Abstract
ADP-ribosylation factor related protein 1 (ARFRP1) is a member of the ARF-family of GTPases which operate as molecular switches in the regulation of intracellular protein traffic. Deletion of the mouse Arfrp1 gene leads to embryonic lethality during early gastrulation, suggesting that ARFRP1 is required for cell adhesion-related processes. Here we show that ARFRP1 specifically controls targeting of ARL1 and its effector Golgin-245 to the trans-Golgi. GTP-bound ARFRP1 (ARFRP1-Q79L mutant) is associated with Golgi membranes and co-localized with the GTPase ARL1. In contrast, the guanine nucleotide exchange defective ARFRP1 mutant (ARFRP1-T31N) clusters within the cytosol. ARFRP1-T31N or depletion of endogenous ARFRP1 by RNA interference disrupts the Golgi association of ARL1 and of the GRIP-domain protein Golgin-245 and alters the distribution of a trans-Golgi network marker, syntaxin 6. In contrast, the targeting of two other Golgi-associated proteins, GM130 and giantin, was unaffected. Furthermore, in Arfrp1−/ − embryos ARL1 dislocated from Golgi membranes whereas it was associated with intracellular membranes in wild-type embryos. These data suggest that lethality of Arfrp1 knockout embryos is due to a specific disruption of protein targeting, e.g., of ARL1 and Golgin-245, to the Golgi.
Introduction
ARF-related protein 1 (ARFRP1) is a 25 kDa protein which belongs to the ARF family of Ras-related GTPases (33 and 39% of amino acids identical with ARF1 and ARL3, respectively; Schürmann et al. [Citation1995]). Members of the ARF-family have been implicated in a large number of cellular processes, including vesicular membrane traffic, regulation of Golgi function, lipid metabolism, and regulation of the actin cytoskeleton (Kahn [Citation2003]). The more distantly related ARF-like proteins (ARL) have been described to exert such divergent functions as regulating vesicle traffic (ARL1, Sar1), microtubule organization (ARL2) and spermatogenesis (ARL4) (Schürmann & Joost [Citation2003]).
As compared with other members of the family, ARFRP1 exhibits several distinct characteristics. ARFRP1 can hydrolyze GTP in the absence of a GTPase activating protein (GAP), and lacks the site for N-myristoylation (glycine 2) which is required for membrane association (Schürmann et al. [Citation1995]). ARFRP1 appears to be involved in a pathway that can inhibit ARF activities, e.g., activation of PLD (Schürmann et al. [Citation1999]). In a two-hybrid screen, a partial sequence encoding the N-terminus and most of the Sec7 domain of the ARF-specific guanine nucleotide exchange factor cytohesin 1 was isolated with the GTPase-negative mutant ARFRP1-Q79L as bait. The interaction between ARFRP1 and the Sec7 domain of cytohesin was GTP-dependent and resulted in the inhibition of the ARF/Sec7-dependent activation of PLD in vitro and in vivo.
Deletion of Arfrp1 in mice resulted in embryonic lethality during early gastrulation (Mueller et al. [Citation2002]). In these knockout mice, it was shown that Arfrp1−/ − blastocysts implanted in vivo and formed egg-cylinder-stage embryos that appeared normal until day 5. At day 6–6.5, the time of early gastrulation, Arfrp1−/ − embryos exhibited profound alterations of the distal part of the egg cylinder. Rounded pyknotic cells, which were TUNEL-positive and therefore apoptotic, were found within this area of the egg cylinder in the proamniotic cavity, and some of these were only loosely attached to the ectodermal cell layer. This observation suggested that ARFRP1 plays a critical role in processes of adhesion-dependent morphogenesis, cytoskeletal reorganization, and/or development of cell polarity, which are known to occur in early gastrulation (Mueller et al. [Citation2002]).
The closest relative of ARFRP1 in yeast is a protein with 43% identity designated Arl3p (Huang et al. [Citation1999]). Arl3p has been suggested to be involved in protein trafficking within the trans-Golgi network (Huang et al. [Citation1999], Rosenwald et al. [Citation2002]). More recently, it has been shown that Arl3p and Arl1p, a second ARF-like protein, act sequentially to recruit Golgin proteins to Golgi membranes. Golgin proteins are large coiled-coil proteins which have been implicated in Golgi formation and maintenance as well as in vesicular transport. Golgin-97 and Golgin-245, along with GCC88 and GCC185, are peripheral members sharing a C-terminal GRIP domain that mediates their localization to the trans-Golgi. ARL1 has been shown to target GRIP domain proteins to the Golgi apparatus and to bind directly to the GRIP domain in a GTP-dependent manner (Setty et al. [Citation2003], Panic et al. [Citation2003a], Panic et al. [Citation2003b], Lu & Hong [Citation2003]). Setty et al. ([Citation2003]) and Panic et al. ([Citation2003b]) demonstrated recently that in yeast Golgi recruitment of GRIP domain proteins by Arl1p is regulated by Arl3p. Both GDP-Arl3p and GDP-Arl1p are localized in the cytosol, whereas the GTP-bound proteins are associated with Golgi membranes. GTP-Arl3p is required for normal Golgi localization of GTP-Arl1p which consequently interacts specifically with the GRIP domain to recruit it to the membrane (Setty et al. [Citation2003], Panic et al. [Citation2003b]). In addition, recently Behnia et al. ([Citation2004]) and Setty et al. ([Citation2004]) demonstrated that Arl3p is N-terminally acetylated by the NatC N-acetyltransferase and that this modification is necessary for its Golgi localization. Moreover, both groups discovered that Golgi targeting of Arl3p also requires Sys1p, an integral Golgi-localized protein.
