pcDNA6-hSloα1 (as used by (Lippiat et al. [Citation2000]) and pcDNA3.1-hSloβ1 (J.D. Lippiat, unpublished) were mutated using the Quikchange site-directed mutagenesis kit (Stratagene) to introduce an in-frame vector sequence encoding V5-His6 and myc-His6 epitope tags, respectively. HEK cells were cultured as described previously (Lippiat et al. [Citation2000]) and transfected with pcDNA6-hSloα1-V5 with Fugene 6 reagent, according to the manufacturer's instructions. Clones that grew in medium supplemented with 5 µg/ml blasticidin (Invitrogen) were isolated and a line stably expressing the epitope-tagged BKCa channels was confirmed by electrophysiology. A subculture was transfected with pcDNA3.1-hSloβ1-myc-His6 and a double stable was generated by selection in medium containing 1 mg/ml G418 (Invitrogen) and 5 µg/ml blasticidin. A line stably co-expressing hSloα-V5-His6 and hSloβ1-myc-His6 was characterized by electrophysiology and western blot analysis (anti-V5-HRP and anti-myc-HRP, Invitrogen). Cells were burst with nitrogen cavitation at 1500p.s.i (Taylor et al. [Citation2001]) and membrane fractions purified by sucrose density centrifugation and resuspended membranes directly used for bilayer recordings (Lever [Citation1977]).
Electrophysiology and planar lipid bilayer recording
Single channel currents were measured at holding voltages from −100 mV to +100 mV. Planar lipid bilayer recordings were carried out as described previously (Hardy et al. [Citation2001]). Briefly, planar lipid bilayers were formed with 1:1 palmitoyl-oleoyl-phosphatidylethanolamine (POPE, Avanti Polar Lipids) and palmitoyl-oleoyl-phosatidylserine (POPS, Avanti Polar Lipids) at 15 mg/ml suspended in n-decane, which was drawn across a 0.25 mm diameter hole in a polystyrene cup separating two solution filled chambers, designated cis and trans. The cis chamber (to which membrane proteins and drugs were added) was grounded. Currents were recorded on a Warner PL501A amplifier, sampled at 10 KHz and filtered at 2 KHz. Only bilayers that had a conductance of less than 10 pS and an initial capacitance of at least 150 pF were used. Unless otherwise stated, bilayers were bathed in symmetrical solutions containing 150 mM KCl; 10 mM HEPES; pH7.2, 1 mM EGTA, 1mM MgCl2 and 1.05 mM or 0.75 mM CaCl2 to yield 50 µM (designated ‘high’) or 0.5 µM (‘low’) free calcium. All recordings were therefore made at [Ca2+] where the β1-subunit is catalytically coupled to the BK channel, i.e., [Ca2+] of = 300 nM in order to eliminate additional zero Ca2+ gating effects (Meera et al. [Citation1996], Nimigean & Magleby [Citation2000]). 17β-Oestradiol and 17α-Oestradiol were from Sigma and prepared in DMSO.
Analysis
Recordings (30–600 s) were analysed off line using WinEDR v2.3.9 software (Strathclyde electrophysiological software). Open probability (Po) vs. voltage (V) plots derived from single-channel data were fitted with a Boltzmann function:where V0.5 is the voltage of half-maximal activation, and k is the voltage dependence of the activation process in mV per e-fold change.
Maximum current amplitudes were determined from the peaks of Gaussian functions fitted to amplitude histograms, and for single-channel recordings, integration of these histograms yielded the probability of a channel being open (Po). Event transitions were detected by setting a threshold at 50% of the maximum current amplitude or 50% of any subconductance amplitude if subconductance states were present in the recording. Channel life times were calculated from exponential fits to dwell time histograms and plotted as a Log-Square root plot. All data shown are the mean±SEM.
Burst Analysis was performed with a tc (time value used to distinguish between intra-burst and inter-burst closed intervals) of 20 ms using WinEDR 2.3.9 software (Carson et al. [Citation1995]). This time was derived from analysis of single channel closed time histograms. Closures greater than 20 ms were considered to define gaps between bursts. In experiments where recordings contained multiple channel openings (≤ 3) only bursts with no superimposed openings were included in the analysis. There was no significant difference between burst durations derived from one active channel and those determined from recordings with more than one active channel.
