Abstract
Gut2, the mitochondrial glycerol-3-phosphate dehydrogenase, was previously shown to become preferentially labelled with photoactivatable phosphatidylcholine (PC), pointing to a functional relation between these molecules. In the present study we analyzed whether Gut2 functioning depends on the PC content of yeast cells, using PC biosynthetic mutants in which the PC content was lowered. PC depletion was found to reduce growth on glycerol and to increase glycerol excretion, both indicating that PC is needed for optimal Gut2 functioning in vivo. Using several in vitro approaches the nature of the dependence of Gut2 functioning on cellular PC contents was investigated. The results of these experiments suggest that it is unlikely that the effects observed in vivo are due to changes in cellular Gut2 content, in specific activity of Gut2 in isolated mitochondria, or in the membrane association of Gut2, upon lowering the PC level. The in vivo effects are more likely an indirect result of PC depletion-induced changes in the cellular context in which Gut2 functions, that are not manifested in the in vitro systems used.
Introduction
Phosphatidylcholine (PC) is a very abundant phospholipid in membranes of eukaryotes. Apart from functioning as an important building block of these membranes, PC is also involved in signal transduction as precursor for lipid second messengers, like PA and DAG Citation[1], Citation[2]. Disturbance of PC metabolism has been found in many pathological conditions, like Gaucher disease Citation[3] and Alzheimer's disease Citation[4], and in apoptosis Citation[5].
To get insight into the specific functions of PC, yeast is an attractive model organism. PC accounts for 40–50% of the total phospholipid content in yeast cells Citation[6]. As in higher eukaryotes, there are two routes in yeast by which PC is produced. The most important one is the triple methylation of PE by the enzymes Cho2 and Opi3 Citation[7]. The CDP-choline or Kennedy pathway recycles choline from PC turnover Citation[8]. This route only contributes to net PC synthesis when choline is supplemented in the growth medium Citation[8], Citation[9].
Yeast strains that are unable to synthesize PC, have problems in maintaining their respiratory competence Citation[10], indicating that PC is important for mitochondrial function, possibly because particular proteins depend on this lipid for proper functioning. To identify mitochondrial proteins that require PC, isolated yeast mitochondria were probed with a photoactivatable radiolabeled PC analogue (TID-PC) for PC interacting proteins. Photoactivation resulted in prominent labeling of Gut2, the FAD-dependent mitochondrial glycerol-3-phosphate dehydrogenase Citation[11], indicating a special relationship between PC and Gut2. In the present study we addressed the question whether PC is required for Gut2 functioning in yeast.
Gut2 is essential for growth of yeast on glycerol (hence its name Glycerol UTilization), by converting glycerol-3-phosphate to dihydroxyacetone phosphate Citation[12], Citation[13]. This reaction is also part of the glycerol-3-phosphate shuttle, in which the cytosolic glycerol-3-phosphate dehydrogenases Gpd1 and Gpd2 oxidize cytosolic NADH by passing the electrons on to dihydroxyacetone phosphate. The glycerol-3-phosphate formed is then oxidized in the mitochondria by Gut2. The result is a stepwise transfer of electrons from the cytosol to the respiratory chain Citation[14], Citation[15]. Gut2 was found to be a mitochondrial peripheral membrane protein Citation[11] that based on its function, is most likely associated with the inner membrane, facing the intermembrane space Citation[16].
To investigate the influence of the PC content on Gut2 functioning, yeast mutant strains were used, in which the PE methylation route for PC synthesis is impaired by deleting the CHO2 and/or OPI3 genes. These strains rely on exogenous choline to obtain wild type PC levels Citation[17–20]. By depriving these strains of choline, the cellular PC contents can be lowered from 40% to less than 5% of total phospholipids Citation[18], Citation[20], thus providing a useful system to analyze the influence of varying PC contents on Gut2 functioning.
The results obtained in vivo, i.e., reduced growth on glycerol and increased glycerol excretion upon PC depletion, support the hypothesis that PC is required for Gut2 functioning. In vitro approaches suggest that the in vivo effects are not due to changes in cellular Gut2 content, in specific activity of Gut2 in isolated mitochondria, or in the membrane association of Gut2, but rather are an indirect result of PC-depletion induced changes in the biological context in which Gut2 functions.
Materials and methods
Yeast strains, media and culture conditions
The yeast strains listed in were maintained on YPD agar plates (1% yeast extract, 2% bactopeptone and 2% glucose). Vitamin-defined synthetic media Citation[10] contained per liter: 5 g of ammonium sulfate, 1 g of potassium phosphate monobasic, 0.5 g of magnesium sulfate, 0.1 g of sodium chloride, 0.1 g of calcium chloride, 0.5 mg of boric acid, 0.04 mg of cupric sulfate, 0.1 mg of potassium iodide, 0.2 mg of ferric chloride, 0.4 mg of manganese sulfate, 0.2 mg of sodium molybdate, 0.4 mg of zinc sulfate, 20 mg of adenine, 20 mg of arginine, 20 mg of histidine, 60 mg of leucine, 230 mg of lysine, 20 mg of methionine, 300 mg of threonine, 20 mg of tryptophan, 40 mg of uracil, 2 µg of biotin, 400 µg of panthothenate, 2 µg of folic acid, 400 µg of niacin, 200 µg of p-aminobenzoic acid, 400 µg of pyridoxine hydrochloride. As carbon source was added 30 g glucose (SD medium), 22 ml lactic acid with 1 g glucose (SL medium, adjusted to pH 5.5 using KOH), or 20 g glycerol with 1 g glucose (SG medium). Media were supplemented with 75 µM inositol and where indicated with 1 mM choline (C+). The CRD1 and crd1 strains were grown to late log phase in YPLac, containing 1% (w/v) yeast extract, 2% (w/v) peptone and 2% (v/v) lactate Citation[21], supplemented with 0.1% (w/v) glucose and with a pH value of 5.5.
Table I. Genotypes of strains employed in this study.
All cultures were grown aerobically at 30°C (with shaking in the case of liquid cultures). Growth was monitored by measuring the OD at 600 nm, using a Hitachi 150–20 double beam spectrophotometer.
Epitope tagging of Gut2
Gut2 was epitope-tagged at its C-terminus by cloning a histidine (His6) and a hemagglutinin (HA) tag at the end of the GUT2 gene into the yeast genome, via homologous recombination with a PCR fragment, also containing the kanMX cassette. The plasmid pU6H3HA (EMBL accession number AJ132966, Citation[22]) was used as a template for PCR amplification. The primers used were 5′-ACT TGA AAA AAC TGT GAA CTT CAT CAA GAC GTT TGG TGT CTC CCA CCA CCA TCA TCA TCA CG-3′ (with the underlined fragment corresponding to nucleotides 1908–1947 of the GUT2 ORF) and 5′-TTA TAT TAT GTA TTG GAA ATA GAA TAT AAA CAC TAG GAA GAC TAT AGG GAG ACC GGC AGA TCC G-3′ (with the underlined sequence corresponding to nucleotides 290–251 downstream of the GUT2 ORF). The PCR product of 1815 bp was used to transform the yeast strain SH922 and positive colonies were selected on G418 containing plates as described Citation[22]. Correct integration of the PCR fragment into the yeast genome was checked by colony PCR. Expression of the hemagglutinin-tagged Gut2 was verified by Western blotting.