In this study, we tested the hypothesis that ARFRP1, like its presumed yeast isologue Arl3p, controls Golgi function. The data suggest that ARFRP1 is required for translocation of ARL1 and Golgin-245 to the trans-Golgi, and that also in vivo, in Arfrp1−/ − embryos correct targeting of ARL1 is disrupted.
Material and methods
Mammalian expression plasmids
ARFRP1-T31N and ARFRP1-Q79L mutants were subcloned into pCMV as described (Schürmann et al. [Citation1999]) or into the pEGFP-N1 vector. ARL1-T31N-GFP and ARL1-Q71L-GFP in pSTAR were described before (Lu et al. [Citation2001]). These constructs were used to transfect HeLa cells, which were incubated with 12 µg/ml doxycyclin at 37°C for 20 h in order to enhance the expression of the protein.
Antibodies
Polyclonal antibody against recombinant GST-ARFRP1 was described before (Schürmann et al. [Citation1995]) and used for immunocytochemistry in a dilution of 1:5000. Affinity purified polyclonal antibody against recombinant ARL1 (1:100; E6P3; 0.3 µg/µl) and antibody against giantin (1:500) were described before (Lowe et al. [Citation1996], Lu & Hong [Citation2003]). For detection of Golgin-245 we used purified mouse anti-p230 trans Golgi (BD Transduction Laboratories, San Diego, USA) in a dilution of 1:30, for detection of syntaxin 6 we used mouse anti-syntaxin 6 (BD Transduction Laboratories, clone 30) in a dilution of 1:50. Antibody against GM130 (1:100) was purchased at BD Transduction Laboratories. Alexa Fluor® 546 F(ab’)2 fragment of goat anti-mouse IgG (H + L) or Alexa Fluor® 488 F(ab’)2 fragment of goat anti mouse IgG (H + L) (Molecular Probes, Eugene, Oregon, USA) were used in a dilution of 1:800 as secondary antibodies.
Cell culture and transfection
HeLa cells were cultured in RPMI with 10% (v/v) FBS. Transient transfections of HeLa cells were performed with Lipofectamine 2000 (Invitrogen, Grand Island, NY, USA) according to manufacturer's protocols.
Immunocytochemistry and indirect immunofluorescence microscopy
At indicated time points cells were washed with PBS and fixed with 99.9% methanol at −20°C for 10 min. Cells were washed with PBS, blocked with PBS/0.1% (v/v) Tween/5% (v/v) normal goat serum for 20 min at room temperature (RT) and incubated with primary antibodies in antibody diluent (Dako, Glostrup, Denmark) for 1 h at RT. After extensive washing with PBS/0.1% (v/v) Tween, cells were incubated with Alexa Fluor® 488 or Alexa Fluor® 546-conjugated secondary antibodies (Molecular Probes) in antibody diluent at RT for 30 min. After washing with PBS/0.1% (v/v) Tween cells were mounted in fluorescent mounting medium (Dako) and analysed with a Leica TCS SP2 Laser Scan inverted microscope. We scanned the cells sequentially with an argon–krypton laser (488 nm) to excite the Alexa488 dye and with a helium–neon laser (543 nm) to excite the Alexa546 dye. The spectral detector recorded light emission at 510–560 nm and 580–660 nm, respectively. We processed images of 1,024×1,024 pixels with Corel PHOTO-PAINT 10.0 (Coral Corporation).
Immunohistochemistry
Paraffin sections of uteri from Arfrp1+/ − females crossed with Arfrp1+/ − males were dewaxed in toluene or acetone and rehydrated, antigen demasking was performed by heat treatment (microwave, 2.5 min, 850 W) in 10 mM Na-citrate, pH 6.0. Endogenous peroxidase was deactivated with 3% hydrogen peroxide for 10 min. Unspecific binding sites were blocked with DAKO antibody diluent for 30 min. A 1:5500 dilution of anti-ARFRP1 rabbit serum and 1:5000 dilution of anti-ARL1 rabbit serum in antibody diluent were applied overnight at 4°C in a humid chamber. Specific antibody binding was visualized by biotin-conjugated donkey anti-rabbit IgG (1:800; Dianova, Hamburg, Germany), for 30 min at 37°C, followed by incubation with a streptavidin-biotin-horseradish peroxidase complex (StreptAB-complex/POD) for 30 min, and diaminobenzidine as substrate according to the manufacturer's specifications. The sections were dehydrated in graded ethanol and toluene and mounted in Hydromount. Microscopic investigation and photo documentation were done with the combined light and fluorescence microscope ECLIPSE E-100 (Nikon, Düsseldorf, Germany) in combination with the video camera CCD_1300CB (Vosskühler, Osnabrück, Germany) and the Analysis System LUCIA (Nikon, Düsseldorf, Germany).