Bionomial and Bayes theorem was used to calculate the distribution of subunit stoichiometry. The Wilcoxon matched pair test (non-parametric) was used to compare values when data did not display a normal distribution. A statistically significant difference was indicated where p<0.05. Non-linear regression and statistical analyses were done using the GraphPad Prism 4 program.
Results
Properties of recombinant α1-subunits
Single channel currents were recorded for the pore forming α1-subunit () at holding voltages from −100 mV to 100 mV in the presence of 50 µM (filled symbols) or 0.5 µM (open symbols) free Ca2+. The channel open probability, Po, was plotted against the membrane potential (mV) and is shown in A (50 µM free Ca2+, filled squares, 0.5 µM free Ca2+, open squares, n=4). Recombinant α1-subunits displayed typical characteristic features of native BK channels – channel conductance was 271±4 pS, (n=4) and the Po increased in response to depolarization and elevated [Ca2+].
Figure 1. Activation properties of BK channels in bilayers. (A) The effect of transmembrane potential on the open probability of a single BK channel (α1 alone) in the presence of low (0.50 µM, □) or high (50 µM, ▪) free calcium (n=4 for each). (B) The effect of transmembrane potential on the open probability of α1+β1-subunit(s) complexes in the presence of high (50 µM, •) free calcium (n=4). A second channel species with BK channel alone characteristics can also be observed (n=4, X)
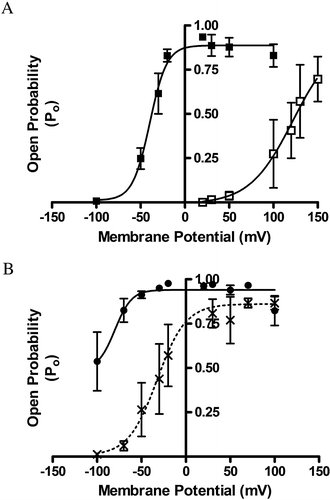
Fitting a Boltzmann function to the Po vs voltage curve for single channel recordings in 50 µM Ca2+ yielded a maximum open probability, Pmax of 0.89±0.03 (n=4); a half maximal activation, V0.5 of −40 mV±3 mV and a slope (k) of 9.6±2 mV. In 0.5 µM Ca2+ the data yielded a Pmax of 0.93±0.16; a V0.5 of 122±53 mV and k of 24±4 mV. A reduced slope reflected the subtle change in the channel gating charge at low Ca2+ levels. The change of ∼100 mV in the V0.5 between the low and high [Ca2+] was observed, which is characteristic of this channel.
Co-expression of the regulatory β1-subunits alters α1-subunit channel characteristics
Next, single channel currents were recorded from bilayers treated with membranes purified from HEK cells expressing both the pore forming α1 and β1-subunits, α1+β1, in the presence of 50 µM Ca2+ (B, filled circles, n=4). Fitting a Boltzmann function to the Po vs voltage curve for single channel recordings yielded a Pmax of 0.921±0.02; a V0.5 of −100±4 mV and a slope (k) of 14.9±4.8 mV. The presence of the β1-subunit increased the half activation voltage by ∼60 mV in high Ca2+ (V0.5=−100±4) and ∼ 80 mV in low Ca2+ (V0.5=−40±3) as compared to α1-subunits alone (A, filled squares) while the single channel conductance remained unaffected (264±5.7 pS, n=4, for α1+β1).
Not all the channels from purified membranes from HEK cells expressing both the pore-forming α1-subunits and β1-subunits displayed increased voltage sensitivity. A subset of channels analysed displayed similar characteristics to the α1-subunit expressed alone. At high Ca2+, this channel population displayed a Pmax (0.861±0.05), V0.5 (32.7±5.8 mV) and slope (16.3±6.2 mV), similar to the α1-subunit expressed alone, and clearly distinguishable from the more active species (B, dotted line, crosses, n=4). The results indicate two populations of channel – one with α1-alone characteristics and one with α1+β1 characteristics.