Deletion of GUT2
In the wild-type strain SH921 the GUT2 gene in the yeast genome was replaced with a PCR fragment containing a kanMX cassette, via homologous recombination. The plasmid pU6H3HA was used as a template for PCR amplification with the primers 5′-GCT ATT GCC ATC ACT GCT ACA AGA CTA AAT ACG TAC TAA TAT ccc tta ata taa ctt cg-3′ (with the underlined sequence corresponding to nucleotides (−42)−(−1) upstream of the GUT2 ORF) and 5′-GTG AAT GTT ATC TTT GTC ACC CTT AAC TAT CAT GAT CGA Tca cct aat aac ttc gta tag c-3′ (with the underlined sequence corresponding to nucleotides 40-1 downstream of the GUT2 ORF). The PCR product of 1637 bp was used to transform the yeast strain SH922. The GUT2 gene was also deleted in the BY4742 background, by replacement with a PCR fragment containing the URA3 gene, obtained using the pRS316 plasmid Citation[23] as template and the primers 5′-TAT TGC CAT CAC TGC TAC AAG ACT AAA TAC GTA CTA ATA TCA TCA GAG CAG ATT GTA CTG AGA AGT GC-3′ (with the underlined sequence corresponding to nucleotides (−40)−(−1) upstream of the GUT2 ORF) and 5′-TTA TAT TAT GTA TTG GAA ATA GAA TAT AAA CAC TAG GAA GGC ATC TGT GCG GTA TTT CAC ACC-3′, with the underlined sequence corresponding to nucleotides 290–250 downstream of the GUT2 ORF). Correct replacement of the GUT2 gene by the PCR fragments was verified by colony PCR.
Choline deprivation
In this study two methods were used to transfer the cho2opi3 cells from choline-containing to choline-free culture medium. For the analysis of growth on different carbon sources, cells were grown in choline-containing synthetic medium to OD600 0.7–1.0. Subsequently the cells were collected by filtration, using 0.45 µm cellulose acetate filters (Nalgene, Rochester, NY, USA), washed with the corresponding choline-free medium and resuspended in fresh medium (with or without choline) to an OD600 of 0.1.
For the isolation of PC-depleted mitochondria, larger quantities of cho2opi3 cells had to be deprived of choline. This was accomplished by collecting the cells (grown to an OD600 of more than 2.0 in SL C+) by centrifugation. The cells were washed twice by resuspension in SL C− followed by centrifugation. Finally the cells were resuspended in SL C− and transferred to fresh SL medium (with or without choline) to an OD600 of 0.1–0.2.
Glycerol production
In order to have corresponding genotypes among the mutants tested, wild type, cho2, opi3 and gut2 strains with a BY4742 background () were used. The strains were grown in yeast nitrogen base without amino acids (YNB, Difco) with an initial pH value of 6.0, supplemented with 5 g/l glucose (10 g/l for pre-cultures) and 120 mg/l of each histidine, leucine, lysine and uracil, as described elsewhere Citation[14]. The glycerol excretion in the medium was measured after 48 h using an enzymatic test kit (R-Biopharm AG, Almere, The Netherlands) according to the manufacturer's instructions.
Isolation of mitochondria
The cho2opi3 strain PR03 or SH922 () was pre-cultured in SL C+. The cells were deprived of choline by centrifugation and washing steps as described above and transferred to fresh medium with or without choline. The cells were grown for the indicated number of generations, harvested by centrifugation (10 min, 3500 g) and washed with water. Spheroplasts were prepared and homogenized as described Citation[24], Citation[25]. Mitochondria were isolated using differential centrifugation as described elsewhere Citation[25]. The obtained pellet of crude mitochondria was resuspended carefully in a minimal volume of H/S buffer (20 mM Hepes/KOH, 0.6 M D-sorbitol, 0.5% (w/v) BSA, pH 7.4). The mitochondria were frozen as 50 µl aliquots in liquid N2 and stored at −80°C. Samples were thawed only once before use.
Sucrose gradient-purified mitochondria of the CRD1 and crd1 strains were isolated as described Citation[25].
Measurement of Gut2 activity
The activity of Gut2 was measured by monitoring spectrophotometrically the phenazine methosulfate (PMS)-mediated reduction of 3-(4,5-dimethylthiazolyl-2)-2,5-diphenyl tetrazolium bromide (MTT) as described Citation[11], Citation[26], with minor changes. The reaction mixture contained 50 mM Hepes pH 7.5, 10 mM KCN, 0.5 mM MTT, 0.2 mM PMS, 0.05% (v/v) Triton X-100, 50 mM DL-glycerol-3-phosphate and 50 µM FAD. In experiments in which the substrate concentration in the assay was varied, no significant influence of PC depletion on the KM value of Gut2 was found (data not shown). Therefore, all Gut2 activities were measured under conditions where the substrate concentration was not rate limiting (data not shown). Where indicated, Triton X-100 was omitted from the assay mixture and 0.6 M D-sorbitol was added, to obtain an isotonic buffer. Gut2 specific activities were determined in a reaction volume of 1 ml, typically containing 100 µg of mitochondrial protein, using a Perkin Elmer Lambda 18 UV/VIS spectrophotometer. The variation of Gut2 activity with PC content was measured in a reaction volume of 200 µl, typically containing 30 µg of mitochondrial protein, and monitored in 96 well plates, using a BioRad Ultramark Microplate Imaging System.
The increase in extinction at 562 nm (560 nm in the case of the plate reader) was recorded for 10 min after the addition of mitochondria. Activities were calculated from the slope, using an extinction coefficient for reduced MTT of 8.1 mM−1 cm−1Citation[11]. The numbers were corrected for background activity by repeating each measurement in the absence of the substrate glycerol-3-phosphate.
Influence of phospholipids on Gut2 activity
To study the effect of phospholipids on Gut2 activity a suspension of mitochondria from strain PR03 in H/S buffer (corresponding to 1.5 mg mitochondrial protein/ml) was mixed 1:4 (v:v) with a 400 µM solution of 1,2-dioleoyl-sn-glycero-3-phosphocholine (DOPC), 1,2-dioleoyl-sn-glycero-3-phosphoethanolamine (DOPE), 1,2-dioleoyl-sn-glycero-3-phosphoglycerol (DOPG) or 1,1′,2,2′-tetraoleoyl-cardiolipin (TOCL) in 0.5% (w/v) Triton X-100. All lipids were obtained from Avanti Polar Lipids (Alabaster, AL, USA). The mixture was incubated for 15 min at room temperature and subsequently the Gut2 activity was measured in aliquots corresponding to 30 µg mitochondrial protein in 96-well plates as described above.