Knockdown of endogenous ARFRP1 by small interference RNA
The mammalian expression vector, pSUPER.basic (OligoEngine) was used for expression of siRNA and targeting human ARFRP1 in HeLa cells. A gene-specific insert which specifies a 19-nucleotide sequence corresponding to nucleotide 691–709 (gtggatggtgaagtgtgtc, Acc. No NM_003224.2, ARFRP1-siRNA) was separated by a 9-nucleotide non-complementary spacer (ttcaagaga) from the reverse complement of the same 19-nucleotide sequence. Both sequences were subcloned into the BglII and HindIII sites of the pSUPER vector and referred to as pSUPER-ARFRP1. As a control, we used a scrambled siRNA in which 3 base pairs (shown in bold letters) were exchanged (CTGTATGGTGAACTGTGTC). HeLa cells were transfected with pSUPER, pSUPER-scrambled or pSUPER-ARFRP1 and processed for Western blot analysis or immunofluorescence after 4–8 days of incubation.
Results
Co-localization of ARFRP1 with ARL1 in Golgi membranes is GTP-dependent
In order to investigate the subcellular localization of GTP and GDP bound ARFRP1, the GTPase-negative (ARFRP1-Q79L) or the guanine nucleotide exchange-defective (ARFRP1-T31N) mutant were overexpressed in HeLa cells as GFP-fusion proteins. As illustrated in (left panels), the GTP-bound ARFRP1 mutant was detected in the perinuclear region while the guanine nucleotide exchange defective ARFRP1 mutant appeared distributed within the cytosol.
Figure 1. Subcellular localization of ARFRP1-GFP and endogenous ARL1 in HeLa cells. HeLa cells were transfected with the cDNA of ARFRP1-Q79L-GFP, ARFRP1-T31N-GFP or ARFRP1-wt-GFP. Cells were fixed with methanol and stained for ARL1 with an affinity purified polyclonal antibody in combination with a TRITC-conjugated secondary antibody. Images of GFP-fluorescence and of TRITC were obtained by confocal laser scanning microscopy as described in Methods. This figure appears in colour in Molecular Membrane Biology online.
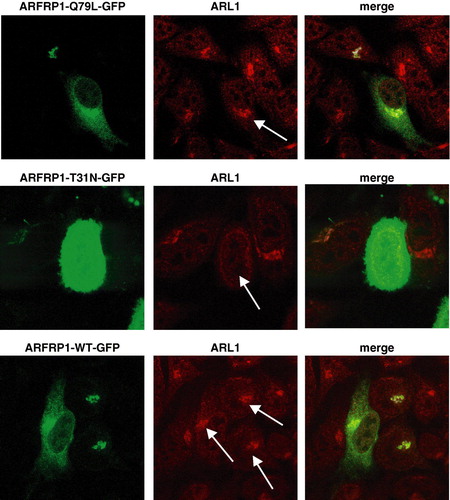
Since the presumed yeast isologue of ARFRP1, Arl3p, has previously been shown to control the Golgi association of the GTPase Arl1p (Setty et al. [Citation2003], Panic et al. [Citation2003b]), we analysed the subcellular distribution of ARL1, the mammalian isologue of Arl1p, in control cells and after overexpression of ARFRP1. As shown in (middle and right panels), GFP-tagged ARFRP1-Q79L partially co-localizes with endogenous ARL1, while the GDP-bound mutant of ARFRP1 (ARFRP1-T31N-GFP) disrupts the Golgi association of ARL1. Quantification of 120 cells 48 h after transfection with pEGFP1-ARFRP1-T31N revealed that 87% of the cells lack visible ARL1 staining at the Golgi. In 14 of the 120 cells in which we detected a Golgi localization of ARL1 the expression of ARFRP-T31N-GFP was very low. These data suggest that ARFRP1, like yeast Arl3p, regulates the subcellular localization of ARL1 in mammalian cells. Wild-type ARFRP1-GFP (lower panel of ) was predominantly associated with Golgi membranes, and co-localized with endogenous ARL1, indicating that the GTPase is predominantly GTP-bound under the conditions of cell culture.
GDP-bound ARFRP1 inhibits the Golgi association of Golgin-245
As we have previously described (Lu & Hong [Citation2003]), GFP-tagged, GTP-bound ARL1 (ARL1-Q71L-GFP) co-localized with Golgin-245 in Golgi membranes, whereas ARL1-T31N-GFP was detected in the cytosol, leading to a dissociation of Golgin-245 from the Golgi (A). The subcellular localization of Golgin-245 was not altered by overexpression of GTP-restricted ARFRP1 (ARFRP1-Q79L). In contrast, expression of GDP-restricted ARFRP1 (ARFRP1-T31N) removed Golgin-245 from the Golgi to the cytosol (B). According to results obtained for ARL1 the quantification of ARFRP1-T31N transfected cells indicated 90% disruption of Golgin-245 from the Golgi. These data indicate that ARFRP1 controls Golgi association of both, ARL1 and Golgin-245.