17β-oestradiol increases BK channel open probability when associated with the β1-subunits
The effect of 17β-oestradiol (5 µM) on the gating characteristics of α1-subunits was determined at high and low calcium, in the presence or absence of the regulatory β1-subunit(s). The membrane voltage was held at 50 mV and recordings lasted for 300 s, with 5 µM 17β-oestradiol added for the latter 150 s. In low calcium, voltage steps to 100 mV were always preformed prior to commencement of the recordings for membrane preparations expressing both α1+β1-subunits. This would confirm that the channel had increased activity and is therefore associated with β1-subunit(s). Channels comprised of α1-subunits alone have very low activity at 50 mV in low Ca2+. In high calcium, voltage steps to −50 mV were performed to distinguish between the two channel populations. Only channel complexes with α1+β1 kinetics were used further.
a demonstrates the effects of 17β-oestradiol on BK channels comprising both the pore-forming and regulatory subunits at low calcium concentration. Further analysis indicated that 17β-oestradiol significantly increased the open probability of the BK channel in membrane preparations expressing both α1+β1-subunit(s), but at low [Ca2+] only (b, open circles). NPo was increased from 0.14±0.04 to 0.23±0.082 (n=6, p<0.03, Wilcoxon matched paired test). 17β-oestradiol increased Po in only four of the seven recordings from channels displaying typical α1+β1 kinetics. All seven recordings were included in statistical analysis of the data. There was no significant change in NPo for α1+β1-subunit(s) in high [Ca2+]: 2.1±0.2 before, 1.9±0.2 after addition of 17β-oestradiol (n=5) or α1-subunits alone at low [Ca2+]: 0.021±0.006 before, 0.0204±0.005 after addition of 17β-oestradiol (b, open squares, n=6), or α1 alone at high [Ca2+]: 0.78±0.16 before, 0.7±0.08 after addition of 17β-oestradiol (n=7). Single channel conductance were unaffected by 17β-oestradiol under all experimental conditions tested (273±8 pS, n=5 each, data not shown). The stereoisomer 17α-oestradiol did not change open probability of the BK channel in membrane preparations expressing both α1+β1-subunit(s), demonstrating a stereospecific as well as a subunit dependent activation of the channel. In contrast, c demonstrates that at high [Ca2+], 17β-oestradiol had no effect on channels comprising the α1-subunit alone or the complex of α1+β1-subunits.
Figure 2. 17β-oestradiol activates BK channels when complexed with regulatory β1 subunits at low [Ca2+]. (A) A trace of three channel complexes of both α1 and β1-subunits, held at −50mV in the presence of 0.5µM Ca2+, shows an increase in opening following 17β-oestradiol addition. (B) Changes in open probability of α1-subunits alone (□) or α1+β1-subunits; (○) following the addition of 5 µM 17β-oestradiol at 150 s in the presence of 0.5µM free Ca2+ (n=−6, p < 0.03, Wilcoxon matched paired test). (C) Changes in open probability of α1-subunits alone (▪) or α1+β1-subunits; (•) following the addition of 5 µM 17β-oestradiol at 150 s in the presence of 50µM free Ca2+.
![Figure 2. 17β-oestradiol activates BK channels when complexed with regulatory β1 subunits at low [Ca2+]. (A) A trace of three channel complexes of both α1 and β1-subunits, held at −50mV in the presence of 0.5µM Ca2+, shows an increase in opening following 17β-oestradiol addition. (B) Changes in open probability of α1-subunits alone (□) or α1+β1-subunits; (○) following the addition of 5 µM 17β-oestradiol at 150 s in the presence of 0.5µM free Ca2+ (n=−6, p < 0.03, Wilcoxon matched paired test). (C) Changes in open probability of α1-subunits alone (▪) or α1+β1-subunits; (•) following the addition of 5 µM 17β-oestradiol at 150 s in the presence of 50µM free Ca2+.](/cms/asset/3adfb535-b9fb-4b48-9bad-3a089fb67a7b/imbc_a_180224_f0002_b.gif)
Subunit stoichiometry calculations
To further investigate the apparent differential effect of 17β-oestradiol stimulation on β1-subunit containing channel complexes, bionomial and Bayes theorem was used to calculate the distribution of subunit stoichiometry. All channels were assumed to contain four possible β1-subunit binding sites and β1-subunit association with pore forming α1-subunits were assumed to be similar and independent, and therefore follow a distribution as a Binomial (4, Φ).
Of a total of 53 recordings from membranes purified from cells co-expressing both α1 and β1-subunits, 15 were identified as α1 channels alone according to their voltage sensitivity profile, and 38 were α1+β1 channel complexes. If inference is carried out using maximum likelihood, the association ratio will be reflected byand the probability of β1-subunit association in our recombinant expression system would be
Using this ratio in the Bayes theorem, it gives the probability of there being 1, 2, 3 or 4 β1-subunits associated with α1 channel complexes to be 0.587, 0.325, 0.080 or 0.007 respectively.