Association of Gut2 with mitoplasts
Mitoplasts were prepared by diluting mitochondria from the PR03 strain at least a factor of 10 in hypotonic buffer H (20 mM Hepes/KOH, pH 7.4) to a concentration of 1 mg protein/ml Citation[27]. The mitochondria were incubated for 20 min on ice, followed by centrifugation for 12 min at 10,000 rpm (10,600 g) in a microfuge at 4°C. The pellet was washed with buffer H, followed by centrifugation as above. The pellet was incubated for 5 min with buffer H, also containing 1 M NaCl, or 1, 2, 10, 20 or 75 mM Na2CO3 (freshly prepared) and centrifuged again. The pellet and supernatant fractions were analyzed by Western blotting.
Other methods
Protein concentrations were measured using the BCA method (Pierce) with 0.1% (w/v) SDS added and bovine serum albumin (BSA) as a standard. Total cell lysate for Western blotting was obtained according to Citation[28]. Western blots were decorated with antibodies against the hemagglutinin tag (Boehringer), porin and the phosphate carrier PiC (both kindly provided by Dr R. Lill) and protein bands were visualized by ECL (Amersham) according to manufacturer's instructions. Protein bands were quantified with a BioRad Model GS-700 Imaging Densitometer. The phospholipid compositions of mitochondria were analyzed by thin layer chromatography (TLC) as described Citation[29].
Results
Effect of PC depletion on Gut2 function in vivo
Effect of PC depletion on the ability of yeast to grow on glycerol. In this study the hypothesis was tested, that PC is required for the function of Gut2, and hence for the ability of S. cerevisiae to grow on glycerol as carbon source. To vary the PC level, yeast cells of the cho2opi3 strain SH922 were transferred to growth medium with or without choline. Cells cultured in the absence of exogenous choline cannot produce PC and therefore PC levels will drop during further growth Citation[17], Citation[20], Citation[30]. In the presence of choline, cells have wild type PC levels. The media contained the fermentable carbon source glucose or the non-fermentable carbon sources lactate or glycerol. It was verified that a gut2 strain with the same genetic background (, PR01) did not grow on glycerol (data not shown).
In the presence of choline, regular growth curves were obtained for all conditions (, squares). In the absence of choline, initially similar growth rates were observed on all three carbon sources (, triangles). However, after approximately 10 h, the rate of growth decreased on all carbon sources compared to the + choline condition, demonstrating the importance of PC for the growth of yeast cells. The strongest decrease in growth rate was observed on glycerol, where cells virtually stopped growing after 13 h, whereas growth on the other carbon sources still continued at a low rate. At this time point, the PC level is estimated to be about 4% (based on the PC content being halved with each doubling of the number of cells, starting from 40% Citation[30]). The decrease in growth rate on glycerol upon PC depletion points to a role of PC in Gut2 functioning. To further test our hypothesis, the glycerol excretion of mutants with varying PC levels was measured.
Figure 1. The effect of choline deprivation on the growth of a cho2opi3 strain on different carbon sources. Strain SH922 was pre-cultured in SD, SG or SL C+ to mid-log phase. Cells were collected by filtration, washed and transferred to fresh SD, SG or SL medium with (squares) or without (triangles) 1 mM choline, as described in the Materials and methods section. Growth was measured as the optical density at 600 nm at successive time points. Results from a typical experiment are shown.
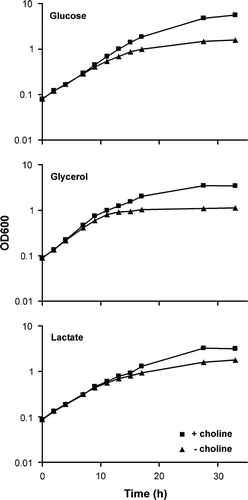
Effect of PC content on glycerol excretion
In yeast several systems exist that recycle cytosolic NADH, with the glycerol-3-phosphate shuttle generating the most ATPs per oxidized NADH. The glycerol-3-phosphate dehydrogenase Gpd1 oxidizes NADH, to produce glycerol-3-phosphate. Subsequently, Gut2 converts glycerol-3-phosphate to dihydroxyacetonephosphate and the reducing equivalents are passed on to the respiratory chain. It has been shown that blocking Gut2 function results in glycerol excretion, probably to get rid of accumulating glycerol-3-phosphate via dephosphorylation by Gpp1 and Gpp2 Citation[14]. Hence, glycerol excretion is a measure of Gut2 dysfunction. It was investigated whether the glycerol production depends on the PC content of yeast cells. For this purpose cho2 and opi3 single deletion strains were used, because the rigorous conditions of PC depletion in the cho2opi3 strain are not compatible with the assay of glycerol production. These strains have wild type levels of PC when grown in the presence of choline. However, in the absence of choline, the PC levels drop to about 9% and 4% of total phospholipid content in cho2 and opi3 strains, respectively Citation[18], Citation[20]. The glycerol production by these strains cultured with or without choline is depicted in , and is compared to the excretion by the wild type and a gut2 strain with the same genetic background.
Figure 2. The effect of the PC content of yeast cells on the glycerol production. The cho2, opi3 and gut2 deletion strains and their parental wild type were grown on yeast nitrogen base, supplemented with 0.5% glucose (w/v) and 120 mg/l of each histidine, leucine, lysine and uracil in the presence (black bars) or absence (grey bars) of 1 mM choline, as described in detail in Materials and methods. The glycerol concentrations in the medium were measured after 48 h and have been normalized to the OD600 values of the cultures. The error bars represent the variation in two experiments.
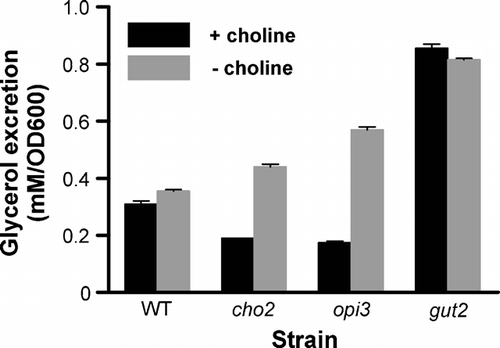
The Figure shows that the deletion of the GUT2 gene in the wild type strain results in a large increase in glycerol excretion in agreement with a previous report Citation[14]. The excretion of glycerol by the wild type and the gut2 strain was independent of the presence of choline in the medium, as expected because of their ability to synthesize PC via the methylation of PE. In the presence of choline the cho2 and opi3 strains have wild type levels of PC Citation[18], but their glycerol excretion was somewhat less compared to that of the wild type strain. We speculate that this is due to the connection between phospholipid and glycerol metabolism. However, in the absence of choline 2.3-fold more glycerol was excreted by the cho2 strain than in the presence of choline and this increase was even higher (3.3-fold) for the opi3 strain which has a lower PC content than the cho2 strain in the absence of choline Citation[17–20]. The observation that glycerol excretion is increased upon lowering the PC content further supports the suggestion that PC is needed for Gut2 activity in vivo.