Figure 2. Subcellular localization of ARL1 (A), ARFRP1 (B), and Golgin-245 (A,B) in HeLa cells. HeLa cells were transfected with the cDNA of (A) ARL1-Q71L-GFP, ARL1-T31N-GFP, or (B) ARFRP1-Q79L-GFP or ARFRP1-T31N-GFP. Cells were fixed with methanol and stained for Golgin-245 with an affinity purified polyclonal antibody in combination with an Alexa546-conjugated secondary antibody. Images of GFP-fluorescence and of Alexa546 were obtained by confocal laser scanning microscopy as described in Methods. Arrows depict cells that expressed the respective ARL1 (A) or ARFRP1 (B) construct. This figure appears in colour in Molecular Membrane Biology online.
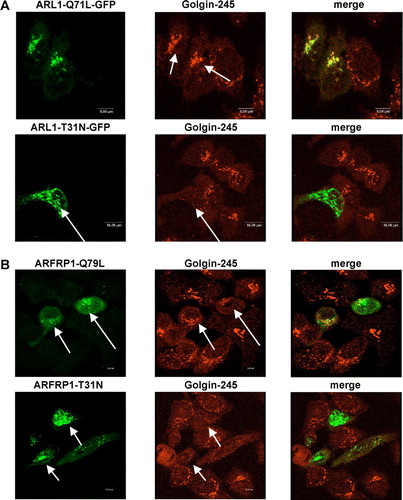
Silencing of ARFRP1 gene in HeLa cells dissociates ARL1 from the trans-Golgi
In order to provide additional evidence for the role of ARFRP1 in recruiting ARL1 to the trans-Golgi, we used the RNA interference method to suppress translation of the endogenous ARFRP1 mRNA. We tested 8 different constructs containing various ARFRP1-specific sequences for their ability to knock-down ARFRP1 by co-expressing the pSUPER-ARFRP1 constructs with ARFRP1-GFP, and found that expression of ARFRP1-GFP was markedly reduced with nucleotides 691–709 (Acc. No. NM_003224.2), whereas other constructs failed to alter the ARFRP1-GFP expression (data not shown). Western blot analysis of cell lysates generated 4, 6 and 8 days after transfection indicates that the endogenous ARFRP1 expression was almost fully suppressed from day 4 to 6 after transfection, but began to recover at day 8 (A).
Figure 3. Silencing of the ARFRP1 gene in HeLa cells disrupts the Golgi association of GTP-bound ARL1. (A) Silencing of endogenous ARFRP1 in HeLa cells by small interference RNA. DNA encoding for small interference RNA (siRNA) targeting the human ARFRP1 gene was subcloned into the pSUPER vector and HeLa cells were transfected with the empty vector (−) or with the pSUPER-ARFRP1 construct (+), cells were lysed 4, 6, or 8 days after transfection and ARFRP1 expression was analysed by Western blotting. (B) Subcellular localization of ARL1 after silencing of ARFRP1 in HeLa cells. HeLa cells were transfected with pSUPER (control) or pSUPER-ARFRP1 (ARFRP1-siRNA). After 4 days, cells were transfected with cDNA of ARL1-Q71L-GFP or ARL1-T31N-GFP. Cells were fixed with methanol and the subcellular localization of the GFP-tagged ARL1 mutants was analyzed by confocal laser scanning microscopy as described in the Methods section. This figure appears in colour in Molecular Membrane Biology online.
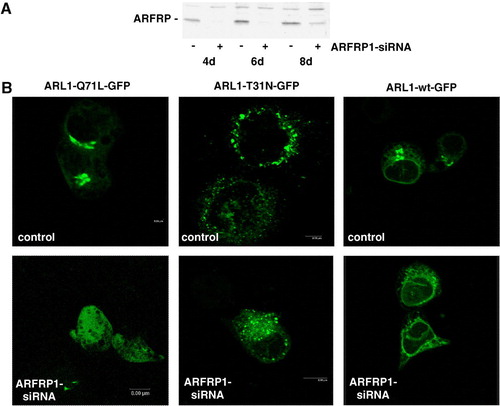
To investigate the subcellular localization of ARL1 and Golgin-245 in the absence of ARFRP1, HeLa cells were first transfected with pSUPER or pSUPER-ARFRP1, and 4 days later with the GFP-tagged ARL1 mutants. In cells transfected with the pSUPER vector (B), ARL1-Q71L-GFP is localized at the Golgi apparatus, whereas it was dissociated from the Golgi in cells transfected with pSUPER-ARFRP1. In contrast, the subcellular localization of the GDP-bound ARL1-mutant appeared unaltered by silencing of endogenous ARFRP1. These results suggest that ARFRP1 is specifically required for the Golgi association of GTP-ARL1.
Silencing of ARFRP1 inhibits the ARL1-dependent targeting of Golgin-245 to the Golgi apparatus
ARL1 is required for targeting GRIP domain proteins to the Golgi apparatus by direct binding to the GRIP domain in a GTP-dependent manner (Lu & Hong [Citation2003], Panic et al. [Citation2003a]). Since we detected a relocation of GTP-bound ARL1 after downregulation of ARFRP1, we next analysed the distribution of Golgin-245, the effector of GTP-ARL1. A shows that endogenous Golgin-245 is co-localized with overexpressed ARL1-Q71L-GFP, and that the knockdown of ARFRP1 caused a relocalization of both ARL1-Q71L-GFP and Golgin-245. These results suggest that ARFRP1 is required for the sequential recruitment of ARL1 and GRIP proteins to Golgi membranes in mammalian cells.