Only channel complexes with α1+β1 voltage profiles were selected for oestrogen experiments; in other words, channels containing at least one β1-subunit. Since only 4 out of 7 recordings showed stimulation by oestrogen, a threshold value of more than one β1-subunit for oestrogen binding can be assumed. The probability of the threshold being 2 or more, 3 or more or 4 β1-subunit(s) is therefore 0.992, 0.007 or 0.001, respectively.
It is therefore most likely that association of two β1-subunits with a pore-forming α1 tetramer is necessary and also sufficient to confer sensitivity to 17β-oestradiol.
17β-oestradiol increases burst duration in α1+β1 channel complexes
The effect of 17β-oestradiol on single channels displaying α1+β1 kinetics was investigated. As before, base-line single channel recordings of 150 s were made before the addition of 5 µM 17β-oestradiol, and then currents were recorded for a further 150 s. Amplitude histograms were fitted by a Gaussian distribution and dwell-time distributions fitted by exponentials.
Single α1+β1 channel open times at 50 mV in low Ca2+ was described by a single exponential with a time constant τ of 7.5±0.5 ms (n=4, A). The channel open distribution remained unchanged by the addition of 17β-oestradiol (τ=7.3±0.5). The closed distribution time for a single α1+β1 was fitted by 3 exponentials (τ1=1.2±0.1 s, τ2=2±0.6 ms, τ3=0.2±0.03 ms, B). This would reflect two short-lived states within bursts and one long closed period between bursts.
Figure 3. 17β-oestradiol enhances channel open probability by increasing burst duration. The single channel open distribution times (A) and closed distribution times (B) of BK α1+β1 subunits in low Ca2+ plus 5 µM 17β-oestradiol is similar to untreated samples (data not shown). Burst analysis (E) before (C) and after (D) the addition of 5 µM 17β-oestradiol (n=6; *p<0.05, two tailed paired t-test).
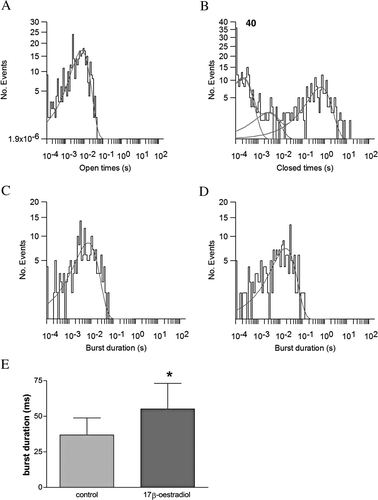
Burst analysis on multiple recordings showed that 17β-oestradiol decreased the duration of the long closed times (τ1) in 3 of the 6 recordings and significantly increased burst duration after the addition of 17β-oestradiol (C, no 17β-oestradiol, τ1=6.7±0.6 ms and D, +17β-oestradiol, τ1=17±1.8 ms; E, n=6, p < 0.05, two tailed paired t-test).
17β-oestradiol induces a subconductance state in α1+β1 channel complexes
We found that the addition of 17β-oestradiol to α1+ β1 channel complexes induced a subconductance state (D). 17 β-oestradiol induced subconductance states in 2 out of 7 recordings with α+β1 in low calcium and also in 1 out of 5 recordings made in high calcium. No subconductance states were observed prior to the addition of oestrogen or for α1-channels alone. The mean subconductance current from these recordings 9.5pA (±0.31) was 70% of the observed full unitary current (13.6pA±0.83). Subconductance states have been previously reported for BK channels (Bello & Magleby [Citation1998], Nimigean & Magleby [Citation1999]). An all-points amplitude histogram shows the full openings only (16 pA for one channel and 32 pA for two channels, A) before the addition of 17β-oestradiol and the induction of new current amplitude following the addition of 17β-oestradiol (11 pA for one level and 23 pA for two channels, B). The 17β-oestradiol-induced subconductance state was thus approximately 75% of the full opening and appeared similar to the subconductance state reported previously for the α1-subunit channel alone (Nimigean & Magleby [Citation1999]). The appearance of the α1+β1 subconductance state was only observed in the presence of 17β-oestradiol and also occurred at high [Ca2+]. This subconductance state could be entered into from both long closed and open states as well as from within bursts (E). This state was therefore assessable from most gating states of the channel. The combined time spent by channels gating in the subconductance state was 2.0% of all recordings of α1+β1 channels in the presence of 17β-oestradiol. This is comparable with 1.1% for α1 subunits alone observed by Nimigean and Magleby ([Citation1999]), although recordings were taken at a different voltage and [Ca2+]i.