Effect of PC depletion on Gut2 function in vitro
Dependence of Gut2 content and activity on PC content
The suggested reduction in Gut2 functioning in response to lower PC levels could be due to a reduction in content or to a decrease in enzyme activity. These possibilities were investigated using the cho2opi3 strain PR03 containing a chromosomally encoded hemagglutinin tagged Gut2. PR03 cells, precultured in the presence of choline, were transferred to medium with or without choline and cultured for different periods. Analysis of cell lysates by Western blotting using an antibody against the hemagglutinin tag of Gut2, revealed that the Gut2 expression level was not affected by PC depletion. After 3 generations the Gut2 content in PC depleted mitochondria was 101% of the Gut2 content in mitochondria with a normal PC level (A).
Figure 3. The effect of PC depletion on the activity and content of Gut2 in the cho2opi3 strain PR03. (A) Gut2 content in total cell lysates. Cells were lysed after growth for the indicated number of generations (1–3) in the presence (+) or absence (−) of 1 mM choline. The Gut2 content in the cell lysate was quantified by Western blotting using an antibody against the hemagglutinin tag of Gut2, with PiC as a loading control (visualized using an antibody against PiC). The faint upper band might represent precursor Gut2, based on the difference in mass and the visualization by the antibody against the hemagglutinin tag. (B) Gut2 activity in isolated mitochondria. Mitochondria were isolated from cells grown for 3 generations in the presence (squares) or absence (triangles) of 1 mM choline. The Gut2 activity was measured in detergent solubilized (upper panel) and intact (lower panel) mitochondria in 96 well plates, as described in Materials and methods. The reduction of the indicator MTT was followed spectrophotometrically at 560 nm. Results of a typical experiment are shown. (C) Quantification of the Gut2 content in mitochondria from cells harvested after growth for 3 generations in the presence or absence of 1 mM choline, using an antibody against the tag of Gut2. Different amounts of mitochondrial protein were loaded as indicated. (D) The influence of choline deprivation on the activity and content of Gut2 in isolated mitochondria from cho2opi3 strain PR03. Mitochondria were isolated from PR03 cells grown for different numbers of generations in the presence or absence of 1 mM choline. The Gut2 activity in detergent solubilized mitochondria, corresponding to 30 µg mitochondrial protein, and the mitochondrial Gut2 content were determined as described in detail in Materials and methods. The levels of Gut2 activity and Gut2 content in PC depleted mitochondria are depicted relative to the levels in mitochondria isolated from cells grown in the presence of choline, for the corresponding number of generations. The error bars at 3 generations represent the SD (n≥3).
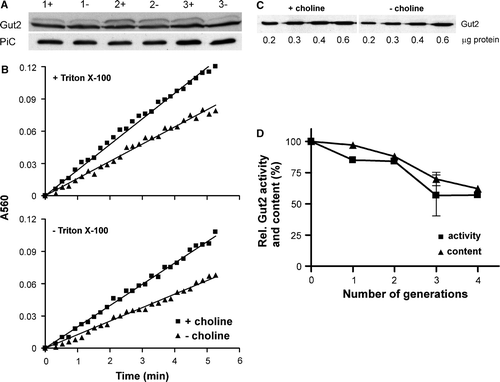
To address the question whether the specific activity of Gut2 depends on PC, mitochondria with different PC contents were isolated from PR03 cells, cultured as above. The enzyme activity of Gut2 was measured in detergent solubilized mitochondria (B, upper panel) and was linear in time. From the slopes a 1.8 fold reduction in enzyme activity was calculated (n=3), after PC depletion for 3 generations. To exclude the possibility that the result of the activity assay was affected by disruption of functional interactions between Gut2 and PC, the Gut2 activity assay was carried out in the absence of this detergent and in the presence of D-sorbitol to obtain an isotonic buffer. In intact mitochondria a similar reduction of activity upon PC depletion was found (B, lower panel), also indicating that the substrate accessibility was not affected by PC depletion.
It should be noted, that the activities were determined for Gut2 that had been tagged with His6HA for quantification purposes. To investigate the influence of the tag, the activity of Gut2 was also measured in mitochondria from the SH922 strain containing untagged Gut2. It was found that the introduction of the His6HA tag caused a reduction in specific activity from 50.0±4.4 to 17.3±2.7 nmol min−1 mg−1 (average±SD, n=5) in Triton X-100 solubilized mitochondria from cells grown in the presence of choline. However, the tagging of Gut2 did not affect the apparent dependence of Gut2 activity on PC. Upon PC depletion a similar reduction in Gut2 enzyme activity was found in the SH922 strain (data not shown) as in the PR03 strain (B).
The decrease in Gut2 activity in mitochondria after PC depletion could be due to a decrease in specific activity of the enzyme or to a decrease of the Gut2 content in the mitochondrial preparations (or a combination of both). To distinguish between these possibilities, the relative amounts of tagged Gut2 per mg mitochondrial protein were determined by Western blotting (C). The blot suggests that there is less Gut2 in the mitochondria after PC depletion. To investigate the correlation between the decreases in Gut2 activity and content in greater detail, mitochondria were isolated at different stages of PC depletion. The mitochondria were analyzed for Gut2 activity after solubilization in Triton X-100 and for Gut2 content (D). Upon PC depletion for 3 generations the Gut2 activity was found to drop to 57±16% of the activity found in mitochondria from cells grown in the presence of choline for the same number of generations. The decline of the amount of Gut2 per mg mitochondrial protein almost coincided with the reduction in Gut2 activity, suggesting that the specific activity of Gut2 is not affected by PC depletion, not even when the mitochondrial PC content has dropped from 42% to 2% of total phospholipids after PC depletion for 4 generations (determined by TLC, data not shown).
As mentioned above, the Gut2 expression level was constant in total cell lysates during PC depletion, which contrasts the observed decline of Gut2 content in isolated mitochondria under the same conditions. A possible explanation is that the purity of the mitochondrial preparations is influenced by PC depletion. Since mitochondria obtained by differential centrifugation are usually contaminated by ER Citation[25], Citation[31], it was investigated whether PC depletion increases the extent of mitochondrial contamination by ER. Mitochondria were isolated from PR03 cells after 4 generations of growth in the presence or absence of choline and subjected to Western blotting, using antibodies against porin and Sec61, a mitochondrial and an ER marker, respectively. As a control, the Sec61/porin ratios were determined in total cell lysates.