Figure 4. Inhibition of ARFRP1 expression in HeLa cells disrupts Golgi association of ARL1-Q71L (A), and endogenous ARL1 (B) and subsequently of Golgin-245 (A, B). (A) ARFRP1 expression was downregulated in HeLa cells by transfection of pSUPER-ARFRP1. After 4 days, cells were transfected with cDNA of ARL1-Q71L-GFP. Cells were fixed with methanol and stained for Golgin-245 with a polyclonal antibody in combination with an Alexa546-conjugated secondary antibody. (B) ARFRP1 expression was downregulated in HeLa cells by transfection of pSUPER-ARFRP1. Control cells were transfected with scrambled siRNA. Four days after transfection, cells were fixed with methanol, and endogenous ARL1 was stained with an affinity-purified polyclonal anti-ARL1 antibody in combination with an Alexa488-conjugated secondary antibody. Golgin-245 was visualized with a polyclonal anti-Golgin-245 antibody in combination with an Alexa546-conjugated secondary antibody. Subcellular localization of the GFP-tagged ARL1-Q71L, of endogenous ARL1, and Golgin-245 was analysed by confocal laser scanning microscopy as described in the Methods. Arrows depict cells with disrupted Golgi association of ARL1 and Golgin-245.
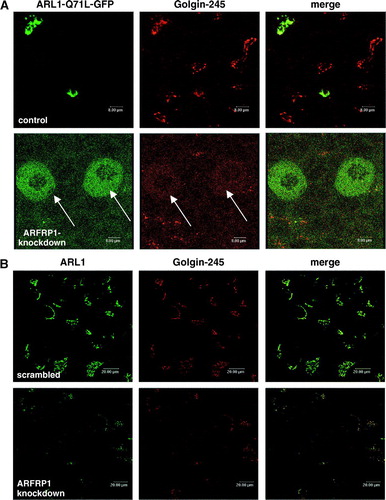
We next analysed the effect of silencing of ARFRP1 on the localization of endogenous ARL1 and its binding partner Golgin-245. In these experiments we used a scrambled siRNA as a control and demonstrated that like in cells transfected with the empty siRNA vector ARL1 and Golgin-245 are co-localized at the trans-Golgi (B). In contrast, in cells lacking ARFRP1, Golgi-association of ARL1 and Golgin-245 is lost (B) suggesting that ARFRP1 is required for the Golgi recruitment of both ARL1 and Golgin-245. These data are consistent with the model of Setty et al. ([Citation2003]) in which the nucleotide cycle of Arl3p regulates the Golgi localization of Arl1p in yeast, and demonstrate that a similar mechanism is operated by ARFRP1 in mammalian cells.
Targeting of ARL1 to the Golgi apparatus is disrupted in Arfrp1−/ − embryos
We next wanted to verify that ARFRP1 is required for the recruitment of ARL1 in cells constitutively expressing the two proteins. Therefore, we analysed the subcellular distribution of ARL1 in wild-type and Arfrp1−/ − embryos. As shown in A, ARFRP1 and ARL1 are co-localized in ectodermal cells of 6.5 day old embryos. In transversal sections of Arfrp1−/ − embryos showing rounded pyknotic cells which are partially released into the proamniotic cavity (Mueller et al. [Citation2002]), no staining for ARL1 could be detected. This result indicates that in-vivo targeting of ARL1 is disrupted in the absence of ARFRP1. In order to be confident that the ARL1 detection was effective in the uterus sections we observed ARL1 staining in fat tissue associated with the uterus. As shown in B, we could detect ARFRP1 and ARL1 in fat cells of the Arfrp1+/ − mother. Both proteins appeared to be co-localized in membrane structures within the fat cells. However, the difference in the intensity of the staining of the control E6.5 embryo (A, upper panel) and of the fat tissue (B) indicates that the expression of both, ARFRP1 and ARL1 in the E6.5 embryo is lower than in fat cells.
Figure 5. ARL1 targeting is disrupted in Arfrp1−/ − embryos. Immunohistochemical detection of ARFRP1 and ARL1 in control and Arfrp1−/ − embryos at day E 6.5 (A) and of fat tissue associated with the uterus of Arfrp1+/ − mother (B). Serial transversal sections of uteri at the stage of E6.5 were prepared and stained with hematoxilin and eosin (HE) or used for immunohistochemical detection of ARFRP1 and ARL1. ee, embryonic ectoderm; pac, proaminotic cavity.