Figure 4. 17β-oestradiol induces a subconductance state in BK α1+β1 channel complexes. An amplitude histogram from a control-recording (A) 150 seconds, and after the addition of 5 µM 17β-oestradiol (B) 150 s. Equivalent channel traces are shown below (C) and (D). Subconductance states are accessible from both open and closed states (E). This subconductance state was observed in 25% of the recordings, but only after 17β-oestradiol addition.
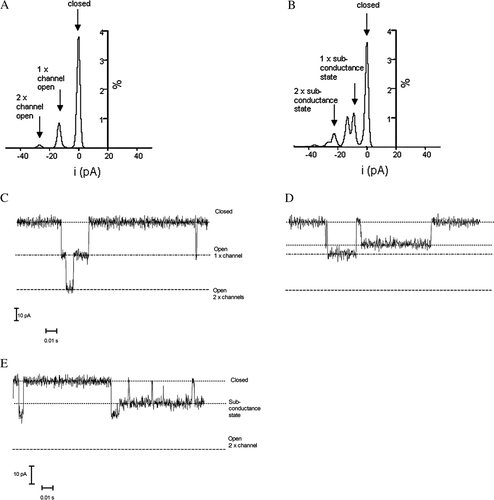
In summary, we have demonstrated that recombinant pore forming α1-subunits can associate with co-expressed β1-subunits to form a functional active channel complex. These α1+β1 channel complexes are activated by the addition of 17β-oestradiol in a Ca2+ dependent manner. Not all α1+β1 channel complexes were activated by 17β-oestradiol. Statistical analysis suggests that association of two β1-subunits with the pore forming α1 tetramer is necessary and sufficient to bind 17β-oestradiol. Single channel analysis revealed that 17β-oestradiol increased channel open probability by increasing burst duration. A Ca2+ independent subconductance state was also observed after the addition of 17β-oestradiol to α1+β1 channel complexes.
Discussion
Regulation of vascular tone by 17β-oestradiol has been linked to the BK channel and in particular, to the regulatory β1-subunit. The β1-subunit is most abundantly expressed in smooth muscle where association with the pore forming α1-subunit increases both voltage and Ca2+ sensitivity (Dworetzky et al. [Citation1996], Tseng-Crank et al. [Citation1996]). The effect of oestrogen on BK channels in vivo will be expected to be a combination of genomic regulation (down regulation by proteosomal degradation) (Korovkina et al. [Citation2004]) and a direct acute interaction with the α1+β1 channel complex. It is therefore important to fully understand the effects of 17β-oestradiol on the channel in isolation, before dissecting the complex in vivo effect on smooth muscle function. Here we report the effects of 17β-oestradiol on recombinant human BK channels studied in artificial planar lipid bilayers, isolated from cellular processes that could regulate protein trafficking or conductance properties.
Recombinantly expressed pore-forming α1-subunits were able to form active channel complexes when reconstituted into artificial bilayers and responded similarly to native channels to changes in membrane potential and [Ca2+]. The previously described inverse relationship of the durations of adjacent open and shut intervals was also observed (McManus et al. [Citation1985]). Co-expression with the regulatory β1-subunit resulted in both increased voltage sensitivity and open probabilities, similar to channels expressed in HEK cells (Lippiat et al. [Citation2003]). Two channel populations with different voltage sensitivities were discernible in purified membrane from HEK cells that co-expressed both subunits. One was similar to α1-subunits alone and one similar to the α1+β1-subunit(s) channel complex. No channels with intermediate properties were observed and the β1-subunit effect on the BK channel appeared to be an all-or-none phenomenon. This was in agreement with reports that functional BK channels can be formed with less than four β-subunits and that β1-subunits had an all-or-none effect on the voltage dependence of α1 gating (Jones et al. [Citation1999], Wang et al. [Citation2002]). This is in contrast with the related β2-subunit that gives an incremental effect as each subunit associates with the α1 tetramer (Jones et al. [Citation1999], Wang et al. [Citation2002]). When membranes purified from cells expressing both α1 and β1 subunits were analysed for channel activity, we observed a 1:3 ratio of channels with kinetics resembling those comprising α1 alone to α1+β1.