The results shown in demonstrate that the Sec61/porin ratio is strongly increased in mitochondria upon PC depletion, reflecting a stronger contamination with ER of the mitochondrial preparations. The increase in ratio of about 30% is consistent with the observed decrease in Gut2 content and suggests that the mitochondrial Gut2 content is not affected by PC depletion.
Table II. Sec61/porin ratios in total cell lysates and isolated mitochondria from PR03 cells, grown in the presence or absence of 1 mM choline for 3 generations. The proteins were quantified by Western blotting using antibodies against Sec61 and porin.
In summary, depleting the mitochondria of PC does not affect the specific activity of Gut2. The possible influence of PC on Gut2 activity was further investigated by measuring the effect of PC addition on the enzyme activity.
Influence of phospholipids on Gut2 activity
Detergent solubilized mitochondria were incubated with excess detergent solubilized lipids and the Gut2 activity was measured (). The activity was not influenced by any of the phospholipids tested, irrespective of the PC content of the solubilized mitochondria, except for a small stimulation by CL. The stimulatory effect of CL on Gut2 activity may suggest a role for CL in Gut2 activity. Therefore, mitochondria were isolated from a crd1 mutant strain (FGY2), lacking CL synthase activity and CL. The Gut2 activity in these mitochondria was found to be comparable to the activity in mitochondria of the parental wild type strain (FGY3), demonstrating that CL is not required for Gut2 activity (data not shown).
Figure 4. The influence of different phospholipids on Gut2 activity. The indicated phospholipids were added to an at least 6-fold molar excess over the corresponding mitochondrial phospholipid (based on De Kroon et al. 1999) to Triton X-100 solubilized mitochondria from cho2opi3 cells grown in the presence (black) or absence (grey) of 1 mM choline for 4 generations. The mixture was incubated for 15 min at room temperature and subsequently the Gut2 activity was measured using the 96 well plate assay as described in the Materials and methods section. The activity in the control (no lipids added) was set at 100%. The error bars represent the SD (n=3).
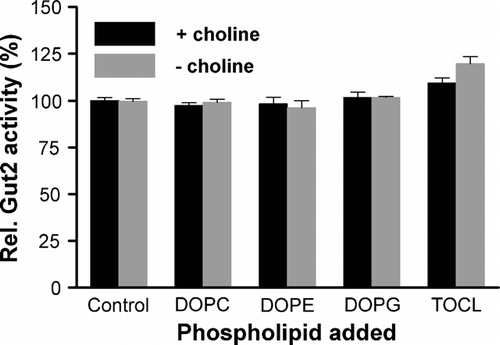
The absence of a stimulatory effect of exogenous PC on the activity of Gut2 in detergent solubilized, PC depleted mitochondria indicates that either the activity of Gut2 itself is not dependent on PC or that there is still sufficient PC left to fulfill a possible PC requirement of Gut2 activity.
Effect of PC content on binding of Gut2 to the mitochondrial membranes
To investigate whether the reduced PC content resulted in Gut2 release from the membrane and in this way affected the activity per Gut2 molecule, the binding of Gut2 to the membrane was studied by washing mitoplasts with carbonate and salt.
Mitochondria from cho2opi3 cells PR03 cultured for 4 generations in the presence or absence of 1 mM choline were isolated, and processed to mitoplasts by disruption of the outer membrane by osmotic shock. This treatment did not induce significant release of Gut2 from the mitoplasts (data not shown). The mitoplasts were washed in hypotonic buffer, or in buffers containing NaCl or sodium carbonate, in order to determine whether PC depletion affects the membrane association of Gut2. After centrifugation the distribution of Gut2 and the integral outer membrane protein porin over the pellet and supernatant fractions was determined by Western blotting (). As expected for an integral membrane protein, porin was recovered exclusively in the pellet fractions under all conditions tested. After washing with the hypotonic buffer all Gut2 was found in the pellet fraction. Washing with 1 M NaCl induced the release of a small portion of Gut2 in the supernatant, irrespective of the PC content of the mitoplasts. The gradual dissociation of Gut2 from the membrane by increasing concentrations of Na2CO3 was not affected by the PC content of the mitoplasts either. This suggests that PC does not regulate Gut2 enzyme activity via association with the membrane. The complete release of Gut2 at low carbonate concentrations (20 mM) indicates that Gut2 is a peripheral membrane protein, in agreement with earlier findings Citation[11].
Figure 5. The effect of PC depletion on the association of Gut2 with mitoplasts. Mitoplasts were prepared by diluting mitochondria from cho2opi3 cells (strain PR03) grown for 4 generations in the presence (+ cho) or absence (− cho) of 1 mM choline, in hypotonic buffer. After washing with buffer H the mitoplasts were incubated for 5 min in buffer H (control) or in buffer H containing 1 M NaCl or Na2CO3 at the concentrations indicated. The mitoplasts were spun down and the amount of Gut2 and porin in the pellet (p) and supernatant (s) fractions was determined by Western blotting, using an antibody against the hemagglutinin tag of Gut2 and against porin, respectively. Results from a typical experiment are shown.
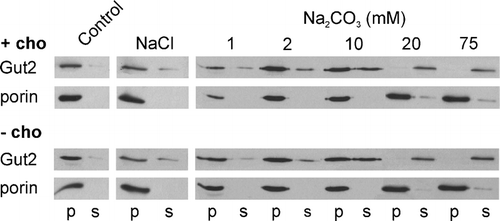
Discussion
In this study the possible influence of PC on the functioning of Gut2 was investigated by in vivo and in vitro approaches, using yeast mutant strains allowing controlled depletion of the PC level.
The results obtained in vivo, the abrogation of growth on glycerol of cho2opi3 cells upon choline deprivation and the glycerol production by cho2 and opi3 cells cultured in the absence of choline, strongly suggest that Gut2 requires PC for efficient functioning. Using different in vitro approaches it was tried to elucidate the underlying mechanism. It appeared that the Gut2 expression level in total cell lysate and the specific activity of Gut2 in mitochondria were not affected by PC depletion, making it unlikely that PC is required for the activity of the enzyme. The latter finding was confirmed by the absence of a stimulatory effect of the addition of phospholipids, including PC, on Gut2 activity in a micellar system, derived from PC depleted mitochondria. No differences in Gut2 activity were observed between detergent solubilized mitochondria and intact mitochondria, suggesting that also the supply of substrate for Gut2 is not responsible for the effects of PC depletion in vivo. Finally, the membrane association of Gut2 was identical in mitoplasts with normal or low PC contents, rendering a regulatory mechanism via membrane docking unlikely.