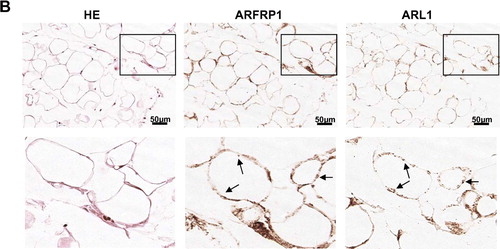
Silencing of ARFRP1 does not alter the cis-Golgi structure
In order to exclude that the observed disruption of ARL1 and Golgin-245 targeting to the trans-Golgi was due to a non-specific desintegration of the Golgi structure, we used two additional Golgi markers and compared their pattern with that of ARL1 and Golgin-245 in control and ARFRP1 knockdown cells. GM130 is a member of the Golgin-family which does not contain a GRIP domain, and giantin is a cis- and medial-Golgi marker. A shows the distribution of ARL1 and GM130 in control cells transfected with the empty vector and in cells transfected with the pSUPER-ARFRP1 construct. In control cells the staining of ARL1 and GM130 revealed a distinct structure within the Golgi apparatus, whereas in ARFRP1-knockdown cells only a dislocation of ARL1 could be detected while GM130 was not dissociated to the cytosol. We made the same observation when Golgin-245 was co-stained for giantin. In control cells Golgin-245 and giantin are both concentrated in the Golgi but as shown in the merged image both proteins do not co-localize. In cells in which the depletion of ARFRP1 resulted in a dissociation of Golgin-245 from Golgi membranes to the cytosol (arrows in B) giantin is still associated with Golgi membranes.
Figure 6. Inhibition of ARFRP1 expression in HeLa cells does not alter the structure of the cis-Golgi. ARFRP1 expression was downregulated in HeLa cells by transfection of pSUPER-ARFRP1 and 4 days later cells were fixed with methanol and (A) stained for ARL1 with an affinity purified polyclonal anti-ARL1 antibody in combination with an Alexa488-conjugated secondary antibody and co-stained for GM130 with a monoclonal antibody in combination with an Alexa546-conjugated secondary antibody or (B) stained for Golgin-245 with a polyclonal antibody in combination with an Alexa488-conjugated secondary antibody and co-stained for giantin with a monoclonal antibody in combination with an Alexa546-conjugated secondary antibody. Immunofluorescence was analysed by confocal laser scanning microscopy as described in the Methods.
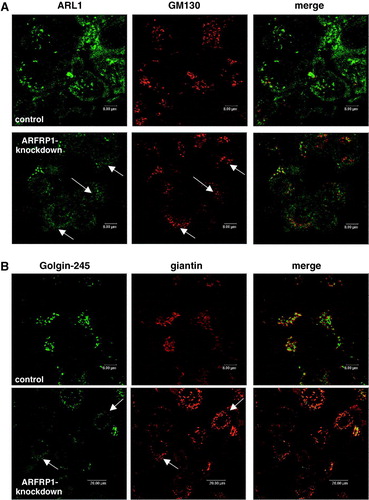
In order to verify if ARFRP1 is required for the maintenance of the TGN structure we analysed the effects of ARFRP1 knockdown on another established TGN marker, syntaxin 6. As shown in in control cells transfected with scrambled siRNA syntaxin 6 was co-localized with ARL1 in the TGN. Depletion of ARFRP1 resulted in a dislocation of syntaxin 6. While ARL1 appears to be distributed in the cytosol syntaxin 6 was still membrane associated but displaced in the periphery indicating that ARFRP1 is required for the appropriate structure and function of the TGN.
Figure 7. Depletion of ARFRP1 expression in HeLa cells alters TGN structure and dislocates syntaxin 6. HeLa cells were transfected with scrambled siRNA or with ARFRP1-specific siRNA and 4 days later cells were fixed with 4% paraformaldehyde and stained for ARL1 in combination with an Alexa546-conjugated secondary antibody and for syntaxin 6 in combination with an Alexa488-conjugated secondary antibody. Immunofluorescence was analysed by confocal laser scanning microscopy as described in the Methods.
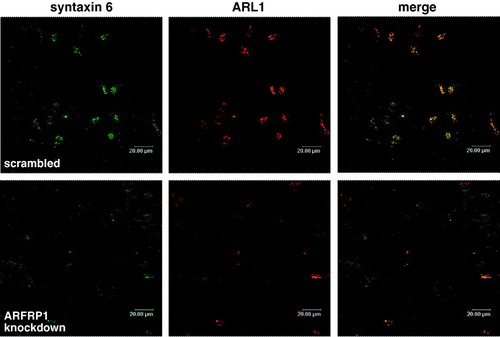
Discussion
With two different knockout approaches, this study demonstrates that ARFRP1 is essential for the targeting of ARL1 and its effector Golgin-245 to Golgi membranes. Therefore, the data prove that ARFRP1 is the functional counterpart of yeast Arl3p, and indicates that in mammalian cells the correct targeting of ARL1 and its effectors is required for the processing of proteins important for instance during embryonic development.