Using channels that exclusively exhibited kinetics corresponding to α1+β1 assemblies, we confirmed that 17β-oestradiol only activated BK channels at the lower [Ca2+]. In agreement with previous studies, the activation is small but consistent (Valverde et al. [Citation1999]). Taking the large conductance of this channel into account and the high input resistance of smooth muscle cells, even a small change in channel open probability could have a large impact on resting membrane potential. The lack of effect at +50mV in the higher [Ca2+] is likely to be due to the channel existing near its maximum PO, and 17β-oestradiol being unable to increase this further.
Interestingly, only 4 of the 7 channels purified and reconstituted from cells expressing α1+β1 subunits were activated by 17β-oestradiol. If one assumed that a minimum of one β1-subunit is necessary for the observed increase in voltage sensitivity, and that the channels existed in the full range of α1+β1 stoichiometries according to a binomial distribution, our results suggest that at least 2 or more β1-subunits are required for modulation by 17β-oestradiol. A ratio of 1:3 of α1 alone to α1+β1 channel complex was found for subunits in membranes purified from our recombinant expression system. We calculated the number of β1 subunits most likely needed to co-assemble with the α1 tetramer to confer activation by oestrogen to be two. This ‘subunit threshold’ of two β1 subunits for 17β-oestradiol binding could explain some of the contradictory reports on the effects of 17β-oestradiol seen in whole cell recordings and giant patches, where the total response of a mixed population would be recorded.
Single channel analysis of α1+β1 channel complexes showed 17β-oestradiol enhanced open probability of the channel by increasing the duration of bursts of openings. β1-subunits have been shown to increase channel open probability by increasing the time of bursting states (Nimigean & Magleby [Citation2000]). It therefore appears that the addition of 17β-oestradiol enhances this β1-subunit effect.
17β-oestradiol also induced a Ca2+ independent subconductance state that was 75% of the full open amplitude in the α1+β1 channel complexes. A 75% subconductance state has previously been reported for the α1 channel and was no longer observed upon association with the β1-subunit (Nimigean & Magleby [Citation1999]). As channel activation by the β1-subunit is mediated through allosteric coupling with the channel gating mechanism, this re-emergence of a subconductance state could indicate functional, but not necessarily physical, uncoupling of β1-subunit and pore forming α1-subunits in presence of 17β-oestradiol (Niu et al. [Citation2004], Qian & Magleby [Citation2003]).
In summary, we report a detailed single channel analysis of the interaction of 17β-oestradiol with human BK channels reconstituted into lipid bilayers. We demonstrate that 17β-oestradiol activates BK channels only in the presence of a regulatory β1-subunit at low [Ca2+]. 17β-oestradiol needs the presence of a minimum of at least two β1-subunits associated with the pore forming α1 tetramer to activate the channel complex and statistical analysis indicates that only two subunits is needed for binding. Single channel analysis revealed that increased open probabilities are the result of increased burst duration. Coupling between the two subunits are affected by 17β-oestradiol and a 75% subconductance state is seen after the addition of oestrogen.
This work was funded by a Human Frontiers Scientific Program awarded to RC. HdW is a Wellcome Trust Training Fellow funded through the OXION initiative and would like to thank Dr A Pintore for helpful discussion with the statistical analysis.