Our finding that Gut2 is a peripheral membrane protein contrasts a recent report, in which Gut2 was classified as an integral outer membrane protein Citation[32], based on the partitioning of Gut2 into the detergent phase in a Triton X-114 phase separation experiment performed on isolated mitochondrial outer membrane vesicles. Previously, it was thought that Gut2 is located at the outside of the inner membrane Citation[16], consistent with our earlier finding that Gut2 is not crosslinked upon incubating isolated outer membranes with TID-PC Citation[11]. The apparent discrepancies in submitochondrial localization and in the nature of membrane association might be explained by a dual localization of Gut2. Alternatively, and more likely in view of the molecular weight of Gut2 reported by Burri et al. Citation[32], these authors may have mistaken Gut2 for its precursor, which has recently been shown to accumulate in the outer membrane of yeast mitochondria Citation[33].
How can we understand the effect of the PC content on Gut2 functioning in vivo? It should be realized that Gut2 activity in vivo is influenced by many factors such as the concentrations of nucleotides Citation[34–36] and the activity of other enzymes involved in electron transfer, like the NADH dehydrogenase Nde1 Citation[34], Citation[36]. It is conceivable that some of these factors are lost during the isolation of the mitochondria thereby masking possible effects of PC depletion in the in vitro Gut2 activity assay.
Another reason for the effects observed in vivo not being reflected in the in vitro experiments might be related to metabolic compartmentation. Enzymes involved in a specific process are often clustered in complexes or are enclosed by a membrane. This can avoid competition for substrates with other processes and can speed up reactions because enzymes ‘sense’ higher substrate concentrations and because reaction intermediates do not have to diffuse from one protein to the next. Compartmentation is well established for enzymes involved in bioenergetics and might apply as well to Gut2, which has been shown to be part of a supermolecular complex Citation[37]. This complex is in tight contact with the mitochondrial inner membrane, as it is composed of proteins localized to both sites of this membrane and contains integral membrane proteins as well. Depletion of an important membrane building block, PC, might disturb the structure of this complex, hindering the transport of substrates or products. This might have been masked under the conditions of the in vitro assays by the ample supply of substrate and electron acceptor, and the uncoupling of the reaction from the respiratory chain used to avoid influences by other processes. An indication that the structural organization of cellular compartments after PC depletion is altered, comes from our observation that mitochondrial preparations of cells grown for 4 generations in the absence of choline, are more contaminated with ER.
These explanations for the lack of support from the in vitro experiments for the observations in vivo indicate that the apparent role of PC in Gut2 functioning results indirectly from changes induced by PC depletion, and not from a direct influence of PC on Gut2 enzyme activity.
The basis for this study was the observation that incubation of yeast mitochondria with TID-PC followed by photoactivation resulted in prominent labeling of the Gut2 protein Citation[11]. This labeling was not the result of a preference of the TID group for Gut2, but reflects a preferential interaction of PC with this protein Citation[11]. In the light of the results in the present study we suggest that under wild type conditions PC is involved in binding Gut2 to the membrane. Upon PC depletion binding is maintained, which suggests that either the residual PC (2% of total phospholipid) is sufficient for binding or that other phospholipids take over the role in binding. This would imply that hydrophobic interactions are important for the membrane association of Gut2, which is supported by the observation that even at 1 M NaCl the binding of Gut2 to the membrane was hardly affected.
To be able to bind hydrophobically to the membrane, Gut2 requires hydrophobic patches on its surface. The identification of these patches is complicated, because no atomic structure of Gut2 or of a homologue has been published. Analysis of Gut2 by a hydrophobic moment plot Citation[38] and the secondary structure prediction program Jpred Citation[39] suggested the presence of several amphipathic helices (detailed in the appendix) of which the hydrophobic sides could be involved in lipid binding. It is also known that glycerol-3-phosphate dehydrogenases from other sources are influenced by membrane lipids with a rather broad specificity Citation[40–42], which is consistent with a prominent role of hydrophobic interactions between Gut2 and the membrane.
This paper was first published online on iFirst on 08 May 2007.
Acknowledgements
This research was supported by an Earth and Life Sciences (ALW) grant with financial aid from the Dutch Organization for Scientific Research (NWO).
References
- Exton JH. Phosphatidylcholine breakdown and signal transduction. Biochim Biophys Acta 1994; 1212: 26–42
- Rudge SA, Pettitt TR, Zhou C, Wakelam MJ, Engebrecht JA. SPO14 separation-of-function mutations define unique roles for phospholipase D in secretion and cellular differentiation in Saccharomyces cerevisiae. Genetics 2001; 158: 1431–1444
- Trajkovic-Bodennec S, Bodennec J, Futerman AH. Phosphatidylcholine metabolism is altered in a monocyte-derived macrophage model of Gaucher disease but not in lymphocytes. Blood Cells Mol Dis 2004; 33: 77–82
- Nitsch RM, Blusztajn JK, Pittas AG, Slack BE, Growdon JH, Wurtman RJ. Evidence for a membrane defect in Alzheimer disease brain. Proc Natl Acad Sci USA 1992; 89: 1671–1675
- Cui Z, Houweling M. Phosphatidylcholine and cell death. Biochim Biophys Acta 2002; 1585: 87–96
- Daum G, Tuller G, Nemec T, Hrastnik C, Balliano G, Cattel L, Milla P, Rocco F, Conzelmann A, Vionnet C, et al. Systematic analysis of yeast strains with possible defects in lipid metabolism. Yeast 1999; 15: 601–614