According to the presented data, the translocation of ARL1 to the Golgi apparatus in mammalian cells requires activated GTP-bound ARFRP1, suggesting that ARFRP1 functions upstream of ARL1. It has previously been shown that GTP-bound, Golgi-associated ARL1 binds to the C-terminal GRIP domain of several proteins e.g. Golgin-245 and recruits these proteins to the Golgi (Lu & Hong [Citation2003], Panic et al. [Citation2003a], Wu et al. [Citation2004]). Here, the essential role of ARFRP1 for targeting of ARL1 and Golgin-245 in mammalian cells was shown with three different but complementary approaches. (i) Expression of the guanine nucleotide exchange-defective mutant ARFRP-T31N which is widely distributed throughout the cytosol inhibits the Golgi association of ARL1 and Golgin-245 whereas the GTPase-negative ARFRP1-Q79L mutant co-localizes with ARL1 and its effector Golgin-245 ( & 2). This indicates that the GDP-restricted mutant ARFRP1-T31N exerts a dominant negative effect on ARL1 and Golgin-245 targeting. In addition, (ii) the depletion of ARFRP1 resulted in a relocation of GTP-bound ARL1 from the Golgi to the cytosol (). The specificity of the effect of ARFRP1 on the translocation of ARL1 to Golgi membranes is ascertained by the finding that Golgi association of the ARL1 effector Golgin-245 is also inhibited in cells lacking ARFRP1 (A). Furthermore, (iii) we could demonstrate that the absence of ARFRP1 affects the distribution of endogenous ARL1 and Golgin-245 (B, A). As the Golgi membrane association of two other proteins, giantin and GM130 is not disturbed by silencing of ARFRP1 (), it can be concluded that the depletion of ARFRP1 specifically affects the localization of GRIP domain proteins but does not alter the whole Golgi structure. However, ARFRP1 depleted cells show changes in the localization of syntaxin 6, an established TGN marker; syntaxin was still membrane associated but redistributed in peripheral puncta. This effect appears to be a consequence of the ARFRP1-dependent dislocation of Golgin-245 because Golgin-245-depleted cells exhibit the same changes in the localization of Golgi markers (Yoshino et al. [Citation2005], Lu et al. [Citation2004]). The observation that syntaxin 6 and thereby the TGN were displaced indicate that ARFRP1 plays an important role for TGN structure and function.
Since it has previously been shown that Arl3p, the yeast isologue of ARFRP1 and Arl1p act sequentially to recruit Imh1p, the sole GRIP domain protein in yeast (Panic et al. [Citation2003b], Setty et al. [Citation2003]), this study indicates that this recruitment is a conserved mechanism also occurring in mammalian cells. The fact that no membrane association of ARL1 was detected in Arfrp1 knockout embryos provides evidence that ARL1 requires the presence of ARFRP1 for correct targeting to Golgi membranes, and that this targeting is necessary for specific steps during embryonic development. While this manuscript was in preparation, Shin et al. ([Citation2005]) showed that ARFRP1 is involved in the Golgi-to-plasma membrane transport as well as in the retrograde transport from endosomes to the trans-Golgi network.
Our earlier study (Lu et al. [Citation2001]) suggests that GDP-GTP exchange of ARL1 is a rate-limiting step in regulating its Golgi targeting, and that this step could be bypassed by a mutation (Q71L) that keeps the mutant (ARL1-Q71L) in the GTP-bound form. Accordingly, ARL1 displays saturable Golgi targeting, while targeting of ARL1-Q71L to the Golgi seems not saturable. It is intriguing to note from our current study that Golgi targeting of ARL1-Q71L is abolished in ARFRP1 knockdown cells. This suggests that there is another rate-limiting event (downstream of guanine nucleotide exchange of ARL1) in regulating Golgi targeting of ARL1. Although the nature of this step is unknown, it is likely that ARFRP1 could govern Golgi targeting of a receptor/adaptor for ARL1-GTP, and that this receptor is no longer available at the Golgi when ARFRP1 is downregulated. Whether ARFRP1 also controls the GDP-GTP exchange of ARL1 remains unknown. Taken together, these observations indicate that Golgi targeting of ARL1 depends on two events: (i) guanine nucleotide exchange, and (ii) availability of a Golgi-associated receptor for ARL1. Our current working model is that ARFRP1 may coordinate both events to regulate Golgi recruitment of ARL1.
The finding that deletion of Arfrp1 in mice leads to early embryonic lethality during gastrulation (Mueller et al. [Citation2002]) suggests that the role of ARFRP1 in regulating the ARL1/Golgin-245 recruitment to the Golgi plays a critical role for protein transport during differentiation processes in multicellular organisms. Consistent with this conclusion, a mutation in Drosophila Arl1 is zygotic lethal which indicates an essential role of ARL1 for metazoans (Tamkun et al. [Citation1991]). In contrast, yeast arl1Δ, arl3Δ and imh1Δ (yeast GRIP-domain containing protein) mutants are viable but have partial defects in sorting of proteins to the lysosome-like vacuole which emphasizes their role for protein sorting in the late Golgi (Bonangelino et al. [Citation2002], Rosenwald et al. [Citation2002], Huang et al. [Citation1999], Lee et al. [Citation1997]). The specific effect of Arfrp1 deletion in mice at an early stage of embryonic development which is associated with a disruption of ARL1 association to the trans-Golgi indicates that the sequential recruitment of ARFRP1, ARL1, and presumably Golgin-97/Golgin-245 is required for the processing of some important proteins, but not for all Golgi functions.
This work was supported by the Deutsche Forschungsgemeinschaft (Sch 750/5-1 and 5-2).The skillful technical assistance of Monika Dopatka, Brigitte Rischke and Elisabeth Meyer is gratefully acknowledged.