References
- Bello RA, Magleby KL. Time-irreversible subconductance gating associated with Ba2+ block of large conductance Ca2+-activated K+ channels. J Gen Physiol 1998; 111: 343–362
- Brenner R, Jegla TJ, Wickenden A, Liu Y, Aldrich RW. Cloning and functional characterization of novel large conductance calcium-activated potassium channel beta subunits, hKCNMB3 and hKCNMB4. J Biol Chem 2000a; 275: 6453–6461
- Brenner R, Perez GJ, Bonev AD, Eckman DM, Kosek JC, Wiler SW, Patterson AJ, Nelson MT, Aldrich RW. Vasoregulation by the beta1 subunit of the calcium-activated potassium channel. Nature 2000b; 407: 870–876
- Carson MR, Travis SM, Welsh MJ. The two nucleotide-binding domains of cystic fibrosis transmembrane conductance regulator (CFTR) have distinct functions in controlling channel activity. J Biol Chem 1995; 270: 1711–1717
- Diaz L, Meera P, Amigo J, Stefani E, Alvarez O, Toro L, Latorre R. Role of the S4 segment in a voltage-dependent calcium-sensitive potassium (hSlo) channel. J Biol Chem 1998; 273: 32430–32436
- Dick GM, Hunter AC, Sanders KM. Ethylbromide tamoxifen, a membrane-impermeant antiestrogen, activates smooth muscle calcium-activated large-conductance potassium channels from the extracellular side. Mol Pharmacol 2002; 61: 1105–1113
- Dick GM, Rossow CF, Smirnov S, Horowitz B, Sanders KM. Tamoxifen activates smooth muscle BK channels through the regulatory beta 1 subunit. J Biol Chem 2001; 276: 34594–34599
- Dick GM, Sanders KM. Xeno)estrogen sensitivity of smooth muscle BK channels conferred by the regulatory beta1 subunit: a study of beta1 knockout mice. J Biol Chem 2001; 276: 44835–44840
- Dworetzky SI, Boissard CG, Lum-Ragan JT, McKay MC, Post-Munson DJ, Trojnacki JT, Chang CP, Gribkoff VK. Phenotypic alteration of a human BK (hSlo) channel by hSlobeta subunit coexpression: changes in blocker sensitivity, activation/relaxation and inactivation kinetics, and protein kinase A modulation. J Neurosci 1996; 16: 4543–4550
- Fernandez-Fernandez JM, Tomas M, Vazquez E, Orio P, Latorre R, Senti M, Marrugat J, Valverde MA. Gain-of-function mutation in the KCNMB1 potassium channel subunit is associated with low prevalence of diastolic hypertension. J Clin Invest 2004; 113: 1032–1039
- Hardy SP, Ritchie C, Allen MC, Ashley RH, Granum PE. Clostridium perfringens type A enterotoxin forms mepacrine-sensitive pores in pure phospholipid bilayers in the absence of putative receptor proteins. Biochim Biophys Acta 2001; 1515: 38–43
- Jones EM, Gray-Keller M, Fettiplace R. The role of Ca2+-activated K+ channel spliced variants in the tonotopic organization of the turtle cochlea. J Physiol 1999; 518(Pt 3)653–665
- Knaus HG, Eberhart A, Glossmann H, Munujos P, Kaczorowski GJ, Garcia ML. Pharmacology and structure of high conductance calcium-activated potassium channels. Cell Signal 1994a; 6: 861–870
- Knaus HG, Eberhart A, Kaczorowski GJ, Garcia ML. Covalent attachment of charybdotoxin to the beta-subunit of the high conductance Ca(2+)-activated K+ channel. Identification of the site of incorporation and implications for channel topology. J Biol Chem 1994b; 269: 23336–23341
- Knaus HG, Folander K, Garcia-Calvo M, Garcia ML, Kaczorowski GJ, Smith M, Swanson R. Primary sequence and immunological characterization of beta-subunit of high conductance Ca(2+)-activated K+ channel from smooth muscle. J Biol Chem 1994c; 269: 17274–17278
- Korovkina VP, Brainard AM, Ismail P, Schmidt TJ, England SK. Estradiol binding to maxi-K channels induces their down-regulation via proteasomal degradation. J Biol Chem 2004; 279: 1217–1223
- Lever JE. Active amino acid transport in plasma membrane vesicles from Simian virus 40-transformed mouse fibroblasts. Characteristics of electrochemical Na+ gradient-stimulated uptake. J Biol Chem 1977; 252: 1990–1997
- Lippiat JD, Standen NB, Davies NW. A residue in the intracellular vestibule of the pore is critical for gating and permeation in Ca2+-activated K+ (BKCa) channels. J Physiol 529 Pt 2000; 1: 131–138
- Lippiat JD, Standen NB, Harrow ID, Phillips SC, Davies NW. Properties of BK(Ca) channels formed by bicistronic expression of hSloalpha and beta1-4 subunits in HEK293 cells. J Membr Biol 2003; 192: 141–148