- Carman GM, Zeimetz GM. Regulation of phospholipid biosynthesis in the yeast Saccharomyces cerevisiae. J Biol Chem 1996; 271: 13293–13296
- McMaster CR, Bell RM. Phosphatidylcholine biosynthesis in Saccharomyces cerevisiae. Regulatory insights from studies employing null and chimeric sn-1,2-diacylglycerol choline- and ethanolaminephosphotransferases. J Biol Chem 1994; 269: 28010–28016
- Carman GM, Henry SA. Phospholipid biosynthesis in the yeast Saccharomyces cerevisiae and interrelationship with other metabolic processes. Prog Lipid Res 1999; 38: 361–399
- Griac P, Swede MJ, Henry SA. The role of phosphatidylcholine biosynthesis in the regulation of the INO1 gene of yeast. J Biol Chem 1996; 271: 25692–25698
- Janssen MJ, van Voorst F, Ploeger GE, Larsen PM, Larsen MR, de Kroon AI, de Kruijff B. Photolabeling identifies an interaction between phosphatidylcholine and glycerol-3-phosphate dehydrogenase (Gut2p) in yeast mitochondria. Biochemistry 2002; 41: 5702–5711
- Sprague GF, Cronan JE. Isolation and characterization of Saccharomyces cerevisiae mutants defective in glycerol catabolism. J Bacteriol 1977; 129: 1335–1342
- Ronnow B, Kielland-Brandt MC. GUT2, a gene for mitochondrial glycerol 3-phosphate dehydrogenase of Saccharomyces cerevisiae. Yeast 1993; 9: 1121–1130
- Larsson C, Pahlman IL, Ansell R, Rigoulet M, Adler L, Gustafsson L. The importance of the glycerol 3-phosphate shuttle during aerobic growth of Saccharomyces cerevisiae. Yeast 1998; 14: 347–357
- Overkamp KM, Bakker BM, Kotter P, van Tuijl A, de Vries S, van Dijken JP, Pronk JT. In vivo analysis of the mechanisms for oxidation of cytosolic NADH by Saccharomyces cerevisiae mitochondria. J Bacteriol 2000; 182: 2823–2830
- von Jagow G, Klingenberg M. Pathways of hydrogen in mitochondria of Saccharomyces carlsbergensis. Eur J Biochem 1970; 12: 583–592
- Kodaki T, Yamashita S. Yeast phosphatidylethanolamine methylation pathway. Cloning and characterization of two distinct methyltransferase genes. J Biol Chem 1987; 262: 15428–15435
- Kodaki T, Yamashita S. Characterization of the methyltransferases in the yeast phosphatidylethanolamine methylation pathway by selective gene disruption. Eur J Biochem 1989; 185: 243–251
- McGraw P, Henry SA. Mutations in the Saccharomyces cerevisiae opi3 gene: Effects on phospholipid methylation, growth and cross-pathway regulation of inositol synthesis. Genetics 1989; 122: 317–330
- Summers EF, Letts VA, McGraw P, Henry SA. Saccharomyces cerevisiae cho2 mutants are deficient in phospholipid methylation and cross-pathway regulation of inositol synthesis. Genetics 1988; 120: 909–922
- Tuller G, Nemec T, Hrastnik C, Daum G. Lipid composition of subcellular membranes of an FY1679-derived haploid yeast wild-type strain grown on different carbon sources. Yeast 1999; 15: 1555–1564
- De Antoni A, Gallwitz D. A novel multi-purpose cassette for repeated integrative epitope tagging of genes in Saccharomyces cerevisiae. Gene 2000; 246: 179–185
- Sikorski RS, Hieter P. A system of shuttle vectors and yeast host strains designed for efficient manipulation of DNA in Saccharomyces cerevisiae. Genetics 1989; 122: 19–27
- Daum G, Bohni PC, Schatz G. Import of proteins into mitochondria. Cytochrome b2 and cytochrome c peroxidase are located in the intermembrane space of yeast mitochondria. J Biol Chem 1982; 257: 13028–13033
- de Kroon AI, Koorengevel MC, Goerdayal SS, Mulders PC, Janssen MJ, de Kruijff B. Isolation and characterization of highly purified mitochondrial outer membranes of the yeast Saccharomyces cerevisiae (method). Mol Membr Biol 1999; 16: 205–211
- Larsson K, Ansell R, Eriksson P, Adler L. A gene encoding sn-glycerol 3-phosphate dehydrogenase (NAD + ) complements an osmosensitive mutant of Saccharomyces cerevisiae. Mol Microbiol 1993; 10: 1101–1111
- Jascur T. Import of precursor proteins into yeast submitochondrial particles. Methods Cell Biol 1991; 34: 359–368
- Kushnirov VV. Rapid and reliable protein extraction from yeast. Yeast 2000; 16: 857–860
- de Kroon AI, Dolis D, Mayer A, Lill R, de Kruijff B. Phospholipid composition of highly purified mitochondrial outer membranes of rat liver and Neurospora crassa. Is cardiolipin present in the mitochondrial outer membrane?. Biochim Biophys Acta 1997; 1325: 108–116
- Boumann HA, Gubbens J, Koorengevel MC, Oh CS, Martin CE, Heck AJ, Patton-Vogt J, Henry SA, de Kruijff B, de Kroon AI. Depletion of phosphatidylcholine in yeast induces shortening and increased saturation of the lipid acyl chains: Evidence for regulation of intrinsic membrane curvature in a eukaryote. Mol Biol Cell 2006; 17: 1006–1017
- Gaigg B, Simbeni R, Hrastnik C, Paltauf F, Daum G. Characterization of a microsomal subfraction associated with mitochondria of the yeast, Saccharomyces cerevisiae. Involvement in synthesis and import of phospholipids into mitochondria. Biochim Biophys Acta 1995; 1234: 214–220
- Burri L, Vascotto K, Gentle IE, Chan NC, Beilharz T, Stapleton DI, Ramage L, Lithgow T. Integral membrane proteins in the mitochondrial outer membrane of Saccharomyces cerevisiae. Febs J 2006; 273: 1507–1515
- Zahedi RP, Sickmann A, Boehm AM, Winkler C, Zufall N, Schonfisch B, Guiard B, Pfanner N, Meisinger C. Proteomic analysis of the yeast mitochondrial outer membrane reveals accumulation of a subclass of preproteins. Mol Biol Cell 2006; 17: 1436–1450
- Bunoust O, Devin A, Averet N, Camougrand N, Rigoulet M. Competition of electrons to enter the respiratory chain: a new regulatory mechanism of oxidative metabolism in Saccharomyces cerevisiae. J Biol Chem 2005; 280: 3407–3413
- Pahlman IL, Gustafsson L, Rigoulet M, Larsson C. Cytosolic redox metabolism in aerobic chemostat cultures of Saccharomyces cerevisiae. Yeast 2001; 18: 611–620
- Pahlman IL, Larsson C, Averet N, Bunoust O, Boubekeur S, Gustafsson L, Rigoulet M. Kinetic regulation of the mitochondrial glycerol-3-phosphate dehydrogenase by the external NADH dehydrogenase in Saccharomyces cerevisiae. J Biol Chem 2002; 277: 27991–27995
- Grandier-Vazeille X, Bathany K, Chaignepain S, Camougrand N, Manon S, Schmitter JM. Yeast mitochondrial dehydrogenases are associated in a supramolecular complex. Biochemistry 2001; 40: 9758–9769
- Eisenberg D, Schwarz E, Komaromy M, Wall R. Analysis of membrane and surface protein sequences with the hydrophobic moment plot. J Mol Biol 1984; 179: 125–142