References
- Behnia R, Panic B, Whyte JR, Munro S. Targeting of the Arf-like GTPase Arl3p to the Golgi requires N-terminal acetylation and the membrane protein Sys1p. Nat Cell Biol 2004; 6: 405–413
- Bonangelino CJ, Chavez EM, Bonifacino JS. Genomic screen for vacuolar protein sorting genes in Saccharomyces cerevisiae. Mol Biol Cell 2002; 13: 2486–2501
- Huang CF, Buu LM, Yu WL, Lee FJ. Characterization of a novel ADP-ribosylation factor-like protein (yArl3) in Saccharomyces cerevisiae. J Biol Chem 1999; 274: 3819–3827
- Kahn RA. ARF family GTPases. Proteins and cell regulation. Kluwer Academic Publishers, Dordrecht 2003; 1–21
- Lee FJ, Huang CF, Yu WL, Buu LM, Lin CY, Huang MC, Moss J, Vaughan M. Characterization of an ADP-ribosylation factor-like 1 protein in Saccharomyces cerevisiae. J Biol Chem 1997; 272: 30998–31005
- Lowe SL, Wong SH, Hong W. The mammalian ARF-like protein 1 (Arl1) is associated with the Golgi complex. J Cell Sci 1996; 10: 209–220
- Lu L, Hong W. Interaction of Arl1-GTP with GRIP domains recruits autoantigens Golgin-97 and Golgin-245/p230 onto the Golgi. Mol Biol Cell 2003; 14: 3767–3781
- Lu L, Horstmann H, Ng C, Hong W. Regulation of Golgi structure and function by ARF-like protein 1 (Arl1). J Cell Sci 2001; 114: 4543–4555
- Lu L, Thai G, Hong W. Autoantigen Golgin-97, an effector of Arl1 GTPase, participates in traffic from the endosome to the trans-golgi network. Mol Biol Cell 2004; 15: 4426–4443
- Mueller AG, Moser M, Kluge R, Leder S, Blum M, Büttner R, Joost H-G, Schürmann A. Embryonic lethality caused by apoptosis during gastrulation in mice lacking the gene of the ADP-ribosylation factor-related protein 1. Mol Cell Biol 2002; 22: 1488–1494
- Panic B, Perisic O, Veprintsev DB, Williams RL, Munro S. Structural basis for Arl1-dependent targeting of homodimeric GRIP domains to the Golgi apparatus. Mol Cell 2003a; 12: 863–874
- Panic B, Whyte JR, Munro S. The ARF-like GTPases Arl1p and Arl3p act in a pathway that interacts with vesicle-tethering factors at the Golgi apparatus. Curr Biol 2003b; 13: 405–410
- Rosenwald AG, Rhodes MA, Van Valkenburgh H, Palanivel V, Chapman G, Boman A, Zhang CJ, Kahn RA. ARL1 and membrane traffic in Saccharomyces cerevisiae. Yeast 2002; 19: 1039–1056
- Schürmann A, Joost H-G. ARF-like proteins. ARF Family GTPases, Ch. 16, RA Kahn. Kluwer Academic Publishers, Dordrecht 2003; 325–350
- Schürmann A, Massmann S, Joost H-G. ARP is a plasma membrane-associated Ras-related GTPase with remote similarity to the family of ADP-ribosylation factors. J Biol Chem 1995; 270: 30657–30663
- Schürmann A, Schmidt M, Asmus M, Bayer S, Fliegert F, Koling S, Massmann S, Schilf C, Subauste MC, Voss M, Jakobs KH, Joost H-G. The ADP-ribosylation factor (Arf)-related GTPase Arf-related protein binds to the Arf-specific guanine nucleotide exchange factor cytohesin and inhibits the Arf-dependent activation of phospholipase D. J Biol Chem 1999; 274: 9744–9751
- Setty SR, Shin ME, Yoshino A, Marks MS, Burd CG. Golgi recruitment of GRIP domain proteins by Arf-like GTPase 1 is regulated by Arf-like GTPase 3. Curr Biol 2003; 13: 401–404
- Setty SR, Strochlic TI, Tong AH, Boone C, Burd CG. Golgi targeting of ARF-like GTPase Arl3p requires its N(alpha)-acetylation and the integral membrane protein Sys1p. Nat Cell Biol 2004; 6: 414–419
- Shin HW, Kobayashi H, Kitamura M, Waguri S, Suganuma T, Uchiyama Y, Nakayama K. Roles of ARFRP1 (ADP-ribosylation factor-related protein 1) in post-Golgi membrane trafficking. J Cell Sci 2005; 118: 4039–4048
- Tamkun JW, Kahn RA, Kissinger M, Brizuela BJ, Rulka C, Scott MP, Kennison JA. The arf-like gene encodes an essential GTP-binding protein in Drosophila. Pro. Natl Acad Sci USA 1991; 88: 3120–3124
- Wu M, Lu L, Hong W, Song H. Structural basis for recruitment of GRIP domain golgin-245 by small GTPase Arl1. Nat Struct Mol Biol 2004; 11: 86–94
- Yoshino A, Setty SR, Poynton C, Whiteman EL, Saint-Pol A, Burd CG, Johannes L, Holzbaur EL, Koval M, McCaffery JM, Marks MS. tGolgin-1 (p230, golgin-245) modulates Shiga-toxin transport to the Golgi and Golgi motility towards the microtubule-organizing centre. J Cell Sci 2005; 118: 2279–2293