- McManus OB, Blatz AL, Magleby KL. Inverse relationship of the durations of adjacent open and shut intervals for C1 and K channels. Nature 1985; 317: 625–627
- Meera P, Wallner M, Jiang Z, Toro L. A calcium switch for the functional coupling between alpha (hslo) and beta subunits (KV,Ca beta) of maxi K channels. FEBS Lett 1996; 382: 84–88
- Nimigean CM, Magleby KL. The beta subunit increases the Ca2+ sensitivity of large conductance Ca2+-activated potassium channels by retaining the gating in the bursting states. J Gen Physiol 1999; 113: 425–440
- Nimigean CM, Magleby KL. Functional coupling of the beta(1) subunit to the large conductance Ca(2+)-activated K(+) channel in the absence of Ca(2+). Increased Ca(2+) sensitivity from a Ca(2+)-independent mechanism. J Gen Physiol 2000; 115: 719–736
- Niu X, Qian X, Magleby KL. Linker-gating ring complex as passive spring and Ca(2+)-dependent machine for a voltage- and Ca(2+)-activated potassium channel. Neuron 2004; 42: 745–756
- Orio P, Rojas P, Ferreira G, Latorre R. New disguises for an old channel: MaxiK channel beta-subunits. News Physiol Sci 2002; 17: 156–161
- Pedram A, Razandi M, Aitkenhead M, Hughes CC, Levin ER. Integration of the non-genomic and genomic actions of estrogen. Membrane-initiated signaling by steroid to transcription and cell biology. J Biol Chem 2002; 277: 50768–50775
- Qian X, Magleby KL. Beta1 subunits facilitate gating of BK channels by acting through the Ca2+, but not the Mg2+, activating mechanisms. Proc Natl Acad Sci USA 2003; 100: 10061–10066
- Quirk JC, Reinhart PH. Identification of a novel tetramerization domain in large conductance K(ca) channels. Neuron 2001; 32: 13–23
- Sausbier M, Arntz C, Bucurenciu I, Zhao H, Zhou XB, Sausbier U, Feil S, Kamm S, Essin K, Sailer CA, Abdullah U, Krippeit-Drews P, Feil R, Hofmann F, Knaus HG, Kenyon C, Shipston MJ, Storm JF, Neuhuber W, Korth M, Schubert R, Gollasch M, Ruth P. Elevated blood pressure linked to primary hyperaldosteronism and impaired vasodilation in BK channel-deficient mice. Circulation 2005; 112: 60–68
- Stampfer MJ, Colditz GA, Willett WC, Manson JE, Rosner B, Speizer FE, Hennekens CH. Postmenopausal estrogen therapy and cardiovascular disease. Ten-year follow-up from the nurses' health study. N Engl J Med 1991; 325: 756–762
- Taylor AM, Storm J, Soceneantu L, Linton KJ, Gabriel M, Martin C, Woodhouse J, Blott E, Higgins CF, Callaghan R. Detailed characterization of cysteine-less P-glycoprotein reveals subtle pharmacological differences in function from wild-type protein. Br J Pharmacol 2001; 134: 1609–1618
- Tseng-Crank J, Godinot N, Johansen TE, Ahring PK, Strobaek D, Mertz R, Foster CD, Olesen SP, Reinhart PH. Cloning, expression, and distribution of a Ca(2+)-activated K+ channel beta-subunit from human brain. Proc Natl Acad Sci USA 1996; 93: 9200–9205
- Uebele VN, Lagrutta A, Wade T, Figueroa DJ, Liu Y, McKenna E, Austin CP, Bennett PB, Swanson R. Cloning and functional expression of two families of beta-subunits of the large conductance calcium-activated K+ channel. J Biol Chem 2000; 275: 23211–23218
- Valverde MA, Rojas P, Amigo J, Cosmelli D, Orio P, Bahamonde MI, Mann GE, Vergara C, Latorre R. Acute activation of Maxi-K channels (hSlo) by estradiol binding to the beta subunit. Science 1999; 285: 1929–1931
- Wallner M, Meera P, Toro L. Determinant for beta-subunit regulation in high-conductance voltage-activated and Ca(2+)-sensitive K+ channels: an additional transmembrane region at the N terminus. Proc Natl Acad Sci USA 1996; 93: 14922–14927
- Wang YW, Ding JP, Xia XM, Lingle CJ. Consequences of the stoichiometry of Slo1 alpha and auxiliary beta subunits on functional properties of large-conductance Ca2+-activated K+ channels. J Neurosci 2002; 22: 1550–1561
- Weiger TM, Holmqvist MH, Levitan IB, Clark FT, Sprague S, Huang WJ, Ge P, Wang C, Lawson D, Jurman ME, Glucksmann MA, Silos-Santiago I, DiStefano PS, Curtis R. A novel nervous system beta subunit that downregulates human large conductance calcium-dependent potassium channels. J Neurosci 2000; 20: 3563–3570
- White RE, Darkow DJ, Lang JL. Estrogen relaxes coronary arteries by opening BKCa channels through a cGMP-dependent mechanism. Circ Res 1995; 77: 936–942