- Cuff JA, Barton GJ. Application of multiple sequence alignment profiles to improve protein secondary structure prediction. Proteins 2000; 40: 502–511
- Amler E, Jasinska R, Drahota Z, Zborowski J. Membrane lateral pressure as a modulator of glycerol-3-phosphate dehydrogenase activity. FEBS Lett 1990; 271: 165–168
- Nalecz MJ, Zborowski J, Famulski KS, Wojtczak L. Effect of phospholipid composition on the surface potential of liposomes and the activity of enzymes incorporated. Eur J Biochem 1980; 112: 75–80
- Walz AC, Demel RA, de Kruijff B, Mutzel R. Aerobic sn-glycerol-3-phosphate dehydrogenase from Escherichia coli binds to the cytoplasmic membrane through an amphipathic alpha-helix. Biochem J 2002; 365: 471–479
- Esser K, Jan PS, Pratje E, Michaelis G. The mitochondrial IMP peptidase of yeast: Functional analysis of domains and identification of Gut2 as a new natural substrate. Mol Genet Genomics 2004; 271: 616–626
- Hofmann K, Stoffel W. TMbase – a database of membrane spanning proteins segments. Biological Chem Hoppe-Seyler 1993; 374: 166
- Cserzo M, Wallin E, Simon I, von Heijne G, Elofsson A. Prediction of transmembrane alpha-helices in prokaryotic membrane proteins: the dense alignment surface method. Protein Eng 1997; 10: 673–676
- Dosztanyi Z, Magyar C, Tusnady GE, Cserzo M, Fiser A, Simon I. Servers for sequence-structure relationship analysis and prediction. Nucleic Acids Res 2003; 31: 3359–3363
- Ikeda M, Arai M, Lao DM, Shimizu T. Transmembrane topology prediction methods: A re-assessment and improvement by a consensus method using a dataset of experimentally-characterized transmembrane topologies. In Silico Biol 2002; 2: 19–33
- Viklund H, Elofsson A. Best alpha-helical transmembrane protein topology predictions are achieved using hidden Markov models and evolutionary information. Protein Sci 2004; 13: 1908–1917
- Cao B, Porollo A, Adamczak R, Jarrell M, Meller J. Enhanced recognition of protein transmembrane domains with prediction-based structural profiles. Bioinformatics 2006; 22: 303–309
- Tusnady GE, Simon I. Principles governing amino acid composition of integral membrane proteins: application to topology prediction. J Mol Biol 1998; 283: 489–506
- Tusnady GE, Simon I. The HMMTOP transmembrane topology prediction server. Bioinformatics 2001; 17: 849–850
- Altschul SF, Gish W, Miller W, Myers EW, Lipman DJ. Basic local alignment search tool. J Mol Biol 1990; 215: 403–410
- Dowd SR, Bier ME, Patton-Vogt JL. Turnover of phosphatidylcholine in Saccharomyces cerevisiae. The role of the CDP-choline pathway. J Biol Chem 2001; 276: 3756–3763
- Jiang F, Gu Z, Granger JM, Greenberg ML. Cardiolipin synthase expression is essential for growth at elevated temperature and is regulated by factors affecting mitochondrial development. Mol Microbiol 1999; 31: 373–379
- Boumann HA, Chin PT, Heck AJ, De Kruijff B, De Kroon AI. The yeast phospholipid N-methyltransferases catalyzing the synthesis of phosphatidylcholine preferentially convert di-C16:1 substrates both in vivo and in vitro. J Biol Chem 2004; 279: 40314–40319
Appendix
Calculation of the secondary structure propensities of Gut2 for membrane interaction
The release of Gut2 from mitoplasts upon carbonate wash, as observed in this study, indicates that Gut2 is a peripheral membrane protein. This contrasts the results from Triton X-114 extraction Citation[32] discussed above. In addition, it contrasts the results of calculations with the transmembrane (TM) prediction tool TMpred Citation[43], Citation[44], and with the dense alignment surface (DAS) algorithm Citation[32], Citation[45], which indicate that Gut2 is an integral membrane protein with two TM helices. We have reanalyzed the occurrence of TM segments in Gut2 by the TMpred and DAS methods and observed that they do not agree on the position and on the length of the proposed TM helices (data not shown). Moreover, it is known that well-established globular proteins are often classified as integral membrane proteins by TM prediction tools Citation[46]. Therefore, we analyzed the primary structure of Gut2 using methods that are more reliable than TMpred and DAS Citation[47], Citation[48] and a newly developed method, MINNOU Citation[49]. The PRO-TMHMM Citation[48], HMMTOP Citation[50], Citation[51]) and MINNOU Citation[49] algorithms did not propose any TM helices in Gut2 (data not shown), in agreement with the conclusion from the carbonate extraction that Gut2 is a peripheral membrane protein. To improve the accuracy of PRO-TMHMM and HMMTOP Citation[48], Gut2 was also analyzed together with its homologues, obtained by a BLAST search (E-value ≤10−5Citation[52]) in the non-redundant SwissProt database. Again no TM helices were found.
Many extrinsic membrane proteins interact with membranes via amphipathic helices. A method to predict amphipathic helices based on the amino acid sequence is by plotting the mean hydrophobic moment of amino acids vs. their mean hydrophobicity, using a window of 11 residues as has been described Citation[38]. As shown in , Gut2 contains several potential amphipathic helices according to this method.
Figure 6. Hydrophobic moment plot of Gut2. For a sliding window of 11 residues the mean hydrophobic moment was calculated and plotted vs. its mean hydrophobicity. In case the hydrophobic moment exceeds a threshold (the oblique line), these 11 residues are proposed to be part of an amphipathic helix as indicated by the squares.
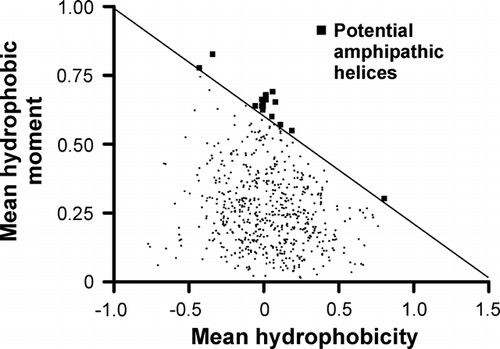
Using the secondary structure prediction program Jpred Citation[39], it was checked whether the candidate sequences were likely to adopt an α-helical structure. This narrowed down the number of potential amphipathic helices to the three depicted in .
Figure 7. Helical wheel representation of potential amphipathic helices in Gut2. The length and direction of the arrow represent the hydrophobic moment of the indicated 11 residues. The N-terminal amino acids in these windows are depicted in the grey boxes.
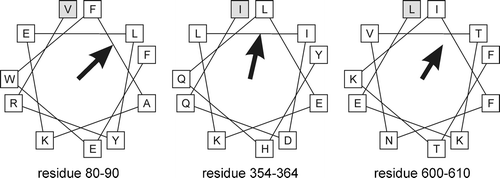
These calculations indicate that Gut2 contains amphipathic helices that could interact hydrophobically with the membrane, in agreement with the experimental data.